Abstract
Objectives: Rutin, quercetin-3-O-rutinoside, a natural flavonol glycoside, has shown various in vitro benefits with potential use treating human diseases, especially cardiovascular system disorders. Antioxidant properties are assumed to underlie the majority of these benefits. Yet rutin pro-oxidant properties have been reported as well. Our research group has recently shown aggravating effects on isoprenaline (ISO)-induced cardiotoxicity in Wistar:Han rats after 24 hours.
Methods: This study was designed to examine in more detail the reasons for the negative effects of rutin (11.5 and 46 mg/kg, i.v.) after administration of ISO (100 mg/kg, s.c.) in rats within 2 hours of continuous experiment and in the H9c2 cardiomyoblast-derived cell line.
Results: Like our previous findings, rutin did not (11.5 or 46 mg/kg, i.v.) reduce the ISO-induced mortality within 2 hours although the lower dose significantly reduced cardiac troponin T (cTnT) and partly improved the histological findings. In contrast, the higher dose increased the mortality in comparison with solvent (1.26% w/v sodium bicarbonate). This was not caused by any specific haemodynamic disturbances. It appears to be associated with oxidative stress as rutin enhanced intracellular reactive oxygen species formation in vitro and had the tendency to increase it in vivo.
Conclusions: Rutin, likely due to its pro-oxidative effects, can exacerbate catecholamine cardiotoxicity depending on the dose used.
Introduction
Flavonoids, which are ubiquitous plant polyphenolic secondary metabolites, are an integral part of the human diet.Citation1,Citation2 Recent epidemiological studies have evaluated the relationship between flavonoid intake and benefits in case of various pathologies including those of the cardiovascular system.Citation2–Citation4 The proposed benefits are probably caused by combination of reactive oxygen species (ROS) scavenging activity, iron/copper chelation, inhibition of free radical-forming enzymes, decrease in expression of inflammatory signalling molecules, and other factors like direct vasodilatory and antiplatelet potential.Citation5–Citation11 However, flavonoids can have pro-oxidative effects as well. These could be based on their transient metal-reducing potential and consequent increase in formation of the hydroxyl radical via Fenton reaction.Citation12–Citation14
The endogenous catecholamines are hormones and neurotransmitters in the sympathetic and central nervous systems. However, excessive concentrations can trigger acute myocardial infarction (AMI).Citation15,Citation16 Interestingly, a cardiotoxic dose of the synthetic non-selective β-agonist isoprenaline (isoproterenol, ISO) induces a pathological state which resembles the acute phase of AMI as shown by marked release in cardiac troponin T (cTnT), calcium overload, ST segment (J-point) elevation, R wave amplitude decrease, and histopathological disturbances.Citation17–Citation23 Although the pathogenesis of ISO-induced cardiotoxicity is multifactorial, overstimulation of β-adrenoceptors, auto-oxidation of catecholamines followed by formation of ROS, energy metabolism dysfunction, microthrombi formation, and membrane permeability alterations play substantial roles.Citation16–Citation18,Citation21,Citation24,Citation25
Stanely Mainzen Prince and coworkersCitation26–Citation29 reported that oral pre-treatment with rutin (40 or 80 mg/kg/day, 42 days, p.o.) had broad preventive effects on various aspects of ISO-induced cardiotoxicity (150 mg/kg/day, 2 days, s.c.) in rats. Similarly, administration of quercetin (10 mg/kg/day, 7 days, p.o.) appeared to be protective against cardiotoxic doses of ISO as well (100 mg/kg/day, respectively 85 mg/kg/day, s.c., 2 days).Citation30 The beneficial effects of the flavonols were particularly associated with their antioxidant, lipid-lowering, mitochondrial structure and function improving, and membrane stabilizing properties. However, Stanely Mainzen Prince and coworkers did not show data on rat survival, haemodynamics, arrhythmias after administration of cardiotoxic doses of ISO, and pharmacokinetics of the flavonoids.
On the other hand, our research group found that a single i.v. dose of rutin in rats either had no effect (11.5 mg/kg) or aggravated (46 mg/kg) ISO-induced cardiotoxicity (100 mg/kg, s.c.) after 24 hours.Citation31 Since such effects are in clear contrast to the foregoing studies, we reanalysed the effects of quercetin on ISO-induced cardiotoxicity. We used the same experimental design as Prince and SathyaCitation30 and we evaluated survival, haemodynamic, and ECG variables as well. Surprisingly, we found that oral administration of quercetin did not ameliorate ISO-induced cardiotoxicity.Citation32
We decided to return to the issue of the i.v. administered rutin and to analyse the reason for higher mortality rate in rats receiving rutin as a premedication. This study was aimed at detailed comparison of the early pathophysiological effects of administered rutin (11.5 and 46 mg/kg, i.v.) on ISO-induced cardiotoxicity (100 mg/kg, s.c.) to explain the mechanisms of its direct negative effects.
Material and methods
Chemicals
Rutin hydrate, sodium bicarbonate, ISO hydrochloride, and urethane were purchased from Sigma-Aldrich Inc. (USA). Water for injection was purchased from Braun (Germany). Ultrapure water was produced by Milli-Q RG (Merck Millipore, USA).
In vivo studies using Wistar:Han rats
Wistar:Han male rats (MediTox, Czech Republic) were kept in an air-conditioned room at constant temperature and humidity. They had free access to a standard pellet diet for rodents (Velaz, Czech Republic) and tap water. After 2 weeks of acclimatization, the rats were deprived of food for 12 hours before the experiments.
The study was approved by the Ethics Committee of Charles University in Prague, Faculty of Pharmacy in Hradec Králové (Study No. MŠMT 2437/2012-30), and it conformed to the guide for the care and use of laboratory animals published by The US National Institutes of Health (NIH Publication No. 85-23, revised 1996).
Haemodynamic study
Rats weighing on average 392 g were randomly divided into nine groups:
control groups received i.v. either sodium bicarbonate dissolved in water for injection (1.26% w/v, the solvent) or rutin dissolved in a solution of sodium bicarbonate as a slow bolus: (a) sodium bicarbonate – 2.3 ml/kg (SB2.3, six rats), (b) sodium bicarbonate – 4.6 ml/kg (SB4.6, six rats), (c) rutin – 11.5 mg/kg (2.3 ml/kg, Ru11.5, six rats), and (d) rutin – 46 mg/kg (4.6 ml/kg, Ru46, six rats). The higher dose of rutin was an equimolar dose to the clinical dose of the standard iron chelator – deferoxamine (50 mg/kg) and was chosen to enable comparison with other compounds.
ISO groups received i.v. either a solution of sodium bicarbonate, a solution of rutin, as described above, or saline (S, 4.6 ml/kg), and a freshly prepared solution of ISO dissolved in water for injection 5 minutes later (100 mg/kg, s.c.): (a) SB2.3+ISO (nine rats), (b) SB4.6+ISO (seven rats), (c) Ru11.5+ISO (seven rats), (d) Ru46+ISO (seven rats), and (e) S4.6+ISO (nine rats).
At the end of the experiment, blood samples were collected from the abdominal aorta into a heparinized tube (BD Vacutainer, BD, USA) and surviving rats were sacrificed using 1 M aqueous solution of potassium chloride (i.v., Sigma-Aldrich Inc., USA). Afterwards, the heart was excised and weighed. The wet ventricles weight was expressed as an index, i.e. weight of ventricles in grams divided by body weight in grams, and expressed in per thousand (‰). The apical part of the heart was separated for further histological analysis and the base part was frozen at −20°C for further biochemical analysis as follows.
Biochemical analysis
cTnT, vitamin E, and vitamin C were measured in serum. cTnT was determined using electrochemiluminescence immunoassay which uses two monoclonal antibodies specifically targeted against cTnT (Elecsys 2010, Roche, Czech Republic). After deproteinization, vitamin E was analysed fluorimetrically using the HPLC system LC-10A (Shimadzu, Japan). Vitamin C was determined using capillary electrophoresis followed by UV detection (System P/ACE 5100, Beckman Coulter Inc.).
Frozen samples of the base part of the heart were dried, weighed, and digested by microwave digestion using nitric acid and hydrogen peroxide (Milestone MLS 1200 MEGA, Milestone Corporation, Italy). Calcium was determined photometrically using flame photometry (Efox 5053, Eppendorf, Germany). Copper was analysed using graphite furnace atomic absorption spectrometry (Unicam, Solaar 959, UK) and zinc was determined using flame atomic absorption spectrometry (Solaar 959, Unicam, UK).
Histological analysis
The excised heart ventricles were rapidly fixed in cold 10% buffered neutral formalin solution for at least 24 hours. After fixation, a heart tissue block was processed using the conventional paraffin-embedding technique. Paraffin sections 5–7 μm were stained with haematoxylin–eosin and Goldner's green trichrome.
The heart tissue was fixed by immersion in 3% glutaraldehyde, postfixed in 1% OsO4 (both in phosphate buffer at pH 7.2–7.4), dehydrated in acetone and propylene oxide, and embedded in the resin mixture Durcupan ACM and Epon 812. Semi-thin sections about 1 μm were cut on the ultramicrotome LKB and stained with toluidine blue.
Evaluation of pathological changes included focal cardiomyocyte damage or small multifocal degeneration with mild degree of inflammatory process, extensive myofibrillar degeneration and/or diffuse inflammatory process, and necrosis with diffuse inflammatory process. For the topographic localization of the heart tissue we used a semi-quantitative scale as follows: (−) absence of pathological changes, (+) mild, (++) moderate, and (+++) marked changes. Photo documentation and image digitizing were performed using the light microscope, OLYMPUS AX-70 (Olympus Ltd, Japan), using a digital camera, Pixelink PL-A642 (Vitana Corp., Canada) and an image analysis software, NIS – ELEMENTS AR 3.2 (Laboratory Imaging, Czech Republic).
Glutathione in whole blood
Rats weighing on average 380 g were randomly divided into eight groups of four rats each and the same experimental design as described above, i.e. control groups: (a) SB2.3, (b) SB4.6, (c) Ru11.5, and (d) Ru46; and ISO groups: (a) SB2.3+ISO, (b) SB4.6+ISO, (c) Ru11.5+ISO, and (d) Ru46+ISO.
The rats were anaesthetized using urethane and the left common iliac artery was cannulated for blood withdrawal. After 15 minutes of equilibration, the drug formulation/solvent was administered as a slow i.v. bolus via the saphenous vein using the same infusion rate as described above. ISO was administrated s.c. 5 minutes later if indicated. Whole blood (150 µl) was collected into a heparinized tube (BD Vacutainer, BD, USA) at the predefined time intervals following the administration of ISO (0, 1, 5, 15, 30, 60, and 120 minutes). Immediately after the sampling, 1-methyl-2-vinylpyridinium trifluoromethanesulphonate (Oxis Research, USA) was added to the blood samples for analysis of oxidized glutathione (GSSG) to prevent spontaneous glutathione oxidation. Afterwards, the samples of oxidized and total glutathione (GSHt) were stored at −80°C until analysis.
GSSG, ratio of reduced and oxidized glutathione (GSH/GSSG ratio), and GSHt were determined colorimetrically using the BIOXYTECH GSH/GSSG-412 kit according to the instructions of the manufacturer (Item No. 21040, Oxis Research, USA) and a spectrophotometer Helios Gamma equipped with VisionLite Software 2.2 (ThermoFisher Scientific Inc., USA). The results were calculated as percent of change vs. baseline level (at time 0 minute, before ISO administration).
8-Isoprostane in rat plasma
Rats weighing on average 350 g were randomly divided into four groups of four rats involving only the higher dose regimes and the same order as above: SB4.6, Ru46, SB4.6+ISO, and Ru46+ISO.
The administration of the drug formulation/solvent and whole blood withdrawal followed the protocol described above. Whole blood (150 µl) was collected at the predefined time intervals following the administration of ISO (0, 5, 15, 30, 45, 60, 90, 120, 150, 180, and 240 minutes). Immediately after the sampling, blood was centrifuged (1733 × g, 10 minutes, a centrifuge MPW-52, MPW Med. Instruments, Poland) in the presence of 0.005% tert-butylhydroxytoluene and plasma was stored at −80°C until analysis.
The free fraction of 8-isoprostane was determined in plasma using the 8-isoprostane EIA kit according to the instructions of the manufacturer (Item No. 516351, Cayman Chemical Company, USA) and a spectrophotometer Synergy HT Multi-Detection Microplate Reader (BioTec Instruments Inc., USA). The results were calculated as percent of change vs. baseline level (at time 0 minute, before ISO administration).
Pharmacokinetic study
A rat weighing 525 g was anaesthetized with urethane and cannulated for blood withdrawals in the same way as described above. Afterwards, rutin dissolved under the same conditions as above was administered at a dose of 46 mg/kg as a slow i.v. bolus via the saphenous vein using the same infusion rate as in the pharmacodynamic study. Whole blood (150 µl) was sampled at predefined time intervals following the administration of the drug formulation (0, 1, 5, 15, 30, 45, 60, 120, 180, and 240 minutes). Immediately after the sampling, whole blood was centrifuged (2500 × g, 10 minutes; a centrifuge MPW-52, MPW Med. Instruments, Poland) and plasma was stored at −80°C until analysis.
Rutin concentrations in plasma were assessed using the UHPLC–MS/MS method. This was a system consisting of Acquity UPLC (Waters Corporation, Czech Republic) and Quattro Micro triple quadrupole mass spectrometer (Waters Corporation, Czech Republic). The separation was performed on a BEH Shield RP C18 (2.1 × 100 mm, 1.7 μm) using gradient elution with methanol and 0.1% formic acid. All injected solutions were stored in the autosampler at 4°C. The partial loop with needle overfill mode was set up to inject 5 μl. The analytical column was kept at 40°C by a column oven.
The MS conditions were finely tuned in positive polarity ESI mode as follows: capillary voltage: +3200 V, ion source temperature: 130°C, extractor: 3.0 V, RF lens: 0.5 V. The desolvation gas was nitrogen at a flow 800 l/hour and at 450°C. Nitrogen was also used as a cone gas (100 l/hour) and argon as a collision gas. Analyses were performed in selected reaction monitoring mode using the precursor ion [M + H]+ and the corresponding product ion (610.9 > 303.3). The cone voltage was 20 V, collision energy 25 eV, and the dwell time was 0.2 seconds. MassLynx MS Software 4.1 (Waters Corporation, Czech Republic) was used for MS control and data acquisition. QuanLynx Software (Waters Corporation, Czech Republic) was used for data processing and peak integration. The sample pre-treatment of plasma samples consisted in fast and simple protein precipitation. Plasma (50 μl) was precipitated with 100 μl of acetonitrile. After 10 minutes the samples were centrifuged for 10 minutes. The supernatant was then diluted 200× with a mixture of acetonitrile/water (2:1), filtered through a PTFE membrane with 0.22 μm pores and injected into a UHPLC system.
Pharmacokinetic parameters were calculated by Kinetica 5.1 (Thermo Fisher Scientific Inc., USA) and PKSolver both using the non-compartmental analysis of plasma after the i.v. bolus input and the linear trapezoidal method.Citation35
In vitro studies using H9c2 cell line
The H9c2 cell line derived from embryonic BD1X rat heart tissue was obtained from the American Type Culture Collection (ATCC, USA).Citation36 Cells were cultured in Dulbecco's modified Eagle's medium (DMEM; Lonza, Switzerland) supplemented with 10% heat-inactivated foetal bovine serum (Lonza, Switzerland), 1% penicillin/streptomycin solution (Lonza, Switzerland), and 10 mM HEPES buffer (pH 7.4; Sigma-Aldrich, USA). Cell cultivation was held in 75 cm2 tissue culture flasks from Techno Plastic Products AG (TPP, Switzerland) at 37°C in a humidified atmosphere of 5% CO2 in air. Cells were subcultured twice a week when they reached approximately 90% confluence.
For particular experiments, cells were seeded into appropriate microplates or Petri dishes (TPP, Switzerland) at given cellular density. Twenty-four hours prior to all cellular experiments, the medium was changed for serum-free cell-culture medium (pyruvate-free DMEM, Sigma-Aldrich, USA) supplemented with 1% penicillin/streptomycin solution (Lonza, Switzerland) and 10 mM HEPES buffer (pH 7.4; Sigma-Aldrich, USA). Serum deprivation was used to stop cellular proliferation to mimic the situation in post-mitotic cardiomyocytes.Citation37 Pyruvate was omitted because it is an antioxidant and could interfere with ROS-related toxicity.
Glutathione in H9c2 cell line
H9c2 cells seeded in Petri dishes (Ø150 mm) at a density of 3 000 000 cells/dish were pre-incubated with rutin (1–1000 µM) and ISO (0.1–1000 µM) was added 5 minutes later. After 2-hour co-incubation, cells were washed twice with ice-cold PBS, harvested by scraper, and centrifuged. Supernatants were discarded, pellets of cells were resuspended in 175 µl of PBS, and aliquots were sampled into microtubes, i.e. (a) 100 µl was mixed with 10 µl of 1-methyl-2-vinylpyridinium trifluoromethanesulphonate (Oxis Research, USA) preventing the spontaneous oxidation of glutathione for GSSG assessment; (b) 50 µl was used for GSHt assessment; and (c) 25 µl for protein assessment. Each step was kept on ice. The samples were stored at −80°C until analysis. GSSG, GSH/GSSG ratio, and GSHt were determined in the same way as in whole blood.
2′,7′-Dichlorodihydrofluorescein diacetate assay
To assess intracellular ROS formation, measurement of 2′,7′-dichlorodihydrofluorescein diacetate (H2DCF -DA; Molecular Probes, USA) fluorescence intensity was used. This originally non-fluorescent reagent diffuses passively through the cellular membranes, its acetate groups are metabolized by intracellular esterases and then oxidized by ROS formed inside the cell (particularly by hydroxyl radicals) to green-fluorescent 2′,7′-dichlorofluorescein (DCF; λex = 485 nm, λem = 525 nm). The fluorescence intensity is proportional to the intracellular concentration of hydroxyl radical leading to its quantitative measurement.
H9c2 cells seeded in a 96-well plate at a density of 10 000 cells/well were washed twice with ADS buffer (116 mM NaCl, 5.3 mM KCl, 1.2 mM MgSO4, 1.13 mM NaH2PO4, 5 mM glucose, 1 mM CaCl2, 20 mM HEPES; pH 7.4) and loaded with 10 μM H2DCF-DA in ADS buffer. After 60-minute incubation, the buffer was discarded, the cells were washed twice with ADS buffer, and pre-incubated with rutin for 5 minutes. ISO was then added (both in ADS buffer). All solutions were pre-warmed to 37°C. Fluorescence intensity was measured at time 0 and 2 hours of co-incubation at 37°C using a microplate spectrophotometer Tecan Infinite 200 M (Tecan Austria, Austria). Hydrogen peroxide and tert-butyl hydroperoxide (tBHP) (10–250 μM) were used as positive controls. Intracellular ROS formation after 2 hours was expressed as the percentage of the untreated control (100%).
Statistical analysis
Data are expressed as means ± SEM. Significant outliers were excluded using Grubb's test. Differences were considered significant at P < 0.05, unless indicated otherwise. Statistical analysis was carried out using GraphPad Prism 6.0 (GraphPad Software, USA).
Survival was analysed using Kaplan–Meier survival curves and the log-rank test. cTnT, vitamin E and vitamin C, elements in myocardium, wet ventricle weight index, and glutathione and intracellular ROS formation in the H9c2 cell line were evaluated using one-way ANOVA followed by Tukey's multiple comparison test. The normality of the data was tested by D'Agostino–Pearson test. Time-dependent variables (haemodynamics, glutathione in whole blood, and 8-isoprostane in rat plasma) were analysed using two-way ANOVA followed by Tukey's multiple comparison test.
Results
In an earlier publication we found that a single dose of rutin (46 mg/kg, i.v.) aggravated ISO-induced cardiotoxicity and reduced survival within 24 hours of administration.Citation31 In the present study, we firstly analysed the effects of rutin and sodium bicarbonate (the solvent) on ISO-induced mortality. No control rats receiving either the solvent or rutin died in the haemodynamic study. The mortality rate for the ISO groups receiving either sodium bicarbonate at the lower dose or the lower dose of rutin was similar while the higher dose of rutin resulted in a higher mortality rate than the corresponding solvent group. Moreover, there was a clear tendency of the higher dose of the solvent to prevent mortality, but this was not significant in comparison with the lower dose of the solvent (Supplementary material Fig. A.1). Moreover, we did a post hoc analysis of the mortality rates (Fig. ). Even though the in vivo studies (haemodynamic study, glutathione in whole blood, and 8-isoprostane in plasma) followed different protocols and the frequent blood withdrawals clearly affected the outcome, the results for 2-hour mortality rate were similar, i.e. the post hoc analysis (Fig. ) and Kaplan–Meier survival curves of the individual in vivo studies (Supplementary material Figs. A.1–A.4). Only one rat receiving rutin (without ISO) at a dose of 46 mg/kg died during the 8-isoprostane study after 115 minutes. In general, the frequent blood withdrawals during this 4-hour study were associated with the higher mortality rates (Supplementary material Fig. A.4).
Figure 1 Kaplan–Meier survival curve of all in vivo studies involving the administration of the solvent (sodium bicarbonate, SB, 2.3 or 4.6 ml/kg, i.v.) or rutin (Ru, 11.5 or 46 mg/kg, i.v.), i.e. controls, and their combination with ISO (100 mg/kg, s.c.). The statistical analysis was performed using the log-rank test.
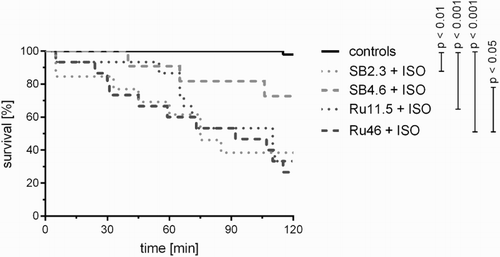
As the solvent, 1.26% sodium bicarbonate, could have affected the results due to its slight alkalinity, we did an additional experiment, in which saline was given in the same volume as the solvent (4.6 ml/kg). There were no significant differences between groups (Supplementary material Fig. A.2).
Based on our previous 24-hour study, in which rutin increased cardiac output dose-dependently after 24 hours,Citation31 and published papers which suggest that flavonoids can decrease blood pressure,Citation38 we assessed haemodynamic variables as these effects could have a negative influence on the early stages of ISO-induced cardiotoxicity. Neither the solvent or rutin themselves nor their combination with ISO significantly modified the haemodynamic variables, in comparison with the corresponding group, during or at the end of the 2-hour continuous recording (Supplementary material Figs. A.5 and A.6). Even if short-term differences were observed for several haemodynamic variables, such fluctuations were apparently caused by increase in intravascular volume rather than a pharmacological action of rutin itself. Analysing ECG variables, ISO-induced mortality was caused by different types of ventricular dysrhythmias including torsades de pointes and AV blocks. There were no specific dysrhythmias in individual groups suggesting rather their coincidence. Moreover, no positive or negative effect of rutin on their frequencies was observed.
In agreement with previously published data, the administration of ISO (100 mg/kg, s.c.) induced a marked release of cTnT into blood. The lower dose of rutin reduced it significantly in comparison with the corresponding ISO group, while there was a dose-dependent tendency of higher dose of rutin to increase cTnT release. However, it was not feasible to assess the hypothesis statistically because of the low survival in the Ru46+ISO group. Interestingly, in agreement with the mortality rate, the higher dose of sodium bicarbonate had a preventive effect, in comparison with the lower dose. Like the mortality, the administration of saline instead of sodium bicarbonate was associated with the same cTnT release. Rats that received the solvent or rutin without ISO had only a negligible cTnT release (Fig. ). No significant differences were found between serum concentrations of vitamin E and vitamin C (Supplementary material Fig. A.7). Similarly, there were no disturbances in myocardial levels of calcium, copper, or zinc (data not shown).
Figure 2 Serum concentration of cTnT after 2-hour haemodynamic study involving the administration of the solvent (sodium bicarbonate, SB, 2.3 or 4.6 ml/kg, i.v.) or rutin (Ru, 11.5 or 46 mg/kg, i.v.), i.e. controls, and their combination with ISO (100 mg/kg, s.c.) including the comparison with saline (S, 4.6 ml/kg, i.v.). Data are expressed as means ± SEM. The statistical analysis was performed using one-way ANOVA followed by Tukey's multiple comparison test. Statistical significance: c vs. the corresponding control group at P < 0.001. Because of high mortality in the group Ru46+ISO, its data were not included in the statistical analysis.
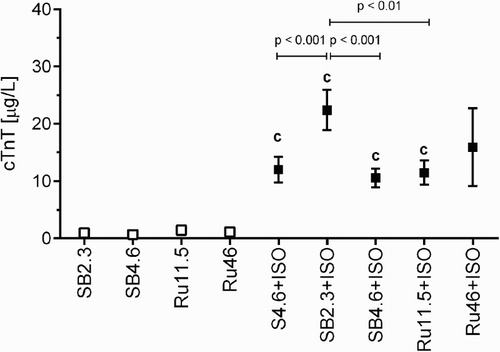
The co-administration of sodium bicarbonate and ISO showed massive diffuse inflammatory infiltrations in all parts of the ventricle using haematoxylin–eosin and Goldner's trichrome green stainings after 2-hour haemodynamic study. Indeed, the presence of large quantities of phagocytes, mast cells together with significant interstitial oedema and foci of necrotic cardiomyocytes were observed, confirming significant alterations of the heart tissue (Fig. C). Although rutin partly ameliorated some of ISO-induced histopathological changes, local capillary hyperaemia, mild interstitial oedema with dilation or swelling of cardiomyocytes, and slight inflammatory cell infiltration were still found in the endocardium and partially in the pericardium (Fig. B, Table ). The structural architecture of all parts of the ventricle was normal in the control groups with the exception of those for rutin in which a slight local capillary hyperaemia was found (Fig. A). It is worth mentioning that no marked differences were observed between the lower dose and the higher dose of rutin and the solvent groups, at all. Moreover, these findings were confirmed at the cellular level using toluidine blue staining (Supplementary material Fig. A.8). The analysis of wet ventricle weight index showed no significant changes (Supplementary material Fig. A.9).
Figure 3 Overview of histological findings in endocardium and myocardium after 2-hour haemodynamic study. Staining: haematoxylin–eosin and Goldner's trichrome green. Representatives of control groups (A; rutin 11.5 mg/kg, i.v.) and ISO groups (100 mg/kg, s.c.) involving a premedication with rutin (B; 11.5 mg/kg, i.v.) or sodium bicarbonate (C; the solvent, 2.3 ml/kg, i.v.). In contrast to normal structural architecture in the control groups (A), marked alterations were found after the administration of ISO (B). Although rutin partly ameliorated histopathological changes, local capillary hyperaemia, mild interstitial oedema with dilation or swelling of cardiomyocytes, and slight inflammatory cell infiltration of phagocytes (▴) were still observed (C). There were no marked differences between the lower dose and the higher dose of rutin, resp. the solvent (not shown).
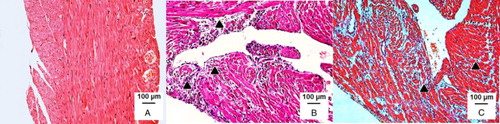
Table 1 Semi-quantitative analysis of histopathological changes in epicardium, myocardium, and endocardium of the left ventricle after 2-hour haemodynamic study
As haemodynamic study and ECG were unable to explain the reason for the higher mortality, we focused on evaluating oxidative stress. Again, there were no significant changes in GSSG, GSH/GSSG ratio, and GSHt levels in whole blood after 2 hours. Interestingly, the higher dose of rutin itself increased the level of GSSG, however, there were no significant differences between the control and ISO groups during the experiment (Supplementary material Fig. A.10). For this reason, we used a simpler model – the rat cardiomyoblast-derived cell line, H9c2, in order to further examine the influence of both rutin and ISO on glutathione levels. Here, as expected, ISO tested over the broad concentration range of 0.1–1000 µM led to slight increase in GSSG levels (Fig. A). Rutin not only failed to prevent the ISO-induced increase in GSSG, it increased it. However, this was significant only at the lowest concentration of ISO (Fig. A). Similarly, a slight increase in glutathione oxidation was revealed through decrease in the GSH/GSSG ratio (Fig. B). Although rutin at the highest concentration tended to increase GSHt levels, there were no significant changes (Fig. C). This may also be the reason for the lower GSH/GSSG ratio (Fig. B).
Figure 4 GSSG (A), the GSH/GSSG ratio (B), and GSHt (C) in the H9c2 cell line exposed to rutin (Ru, 1–1000 µM), ISO (0.1–1000 µM), or their combination, i.e. ISO (0.1–1000 µM) and Ru (1000 µM). Data are expressed as means ± SEM of at least two independent experiments, which were performed in duplicates. The statistical analysis was performed using one-way ANOVA followed by Tukey's multiple comparison test. Statistical significance: *P < 0.05 vs. control; – P < 0.05 vs. Ru 1000 µM.
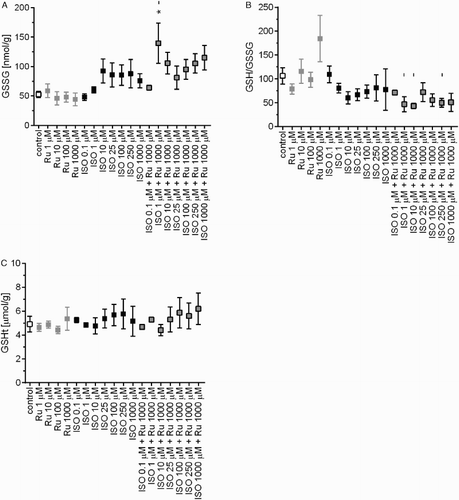
Owing to the low/even negligible changes in plasma glutathione and the H9c2 cell line, we used a more selective biomarker of free radical-initiated peroxidation, 8-isoprostane. In this case, the free fraction level in plasma increased significantly in the SB4.6+ISO group compared with solvent (P < 0.001). The two-way ANOVA showed an overall significant difference between groups sets (P < 0.001) but the post hoc Tukey's multiple comparison showed no significant differences at various time intervals between groups, likely due to the high variability in the Ru46+ISO group (Fig. ).
Figure 5 Changes of the free fraction of 8-isoprostane in plasma following the administration of the solvent (sodium bicarbonate, SB, 4.6 ml/kg, i.v.) or rutin (Ru, 46 mg/kg, i.v.), i.e. controls, and their combination with ISO (100 mg/kg, s.c.). The changes were calculated vs. baseline level (at time 0 minute, before ISO administration). Data are expressed as means ± SEM. Each sample was tested at least in duplicate. Two-way ANOVA showed significant differences between means of data sets (P < 0.001).
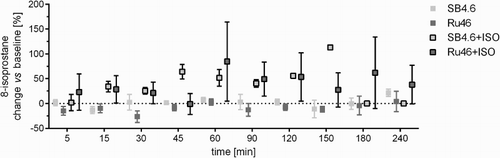
Due to the minor changes in oxidative stress found in plasma, intracellular ROS formation was determined using the H2DCF-DA assay. As seen in Fig. , none of the concentrations of ISO induced ROS production inside H9c2 cells after 2-hour incubation in comparison with the control group. Interestingly, a dose-dependent decrease was observed. However, co-incubation with rutin had the opposite effect. The most significant induction was seen with the combination of 1000 µM rutin and 10 µM ISO. As predicted the positive controls, tBHP, and hydrogen peroxide dose-dependently resulted in intracellular ROS formation (Supplementary material Fig. A.11).
Figure 6 Intracellular ROS formation in H9c2 cardiomyoblasts determined using the H2DCF-DA assay after 2-hour treatment with ISO (0.1–1000 µM), rutin (Ru, 0.1–1000 µM), or their combination, i.e. ISO (0.1–1000 µM) and Ru (1000 µM). Data are expressed as means ± SEM; n = 3. The statistical analysis was performed using one-way ANOVA followed by Tukey's multiple comparison test. Statistical significance: *P < 0.05 vs. control; + P < 0.05, ++ P < 0.01, and +++ P < 0.001 vs. the corresponding ISO group without rutin.
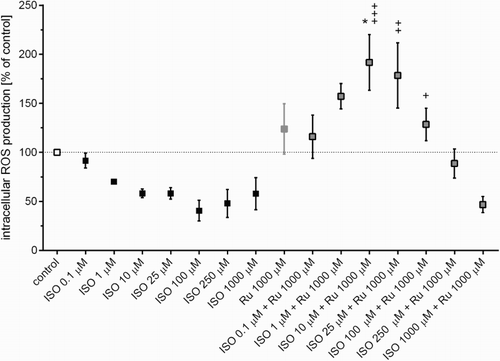
To confirm the presence of rutin in rat plasma, whole blood was withdrawn from one rat for analysis of the pharmacokinetic profile after the slow i.v. bolus (Supplementary material Fig. A.12 and Supplementary material Tab. A.1). Calculations were carried out using two independent programmes (Kinetica 5.1 and PKSolver). The minor difference between the area under the curve at the last analysed time-point (AUC0– t) and that extrapolated to infinity (AUC0–∞) confirmed sufficient duration of the study and thus accuracy of analysed pharmacokinetic parameters.Citation39
Discussion
Rutin, quercetin-3-O-rutinoside, a natural flavonol glycoside, has shown various beneficial pharmacological properties which could be useful in the treatment of many human diseases, particularly those of the cardiovascular system.Citation40 These benefits have been mostly attributed to its antioxidant effects involving the combination of scavenging activities towards various ROS (e.g. superoxide, hypochlorite, and peroxynitrate), iron/copper chelation properties, and inhibition of free radical-forming enzymes (e.g. xanthine oxidase) and anti-inflammatory effects.Citation6,Citation8,Citation11,Citation41–Citation44 It may improve endothelial function by augmenting NO production and inhibiting human platelet aggregation, as well.Citation45,Citation46 However, rutin can also have pro-oxidative effects, which could be based on its metal-reducing potential and increased formation of the hydroxyl radical via Fenton chemistry.Citation12 This may also depend on other factors such as concentrations used, presence of other reductants, and/or the atmospheric oxygen access.Citation47
Stanely Mainzen Prince and coworkers reported a broad spectrum of prophylactic effects of rutin (40 or 80 mg/kg/day, p.o., 42 days) in the case of various aspects of ISO-induced cardiotoxicity (150 mg/kg/day, s.c., 2 days), in the same rat strain as used in this study. The above authors suggested the beneficial effects of rutin were associated with free radical scavenging activity, lipid-lowering effects, both mitochondrial structure and function improvement, and membrane stabilizing properties in particular.Citation26–Citation29 It is not easy to reconcile such results with the findings concerning the current study and our published study over 24 hours.
Firstly, Stanely Mainzen Prince and coworkers administered rutin orally for 42 days. We used a single i.v. dose. This is an important factor as rutin is not absorbed in the intestine. A number of clinical and experimental studies have reported that rutin is cleaved in the caecum via bacterial microflora and further metabolized by both bacterial and human enzymes to form various metabolites such as quercetin and its glucuronide and sulphate, tamarixetin, isorhamnetin, and various phenolic acids, resulting to no absorption of the parent rutin itself.Citation31,Citation48–Citation52 Moreover, the relatively short elimination half-life of i.v. rutin (Supplementary material Fig. A.10 and Supplementary material Tab. A.1) suggests that the positive effects of oral rutin on the ISO-induced oxidative stress were caused by its metabolites, including quercetin conjugates and small phenolic acids, and/or some adaptation mechanism(s).Citation53–Citation55 Therefore, if rutin had positive effects, these benefits probably were not caused by rutin per se.
Secondly, no mortality data were provided by Stanely Mainzen Prince and coworkers, surprisingly, only positive results. Moreover, the results were linearly related to dose. It is very uncommon that 42-day oral treatment results in a high linear dose/effect relationship. To test this, we performed a similar analysis to the one we reported recently.Citation56 In short, mean control values were considered 100% (untreated rats), the mean ISO values were considered 0% (the pathological state), and mean effects of rutin were expressed as percent inhibition due to ISO effect. Surprisingly, a linear relationship describing inhibition of ISO-induced cardiotoxicity was found in most of the cases. Indeed, the linear regression coefficients in 5/34 cases were equal to 1.000 (15%), in 15/34 (44%) cases higher than 0.990, and in 25/34 (74%) cases higher than 0.975, respectively. Such high linearity for so many measured variables in the studies of Stanely Mainzen Prince and coworkers is unlikely. It is also well known that the bioavailability and pharmacokinetics of oral rutin are non-linear.Citation49,Citation50,Citation57
In contrast, we found that a single i.v. dose of rutin either did not influence (11.5 mg/kg) or aggravate (46 mg/kg) ISO-induced cardiotoxicity (100 mg/kg, s.c.) in Wistar rats after 24 hours.Citation31 In agreement, the higher dose of rutin resulted in increased mortality rate from 30 to 52%, while the lower dose had no effect on mortality. Moreover, such outcomes are in agreement with cTnT concentrations; myocardial calcium levels, and in vitro experiments, in which co-incubation with higher concentrations of rutin was found to significantly increase ISO-induced toxicity in the H9c2 cell line.Citation31
Similar findings were confirmed in this study. Indeed, rutin was not able to protect myocardium against ISO-induced cardiotoxicity within 2 hours of continuous experiment. Although the mortality rate was higher in general, likely due to invasive approach and/or frequent blood withdrawals, the outcome was similar (Fig. ). Here, we discovered three interesting facts: (1) the lower dose of rutin did not affect the mortality rate but it decreased cTnT release after 2 hours, (2) the greater volume of administered solution itself was protective regardless whether it was saline or 1.26% sodium bicarbonate, (Fig. ), and (3) both doses of rutin had some positive effect on histological myocardial changes after 2 hours due to ISO regardless of the high mortality rate and different effect on cTnT release. The results extended our previous findings demonstrating that i.v. rutin was unable to provide sufficient protection against ISO-induced cardiotoxicity. pH is markedly reduced during ischaemiaCitation58 and we speculated that slightly alkaline solvent might afford some degree of protection but this was not the case as saline was similarly protective. One reason could be that ISO results in massive vasodilation due to β2-adrenergic hyperstimulation together with myocardial hyperstimulation through β1-receptors causing an acute state of circulatory shockCitation21 and increase in circulating blood by administration of a higher dose of fluid can partly reverse it.
The reason why both doses of rutin had partial protective effects from the histological findings after 2 hours without any effect on histology after 24 hoursCitation31 or mortality is not clear. Since there was a marked difference between acute (2 hours) changes in myocardial histology which were mainly of an inflammatory character and subacute (24 hours) changes which included myocardial necrosis, we suggest the acute positive effects may be based on the anti-inflammatory effects of flavonoids.Citation5,Citation59
Apropos oxidative stress variables in blood, rutin itself dose-dependently increased the level of GSHt after 24 hours, however, ISO abolished this. The effect appears to correspond with our outcomes, in which both GSHt and the GSH/GSSG ratio tended to increase at the higher concentration of rutin in the H9c2 cardiomyoblast-derived cell line (Fig. B and C). No significant differences in thiobarbituric acid reactive substances or vitamin E have been found after 24 hours.Citation31 The latter corresponds to this study, in which no significant changes in blood variables were observed 2 hours after the ISO treatment. This was valid for the continuous analysis of GSSG in blood as well. Even though there were only minor differences in oxidative stress-related parameters between groups, the mortality rates were markedly different. These data clearly suggest that antioxidant markers and markers of oxidative stress in blood might not be the valid markers of ISO-induced cardiotoxicity described.Citation60,Citation61
Interestingly, the inability of ISO to induce detectable formation of ROS was in accordance with our previous studies.Citation62,Citation63 This phenomenon has also been described by Costa et al.,Citation64 where no changes were shown in lipid peroxidation, protein carbonylation, or activity of various antioxidant enzymes after up to 3 hours of incubation of isolated adult rat cardiomyocytes with 500 μM adrenaline. Moreover, direct antioxidant activity of catecholamines under pro-oxidant conditions has been described. Adrenaline elicited intracellular survival pathways in isolated rat cardiomyocytes resembling those described for the phenomenon of ischaemic preconditioning.Citation65 This may explain the above mentioned changes in glutathione in our 2- and 24-hour in vivo studies as well.
Although we found no convincing data in whole blood, our results clearly showed that rutin aggravated intracellular oxidative stress under in vitro conditions (Fig. ) and was not able to reverse oxidative stress caused by ISO (Fig. ). However, the clinical impact of these effects is unknown because the mortality rates for SB2.3/4.6+ISO and Ru11.5+ISO were not different, and there was a decrease in cTnT levels in Ru11.5+ISO (Figs. and ). Similarly, in contrast to the cell experiments, analysis of the free fraction of 8-isoprostane in rat plasma, a specific marker of free radical-initiated peroxidation of arachidonic acid,Citation66 revealed only differences between ISO and sodium bicarbonate (Fig. ). Conclusively, although Stanely Mainzen Prince and coworkers reported positive effects of oral rutin on various oxidative stress markers, this does not mean that rutin is able to revert negative haemodynamic effects and clinical outcome (mortality) of ISO administration. In line with our previous findings that refute any positive effect of oral quercetin on ISO-induced cardiotoxicity,Citation32 claiming rutin is an oral protective substance against ISO-induced cardiac injury will need detailed study showing haemodynamic, ECG, and mortality rates as well. However, from our quercetin findings, we suspect such outcomes are unlikely, mainly due to the complex mechanisms of ISO-induced cardiotoxicity.
Conclusion
In contrast to studies reporting a wide spectrum of preventive effects of continuous oral administration of rutin against ISO-induced cardiotoxicity, the results described here of the direct effects of a single i.v. dose of rutin within 2 hours confirmed our previous findings of no direct protection of rutin. Rutin did not reduce the mortality rate and it increased intracellular ROS formation in vitro and had the tendency to increase it in vivo. The higher dose of sodium bicarbonate (4.6 ml/kg, 1.26% w/v solution) was found to protect rat myocardium possibly due to assuaging the state of shock accompanying ISO-induced cardiotoxicity. This is supported by significant decrease in cTnT level and greater survival rate.
Disclaimer statements
Contributors None.
Funding This work was supported by The Czech Science Foundation [grant number P303/12/G163], Charles University in Prague [grant numbers GAUK/605712C and No. SVV 260 064]. The publication is co-financed by the European Social Fund and the State Budget of the Czech Republic (Operational Program) [grant number CZ.1.07/2.3.00/30.0061].
Conflicts of interest The authors declare that they have no conflict of interest.
Ethics approval The study was approved by the Ethics Committee of Charles University in Prague, Faculty of Pharmacy in Hradec Králové (Study No. MŠMT 2437/2012-30), and it conformed to the guide for the care and use of laboratory animals published by The US National Institutes of Health (NIH Publication No. 85-23, revised 1996).
Supplementary data The underlying research materials for this article can be accessed at <https://dx.doi.org/10.1080/13510002.2016.1159817>
Appendix_-_Supplementary_data_-_Redox_Report_-_Revision.docx
Download MS Word (3.9 MB)Acknowledgements
The authors wish to thank Mrs Pavlína Lukešová, Mrs Anežka Kunová, and Miss Renata Exnarová for their excellent technical assistance.
References
- Bravo L. Polyphenols: chemistry, dietary sources, metabolism, and nutritional significance. Nutr Rev 1998;56(11):317–33. doi: 10.1111/j.1753-4887.1998.tb01670.x
- Toh JY, Tan VM, Lim PC, Lim ST, Chong MF. Flavonoids from fruit and vegetables: a focus on cardiovascular risk factors. Curr Atheroscler Rep 2013;15(12):368. Available from: http://www.ncbi.nlm.nih.gov/pubmed/24091782. doi: 10.1007/s11883-013-0368-y
- Mink PJ, Scrafford CG, Barraj LM, Harnack L, Hong CP, Nettleton JA, et al. Flavonoid intake and cardiovascular disease mortality: a prospective study in postmenopausal women. Am J Clin Nutr 2007;85(3):895–909.
- Hooper L, Kroon PA, Rimm EB, Cohn JS, Harvey I, Le Cornu KA, et al. Flavonoids, flavonoid-rich foods, and cardiovascular risk: a meta-analysis of randomized controlled trials. Am J Clin Nutr 2008;88(1):38–50.
- Mladenka P, Zatloukalova L, Filipsky T, Hrdina R. Cardiovascular effects of flavonoids are not caused only by direct antioxidant activity. Free Radic Biol Med 2010;49(6):963–75. doi: 10.1016/j.freeradbiomed.2010.06.010
- Mladenka P, Macakova K, Filipsky T, Zatloukalova L, Jahodar L, Bovicelli P, et al. In vitro analysis of iron chelating activity of flavonoids. J Inorg Biochem 2011;105(5):693–701. doi: 10.1016/j.jinorgbio.2011.02.003
- Seyoum A, Asres K, El-Fiky FK. Structure–radical scavenging activity relationships of flavonoids. Phytochemistry 2006;67(18):2058–70. doi: 10.1016/j.phytochem.2006.07.002
- Nagao A, Seki M, Kobayashi H. Inhibition of xanthine oxidase by flavonoids. Biosci Biotechnol Biochem 1999;63(10):1787–90. doi: 10.1271/bbb.63.1787
- Mira L, Fernandez MT, Santos M, Rocha R, Florencio MH, Jennings KR. Interactions of flavonoids with iron and copper ions: a mechanism for their antioxidant activity. Free Radic Res 2002;36(11):1199–208. doi: 10.1080/1071576021000016463
- El Haouari M, Rosado JA. Modulation of platelet function and signaling by flavonoids. Mini Rev Med Chem 2011;11(2):131–42. doi: 10.2174/138955711794519537
- Riha M, Karlickova J, Filipsky T, Macakova K, Rocha L, Bovicelli P, et al. In vitro evaluation of copper-chelating properties of flavonoids. RSC Adv 2014;4(62):32628–38. doi: 10.1039/C4RA04575K
- Macakova K, Mladenka P, Filipsky T, Riha M, Jahodar L, Trejtnar F, et al. Iron reduction potentiates hydroxyl radical formation only in flavonols. Food Chem 2012;135(4):2584–92. doi: 10.1016/j.foodchem.2012.06.107
- Prochazkova D, Bousova I, Wilhelmova N. Antioxidant and prooxidant properties of flavonoids. Fitoterapia 2011;82(4):513–23. doi: 10.1016/j.fitote.2011.01.018
- Cao G, Sofic E, Prior RL. Antioxidant and prooxidant behavior of flavonoids: structure-activity relationships. Free Radic Biol Med 1997;22(5):749–60. doi: 10.1016/S0891-5849(96)00351-6
- Kloner RA. Natural and unnatural triggers of myocardial infarction. Prog Cardiovasc Dis 2006;48(4):285–300. doi: 10.1016/j.pcad.2005.07.001
- Schömig A. Catecholamines in myocardial ischemia. Systemic and cardiac release. Circulation 1990;82(3 Suppl):II13–22.
- Chagoya de Sánchez V, Hernández-Muñoz R, López-Barrera F, Yañez L, Vidrio S, Suárez J, et al. Sequential changes of energy metabolism and mitochondrial function in myocardial infarction induced by isoproterenol in rats: a long-term and integrative study. Can J Physiol Pharmacol 1997;75(12):1300–11. doi: 10.1139/y97-154
- Díaz-Muñoz M, Álvarez-Pérez MA, Yáñez L, Vidrio S, Martínez L, Rosas G, et al. Correlation between oxidative stress and alteration of intracellular calcium handling in isoproterenol-induced myocardial infarction. Mol Cell Biochem 2006;289(1–2):125–36. doi: 10.1007/s11010-006-9155-1
- Mladěnka P, Hrdina R, Bobrovová Z, Semecký V, Vávrová J, Holecková M, et al. Cardiac biomarkers in a model of acute catecholamine cardiotoxicity. Hum Exp Toxicol 2009;28(10):631–40. doi: 10.1177/0960327109350665
- Berenshtein E, Vaisman B, Goldberg-Langerman C, Kitrossky N, Konijn AM, Chevion M. Roles of ferritin and iron in ischemic preconditioning of the heart. Mol Cell Biochem 2002;234–235(1–2):283–92. doi: 10.1023/A:1015923202082
- Rona G. Catecholamine cardiotoxicity. J Mol Cell Cardiol 1985;17(4):291–306. doi: 10.1016/S0022-2828(85)80130-9
- Chappel CI, Rona G, Balazs T, Gaudry R. Severe myocardial necrosis produced by isoproterenol in the rat. Arch Int Pharmacodyn Ther 1959;122:123–8.
- Rona G, Chappel CI, Balazs T, Gaudry R. An infarct-like myocardial lesion and other toxic manifestations produced by isoproterenol in the rat. AMA Arch Pathol 1959;67(4):443–55.
- Bindoli A, Rigobello MP, Deeble DJ. Biochemical and toxicological properties of the oxidation products of catecholamines. Free Radic Biol Med 1992;13(4):391–405. doi: 10.1016/0891-5849(92)90182-G
- Todd GL, Cullan GE, Cullan GM. Isoproterenol-induced myocardial necrosis and membrane permeability alterations in the isolated perfused rabbit heart. Exp Mol Pathol 1980;33(1):43–54. doi: 10.1016/0014-4800(80)90006-4
- Karthick M, Stanely Mainzen Prince P. Preventive effect of rutin, a bioflavonoid, on lipid peroxides and antioxidants in isoproterenol-induced myocardial infarction in rats. J Pharm Pharmacol 2006;58(5):701–7. doi: 10.1211/jpp.58.5.0016
- Stanely Mainzen Prince P, Karthick M. Preventive effect of rutin on lipids, lipoproteins, and ATPases in normal and isoproterenol-induced myocardial infarction in rats. J Biochem Mol Toxicol 2007;21(1):1–6. doi: 10.1002/jbt.20151
- Stanely Mainzen Prince P, Priya S. Preventive effects of rutin on lysosomal enzymes in isoproterenol induced cardio toxic rats: biochemical, histological and in vitro evidences. Eur J Pharmacol 2010;649(1–3):229–35. doi: 10.1016/j.ejphar.2010.08.054
- Punithavathi VR, Shanmugapriya K, Prince PS. Protective effects of rutin on mitochondrial damage in isoproterenol-induced cardiotoxic rats: an in vivo and in vitro study. Cardiovasc Toxicol 2010;10(3):181–9. doi: 10.1007/s12012-010-9077-8
- Prince PS, Sathya B. Pretreatment with quercetin ameliorates lipids, lipoproteins and marker enzymes of lipid metabolism in isoproterenol treated cardiotoxic male Wistar rats. Eur J Pharmacol 2010;635(1–3):142–8. doi: 10.1016/j.ejphar.2010.02.019
- Mladěnka P, Zatloukalová L, Šimůnek T, Bobrovová Z, Semecký V, Nachtigal P, et al. Direct administration of rutin does not protect against catecholamine cardiotoxicity. Toxicology 2009;255(1–2):25–32. doi: 10.1016/j.tox.2008.09.027
- Riha M, Voprsalova M, Pilarova V, Semecky V, Holeckova M, Vavrova J, et al. Oral administration of quercetin is unable to protect against isoproterenol cardiotoxicity. Naunyn Schmiedebergs Arch Pharmacol 2014;387(9):823–35. doi: 10.1007/s00210-014-0995-z
- Filipský T, Zatloukalová L, Mladěnka P, Hrdina R. Acute initial haemodynamic changes in a rat isoprenaline model of cardiotoxicity. Hum Exp Toxicol 2012;31(8):830–43. doi: 10.1177/0960327112438927
- Zatloukalová L, Filipský T, Mladěnka P, Semecký V, Macaková K, Holecková M, et al. Dexrazoxane provided moderate protection in a catecholamine model of severe cardiotoxicity. Can J Physiol Pharmacol 2012;90(4):473–84. doi: 10.1139/y2012-009
- Zhang Y, Huo M, Zhou J, Xie S. PKSolver: an add-in program for pharmacokinetic and pharmacodynamic data analysis in Microsoft Excel. Comput Methods Programs Biomed 2010;99(3):306–14. doi: 10.1016/j.cmpb.2010.01.007
- Kimes BW, Brandt BL. Properties of a clonal muscle cell line from rat heart. Exp Cell Res 1976;98(2):367–81. doi: 10.1016/0014-4827(76)90447-X
- Šimůnek T, Štěrba M, Popelová O, Kaiserová H, Adamcová M, Hroch M, et al. Anthracycline toxicity to cardiomyocytes or cancer cells is differently affected by iron chelation with salicylaldehyde isonicotinoyl hydrazone. Br J Pharmacol 2008;155(1):138–48. doi: 10.1038/bjp.2008.236
- Chan EC, Pannangpetch P, Woodman OL. Relaxation to flavones and flavonols in rat isolated thoracic aorta: mechanism of action and structure–activity relationships. J Cardiovasc Pharmacol 2000;35(2):326–33. doi: 10.1097/00005344-200002000-00023
- Jambhekar SS, Breen PJ. Basic pharmacokinetics. London: Pharmaceutical Press; 2009.
- Sharma S, Ali A, Ali J, Sahni JK, Baboota S. Rutin: therapeutic potential and recent advances in drug delivery. Expert Opin Investig Drugs 2013;22(8):1063–79. doi: 10.1517/13543784.2013.805744
- Haenen GRMM, Paquay JBG, Korthouwer REM, Bast A. Peroxynitrite scavenging by flavonoids. Biochem Biophys Res Commun 1997;236(3):591–3. doi: 10.1006/bbrc.1997.7016
- Moridani MY, Pourahmad J, Bui H, Siraki A, O'Brien PJ. Dietary flavonoid iron complexes as cytoprotective superoxide radical scavengers. Free Radic Biol Med 2003;34(2):243–53. doi: 10.1016/S0891-5849(02)01241-8
- Firuzi O, Mladênka Pe, Petrucci R, Marrosu G, Saso L. Hypochlorite scavenging activity of flavonoids. J Pharm Pharmacol 2004;56(6):801–7. doi: 10.1211/0022357023556
- Guardia T, Rotelli AE, Juarez AO, Pelzer LE. Anti-inflammatory properties of plant flavonoids. Effects of rutin, quercetin and hesperidin on adjuvant arthritis in rat. Farmaco 2001;56(9):683–7. doi: 10.1016/S0014-827X(01)01111-9
- Ugusman A, Zakaria Z, Chua KH, Megat Mohd Nordin NA, Abdullah Mahdy Z. Role of rutin on nitric oxide synthesis in human umbilical vein endothelial cells. ScientificWorldJournal 2014;2014:169370. Available from: http://www.hindawi.com/journals/tswj/2014/169370/ doi: 10.1155/2014/169370
- Sheu J-R, Hsiao G, Chou P-H, Shen M-Y, Chou D-S. Mechanisms involved in the antiplatelet activity of rutin, a glycoside of the flavonol quercetin, in human platelets. J Agric Food Chem 2004;52(14):4414–8. doi: 10.1021/jf040059f
- Labuda J, Bučková M, Heilerová Ľ, Šilhár S, Štepánek I. Evaluation of the redox properties and anti/pro-oxidant effects of selected flavonoids by means of a DNA-based electrochemical biosensor. Anal Bioanal Chem 2003;376(2):168–73.
- Manach C, Morand C, Texier O, Favier ML, Agullo G, Demigne C, et al. Quercetin metabolites in plasma of rats fed diets containing rutin or quercetin. J Nutr 1995;125(7):1911–22.
- Erlund I, Kosonen T, Alfthan G, Maenpaa J, Perttunen K, Kenraali J, et al. Pharmacokinetics of quercetin from quercetin aglycone and rutin in healthy volunteers. Eur J Clin Pharmacol 2000;56(8):545–53. doi: 10.1007/s002280000197
- Rechner AR, Smith MA, Kuhnle G, Gibson GR, Debnam ES, Srai SK, et al. Colonic metabolism of dietary polyphenols: influence of structure on microbial fermentation products. Free Radic Biol Med 2004;36(2):212–25. doi: 10.1016/j.freeradbiomed.2003.09.022
- Serra A, Macià A, Romero M-P, Reguant J, Ortega N, Motilva M-J. Metabolic pathways of the colonic metabolism of flavonoids (flavonols, flavones and flavanones) and phenolic acids. Food Chem 2012;130(2):383–93. doi: 10.1016/j.foodchem.2011.07.055
- Choudhury R, Srai SK, Debnam E, Rice-Evans CA. Urinary excretion of hydroxycinnamates and flavonoids after oral and intravenous administration. Free Radic Biol Med 1999;27(3–4):278–86. doi: 10.1016/S0891-5849(99)00054-4
- Tang D, Yin Y, Zhang Z, Gao Y, Wei Y, Chen Y, et al. Simultaneous HPLC-DAD analysis of five flavonoids in diabetic rat plasma and its application in the study of pharmacokinetics. Acta Chromatographica 2009;21(3):483–97. doi: 10.1556/AChrom.21.2009.3.11
- Wang SY, Chai JY, Zhang WJ, Liu X, Du Y, Cheng ZZ, et al. HPLC determination of five polyphenols in rat plasma after intravenous administration of hawthorn leaves extract and its application to pharmacokinetic study. Yakugaku Zasshi 2010;130(11):1603–13. doi: 10.1248/yakushi.130.1603
- Yang CY, Hsiu SL, Wen KC, Lin SP, Tsai SY, Hou YC, et al. Bioavailability and metabolic pharmacokinetics of rutin and quercetin in rats. J Food Drug Anal 2004;13(3):244–50.
- Mladenka P, Hrdina R, Filipsky T, Riha M, Palicka V. Is a highly linear relationship between the dose of quercetin and the pharmacological effect possible? – a comment on Liu, et al. Evaluation of antioxidant and immunity activities of quercetin in isoproterenol-treated rats. Molecules 2012;17:4281–4291. Molecules 2014;19(7):9606–9. doi: 10.3390/molecules19079606
- Manach C, Morand C, Demigne C, Texier O, Regerat F, Remesy C. Bioavailability of rutin and quercetin in rats. FEBS Lett 1997;409(1):12–6. doi: 10.1016/S0014-5793(97)00467-5
- Ambrosio G, Zweier JL, Jacobus WE, Weisfeldt ML, Flaherty JT. Improvement of postischemic myocardial function and metabolism induced by administration of deferoxamine at the time of reflow: the role of iron in the pathogenesis of reperfusion injury. Circulation 1987;76(4):906–15. doi: 10.1161/01.CIR.76.4.906
- Garcia-Lafuente A, Guillamon E, Villares A, Rostagno MA, Martinez JA. Flavonoids as anti-inflammatory agents: implications in cancer and cardiovascular disease. Inflamm Res 2009;58(9):537–52. doi: 10.1007/s00011-009-0037-3
- Strobel NA, Fassett RG, Marsh SA, Coombes JS. Oxidative stress biomarkers as predictors of cardiovascular disease. Int J Cardiol 2011;147(2):191–201. doi: 10.1016/j.ijcard.2010.08.008
- Mladenka P, Zatloukalova L, Filipsky T, Vavrova J, Holeckova M, Palicka V, et al. Common biomarkers of oxidative stress do not reflect cardiovascular dys/function in rats. Biomed Pap Med Fac Univ Palacky Olomouc Czech Repub 2013;157(2):146–52.
- Haskova P, Kovarikova P, Koubkova L, Vavrova A, Mackova E, Simunek T. Iron chelation with salicylaldehyde isonicotinoyl hydrazone protects against catecholamine autoxidation and cardiotoxicity. Free Radic Biol Med 2011;50(4):537–49. doi: 10.1016/j.freeradbiomed.2010.12.004
- Mladenka P, Kalinowski DS, Haskova P, Bobrovova Z, Hrdina R, Simunek T, et al. The novel iron chelator, 2-pyridylcarboxaldehyde 2-thiophenecarboxyl hydrazone, reduces catecholamine-mediated myocardial toxicity. Chem Res Toxicol 2009;22(1):208–17. doi: 10.1021/tx800331j
- Costa VM, Silva R, Ferreira LM, Branco PS, Carvalho F, Bastos ML, et al. Oxidation process of adrenaline in freshly isolated rat cardiomyocytes: formation of adrenochrome, quinoproteins, and GSH adduct. Chem Res Toxicol 2007;20(8):1183–91. doi: 10.1021/tx7000916
- Costa VM, Silva R, Ferreira R, Amado F, Carvalho F, de Lourdes Bastos M, et al. Adrenaline in pro-oxidant conditions elicits intracellular survival pathways in isolated rat cardiomyocytes. Toxicology 2009;257(1–2):70–9. doi: 10.1016/j.tox.2008.12.010
- Milne GL, Musiek ES, Morrow JD. F2-isoprostanes as markers of oxidative stress in vivo: an overview. Biomarkers 2005;10(Suppl 1):S10–23. doi: 10.1080/13547500500216546