Abstract
Colletotrichum lindemuthianum is a fungus that causes bean anthracnose. This study analyzed the virulence and genetic variability among and within C. lindemuthianum isolates occurring in bean-growing areas of southern Ethiopia. Symptomatic common bean leaves and pods were collected from southern Ethiopia. Forty single-spore isolates obtained from the bean leaves and pods were used for this study. The isolates were grouped into 31 physiological races based on their responses to a differential set of common beans. Five of the physiological races (2047, 4031, 2052, 2064, 2174 and 2176) were extremely virulent, causing susceptible reactions on 10 to 11 different cultivars. Rep-polymerase chain reaction revealed high polymorphism, with an average of 87.5% polymorphic loci. The mean Na, Ne, He and I were 1.83, 1.465, 0.278 and 0.427, respectively, within C. lindemuthianum races. Analysis of molecular variance (AMOVA) partitioned a larger part of the total genetic variation within populations (80%). Our collection includes highly virulent races that infected the differential cultivars G2333, Perry marrow and Pi207262 as a possible source of resistance. The differential cultivar G2333 showed strong resistance and can be a potential source of resistance, while the differential cultivars Perry marrow and Pi207262 would be alternative donor parents as sources of resistance in breeding programs for the development of varieties with broader resistance. This study could be a scientific basis for disease prevention and for development of common bean cultivars resistant to bean anthracnose. Identification of a wider range of isolates must be carried out, collecting common bean in different seasons.
Introduction
Common bean (Phaseolus vulgaris L.) is a basic component of the cropping systems of small farmers in Ethiopia. It is an important traditional crop, particularly in the Rift Valley, Hararghe Highlands, and Southern Region of the country. It plays a vital role in the national economy and is used as a food and income source for smallholder households [Citation1]. However, common bean diseases have become major constraint to the production of this high value crop [Citation2]. Among these diseases, bean anthracnose caused by the fungus Colletotrichum lindemuthianum (Sacc. & Magnus) is the major one, causing up to 100% common bean yield loss [Citation2–4].
The pathogenicity of C. lindemuthianum isolates has been reported from different parts of the world. The highest pathogenic variation of C. lindemuthianum was observed in the Mesoamerican and Andean regions, which are thought to be the centers of origin of its host [Citation5]. The Mesoamerican pathotypes of C. lindemuthianum have a wider virulence range and primarily attack small-seeded Mesoamerican cultivars, while the Andean pathotypes have a narrower virulence range and primarily attack large-seeded common bean cultivars of Andean origin [Citation6].
C. lindemuthianum shows wide variability. Therefore, the possibility for newly emerging virulent races poses a challenge for bean breeders [Citation7], resulting in the breakdown of resistance in commercial cultivars [Citation4, Citation8, Citation9] and making the development of resistant cultivars difficult [Citation10].
Studies on the variability of C. lindemuthianum contribute to our understanding of the variation among the races either at the physiological or molecular level, which supports breeding efforts towards long-term resistance to anthracnose [Citation5, Citation9, Citation10]. The pathogenic variability of C. lindemuthianum has been assessed based on the reaction of the isolate to a standard differential series of 12 common bean genotypes and a binary system according to the position of each cultivar within the series [Citation11]. Different types of molecular markers have been used to investigate the genetic diversity of C. lindemuthianum. Among them, random amplified polymorphic DNAs (RAPDs) [Citation9], amplified fragment length polymorphism (AFLP) [Citation6] and random amplified microsatellites (RAMs) and repetitive DNA sequence based polymerase chain reaction (rep-PCR) [Citation9] were used to elucidate the genetic diversity of C. lindemuthianum from diverse regions of the world. Analysis using a combination of virulence and molecular data leads to a better understanding of the variability present in C. lindemuthianum [Citation12].
C. lindemuthianum has become a devastating pathogen in western and southern parts of Ethiopia, and farmers face a serious challenge [Citation13, Citation14]. In most cases, small-scale farmers in Ethiopia have not been using genetically resistant common bean cultivars, and they grow infected seeds. It would be very critical to have adequate knowledge on the virulence and genetic variability among the isolates of C. lindemuthianum in order for the common bean improvement program to guide the deployment of resistance genes to anthracnose disease [Citation15]. The first step in developing breeding interventions to develop common bean varieties resistant against anthracnose is to understand the virulence and genetic variability in C. lindemuthianum. However, the scarcity of information regarding the pathogenicity and genetic variability of Ethiopian C. lindemuthianum isolates has become a problem for the development of durable anthracnose resistance in common bean cultivars. The main aim of this study was to analyze the virulence and genetic variability among and within C. lindemuthianum isolates occurring in bean-growing areas of southern Ethiopia.
Materials and methods
Sample collection
Common bean leaves and pods showing typical anthracnose symptoms (small, reddish-brown, slightly sinking spots form on the pods and leaf veins) were collected from the Gofa, Areka and Hawassa areas () during the main cropping season of 2021. The areas were selected purposefully based on the intensity of bean production and the presence of bean anthracnose disease [Citation16]. The symptomatic leaves and pods were randomly sampled from common bean fields at each location. The collected samples were placed in paper bags and transported to the Molecular Biotech Lab at the Southern Agricultural Research Institute, Hawassa, Ethiopia.
Isolation of C. lindemuthianum from symptomatic samples
Potato Dextrose Agar (PDA) medium was prepared by mixing 39 g of PDA (Acumedia, Baltimore, USA) with 1 L distilled water and autoclaving at 120 °C for 20 min. To restrict bacterial growth, two drops of an antibiotic (rifampicin) were added, mixed carefully, and poured into sterilized Petri dishes and left for 24 h. Leaves and pods of symptomatic common bean samples were cut into small pieces (2–3 cm) and surface sterilized according to the method described by Kiryowa et al. [Citation17]. Each tissue was placed into a small beaker and 10 mL of sodium hypochlorite bleach was added, stirred for 2 min. Then, 10 mL ethanol was added and removed 2 min later. The tissue was rinsed with sterilized distilled water. A small portion of conidia present in the lesions of infected, surface-sterilized plant tissue was transferred to the clean PDA medium, followed by incubation in darkness at 22–24 °C for 10 days. The colonies had gray morphology, and some had dirty white color (). The developed C. lindemuthianum colonies were identified under a microscope for the development of a pure culture of C. lindemuthianum. Forty pure culture isolates had typical cultural and morphological characteristics of C. lindemuthianum, according to Schwartz [Citation18].
Inoculum preparation
PDA plates were flooded with 5 mL of sterile distilled water, the surface was scraped with a sterile spatula, and the spores of C. lindemuthianum pure culture isolates were filtered through three layers of cheese cloth to get enough fungal spore concentration for further testing, according to the techniques described by Kiryowa et al. [Citation17]. The concentration of the spore suspension was prepared for each isolate and adjusted to 1.2 × 106 spores/mL using a hemocytometer according to the method described by Sharma et al. [Citation19].
Differential cultivars
Virulence of C. lindemuthianum was determined using a set of 12 internationally recognized anthracnose race-differential common bean cultivars [Citation11] obtained from the Hawassa bean improvement program. The standard cultivars that were used for race classification comprised two gene pools: four Andean and eight Mesoamerican origins [Citation17]. The designated binary code and characteristics of the standard cultivars [Citation20] are presented in . From each differential cultivar, two seeds were grown in plastic pots containing a mixture of soil, sand and organic manure in an air-conditioned greenhouse at 18–22 °C for 21 days.
Table 1. Standard differential cultivars used to characterize Colletotrichum lindemuthianum, their binary Codes, resistance genes and gene pool.
Inoculation and determining the pathogenicity of C. lindemuthianum
The C. lindemuthianum isolates were inoculated using a "detached leaf" technique [Citation21]. The middle leaflet of the first trifoliate leaves of each differential cultivar was detached from a 21-day-old common bean seedling. The leaflets were inoculated by immersion into 1.2 × 106 mL per liter spore suspension, placing the upper side of the leaf onto cotton moistened with 3.0 mL of tap water in Petri dishes [Citation16]. Detached leaf of each of the deferential cultivars was inoculated with clean distilled water as a control treatment. The pathogenicity was evaluated by observing the reaction of each differential bean cultivar based on a 1–9 scoring system proposed by Balardin et al. [Citation22] for 9 days at an interval of 3 days. After evaluation of all differential cultivars, each isolate was assigned a name (race number) based on the binary nomenclature system by adding the numerical binary values of the susceptible cultivars together as previously described by Keterew et al. [Citation23]. The experimental setup was replicated twice.
Extraction of C. lindemuthianum DNA
C. lindemuthianum mycelium was obtained from the differential common bean cultivars and cultured on 3.9% PDA medium. Actively growing fungal plugs (2–3 cm) were cut from mother cultures using a sterile spatula and placed on fresh PDA medium and left to grow for 10 days on laboratory benches, or until enough mycelium was obtained. The mycelium was harvested by filtration. Genomic DNA was extracted according to a protocol described by Rezene et al. [Citation16]. Fresh fungal mycelia were gently scraped from the surface of single spore cultures grown on PDA medium and transferred into sterilized 2 mL microcentrifuge tubes. The samples were suspended in 500 μL fresh extraction buffer solution (0.2 mol/L Tris-HCl pH 8.0, 10 mmol/L EDTA pH 8.0, 0.5 M NaCl, 1% CTAB, 1% SDS) and then incubated in a water bath at 65 °C for 30 min. The suspension was mixed vigorously with one volume of chloroform: isoamylalcohol (24:1) and centrifuged for 15 min at 20,800 g. The supernatant was transferred to a new tube; ice cold isopropanol was added, and the samples were centrifuged for 10 min at 20,800 g. The pellet was washed with 70% cold ethanol, air dried and suspended in 100 µL TE buffer. DNA concentrations were determined spectrophotometrically using Nanodrop-2000 and finally diluted to 20 ng µL−1.
Repetitive DNA sequence-based PCR (rep-PCR) amplification
From the three families of repetitive sequences, repetitive extragenic palindromic (REP), the most used, sequence was used for rep-PCR analysis [Citation24]. Two types of rep-primer sequences, rep1 R-I 5′ III CGI CGI CAT CIG GC 3′ and rep2 R-I 5′ ICG ICT TAT GIG GCC TAC 3′) [Citation25] were used for PCR amplification of C. lindemuthianum isolates DNA. The PCR amplifications were performed with a thermal cycler (Applied Biosystems). The reactions were carried out in a 25 µL reaction volume, containing 4 µL forward and reverse primers (2 µL each), 19 µL molecular grade water, 2 µL extracted DNA sample, and a PCR bead (pre-mixed chemicals containing each dNTP, 10x PCR buffer, Taq DNA polymerase, and MgCl2 (Eillustra, Ready-To-Go, Healthcare companies, http//www.gehealthcare.com/illustra). The amplification program for the primer sets used was as described by Barcelos et al. [Citation10]. Denaturation at 94 °C for 2 min, primer annealing at 37 °C for 15 s, primer extension at 72 °C for 1 min, followed by final extension at 72 °C for 2 min. The PCR product was run by preparing an agarose gel (2%) using Tris-acetate-EDTA buffer. The rep-PCR profiles and fingerprinting patterns were visualized using ethidium bromide under UV light. The data were recorded as 1 (present) or 0 (absent) for the DNA band. Faint bands were not considered for data analysis.
Statistical analysis
The pathogenic variability of C. lindemuthianum was identified according to the resistance or susceptibility reaction patterns of the differential cultivars. The genetic diversity parameters, which include the number of polymorphic loci (No. of PPL), the percentage of polymorphic loci (%PPL), the observed number of alleles (Na) and the effective number of alleles (Ne), Nei’s [Citation26] gene diversity (He), and Shannon’s information index of diversity (I) Lewontin [Citation27], were calculated by GenAlEx version 6.1 [Citation28]. Analysis of molecular variance (AMOVA) was performed using the same program to assess genotypic variations across the populations. The genetic clustering was assessed by performing a principal coordinate analysis (PCoA) using the same program. A dendrogram was constructed using neighbor-joining algorithms [Citation29] by MEGA 6 [Citation30] to examine the genetic relationships among individuals.
Results
Colletotrichum lindemuthianum races
A total of 31 pathotypes of C. lindemuthianum were identified. Nineteen races (312, 314, 944, 952, 963, 1402, 1971, 2042, 2047, 2052, 2064, 2174, 2176, 2194, 3200, 3204, 3461, 3848 and 4031) were isolated from Hawassa collection; ten races (512, 1280, 2560, 3200, 3586, 3592, 3618, 3625, 3868 and 3996) were isolated from Areka collection and two races (0 and 2112) were isolated from Gofa collections (). Race 3586, found from two Areka isolates (etar-043 and etar-049), infected three differential cultivars. Races 4031 and 2047 were extremely virulent, eliciting a susceptible response in 11 different cultivars. Race 2042 was virulent on nine differential cultivars, while races 3996 and 1971 were virulent on eight differential cultivars. Five races were virulent on only bean cultivars of Middle-American origin, whereas 15 races infected both Andean and Mesoamerican bean cultivars. There was no race that was only virulent to Andean differential cultivars. Most of the differential cultivars were resistant to the C. lindemuthianum race 512 except TU. One race that did not infect both Andean and Mesoamerican bean cultivars was subsequently classified as race 0. This race most frequently appeared at the Gofa location, and all sets of bean differentials were resistant to it.
Table 2. Race designation, number of isolates and susceptible (S) and resistant (R) reactions of common bean differential cultivars to 31 virulence races of Colletotrichum lindemuthianum identified from 40 isolates collected from Southern common bean growing area.
Pathogenic variability in C. lindemuthianum races
Based on the resistance reactions to the 31 races in the set of 12 differential cultivars, C. lindemuthianum isolates showed high pathogenic variability in the areas from which they were collected (). Nine physiological races (2112, 2560, 3586, 3592, 3618, 3625, 3868, 3996 and 4031) caused susceptible reactions on a differential cultivar, G2333. These races were able to infect the highly resistant genotype with resistance genes Co-42, Co-52 and Co-7 and were identified as highly virulent races that could potentially cause extensive damage to the common bean production. The differential cultivar G2333 showed the strongest resistant reaction (0.68) against 27 physiological races. This was followed by the cultivars Perry Marrow (20 races), Michelite and Mexico 222, which were each resistant to 17 and 16 races, respectively. M.D.R.K.* and Pi207262 showed resistant reactions to 12 and 13 races, respectively.
Figure 3. Frequency of resistance reaction of 12 differential common bean cultivars using 40 Colletotrichum lindemuthianum isolates collected from southern Ethiopia.
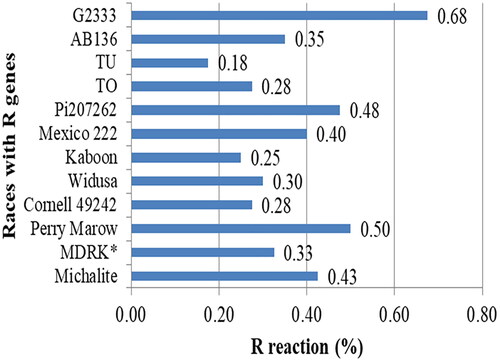
Differential cultivars carrying the alleles Co-42, Co-52, Co-7 (G2333), Co-13 (Perry Marow), Co-43 Co-9 (Pi207262) showed the highest resistance to anthracnose disease in the study area, with the highest resistance percent frequency values of 68, 50 and 48%, respectively. The Mexico222 and Michalite strains are moderately resistant to C. lindemuthianum pathotypes. The differential cultivars TU and Kaboon were the most susceptible cultivars with R reactions of 0.18 and 0.25, respectively, while the differential cultivars G2333, Perry marrow and Pi207262 were the most resistant cultivars with R reaction values of 0.68, 0.50 and 0.48, respectively ().
Genetic variability of C. lindemuthianum
The genomic DNA patterns found among the C. lindemuthianum isolates varied in size depending on each of the C. lindemuthianum isolates. The isolates located on lane 1 displayed different profiles, and lanes 6, 7, 8, 9 and 10 did not appear to share common bands (). However, common bands could be identified by similar strains (lanes 2, 3, 4, and 5, 13 and 14, and lanes 15 and 16).
Figure 4. Banding patterns of some Colletotrichum lindemuthianum isolates collected from common bean genotypes and generated by rep-PCR. Lane M is 100 bp DNA molecular size marker (Cleaver Scientific DNA Marker CSL-MDNA-100BP for Gel Electrophoresis 100–1500 bp, https://www.reagecon.com/en-gb/cleaver-scientific-dna-marker-csl-mdna-100bp-for-6256961); Lanes 1–20 represent of C. lindemuthianum isolates etar-041, eta-002, etar-043, etar-049, etar-045, eta-001, etar-042, etar-044, eta-083, eta-009, eta-007, eta-081, eta-003, eta-006, etg-068, etar-047, eta-010, etg-066, etar-046 and eta-080, respectively.
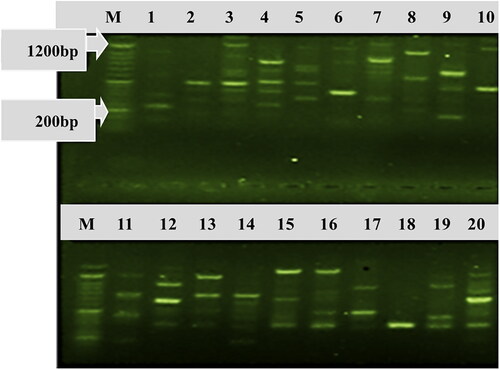
Genetic diversity of C. lindemuthianum
The rep primers based on rep-PCR resulted in a total of 24 loci, with each population having 8 loci. Of which, 21 (87.5%) were polymorphic. The amount and percentage of polymorphic loci (%PPL) within populations ranged from 6 (75% in Areka) to 8 (100% in Hawassa), with an average of 7 PPL. The highest value of Na was recorded in Hawassa and Gofa (2.00) isolates, while the lowest Na (1.50) was recorded in Areka isolates. The highest Ne (1.548) was recorded in Hawassa isolates, while the lowest Ne (1.424) was observed in Gofa and Areka isolates. The average gene diversity ranged from He = 0.247 (Areka isolates) to He = 0.324 (Hawassa isolates), with an average of He = 0.278 (). The estimates of the Shannon information index of diversity (I) ranged from I = 0.373 for Areka isolates to I = 0.490 for Hawassa isolates.
Table 3. Genetic diversity parameters indicating the presence of genetic diversity among three Colletotrichum lindemuthianum populations.
Analysis of molecular variance (AMOVA)
Analysis of genetic variation among and within the Hawassa, Areka and Gofa isolates of C. lindemuthianum showed that most of the genetic variations were found within populations (80%), while the remaining 20% were among populations. The average Fst among the three populations was 0.198 ().
Table 4. Analysis of variance (AMOVA) of 40 Colletotrichum lindemuthianum isolates collected from three sites of Southern Ethiopia, indicating genetic variation within and among the isolates.
Cluster analysis
The two-dimensional plot of the PCoA () represented 39.19% and 30.94% (in total, 70.08%) of detected variability. Neighbor joining dendrogram distributed the C. lindemuthianum isolates in 2 clusters: a minor composed of 19 isolates, and a major cluster consisted of 21 isolated (). Most of the isolates from Hawassa and Areka were clustered in the minor cluster. Seven (36.8%) isolates (eta-001, eta-002, et-003, eta-004, eta-005, eta-006, eta-007, eta-008 and eta-009) of minor cluster were Hawassa isolates. The isolates with similar genomic DNA fingerprinting patterns were clustered in the same groups. The major cluster showed clear genotype admixture, regardless of the source populations.
Figure 5. A two-dimensional plot of the Principal Coordinate Analysis (PCoA) of Colletotrichum lindemuthianum isolates from common bean based on rep-PCR data based on populations. Pop1, Pop2 and Pop3 indicate the isolates collected from Hawassa, Areka and Gofa, respectively.
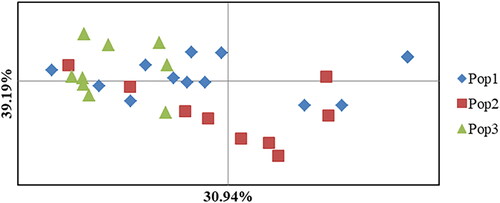
Figure 6. Neighbor-Joining (NJ) tree showing genetic relationships among 40 Colletotrichum lindemuthianum isolates from common bean genotypes. The genogram was generated based on neighbor-joining algorithms [Citation28] by MEGA 6 [Citation29].
![Figure 6. Neighbor-Joining (NJ) tree showing genetic relationships among 40 Colletotrichum lindemuthianum isolates from common bean genotypes. The genogram was generated based on neighbor-joining algorithms [Citation28] by MEGA 6 [Citation29].](/cms/asset/67cbb9fb-1f21-4ada-9b8e-d54a5e9f2301/tbeq_a_2206493_f0006_c.jpg)
Discussion
The study revealed 31 different pathotypes of C. lindemuthianum from 40 isolates (). Among them, there were four races (0, 944, 2047 and 2560) which have been frequently reported from different countries [Citation31, Citation32]. All differential common bean cultivars were resistant to the Race 0 isolate. Similarly, Ansari et al. [Citation6] and Batureine [Citation12] reported that race 0 was not pathogenic and did not cause infection in the differential cultivars. Race 944 was reported in Tanzania [Citation4]. Races 2047 and 2560 were reported in Turkey [Citation33]. Race 2047 was reported in Colombia, Brazil and Uganda [Citation17].
Races 4031 and 2047 were extremely virulent, causing a susceptible reaction on 11 differential cultivars, followed by races 2052 and 2064, which showed a susceptible reaction on 10 differential cultivars. Previously, race 2047 was also reported by different authors as the most aggressive and showed compatible reaction with seven differentials cultivars [Citation9, Citation17, Citation34, Citation35]. Race 512, which carries the Co-5 gene and infects the differentiation cultivar TU, was found to be the least virulent pathotype.
Nine pathotypes, 2112, 2560, 3586, 3592, 3618, 3625, 3868, 3996 and 4031, were identified as highly virulent races on differential cultivar G2333, causing susceptible reactions and significant damage. Gezahegn et al. [Citation36] reported four races (3047, 2260, 2225 and 2073) that were able to infect the highly resistant differential cultivar G2333. Kiryowa et al. [Citation17] and Mwesigwa [Citation37] reported on the susceptibility of cultivar G2333 to two virulent pathogens that were collected in Uganda. This may indicate that the Ethiopian C. lindemuthianum populations might be composed of highly virulent races, although cultivar G2333 was widely reported as a possible source of resistance [Citation4, Citation23, Citation38].
The differential cultivars Perry Marrow and Pi207262 also exhibited more resistant reactions. These cultivars could be recommended as donor parents in breeding programs and are necessary for the development of varieties with durable resistance. Similarly, Gonzaz [Citation37] suggested that the differential cultivar Perry Marrow exhibited resistance to C. lindemuthianum when tested under field conditions. Differential cultivars TU and Kaboon demonstrated low R reactions of 0.18 and 0.25, respectively. Similarly, Gonzaz [Citation38] reported that these differential cultivars had a low number of resistance genes to the fungus C. lindemuthianum. The detected high pathogenic variability of C. lindemuthianum may be due to differences in common bean germplasm and agricultural practices used in different agro-ecologies, and cultivar resistance to pathogens may vary depending on geographic location.
The rep-PCR analysis revealed clearly distinct patterns for C. lindemuthianum isolates and resulted in a total of 24 loci. Of which, 21 (87.5%) showed polymorphism. Common bands that could be identified with similar strains imply genetic similarity among the pathogens. However, there are isolates that were very distinct from each other as reflected by different banding patterns, indicating the presence of high variability among C. lindemuthianum isolates existing in the study areas. Similarly, Mahuku and Riascos [Citation9] and Batureine [Citation12] reported high haplotypic diversity in C. lindemuthianum, which was expected because isolates of the same pathotype might not necessarily be closely related [Citation39].
The highest genetic diversity of C. lindemuthianum (He = 1.548) was recorded in Hawassa isolates rather than Gofa and Areka. This indicates the Hawassa C. lindemuthianum population might be the original population from which the Areka population diverted. However, the He values were lower than the He value in Uganda isolates (He = 326) [Citation12]. The Shannon information index ranged from a mean of 0.373 to 0.490, indicating the presence of abundant genetic diversity among C. lindemuthianum populations. As a result, C. lindemuthianum should be considered for developing durable resistance in bean varieties.
Analysis of molecular variance (AMOVA) partitioned the total genetic variation into within-groups and among-groups, where most of the genetic variations (80%) were distributed within populations, while the remaining 20% were among populations, implying strong genetic differentiation of C. lindemuthianum within populations. The value is comparable to other findings. Batureine [Citation12] reported 88.7% genetic variability within C. lindemuthianum populations and 11.3% among populations. Sicard et al. [Citation40] reported 88.3% and 11.7% genetic variability within and among wild C. lindemuthianum populations, respectively, using RAPD markers. Silva-Damasceno et al. [Citation41] also identified 3.94% and 96.06% of the genetic variability as being among regions and within three regions (populations) of C. lindemuthianum isolates collected from Brazil.
Principal Coordinate Analysis (PCoA) depicted a clear admixture of populations, regardless of the source populations. The first two axes of PCoA accounted for 70.08% of the total variation, showing that C. lindemuthianum isolates collected from different sites clustered together. Cluster analysis also depicted similar result. This agrees with the report of Talamini et al. [Citation42], where C. lindemuthianum from the Zona da Mata and Sul de Minas and was clustered together. Cluster analysis also depicted a similar result. The exchange of infected seeds with C. lindemuthianum may have contributed to the grouping of isolates from different common bean-growing areas. A high degree of shared similarity between geographically isolated populations is evidence of gene flow among populations [Citation43]. This could be due to the coexistence of different host genotypes, the use of an informal seed system by small-scale farmers, the movement of infected agricultural instruments between different locations in common bean-growing areas, and the fact that humans and insects may play a significant role in spore dispersal, resulting in the distribution of C. lindemuthianum in different geographical areas. The common bean seed system in Ethiopia is dominated by an informal seed system, and bean-growing farmers can have the chance of growing infected planting material, while farmers in the southern regions have experience exchanging seeds as planting material [Citation44]. The observed similarity of C. lindemuthianum isolates collected from Hawassa and Areka () may be related to the geographical localization. Isolates found in the same place may have a higher chance of harboring the same genetic material [Citation45].
Conclusions
Here we report the pathogenic and genetic variability of C. lindemuthianum in the southern region of Ethiopia with the aim of understanding the virulence and variability of C. lindemuthianum. The physiological variation in C. lindemuthianum was revealed on the basis of 31 pathotypes, which were defined by standard bean differential cultivars. Races 4031 and 2047 were extremely virulent and aggressive enough to cause a susceptible reaction. The differential cultivar G2333 showed strong resistance to most of the isolates and can be used as a potential source of resistance in the breeding program. The two common bean differential cultivars, Perry Marrow and Pi207262, are promising sources of resistance to be used as alternative parental lines for developing anthracnose resistance in the study area. In rep-PCR-based analysis, the majority of C. lindemuthianum isolates revealed polymorphic loci (87.5%) and high genetic diversity in C. lindemuthianum (average He = 0.278). Overall, this study generated useful information for designing anthracnose management strategies and for the selection and development of resistant common bean varieties that can help farmers increase their income by producing disease-free and high-yielding varieties resistant to C. lindemuthianum.
Authors’ contributions
All authors made a significant contribution to the work reported, whether that was in the conception, study design, execution, acquisition of data, analysis, or interpretation. YR conceived and designed the study. NH, EW and YR conceptualized the research work and were actively involved in the research investigation, documentation and publication process. NH drafted the first version of the manuscript. EW prepared the final draft of the manuscript. All authors read and approved the final manuscript, and agreed on the journal to which the article has been submitted.
Acknowledgments
The authors would like to thank the Southern Agricultural Research Institute (SARI) Kirkhouse Trust project for allowing materials in Molecular Biotech Laboratory and Wolaita Sodo University for Financial support. The authors would also like to thank Aklilu Banjaw (Department of Biotechnology, Wolkite University) for the support given to the first author at different stages of the study.
Data availability statement
All data that support the findings reported in this study are available from the corresponding author [EW] upon reasonable request.
Disclosure statement
The authors confirmed that there is no conflict of interest.
Additional information
Funding
References
- Teshome A, Amsalu B, Gabrekiristos E. On-farm improved common bean variety demonstration and evaluation in Central Rift Valley of Ethiopia: case of bora, Adami-Tullu, jido and kombolcha districts. Ethiop J Crop Sci. 2022;9:1–10.
- Gabrekiristos E, Wondimu M. Emerging and reemerging diseases of common bean (Phaseolus vulgaris L.) in major production areas: in the case of Ethiopia. J Agric Sci. 2022;14(4):19–34.
- Gonçalves-Vidigal MC, Meirelles AC, Poletine JP, et al. Genetic analysis of anthracnose resistance in ‘pitanga’ dry bean cultivar. Plant Dis. 2012;131(3):423–429.
- Mpeguzi M, Susan NM, Mabagala R, et al. Races of C. lindemuthianum (sacc. & magnus) Briosi & cavara in major bean growing regions in tanzania. Afr J Plant Sci. 2020;14(8):308–314.
- Pastor-Corrales MA, Otoya MM, Molina A. Resistance to colletotrichum lindemuthianum isolates from Middle america and andean South america in different common bean races. Plant Dis. 1995;79(1):63–67.
- Ansari KI, Palacios N, Araya C, et al. Pathogenic and genetic variability among colletotrichum lindemuthianum isolates of different geographic origins. Plant Pathol. 2004;53(5):635–642.
- Mahuku GS, Jara CE, Cajiao C, et al. Sources of resistance to C. lindemuthianum in the secondary gene Pool of Phaseolus vulgaris and in crosses of primary and secondary gene pools. Plant Dis. 2002;86(12):1383–1387.
- Kelly JD, Afanador L, Cameron L. New races of colletotrichum lindemuthianum in Michigan and implications in dry bean resistance breeding. Plant Dis. 1994;78(9):892–894.
- Mahuku SG, Riascos JJ. Virulence and molecular diversity within C. lindemuthianum isolates from andean and mesoamerican bean varieties and regions. European J Plant Pathol. 2004;110(3):253–263. 10.1023/B:EJPP.0000019795.18984.74.
- Barcelos Q, Souza E, Silva KJD. Vegetative compatibility and genetic analysis of colletotrichum lindemuthianum isolates from Brazil. Genet Mol Res. 2011;10(1):230–242.
- Pastor-Corrales MA. Standardization of differential varieties and designation of races of colletotrichum lindemuthianum. Phytopatholoy. 1991;81(6):694.
- Batureine MJ. Diversity of Colletotrichum lindemuthianum and reaction of common bean germplasm to anthracnose disease MSc thesis presented to the school of graduate studies of makerere university 2009.
- Meziadi C, Richard MS, Derquennes A, et al. Development of molecular markers linked to disease resistance genes in common bean based on whole genome sequence. Plant Sci. 2016;242:351–357.
- BARC. Crop protection division progress report., Bako Research Center, Bako, Ethiopia. 2011.
- Rezene Y, Genome Wide Marker Trait Association Study, Molecular Characterization and Pathogenic Variability among Pseudocercospora griseola (Sacc.) Crous & Braun, Isolates the Causal Agent of Angular Leaf Spot Disease of Common Bean in Ethiopia. Doctoral Dissertation, Department of Cellular Microbial and Molecular Biology, Addis Ababa University. 2018.
- Rezene Y, Tesfaye K, Clare M, et al. Rep-PCR genomic fingerprinting revealed genetic diversity and population structure among ethiopian isolates of pseudocercospora griseola pathogen of the common bean (Phaseolus vulgaris L.). J Plant Pathol Microbiol. 2018;9(11):1–9.
- Kiryowa MJ, Ebinu A, Kyaligonza V, et al. Pathogenic variation of colletotrichum lindenuthianum causing anthracnose of beans (Phaseolus vulgaris) in Uganda. Int. J. Phytopathol. 2017;5(3):89–98.
- Schwartz HF. Anthracnose. In: hall, E. Compendium of bean diseases. St Paul, APS Press, 1991. pp. 16–17.
- Sharma N, Kumari N, Sharma SK, et al. Investigating the virulence and genetic diversity of colletotrichum lindemuthianum populations distributed in the North Western himalayan hill states. J Plant Pathol. 2019;101(3):677–688.
- Kelly JD, Vallejo VA. A comprehensive review of the major genes conditioning resistance to anthracnose in common bean. HortSci. 2004;39(6):1196–1207.
- Tu JC. A detached leaf technique for screening beans (Phaseolus vulgaris L.) in vitro against anthracnose (colletotrichum lindemuthianum. Can. J. Plant Sci. 1986;66(3):805–809.) https://cdnsciencepub.com/doi/10.4141/cjps86-100
- Balardin RS, Kelly JD. Interaction between colletotrichum lindemuthianum races and gene Pool diversity in Phaseolus vulgaris. jashs. 1998;123(6):1038–1047.
- Keterew Y, Dawit W, Thangavel S, et al. Physiological characterization and evaluation of common bean genotypes against anthracnose (colletotrichum lindemuthianum (sacc. and magnus) Lams-Scriber) races in west shewa. Ethiopia. Int J Life Sci. 2018;6(3):744–754.
- Versalovic J, de Bruijn FJ, Lupski JR. Repetitive sequence-based PCR (rep-PCR) DNA fingerprinting of bacterial genomes. In: de Bruijn FJ ,Lupski JR, Weinstock GM (eds) Bacterial genomes. Springer, Boston. 1998.
- De Bruijn FJ. Use of repetitive (repetitive extragenic palindromic and enterobacterial repetitive intergeneric consensus) sequences and the polymerase chain reaction to fingerprint the genomes of Rhizobium meliloti isolates and other soil bacteria. Appl Environ Microbiol. 1992;58(7):2180–2187.
- Nei M. Analysis of gene diversity in subdivided populations. Proc Natl Acad Sci U S A. 1973;70(12):3321–3323.
- Lewontin RC. Testing the theory of natural selection. Nature. 1972;236(5343):181–182.
- Peakall R, Smouse PE. GENALEX 6.1: genetic analysis in excel. Population genetic software for teaching and research. Mol Ecol Notes. 2006;6(1):288–295.
- Saitou N, Nei M. The NJ method: a new method for reconstructing phylogenetic trees. Mol Biol Evol. 1987;4(4):406–425.
- Tamura K, Stecher G, Peterson D, et al. MEGA6: molecular evolutionary genetics analysis version 6.0. Mol Biol Evol. 2013;30(12):2725–2729.
- González-Chavira M, Rodríguez-Guerra R, Hernández-Godínes F, et al. Analysis of pathotypes of colletotrichum lindemuthianum found in the Central region of Mexico and resistance in elite germplasm of Phaseolus vulgaris. Plant Dis. 2004;88(2):152–156. 1094/PDIS.2004.88.2.152
- Sharma PN, Padder BA, Sharma OP, et al. Pathological and molecular diversity in colletotrichum lindemuthianum (bean anthracnose) across Himachal Pradesh, a North-Western himalayan state of India. Austral. Plant Pathol. 2007;36(2):191–197.
- Padder BA, Sharma PN, Awale HE, et al. Colletotrichum lindemuthianum, the causal agent of bean anthracnose. J Plant Pathol. 2017;99(2):317–330.
- Madakbas SY, Hiz CM, Kucukyan S, et al. Transfer of Co-1 gene locus for anthracnose disease resistance to fresh bean (Phaseolus vulgaris L.) through hybridization and molecular marker-assisted selection (MAS). JAS. 2013;5(4):94–102.
- Castro SA, Gonçalves-Vidigal MC, Gilio TAS, et al. Genetics and mapping of a new anthracnose resistance locus in andean common bean paloma. BMC Genomics. 2017;18(1):306.
- Gezahegn A, Chala A, Ayana G. Physiological races of colletotrichum lindemuthianum, the cause of bean anthracnose in major bean growing regions of Southern and Central Ethiopia. Eur J Plant Pathol. 2021;15(2):103–114.
- Mwesigwa JB. Diversity of Colletotrichum lindemuthianum and reaction of common bean germplasm to anthracnose disease. PhD thesis Makerere University, Uganda, 2009.
- Gonzaz KK. Identification of Colletotrichum lindemuthianum and introgression of its resistance gene(s) to common bean (Phaseolus vulgaris L.) adapted in Tanzania. MSc thesis presented to the School of Graduate studies of Sokoine University, Tanzania, 2016.
- Ddamulira G. Characterization of Pseudocercospora griseola, identification of local sources of resistance and effectiveness of gene pyramiding in controlling angular leaf spot in common bean. A PhD Thesis, Makerere University Kampala, Uganda, 2015.
- Sicard D, Buchet S, Michalakis Y, et al. Genetic variability of colletotrichum lindemuthianum in wild populations of common bean. Plant Pathol. 1997;46(3):355–365.
- Silva-Damasceno KJ, Souza EA, Ishikawa FH. Characterization of colletotrichum lindemuthianum isolates from the state of Minas Gerais, Brazil. J Phytopathol. 2007;155(4):241–247.
- Talamini V, Souza EA, Pozza EA, et al. Genetic divergence among and within colletotrichum lindemuthianum races assessed by RAPD. Fitopatol. bras. 2006;31(6):545–550.
- Marulanda ML, López AM, Isaza L, et al. Microsatellite isolation and characterization for colletotrichum spp., causal agent of anthracnose in andean blackberry. Genet Mol Res. 2014;13(3):7673–7685.
- Zelalem F, Tesfaye K, Dagne K, et al. Genetic diversity and population structure of common bean (Phaseolus vulgaris L) germplasm of Ethiopia as revealed by microsatellite markers. Afr. J. Biotechnol. 2016;15(52):2824–2847.
- Da Silva RB, Valicente FH. Molecular characterization of Bacillus thuringiensis using rep PCR. Springer Plus. 2013;2(1):641.