Abstract
Sensitized photooxidation processes in the presence of natural pigments may provide an alternative to antibiotics degradation since these compounds are transparent to natural light irradiation, therefore, they can be degraded by the action of photosensitizers which absorb light and produce highly reactive species, especially those derived from molecular oxygen (ROS). Most antibiotics used currently belong to a group of pharmaceutical substances that have been considered a new type of contaminants due to their persistence and bioaccumulation in the environment.
Objective: In this context, we decided to investigate the kinetic and mechanistic aspects of Vancomycin (Vanco) photosensitized degradation in the presence of the natural pigment Riboflavin (Vitamin B2, Rf) and the artificial dye Rose Bengal (RB) for comparative purposes.
Methods: The study have been done by using Stationary photolysis, Laser flash photolysis, Time-resolved phosphorence detection of O2(1Δg) experiments and Bactericidal activity evaluation. The experiments were carried out in aqueous solution at different pH values in order to establish relationships between the structure of the compound and its susceptibility to ROS-mediated photooxidation.
Results: Experimental evidence indicates that in the presence of Rf there is considerable contribution of the radical-mediated mechanism, while in the presence of RB the photooxidation process occurs exclusively through O2(1Δg) and the reactivity to this excited species increases with increasing pH of the environment.
Discussion: The results obtained, have been shown that Rf can raise the photodegradation of Vanco by both the radical pathway and the O2(1Δg) mediated. Furthermore, the antibiotic is able to interact with the excited electronic states of Rf as well as O2(1Δg) generated by energy transfer between the excited triplet state of the photosensitizer and the oxygen ground state. The predominant mechanism for photodegradation of Vanco in the presence of the Rf is the radical via because of the considerable interaction with the excited triplet state of the photosensitizer demonstrated by laser flash photolysis experiments.
Microbiological test on Staphylococcus aureus ATCC25923 showed that the bactericidal activity of the antibiotic on the strain studied was affected by the sensitized photodegradation process, suggesting that photoproducts generated eventually do not retain the bactericidal properties of the original antibiotic.
Introduction
The degradation of pharmaceutical compounds has received particular attention during the last decades.Citation1–Citation6 Particularly, in the case of antibiotics, photosensitized processes can produce oxidative damage altering the specific therapeutic properties of the drugs.Citation7–Citation10
Different reactive oxygen species (ROS) can be produced in natural oxidative stress scenarios. These species and the pharmaceutical drugs can be localized in the same environment. Due to this fact, the study of the photodegradation of pharmaceuticals, particularly antibiotics mediated by ROS is of great relevance.
In vitro, and in the presence of oxygen, ROS can be generated by using other compound able to absorb environmental light, the so-called photosensitizer. In order to closely model biological or environmental conditions, the naturally occurring pigment riboflavin (Rf, vitamin B2) known to react through both Type I (radical mediated) and Type II (via singlet oxygen, O2(1Δg)) mechanisms, present in practically all kind of water courses, water mirrorsCitation11,Citation12 and in all living organisms,Citation13 was employed as a photosensitizer. The artificial photosensitizer Rose Bengal (RB), an exclusively O2(1Δg)-generator,Citation14 was used for comparative purposes. Rf has been reported as a frequent photosensitizer for the oxidative degradation of numerous relevant natural substrates.Citation15 The pigment produces the highly reactive species O2(1Δg). Besides, numerous cases of other aggressive ROS, such as superoxide radical anion (O2•–) produced through Rf-photosensitization have been reported.Citation16
Another important aspect related to antibiotics is that most of them do not undergo metabolic inactivation and they are inevitably discharged into aquatic environments, persisting at trace levels even after conventional wastewater treatment.Citation17–Citation23 The presence of antibiotics in aquatic systems could contribute to the proliferation of strains of bacteria that are resistant to major classes of antibiotics.Citation24,Citation25 This fact constitutes a serious potential risk for the natural environment and could cause difficulties in the treatment of infectious diseases. Thus, the persistence/oxidability of these drugs under natural-light- irradiation in the presence of a native dye-sensitizer constitutes a topic of environmental relevance.
In the present contribution, vancomycin (Vanco), a glycopeptide antibiotic has been selected for this study (Scheme ). Vanco is a powerful antibiotic often used as a last resort in infections,Citation26 particularly, in nosocomial infections caused by Enterococcus and Staphylococcus aureus resistant to methicillin.Citation27 For this reason, S. aureus was selected for this study. Besides, this microorganism can cause serious multiple infections including boils, abscesses, endocarditis, osteomyelitis, sepsis, and septic shock. It can also infect pacemakers, artificial heart valves, and joint implants.Citation28
Vanco and its glycopeptides derivatives interfere with the late stages of peptidoglycan synthesis of the bacterial cell wall. Vanco and its analogs are not able to cross the inner membrane of the bacterial cell wall. Due to this fact, Gram negative bacteria result resistant to this kind of antibiotics.Citation26 However, this glycopeptide has bactericide effect and also alters the citoplasmatic membrane permeability. For this reason, it turns out to be efficient against protoplasts and moreover, inhibits the ARN synthesis.Citation29
The purpose for the selection of Vanco in the present study is the fact that, although there are many worksCitation7–Citation10 on sensitized photooxidation of antibiotics in literature, to the best of our knowledge there are no systematic studies (kinetic and mechanistic aspects) with glycopeptides antibiotics.
In this context, the aim of the present contribution is to investigate, in vitro, the kinetic and mechanistic aspects of the photosensitized degradation process of Vanco in the presence of the photosensitizers mentioned above. The study has been carried out in pure water at different pH conditions to establish relationships between the structure of the substrate and the susceptibility to photooxidation mediated by ROS. Also, the possible interactions between the antibiotic and the photosensitizers have been evaluated. On the other hand, since antibiotics degraded by photogenerated ROS can decrease its specific therapeutic activity,Citation7–Citation10 microbiological tests which relate the effects of the sensitized photodegradation of Vanco and its antimicrobial activity were performed in this study on S. aureus strains.
The knowledge achieved through this type of study could help the prediction of the potential impact of glycopeptides antibiotics in aquatic ecosystems. Also, the understanding of the relations between the structure of these pollutants and their reactivity against photogenerated ROS makes the development of compounds with scheduled natural decay possible.
Experimental
Materials
Riboflavin (Rf), vancomycin hydrochloride hydrate, superoxide dismutase from bovine erythrocytes (SOD), catalase from bovine liver (CAT) and deuterium oxide 99.9% (D2O) were purchased from Sigma Chem. Co. Rose Bengal (RB), sodium azide (NaN3), and d-mannitol, from Sigma Aldrich. Furfuryl alcohol (FFA), from Riedel de Haën. All these compounds were used as received.
The water used was triply distilled. The pH-pD values 7.4 and 11 of the final aqueous solutions for all photochemical experiments were adjusted using buffer solutions of KH2PO4, Na2HPO4 and NaOH,Citation30 provided by Aldrich Chemical Company. All measurements were carried out at room temperature with freshly prepared solutions.
Culture media: Tryptic Soy Broth (TSB), Tryptic soy agar (TSA), Agar-agar (AA), and Mueller Hinton Agar (MHA) were purchased from Britain. The microorganism used in this study was the reference strain S. aureus ATCC 25923 provided by the Department of Microbiology UNRC, Argentina.
Methods
Stationary photolysis experiment
The experiences of sensitized photooxidation under stationary conditions were carried out by the irradiation with visible light of an aqueous solution containing the photosensitizer (Rf or RB) and the substrate in a home-made photolyser.Citation31,Citation32 The equipment has a 150 W quartz-halogen lamp (OSRAM XENOPHOT HPLX 64640) focused according to experience, in a reaction cell hermetically sealed with an oxygen electrode (ORION 97–08 specific for dissolved oxygen, coupled to an Orion 720 pH meter), or 1 × 1 cm spectrophotometric cuvette. In all cases the solution was continuously stirred. Additionally, the equipment has appropriate cut-off filters in order to remove radiation below 350 nm and to ensure the light to be only absorbed by the sensitizers.
The reactive rate constant for the chemical reaction of O2(1Δg) with Vanco (kr, see Scheme ) was determined by using the method described by Foote and Ching,Citation33 for which the knowledge of the reactive rate constant for the photooxidation of a reference compound R is required (equation (Equation1(1) )).
(1)
slopeVanco and slopeR are the respective slope of the first-order plots of oxygen consumption by Vanco and by the reference compound. RB was used as dye-sensitizer in the determination of kr. Assuming that the reaction of O2(1Δg) with the quencher is the only possible pathway of oxygen consumption, the ratio of the first-order slope of oxygen uptake by the substrate and the reference compound, each at the same concentration (slopeVanco/slopeR) yields kr/krR. The reference was FFA, with reported pH-independent krR valueCitation34 of 1.2 × 108 M−1 s−1 (FFA react with O2(1Δg) and is currently used as a reference for kinetic determination in photodynamic action).Citation35
Scheme 2 Possible reactions pathways for the visible-light mediated photosensitized degradation of Vanco (A) and reaction of ROS with specific auxiliary quenchers. S: sensitizer (Rose Bengal or riboflavin).
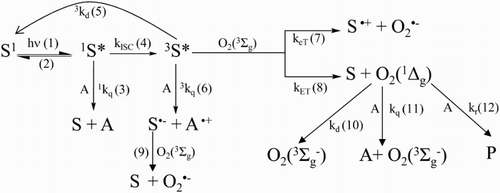
To establish the possible involvement of different ROS in the sensitized photodegradation of Vanco, several experiments of oxygen uptake vs. irradiation time were carried out. The relative rates of oxygen consumption (v−ΔO2 ) were evaluated in the presence and in the absence of specific ROS scavengers.
The aerobic and anaerobic photodegradation rates of Rf were evaluated from the decrease in the 445 nm absorption band as a function of the irradiation time.
Ground state absorption spectra were registered in a Hewlett Packard 8453A diode array spectrophotometer. If a more accurate determination was required, a Shimadzu UV-2401 double beam equipment was used for blank correction.
Laser flash photolysis experiment
Argon-saturated 0.02 mM Rf aqueous solution was photolysed using a flash photolysis apparatus with the frequency-double output of a Nd-YAG, Lasser System Spectron, SL400 where 355 nm was used as excitation wavelength, employing a 150 W Xenon lamp as a source for the analyzing light. The detection system comprised a PTI monochromator and a PMT red-extended photomultiplier (Hamamatsu R666). The signal, acquired and averaged by a digital oscilloscope (Agilent Technologies DSO 6012 A), was transferred to a PC via HPIB parallel interface, where it was analyzed and stored.Citation36
3Rf* disappearance, a species generated by the 355 nm pulse, was monitored from the first-order decay of the absorbance at 670 nm, a zone where the interference from other possible species is negligible. To avoid self-quenching and triplet–triplet annihilation, the triplet decays were measured at low Rf concentrations and at rather low laser energy.
For the determination of the rate constant of the interaction between 3RF* and Vanco (3
kq, see Scheme 2), the Stern–Volmer expression shown in equation (Equation2(2) ) was employed,
(2)
where 3
τ and 3
τ0 are the experimentally determined lifetimes of 3Rf* in the presence and in the absence of Vanco, respectively.
Transient absorption spectra were determined in Argon-saturated 0.02 mM Rf and 0.02 mM Rf + 0.05 mM vancomycin aqueous solution using the flash photolysis apparatus described above.
Quantum yield of •RfH generation (Φ•RfH) by electron transfer was determined in aqueous solution relative to the triplet yield of 3Rf* in the same solvent (ΦT = 0.5),Citation37,Citation38 employing equation (3):(3)
where OD
T
is the absorbance of 3Rf* at 670 nm immediately after the laser pulse, OD
R
is the absorbance of •RfH at 570 nm, ϵ
T
is the molar absorption coefficientCitation39 of 3Rf* (4.4 × 103 M−1 cm−1) and ϵ
R
corresponds to molar absorption coefficientCitation40 of •RfH (5.1 × 103 M−1 cm−1).
Time-resolved phosphorescence detection of O2(1Δg)
The total quenching rate constant of deactivation of O2(1Δg) by Vanco (kt, see Scheme 2) was determined using a system previously reported.Citation41 Briefly, it consisted of a Nd:YAG laser (Spectron Laser System, SL400) as excitation source. The frequency-doubled output at 532 nm was employed to excite the photosensitizer RB. The emitted radiation O2(1Δg) phosphorescence, 1270 nm was detected at right angles using an amplified Judson J16/8Sp germanium detector, after passing through the appropriate filters. The output of the detector was coupled to a digital oscilloscope Agilent Technologies DSO 6012 A and to a personal computer to carry out the signal processing. Usually, in order to achieve a good signal to noise ratio, 6–8 shots are needed for averaging from which the decay time was determined.Citation42 Air-equilibrated solutions were employed in all cases.
The absorbance of the photosensitizer RB was 0.2 at the laser excitation wavelength. The decay kinetics was first order in all cases. D2O was employed in the time-resolved determinations of O2(1Δg) phosphorescence in order to enlarge the lifetime of this species.Citation33,Citation43
The O2(1Δg) lifetimes were evaluated in the presence (τ) and in the absence (τ0) of Vanco and the data were plotted as a function of substrate concentration, according to a simple Stern–Volmer treatment, equation (4):(4)
Bactericidal activity evaluation
The standard strain S. aureus ATCC 25923 was used in the present study. Microbial cells were grown aerobically in sterile conditions on a rotator shaker at 100 rpm overnight at 37°C in 4 ml of tryptic soy broth (TSB).
The number of microorganisms was determined by the spread plate technique as described below. An aliquot (20 µl) of S. aureus strain overnight culture was aseptically transferred to 50 ml of fresh medium (TSB). This microbial suspension was distributed in Pirex® brand culture tubes (13 mm × 100 mm) and the Vanco-sensitizer solution was added from a stock solution in water, so that the final concentration in the tubes was 0.75 mg/l, before and after photolysis. Bacterial cultures without Vanco were grown under the same conditions to serve as controls. All tubes were incubated with stirring at 37°C for 2.5 h in order to observe the effect. After that, cellular suspensions were serially diluted with PBS. Each solution was spread on TSA and the number of colonies formed after 18–24 h incubation at 37°C in the dark was determined.
The experiment was performed in triplicate.
Result and discussion
Proposed kinetic scheme
The possible processes that take place when a solution containing Vanco (A) and a dye photosensitizer S (Rf or RB, in the present case) is irradiated with visible light in the presence of oxygen are shown in Scheme 2, as well as the respective rate constants. The proposed mechanisms include photoinduced reactions in the presence and in the absence of Vanco.
Upon absorption of a photon, S gives rise to its excited singlet state (1S*) (process 1) and through intersystem crossing, to its excited triplet state (3S*) (process 4). 3S* can transfer an electron or energy to the ground state oxygen (O2(3Σg−)), in the aerated solution, generating superoxide radical anion (O2 • −) (process 7) and singlet oxygen (O2(1Δg)) (process 8), respectively. The species O2 • − can be also produced through an indirect process via the reaction of an electron donor with 3S* (process 6 and 9).
On the other hand, O2(1Δg) can decay by collision with solvent molecules (process 10), and can interact physically and/or chemically with the substrate A (process 11 and 12, respectively).
Stationary irradiation of solution of vancomycin/Rf
As a consequence of irradiation of air-equilibrate solutions of Vanco (0.1 mM) with visible light in the presence of the Rf dye (0.02 mM) in a buffer at pH 7.4, spectral changes are observed (Fig. ). No modifications occur in the absence of light, indicating that there is no association between Rf and Vanco in the fundamental state.
Figure 1 Spectral changes of 0.1 mM vancomycin + 0.02 mM riboflavin vs. 0.02 mM riboflavin upon visible light photoirradiation in aqueous solution at pH 7.4. The numbers on the spectra represent irradiation time in min. Inset I: Relative rates of oxygen uptake (v−ΔO2) of: (a) 0.02 mM riboflavin + 0.5 mM vancomycin; (b) 0.02 mM riboflavin + 0.5 mM vancomycin + 1 mM sodium azide; (c) 0.02 mM riboflavin + 0.5 mM vancomycin + 1 mg/100 ml superoxide dismutase; (d) 0.02 mM riboflavin + 0.5 mM vancomycin + 10 mM d-mannitol; (e) 0.02 mM riboflavin + 0.5 mM vancomycin + 1 mg/100 ml catalase. Inset II: Spectral changes in Argon-saturated solution of 0.02 mM riboflavin upon visible light photoirradaition (a) without vancomycin and non-irradiated; (b) without vancomycin, 5 minutes irradiation; (c) in the presence of 0.5 mM vancomycin, 5 minutes irradiation. Solvent: aqueous solution at pH 7.4.
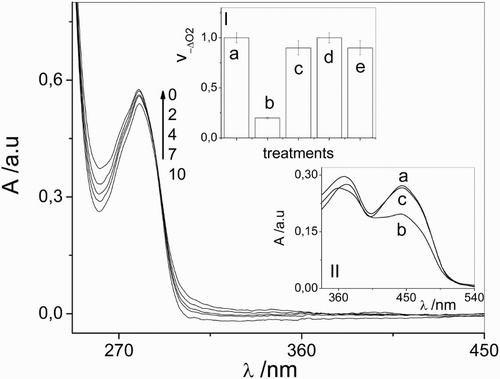
The modification observed in the absorption spectra can be mainly attributed to the chemical transformation of Rf and/or in the antibiotic.
It is worth mentioning that, in Fig. , there is no presence of the absorption spectra of Rf due to the fact that the photosensitizer was used as a blank.
In parallel experiment, oxygen consumption was detected upon irradiation of solution containing 0.02 mM Rf and 0.5 mM Vanco. In order to elucidate the nature of ROS involved in Rf-photosensitized process, experiments of oxygen uptake in the presence and in the absence of additives with ROS-scavenging capacity: NaN3, SOD, CAT, and d-mannitol were made. As shown in inset I of Fig. , the rate of oxygen consumption was clearly diminished in the presence of 1 mM NaN3 as compared to the respective oxygen consumption in the absence of the salt. This salt is an exclusive physical quencher of O2 (1Δg) with a rate constant of 3 × 108 M−1 s−1 in aqueous solution (process 13) and has been used previously,Citation33 in similar concentration, to ascertain whether O2(1Δg) participates in a given reaction mechanism. Then, these results suggest the involvement of O2(1Δg) as a potential reaction pathway in Vanco photodegradation (Rf generates O2(1Δg) in water with a quantum yield of 0.48, process 8).Citation44
Since Rf also can produce O2•− (process 9, Scheme 2), the same experiment was performed in the presence of 1 mg/100 ml SOD. SOD, which catalyses the specifically dismutation of O2•− through the process 14, has been utilized as a quencher of O2•−-mediated photooxidation, in similar concentration to those employed in this work.Citation40,Citation45,Citation46 However, no change was observed in the relative rate of oxygen uptake in the presence of this enzyme, demonstrating that O2•− was not initially involved in the Vanco photooxidative process.
Due to the fact that eventually other ROS such as •OH and H2O2 can be generated from O2•−,Citation47,Citation48 the oxygen consumptions of Vanco in the presence of specifically scavengers for •OH and H2O2 (10 mM d-mannitol and 1 mg/100 ml CAT, respectively) have been investigated. The species •OH reacts with d-mannitol by the process 15 and H2O2 is decomposed by CAT according to process 16:
However, as shown in the inset I of Fig. , the relative rate of the oxygen consumption of individual Vanco/Rf system was practically the same in the presence of d-mannitol and CAT. This fact could indicate that, initially, the mentioned species (•OH and H2O2) are not involved in the photooxidation mechanism.
On the other hand, we observed that both the anaerobic and aerobic photodegradation rates of Rf, processes that are well known to occur from electronically excited states of the vitamin (anaerobically)Citation15 and/or from autosensitisation via O2(1Δg) (aerobically),Citation12 suffer a decrease in the presence of Vanco, in a mM concentration. This fact, illustrated in Fig. (inset II), suggests that either Rf electronic excited states or ROS produced through these states, or even both processes operating simultaneously, are responsible for the photodegradation of Vanco.
Based on the experimental evidences shown, we decided to carry out a systematic kinetic study in order to elucidate the possible processes involved in the Rf-sensitized degradation of the antibiotic studied.
Quenching of Rf electronically excited states
The participation of singlet excited Rf (1Rf*, process 3) in the photodegradative pathway of Vanco can be disregarded. Since a lifetime of ca. 4.7 ns has been reportedCitation39,Citation49 for 1Rf*, a Vanco concentration in the sub-mM range, similar to that employed in the present work, is not enough to significantly intercept the excited singlet species of the vitamin.
The specific interaction of 3Rf* with Vanco was studied by the laser flash photolysis experiment in the absence of oxygen. A reduction of 3Rf* lifetime was observed in the presence of Vanco, supporting the occurrence of process 6 between the antibiotic and 3Rf*, for which a bimolecular rate constant value 3 kq = 7.19 × 109 M–1 s–1 was graphically determined, as shown in the Stern–Volmer plot in Fig. , inset I. The equation used was previously described in the experimental section.
Figure 2 Transient absorption spectra of (a) 0.01 mM riboflavin and (b) 0.01 mM riboflavin + 0.05 mM vancomycin taken at 1 and 30 μs after the laser pulse, respectively, in Argon-saturated aqueous solutions. Inset I: Stern–Volmer plot for the interaction of 3Rf* with vancomycin. Inset II: transient absorption spectra of (a) 0.01 mM riboflavin (1 μs) and (b) 0.01 mM riboflavin + 0.05 mM vancomycin (30 μs) normalized at 670 nm, in Argon-saturated aqueous solutions.
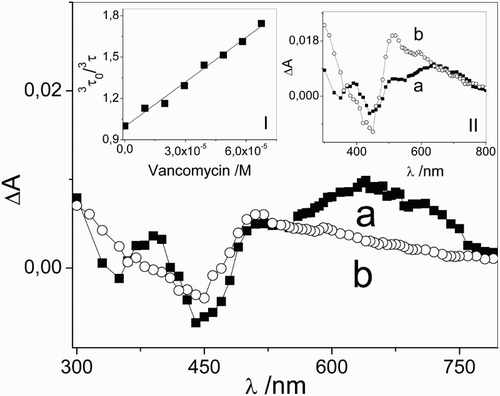
The 3Rf–Vanco interaction was also studied by recording the transient absorption spectra in Argon-saturated aqueous solution at pH 7.4. In the absence of the antibiotic, a similar spectrum to the expected one for 3Rf* was observedCitation37,Citation39,Citation50 after the laser pulse, while in the presence of 0.05 mM (ca. 90% 3Rf* quenched by Vanco) the spectrum, determined at 30 µs after the laser pulse and in identical experimental conditions, shows an important decay in the region between 600 and 750 nm (Fig. ). The occurrence of absorption between 500 and 600 nm in the normalized spectrum at 670 nm (Fig. , inset II) is attributedCitation39,Citation51 to the semiquinone radical of Rf (•RfH). At pH 7, this species (pKa = 8.3) must be formed after protonation of the species Rf•−. •RfH has been formerly detected as a product of electron transfer process to 3Rf* from several electron-donor substrates of environmental and biological importance.Citation15,Citation49,Citation52,Citation53 A quantum yield for the semireduced Rf generation (Φ•RfH) was determined from equation (3) (explained in the experimental section), and the value obtained was 0.59.
The results obtained from the laser flash photolysis experiment indicate that the electron transfer process is the main mechanism of Vanco besides reaction via O2(1Δg).
The predominance of Vanco photooxidation upon reaction with O2(1Δg) or the radical process will depend on the competition between O2(3∑g − ) and the antibiotic for the quenching of 3Rf*. This competition can be evaluated in kinetic terms, as follows:
3Rf* deactivation occurs with a rate constant for the energy transfer, kET, in water of ca. 7 × 108 M−1 s−1, i.e. ca. 1/9 of the value of a diffusional process.Citation54,Citation55 From this value and the 3 kq (71.9 × 107 M−1 s−1) by assuming a concentration of 0.2 mM for the dissolved O2(3∑g − ) in air-saturated aqueous solution,Citation56 it appears that, under our experimental conditions ([Vanco] = 0.5 mM), 3 kq [Vanco] > kET [O2(3Σg−)], the predominant process occurs via Rf•–.
Although experiments with ROS-scavengers demonstrate pronounced effect of NaN3 and no effect of SOD, the results obtained in this study could indicate that the species O2•− possibly generated via electron transfer between 3Rf* and Vanco, as observed from laser flash photolysis experiments, but the antibiotic is not susceptible to oxidation by this species, while it is sensitive to the action of O2(1Δg) generated by energy transfer between 3Rf* and O2(3∑g − ).
Quenching of O2 (1Δg) by vancomycin – effect of pH
In the O2(1Δg)-mediated experiments at pH 7.4 and 11, the xantenic dye RB was employed as a photosensitizer instead of Rf. Rf was not used in this kinetic study in order to avoid interferences by other oxidative species generated in the medium, and because this pigment suffers a rapid photodegradation in alkaline conditions. RB is one of the most commonly employed photosensitizer in O2(1Δg) reactions.Citation14 It generates this oxidative species in a predominant fashion, with a quantum yield of ca. 0.7 in aqueous media.Citation57,Citation58
When an aerated solution of Vanco (0.1 mM) in the presence of RB (A549 nm = 0.5) at pH 7.4 and 11 was irradiated with visible light, changes in the absorption spectra of the antibiotic were observed.
The magnitude of the spectral changes was different at the two pH values studied. The reactivity under alkaline medium (Fig. ) was much higher than that observed at pH 7.4 (Fig. , inset I). It needs to be clarified that the spectra shown in Fig. were taken using RB as a blank. Moreover, the maximum absorption wavelength of RB in water at pH 7.4 is 549 nm. For this reason, the spectral modifications observed at 500 nm in Fig. cannot be assigned to the photobleaching of this sensitizer.
Figure 3 Spectral evolution of 0.1 mM vancomycin upon sensitized photoirradiation with visible light in aqueous solution in the presence of Rose Bengal (A549 nm = 0.5) of vancomycin/Rose Bengal vs. Rose Bengal at pH 11. Inset: Spectral evolution of 0.1 mM vancomycin upon sensitized photoirradiation with visible light in aqueous solution in the presence of Rose Bengal (A549 nm = 0.5) of vancomycin/Rose Bengal vs. Rose Bengal at pH 7.4. The numbers on the spectra represent irradiation time in minuite.
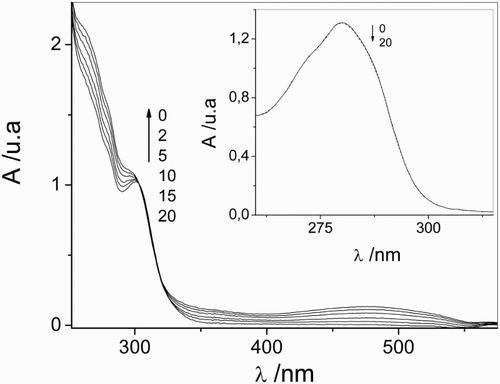
In parallel, the rate constant of the overall reaction of Vanco with O2(1Δg) (kt) was determined employing time-resolved detection of O2(1Δg) phosphorescence. kt is defined as the sum of the rate constant of physical deactivation to ground state molecular oxygen (kq, process 11), and the chemical or reactive quenching (kr, process 12). The latter was determined by comparative method already described in the experimental section.
As well as in the absorption spectra, the same effect with the pH was observed in the first-order plots (Fig. ) and in the Stern–Volmer plots (Fig. , inset I) for the determination of kr and kt, respectively. The values obtained for the rates constant at each pH are given in Table .
Figure 4 First-order plots for 0.5 mM vancomycin consumption plus Rose Bengal (A549 nm = 0.5) in aqueous solution at (a) pH 7.4 and (b) pH 11. Inset: Stern–Volmer plots for the quenching of O2 (1Δg)-phosphorescence emission by vancomycin in Rose Bengal (A532 nm = 0.3)/D2O at (a) pD 7.4 and (b) pD 11.
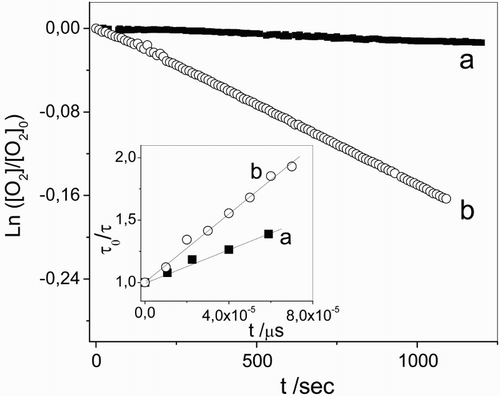
Table 1 Overall (kt) and reactive (kr) rate constants for the interaction of O2(1Δg) with vancomycin at pH/pD 7.4 and 11 in the presence of RB; kr/kt ratio
The combination of stationary and time-resolved experiments unambiguously demonstrates the interaction of O2(1Δg) with the antibiotic, especially in alkaline conditions.
A useful approach is the evaluation of the kr/kt ratio (included in Table ), i.e. the effective fraction of overall quenching that leads to chemical reaction.
Table clearly shows that both kr and kt values and the ratio kr/kt increase with increasing of pH of the medium. This behavior can be explained on the basis that the OH-ionization in OH-aromatic compounds produces an enhancement in the electron-donor ability of the compound and, hence, an increase in its O2(1Δg) quenching ability. The mechanism may involve an intermediate complex possessing charge-transfer character extensively studied.Citation59,Citation60 The deactivation of the encounter complex will merely lead to physical quenching, while complete electron transfer will produce chemical oxidation of the substrate. The balance between physical and chemical quenching is a sensitive function of spin–orbit coupling properties and entropy factors.
Vanco has multiple phenolic groups in its structure, in alkaline condition a large number of molecules will have the –OH group ionized (pKa4 = 9.59, pKa5 = 10.4, pKa6 = 12),Citation61 therefore, the chemical reaction with O2(1Δg) in this condition, is predominant.
A similar effect was observed for the β lactam antibiotic amoxicillin, in the presence of OH-ionized group at alkaline pH results in higher reactivity with O2(1Δg) compared with that obtained at physiological pH and compared to another β- lactam antibiotic, cephalexin, which has a similar chemical structure but no OH ionizable group.Citation9
Microbiological experiment
Since the photochemical experiments indicate that Vanco is sensitive to oxidation by O2(1Δg), the impact of the sensitized irradiation of Vanco on their bactericidal effect power was qualitatively tested in the presence of the sensitizer. It has been demonstratedCitation62,Citation63 that Rf, under UVA irradiation (365 nm), has antimicrobial properties against common pathogens such as S. aureus, Pseudomona aeruginosa, and Staphylococcus epidermidis. For this reason, the sensitizer RB was used instead of Rf in these microbiological assays. This dye, as it was mentioned before, is an exclusively O2(1Δg)-generatorCitation14 and it has been employed as photosensitizer in several microbiological tests in order to evaluate the bactericidal activity in a parallel fashion with the photodegradation of different drugs.
The result obtained (Fig. ) shows significant variation in the number of microorganisms treated with 0.75 mg/l of Vanco with respect to S. aureus culture without treatment.
Figure 5 Effect of 0.75 mg/l vancomycin: (c) before and (d) after 2.5 h Rose Bengal-sensitized photolysis on S. aureus ATCC 25923; (a) cellular control without vancomycin; (b) cellular control with vancomycin and without Rose Bengal.
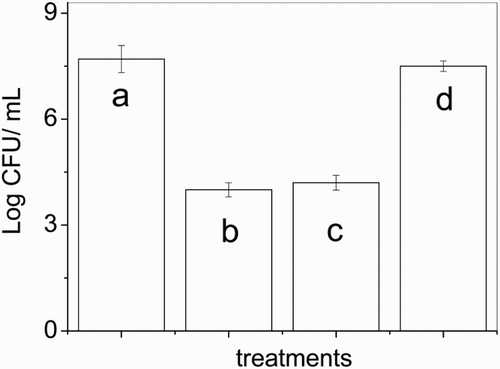
A diminution of 3.5 log in relation to culture control was expected because of the bactericidal effect of this antibiotic.
On the other hand, after the Vanco photolysis, antimicrobial activity was deeply affected. A difference of 3.1 log is observed, compared to the intact Vanco. This result proves the loss of bactericidal effect by Vanco as a consequence of photosensitized-degradation on studied S. aureus strains, under our experimental conditions. This fact may be suggested that the photoproducts, which may eventually arise as a result of the oxidative process, lose the bactericidal properties of the original Vanco.
Wammer et al.Citation64 use a similar bacterial assay to determine loss of activity of three fluoroquinolones under direct photolysis. They found that norfloxacin and ofloxacin photoproducts are inactive; however, enrofloxacin photoproducts do retain significant antibacterial activity on E. coli strain.
On the other hand, other families of antibiotics such as tetracycline, sulfa drugs and some β-lactam such as amoxicillin, cefalexin, ceftriaxone, and cefotaxime, suffer sensitized photooxidation with concomitant loss of antimicrobial effect against B. subtilis, P. aeruginosa, and S. aureus.Citation7–Citation10 Besides, the loss of antimicrobial activity under other photodegradative treatments such Fenton and Photo-Fenton,Citation65 have also been observed for ampicillin photoproducts on S. aureus strains.
Conclusions
The results obtained and discussed in this work, demonstrate that Rf can raise the photodegradation of Vanco by both the radical pathway and the O2(1Δg) mediated. Furthermore, the antibiotic is able to interact with the excited electronic states of Rf as well as O2(1Δg) generated by energy transfer between the excited triplet state of the photosensitizer and the oxygen ground state. The predominant mechanism for photodegradation of Vanco in the presence of the Rf is the radical via because of the considerable interaction with the excited triplet state of the photosensitizer demonstrated by laser flash photolysis experiments.
In the presence of RB, the process occurs via O2(1Δg) and it is favored under alkaline conditions due to ionization of the phenolic groups present in the chemical structure of the antibiotic. Photooxidation reaction between O2(1Δg) and Vanco can be explained by a mechanism that involves the formation of an encounter complex that possesses partial charge-transfer character, acting O2(1Δg) as an electron acceptor, which stabilizes in the presence of electron donating groups and can be deactivated by chemical and/or physical reaction. Finally, the evidence found on the microbiological test shows that the bactericidal capacity of Vanco is affected by photodegradation sensitized process on Staphylococcus aureus ATCC 25923.
The results obtained in this work demonstrate that the processes of sensitized photodegradation of antibiotics can contribute as a tool for the elimination of these compounds of the environment.
Disclaimer statements
Contributors All authors contributed equally.
Funding CONICET (11220110100279).
Conflict of interest There is no conflict of interest.
Ethics approval Ethical approval is not required.
Acknowledgements
Financial support from Consejo Nacional de Investigaciones Científicas y Técnicas (CONICET) and Secretaría de Ciencia y Tecnica de la Universidad Nacional de Río Cuarto (SECyT UNRC), both from Argentine, is gratefully acknowledged. We are grateful to Prof. Mabel Cieri who participated in the reading and editing of the final manuscript of this work, making the necessary correction as far as the English version is concerned. (Prof. Mabel Cieri works a ‘Profesora Superior de Ingles’ at U.N.R.C).
References
- Murgida DH, Aramendia PF, Balsells RE. Photosensitized oxidation of oxopurines by Rose Bengal. Photochem. Photobiol. 1998;68:467–473. doi: 10.1111/j.1751-1097.1998.tb02501.x
- Díaz M, Luiz M, Bertolotti S, Miskoski S, García NA. Scavenging of photogenerated singlet molecular oxygen and superoxide radical anion by sulpha drugs. Kinetics and mechanism. J. Chem. 2004;82:1752–1759.
- García NA, Criado SN, Massad WA. Riboflavin as a visible-light-sensitiser in aerobic photodegradation of ophthalmic and sympathomimetic drugs. In: Silva E, Edwards AM, (eds.) Comprehensive series in photosciences. Flavins: photochemistry and photobiology. Cambridge: The Royal Society of Chemistry; 2006. p. 61–82.
- Trovó AG, Santos Melo SA, Nogueira RFP. Photodegradation of the pharmaceuticals amoxicillin, bezafibrate and paracetamol by the photo-Fenton process—application to sewage treatment plant effluent. J. Photochem. Photobiol. A 2008;198:215–220. doi: 10.1016/j.jphotochem.2008.03.011
- Jung YJ, Kim WG, Yoon Y, Kang JW, Hong YM, Kim HW. Removal of amoxicillin by UV and UV/H2O2 processes. Sci. Total Environ. 2012;420:160–167. doi: 10.1016/j.scitotenv.2011.12.011
- Yang L, Yu LE, Ray MB. Degradation of paracetamol in aqueous solutions by TiO2 photocatalysis. Wat. Res. 2008;42:3480–3488. doi: 10.1016/j.watres.2008.04.023
- Posadaz A, Sánchez E, Gutiérrez MI, Calderón M, Bertolotti S, Biasutti MA, et al. Riboflavin and Rose Bengal sensitized photooxidation of sulphathiazole and succinylsuphathiazole. Kinetic study and microbiological implications. Dyes Pigments 2000;45:219–228. doi: 10.1016/S0143-7208(00)00010-3
- Castillo C, Criado S, Diaz M, García NA. Riboflavin as a sensitiser in the photodegradation of tetracyclines. Kinetics, mechanism and microbiological implications. Dyes Pigments 2007;72:178–184. doi: 10.1016/j.dyepig.2005.08.025
- Reynoso E, Nesci A, Allegretti P, Criado S, Biasutti MA. Kinetic and mechanistic aspects of sensitized photodegradation of β-lactam antibiotics: microbiological implications. Red. Rep. 2012;17:275–283. doi: 10.1179/1351000212Y.0000000032
- Reynoso E, Spesia MB, García NA, Biasutti MA, Criado S. Riboflavin-sensitized photooxidation of ceftriaxone and cefotaxime. Kinetic study and effect on Staphylococcus aureus. J. Photochem. Photobiol. B 2015;142:35–42. doi: 10.1016/j.jphotobiol.2014.11.004
- Momzikoff A, Santus R, Giraud M. A study of the photosensitizing properties of seawater. Marine Chem. 1983;12:1–14. doi: 10.1016/0304-4203(83)90024-5
- Chacon JN, McLearie J, Sinclair RS. Singlet oxygen yields and radical contributions in the dye-sensitised photo-oxidation in methanol of esters of polyunsatured fatty acids (oleic, linoleic, linolenic and arachidonic). Photochem. Photobiol. 1988;47:647–656. doi: 10.1111/j.1751-1097.1988.tb02760.x
- Edwards AM, Silva E. Effect of visible light on selected enzymes vitamins and amino acids. J. Photochem. Photobiol. B 2001;63:126–131. doi: 10.1016/S1011-1344(01)00209-3
- Neckers DC. Rose Bengal. Review. J. Photochem. Photobiol. A 1989;47:1–29. doi: 10.1016/1010-6030(89)85002-6
- Heelis PF. The photophysical and photochemical properties of flavins (isoalloxazines). Chem. Soc. Rev. 1982;11:15–39. doi: 10.1039/cs9821100015
- Heelis PF. The photochemistry of flavinas. In: Müller F, (ed.) Chemistry and biochemistry of flavoenzymes, Boca Ratón, FL: CRC Press; 1991.
- Halling-Sorensen B, Nielsen SN, Lanzky PF, Ingerslev F, Lutzhft HCH, Jorgensen SE. Occurrence, fate and effects of pharmaceutical substances in the environment – a review. Chemosphere 1998;36:357–393. doi: 10.1016/S0045-6535(97)00354-8
- Kümmerer K. Antibiotics in the aquatic environment – a review – part I. Chemosphere 2009;75:417–434. doi: 10.1016/j.chemosphere.2008.11.086
- Kümmerer K. Antibiotics in the aquatic environment – a review – part II. Chemosphere 2009;75:435–441. doi: 10.1016/j.chemosphere.2008.12.006
- Hirsch R, Ternes T, Haberer K, Kratz KL. Occurrence of antibiotics in the aquatic environment. Sci. Total Environ. 1999;225:109–118. doi: 10.1016/S0048-9697(98)00337-4
- Zwiener C, Gremm TJ, Frimmel FH. Pharmaceuticals in the environment: sources fate, effects, and risks. Weinheim, Germany: Springer; 2004.
- Andreozzi R, Canterino M, Marotta R, Paxeus N. Antibiotic removal from waste waters: the ozonation of amoxicillin. J. Hazard. Mater. 2005;122:243–250. doi: 10.1016/j.jhazmat.2005.03.004
- Gartiser S, Urich E, Alexy R, Kümmerer K. Ultimate biodegradation and elimination of antibiotics in inherent tests. Chemosphere 2007;67:604–613. doi: 10.1016/j.chemosphere.2006.08.038
- Gilliver MA, Bennett M, Begon M, Hazel SM, Hart CA. Enterobacteria: antibiotic resistance found in wild rodents. Nature 1999;401:233–234. doi: 10.1038/45724
- Gould IM. A review of the role of antibiotic policies in the control of antibiotic resistance. J. Antimicrob. Chemother. 1999;43:459–465. doi: 10.1093/jac/43.4.459
- Johnson JLH, Yalkowsky SH. Reformulation of a new vancomycin analog: an example of the importance of buffer species and strength. AAPS Pharm. Sci. Technol. 2006;7:E1–E5.
- Wolter F, Schoof S, Süssmuth RD. Synopsis of structural, biosynthetic, and chemical aspects of glycopeptide antibiotics. Top. Curr. Chem. 2007;267:143–185. doi: 10.1007/128_041
- Sinha B, Fraunholz M. Staphylococcus aureus host cell invasion and post-invasion events. Int. J. Med. Microbiol. 2010;300:170–175. doi: 10.1016/j.ijmm.2009.08.019
- Mensa J, Gatell JM, Azanza JR, Dominguez-Gil A, García JE, Jiménez de anta MT, et al. Guía terapéutica antimicrobiana. 18th ed. Barcelona: Elsevier Masson; 2008.
- West R. (Ed). Handbook of chemitry and physics. Boca Ratón, FL: CRC; 1981.
- Miskoski S, García NA. Dark and photoinduced interactions between riboflavin and indole auxins. Collect. Czechoslovak Chem. Commun. 1991;56:1838–1849. doi: 10.1135/cccc19911838
- Bertolotti SG, Arguello GA, García NA. Effect of the peptide bond on the singlet molecular oxygen mediated photooxidation of tyrosine and tryptophan dipeptides. A kinetic study. J. Photochem. Photobiol. B 1991;10:57–70. doi: 10.1016/1011-1344(91)80212-Z
- Foote S, Ching TY. Chemistry of singlet oxygen XXI. Kinetics of bilirubin photooxygenation. J. Am. Chem. Soc. 1975;97:6209–6214. doi: 10.1021/ja00854a045
- Wilkinson F, Helman WP, Ross AB. Rate constant for the decay and reaction of the lowest electronically excited state of molecular oxygen in solution. An extended and revised compilation. J. Phys. Chem. Ref. Data 1995;24:663–1021. doi: 10.1063/1.555965
- Scully FE, Hoigné J. Rate constant for the reaction of singlet oxygen with phenols and other compound in water. Chemosphere 1987;16:694–699. doi: 10.1016/0045-6535(87)90004-X
- Díaz M, Luiz M, Alegretti P, Furlong J, Amat-Guerri F, Massad W, et al. Visible-light-mediated photodegradation of 17β-estardiol: kinetics, mechanism and photoproducts. J. Photochem. Photobiol. A 2009;202:221–227. doi: 10.1016/j.jphotochem.2008.12.008
- Moore WM, McLearie J, McDaniels JC, Hen JA. The photochemistry of Riboflavin-VI. The photophysical properties of isoalloxacine. Photochem. Photobiol. 1977;25:505–512. doi: 10.1111/j.1751-1097.1977.tb09120.x
- Orellana B, Rufs AM, Encinas MV, Previtali CM, Bertolotti S. The photoinitiation mechanism of vinyl polymerization by Riboflavin/triethanolamine in aqueous medium. Macromolecules 1999;32:6570–6573. doi: 10.1021/ma990946x
- Schreiner S, Steiner U, Kramer HEA. Determination of the pK values of the Lumiflavin triplet state by flash photolysis. Photochem. Photobiol. 1975;21:81–84. doi: 10.1111/j.1751-1097.1975.tb06632.x
- Encinas MV, Rufs AM, Bertolotti S, Previtali CM. Free radical polymerization photoinitiated by riboflavin/amines. Effect of the amine structure. Macromolecules 2001;34:2845–2847. doi: 10.1021/ma001649r
- Criado S, Bertolotti S, García NA. Kinetic aspect of the rose Bengal-sensitized photo-oxygenation of tryptophan alkyl esters. J. Photochem. Photobiol. B 1996;34:79–86. doi: 10.1016/1011-1344(95)07274-8
- Schweitzer C, Scmidt R. Physical mechanisms of generation and deactivation of singlet oxygen. Chem. Rev. 2003;103:1685–1757. doi: 10.1021/cr010371d
- Nonell SL, Moncayo F, Trull F, Amat-Guerri F, Lissi E, Soltermann AT. Solvent influence on the kinetics of the photodynamic degradation of Trolox, a water-soluble model compound for vitamin E. J. Photochem. Photobiol. B 1995;29:157–162. doi: 10.1016/1011-1344(95)07137-Q
- Krishna CM, Uppuluri S, Riesz P, Zigler JS, Balasubramanian D. A study of the photodynamic efficiencies of some eye lens constituents. Photochem. Photobiol. 1991;54:51–58. doi: 10.1111/j.1751-1097.1991.tb01984.x
- Baxer RM, Carey JH. Evidence for photochemical generation of superoxide ion in humic water. Nature 1983;306:575–576. doi: 10.1038/306575a0
- Zang PG, Misra HP. Superoxide radicals generated during the autooxidation of 1-methyl-4-phenyl-2,3-dihydropyridinium perchlorate. J. Biol. Chem. 1992;267:17547–17552.
- Iuliano L, Pratico D, Ghiselli A, Bonavita MS, Violi F. Reaction of dipyridamole with hydroxyl radical. Lipids 1992;27:349–353. doi: 10.1007/BF02536149
- Silva E, Ugarte R, Andrade A, Edwards AM. Riboflavin-sensitised photoprocesses of tryptophan. J. Photochem. Photobiol. B: Biol. 1994;23:43–48. doi: 10.1016/1011-1344(93)06984-B
- Criado SN, García NA. Vitamin B2-sensitised photooxidation of the ophthalmic drugs Timolol and pindolol. Kinetics and mechanism. Red. Rep. 2004;9:291–297. doi: 10.1179/135100004225006047
- Haggi E, Bertolotti S, Miskoski S, Amat-Guerri F, García NA. Environmental photodegradation of pyrimidine fungicides. Kinetics of the visible-light-promoted interactions between riboflavin and 2-amino-4-hydroxy-6-methylpyrimidine. Can. J. Chem. 2002;80:62–67. doi: 10.1139/v01-192
- Bertolotti S, Previtali CM, Rufs AM, Encinas MV. Riboflavin/triethanolamine as photoinitiator system of vinyl polymerization. A mechanistic study by laser flash photolysis. Macromolecules 1999;32:2920–2924. doi: 10.1021/ma981246f
- Massad WA, Bertolotti SG, García NA. Visible-light-induced degradation of medicament. Kinetic and mechanism of the Vitamin B2-sensitized photooxidation of isoproterenol. Photochem. Photobiol. 2004;79:428–433. doi: 10.1562/RA-028R.1
- Pajares A, Bregliani M, Montaña P, Criado S, Massad W, Gianotti J, et al. Visible-light promoted photoprocesses on aqueous gallic acid in the presence of riboflavin. Kinetics and mechanism. J. Photochem. Photobiol. A 2010;209:89–94. doi: 10.1016/j.jphotochem.2009.10.011
- Koizumi M, Kato S, Mataga N, Matsuura T, Isui I. Photosensitized reactions. Kyoto: Kagakudogin; 1978.
- Calvert J, Pitts J Jr. Photochemistry. New York: John Wiley & Sons; 1966.
- Murov SL. Handbook of photochemistry. New York: M. Decker; 1973.
- Wilkinson F, Helman WP, Ross AB. Quantum yields for the photosensitized formation of the lowest electronically excited singlet state of molecular oxygen in solution. J. Phys. Chem. Ref. Data 1993;22:133–262. doi: 10.1063/1.555934
- Lambert CR, Kochevar IE. Does Rose Bengal generate superoxide anion? J. Am. Chem. Soc. 1996;118:3297–3298. doi: 10.1021/ja9600800
- García NA. New trends in photobiology: singlet-molecular-oxygen-mediated photodegradation of aquatic phenolic pollutants. A kinetic and mechanistic overview. J. Photochem. Photobiol. B 1994;22:185–196. doi: 10.1016/1011-1344(93)06932-S
- Soltermann AT, Luiz M, Biasutti MA, Carrascoso M, Amat-Guerri F, García NA. Monosubtituted naphthalenes as quenchers and generators of singlet molecular oxygen. J. Photochem. Photobiol. A 1999;129:25–32. doi: 10.1016/S1010-6030(99)00172-0
- Takács-Novák K, Noszál B, Tokes-Kovesdi M, Szasz G. Acid base properties and proton-speciation of vancomycin. Int. J. Pharm. 1993;89:261–263. doi: 10.1016/0378-5173(93)90252-B
- Martins SAR, Castro Combs J, Noguera G, Camacho W, Wittmann P, Walther R, et al. Antimicrobial efficacy of riboflavin/UVAcombination (365 nm) in vitro for bacterial and fungal isolates: a potential new treatment for infectious keratitis, invest. Ophthalmol. Visual Sci. 2008;49:3402–3408. doi: 10.1167/iovs.07-1592
- Makdoumi K, Mortensen J, Sorkhabi O, Malmvall B, Crafoord S. UVA riboflavin photochemical therapy of bacterial keratitis: a pilot study. Graefes Arch. Clin. Exp. Ophthalmol. 2012;250:95–102. doi: 10.1007/s00417-011-1754-1
- Wammer KH, Korte AR, Lundeen RA, Sundberg JE, McNeill K, Arnold WA. Direct photochemistry of three fluoroquinolone antibacterials: Norfloxacin, ofloxacin, and enrofloxacin. Water Res. 2013;47:439–448. doi: 10.1016/j.watres.2012.10.025
- Rozas O, Contreras D, Mondaca MA, Pérez-Moya M, Mansilla HD. Experimental design of Fenton and photo-Fenton reactions for the treatment of ampicillin solutions. J. Hazard. Mater. 2010;177:1025–1030. doi: 10.1016/j.jhazmat.2010.01.023