Abstract
The effects of fluorobenzaldehydes (2-,3- and 4-fluorobenzaldehyde) on the activity of mushroom tyrosinase have been studied. The results show that fluorobenzaldehydes can strongly inhibit both monophenolase activity and diphenolase activity of the enzyme and the inhibition is reversible. The IC50 values were estimated as 1.62 mM, 1.06 mM and 0.16 mM for diphenolase activity and as 1.35 mM, 1.18 mM and 1.05 mM for monophenolase activity, respectively. The lag time of the monophenolase was obviously lengthened by these three fluorobenzaldehydes. When the concentration of inhibitors reached 2.0 mM, the lag time was lengthened from 33 s to 142 s, 168 s and 190 s, respectively. Kinetic analyses show that the inhibition mechanism of 2-fluorobenzaldehyde on the diphenolase was competitive inhibition of the diphenolase activity, and that of 3-fluorobenzaldehyde and 4-fluorobenzaldehyde were of a mixed-type. The inhibition constants for these three fluorobenzaldehydes on the diphenolase were determined and compared.
Introduction
Tyrosinase (EC 1.14.18.1) is a copper-containing enzyme that is widely distributed in microorganisms, animals, and plants. It is the responsible for not only melanization in animals but also browning in plants Citation1-3. The browning is an undesirable reaction that is responsible for a less attractive appearance and a loss in nutritional quality. It becomes a major problem in the food industry and is one of the main causes of quality loss during postharvest handling and processing [Citation4]. Therefore, control of tyrosinase activity is important in preventing the synthesis of melanin in the browning of mushrooms. In addition, tyrosinase inhibitors could be clinically useful for the treatment of some dermatological disorders associated with melanin hyperpigmentation [Citation5] and also important in cosmetics for whitening and depigmentation after sunburn [Citation6]. In addition, tyrosinase is known to be involved in the moulting process of insects [Citation5]. Consequently, tyrosinase inhibitors should have broad applications.
It is well known that tyrosinase can be inhibited by aromatic aldehydes [Citation7] and acids [Citation8], tropolone [Citation9] and kojic acid [Citation10]. In our continuing investigation of tyrosinase inhibitors, alkylbenzaldehydes were found to inhibit the monophenolase activity and o-diphenolase activity of mushroom tyrosinase, and were shown to be a reversible noncompetitive inhibitors for the oxidation of l-3,4-dihydroxyphenylalanine [Citation11]. In our efforts to develop new, low-cost, easy-to-prepare and highly potent tyrosinase inhibitors, fluorobenzaldehydes 2-,3- and 4-fluorobenzaldehyde, were investigated by carrying out a kinetic study of the inhibition on the monophenolase and o-diphenolase activity of mushroom tyrosinase, with an evaluation of the kinetic parameters and constants characterizing the system.
Materials and methods
Mushroom tyrosinase (EC 1.14.18.1), 2-fluorobenzaldehyde (a); 3-fluorobenzaldehyde (b) and 4-fluorobenzaldehyde (c) (see for structures) were purchased from Sigma (St. Louis, MO, USA). Dimethyl sulfoxide (DMSO), l-3,4-dihydroxyphenylalanine (l-DOPA) and l-tyrosine (Tyr) were obtained from Aldrich (St. Louis, MO, USA). All other reagents were local analytical grade. Re-distillated and ion-free water was used.
Figure 1 Chemical structure of 2-fluorobenzaldehyde (a), 3-fluorobenzaldehyde (b), and 4-fluorobenzaldehyde (c).
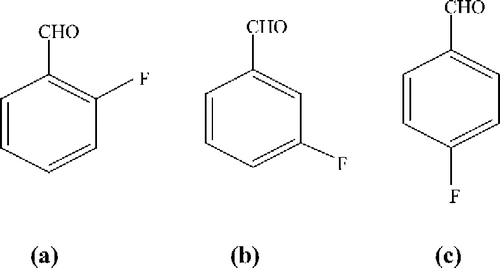
The monophenolase activity and diphenolase activity assay was performed as reported by Chen et al. [Citation11]. In this investigation, Tyr was used as the substrate for the monophenolase activity assay, and l-DOPA was used as the substrate for the diphenolase activity assay. The assay reaction media (3 ml) for contained 2.0 mM Tyr or 0.5 mM l-DOPA in 50 mM Na2HPO4–NaH2PO4 buffer (pH 6.8). The final concentration of mushroom tyrosinase was 33.33 μg/ml for the monophenolase activity and 6.67 μg/ml for the o-diphenolase activity. Enzyme activity was determined by following the increase in absorbance at 475 nm accompanying the oxidation of the substrates with a molar absorption coefficient of 3700 (M− 1 cm− 1) for the product [Citation12] by using a Beckman UV-650 spectrophotometer at 30°C.
The inhibitor was dissolved in DMSO and diluted 30-fold for the experiment; the final concentration of DMSO in the test solution was 3.3%. The extent of inhibition by the addition of the sample was expressed as the percentage necessary for 50% inhibition (IC50). Controls, without inhibitor but containing 3.3% DMSO, were routinely carried out. The inhibition type was determined by a Lineweaver-Burk plot, and the inhibition constant was determined by secondary plots of the apparent Km/Vmax or 1/Vmax versus the concentration of the inhibitor as described by Chen [Citation13].
Results
Inhibitory effects of fluorobenzaldehydes on the monophenolase activity of mushroom tyrosinase
Tyrosinase can catalyze the hydroxylation of monophenols (monophenolase activity) and the oxidation of o-diphenols to o-quinones (diphenolase activity), both depending on molecular oxygen [Citation14]. The o-quinones evolve non-enzymatically to yield several unstable intermediates, which then polymerize to give melanins. When the enzymatic oxidation reaction used 2.0 mM of Tyr as substrate, the time course was shown as curve 0 in . There was a marked lag period, characteristic of monophenolase activity, which was estimated by extrapolation curve to the abscissa (the arrow in ). The system reached a constant rate (the steady-state rate) after the lag period. The effects of fluorobenzaldehydes on the oxidation of Tyr by tyrosinase were studied. The kinetic course of the oxidation of the substrate in the presence of 1.0 mM of (a), (b) and (c) are shown as curves 1, 2, and 3 in , respectively. The results showed that all of these three compounds have inhibitory effects on the monophenolase activity of mushroom tyrosinase. The lag time for the oxidation of Tyr by the enzyme inhibited by (a), (b) and (c) was determined. The results showed that the lag time observably increased with increasing concentration of the compounds as shown in . The lag time was estimated to be 33 seconds in the absence of the compound. With the concentrations of the compounds (a), (b) and (c) at 2.0 mM, the lag time was extended to 142, 168 and 190 seconds respectively, an increase of 3.30, 4.09 and 4.76 fold. On the other hand, the steady-state rate (vss) decreased exponentially with increasing concentration of the compounds as shown in . With the concentration of compounds (a), (b) and (c) at 2.0 mM, the remaining enzyme activity was found to be 41%, 37% and 33%, respectively. The inhibitor concentration (IC50) leading to a loss of 50% activity was estimated to be 1.35 mM (for compound a), 1.18 mM (b) and 1.05 mM (c). The results obtained are summarized in . The results indicated that inhibitor potency follows the order: 4-fluorobenzaldehyde > 3-fluorobenzaldehyde > 2-fluorobenzaldehyde.
Figure 2 Course of the oxidation of Tyr by mushroom tyrosinase in absence of inhibitor (curve 1), and in the presence of 1.0 mM of inhibitor: 2-fluorobenzaldehyde (curve 2), 3-fluorobenzaldehyde (curve3) and 4-fluorobenzaldehyde (curve 4). Assay conditions were 3 ml reaction system containing 50 mM Na2HPO4–NaH2PO4 buffer (pH 6.8), 2.0 mM Tyr, 33.3 μg/ml of enzyme, and 3.3% DMSO. The arrows show the lag time.
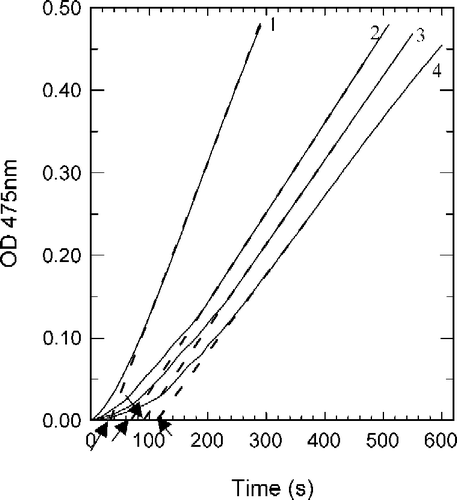
Figure 3 Effects on the lag time of mushroom tyrosinase for the catalysis of Tyr by different concentrations of 2-fluorobenzaldehyde (a), 3-fluorobenzaldehyde (b), and 4-fluorobenzaldehyde (c). Assay conditions were described in .
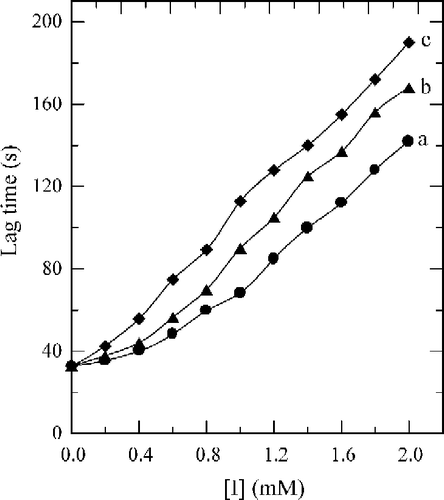
Figure 4 Effects on the steady-state rate of mushroom tyrosinase for the catalysis of Tyr by different concentrations of 2-fluorobenzaldehyde (a), 3-fluorobenzaldehyde (b), and 4-fluorobenzaldehyde (c). Assay conditions were described in .
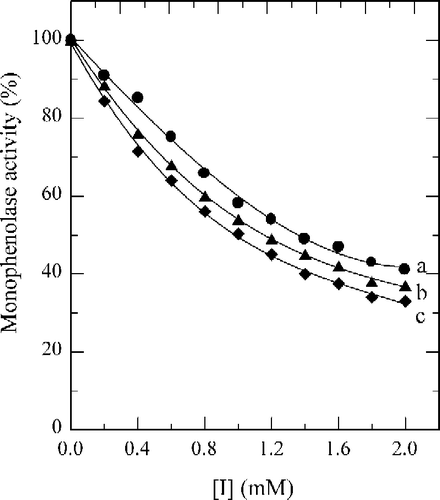
Table I. Kinetics and inhibition constants of fluorobenzaldehydes on mushroom tyrosinase.
Concentration effects of fluorobenzaldehydes on the diphenolase activity of mushroom tyrosinase
When the diphenolase activity of tyrosinase was assayed by using l-DOPA as substrate, the reaction course immediately reached a steady-state rate. The time course was a straight line that passed through the origin as shown as the line 0 in . When the compounds (a), (b), and (c) were tested for their effects on the oxidation of l-DOPA by mushroom tyrosinase, the time courses gave the lines 1–3 in , respectively; they all passed through the origin with different slopes. On increasing the concentrations of fluorobenzaldehydes, the diphenolase activity of mushroom tyrosinase markedly decreased in a concentration-dependent manner (). From the , the IC50 values, of compounds (a), (b) and (c) were estimated to be 1.62 mM, 1.06 mM and 0.16 mM, respectively.
Figure 5 Course of the oxidation of l-DOPA by mushroom tyrosinase in absence of inhibitor (line 1), and in the presence of 1.0 mM of inhibitor: 2-fluorobenzaldehyde (line 2), 3-fluorobenzaldehyde (line 3) and 4-fluorobenzaldehyde (line 4). Assay conditions were 3 ml reaction system containing 50 mM Na2HPO4–NaH2PO4 buffer (pH 6.8), 0.5 mM l-DOPA, 6.67 μg/ml of enzyme, and 3.3% DMSO.
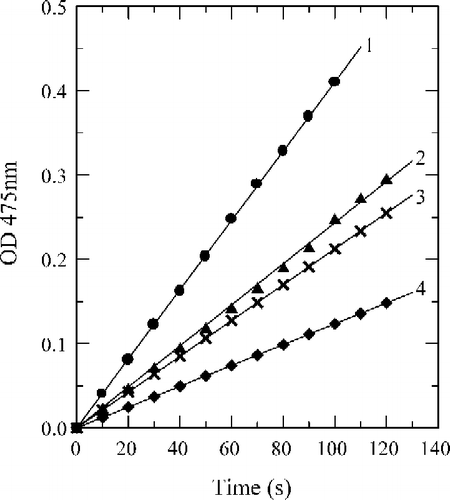
The inhibition by fluorobenzaldehydes of the diphenolase activity of mushroom tyrosinase was shown to be reversible
Taking 2-fluorobenzaldehyde (a), 3-fluorobenzaldehyde (b) and 4-fluorobenzaldehyde (c) as inhibitors, we studied their inhibition mechanism on the enzyme for the oxidation of l-DOPA. Plots of the remaining enzyme activity versus the concentration of enzyme at different inhibitor concentrations gave a family of straight lines, which all passed through the origin (). Increasing the inhibitor concentration resulted in a decrease in the slope of the line, indicating that the inhibition by fluorobenzaldehydes was reversible. The presence of these inhibitors did not decrease the amount of efficient enzyme, but just resulted in its inhibition and a decrease in the activity of the enzyme for oxidation of l-DOPA.
Inhibition type and inhibition constants for fluorobenzaldehydes on the diphenolase activity of mushroom tyrosinase
The kinetic behavior of mushroom tyrosinase during the oxidation of l-DOPA was studied. Under the conditions employed in the present investigation, the oxidation reaction of l-DOPA by mushroom tyrosinase follows Michaelis-Menten kinetics. In the presence of 2-fluorobenzaldehyde (a), a kinetic study of the enzyme inhibition by a Lineweaver-Burk plot is shown in . The results showed that compound (a) was a competitive inhibitor since increasing the concentration of compound (a) resulted in a family of lines with a common intercept on the ordinate but with different slopes. The equilibrium constant for inhibitor binding with free enzyme, was obtained from a plot of the apparent Michaelis-Menten constant (Km) versus the inhibitor concentration, which was linear as shown in the inset. Using 3-fluorobenzaldehyde (b) as inhibitor, the Lineweaver-Burk plot () showed that compound (b) was a mixed-type inhibitor since increasing its concentration resulted in a family of lines with different slope and intercept, but they intersected with one another in the second quadrant. This behavior is observed when the inhibitor can bind not only with free enzyme, but also with the enzyme-substrate complex, and their equilibrium constants are different. The inhibition constant for the inhibitor binding with the free enzyme (E), KI, was obtained from a plot of the slopes of the straight lines versus the inhibitor concentrations ( inset). The inhibition constant for the inhibitor binding with the enzyme-substrate complex (ES), KIS, was obtained from a plot of the intercepts of the straight lines versus the inhibitor concentrations ( inset). The values obtained are summarized in . Similar results were obtained with compound (c) which was also a mixed-type inhibitor; KI and KIS values were obtained from secondary plots and are summarized in .
Figure 8 Lineweaver-Burk plots for inhibition of 2-fluorobenzaldehyde (a) on the oxidation of DOPA by mushroom tyrosinase. Concentration of (a) for curves 1–5 was 0, 0.25, 0.50, 0.75, 1.00 mM, respectively. The inset represents the plot of Km versus the concentration of (a) to determine the inhibition constant. The line is drawn using linear least squares fit.
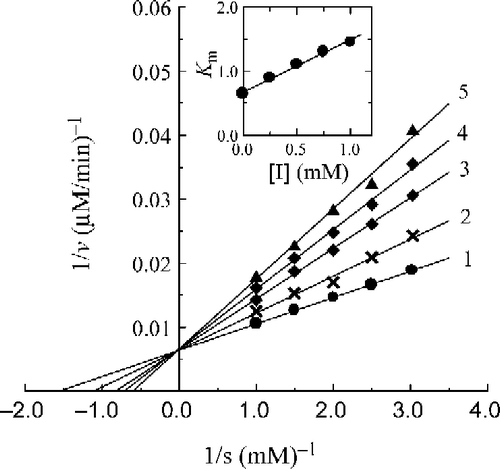
Figure 9 Lineweaver-Burk plots for inhibition by 3-fluorobenzaldehyde (b) on the oxidation of DOPA by mushroom tyrosinase. Concentration of (b) for curves 1–5 was 0, 0.25, 0.50, 0.75, 1.00 mM, respectively. The insets represent the plot of the slope and the intercept versus the concentration of (b) to determine the inhibition constant. The line is drawn using linear least squares fit.
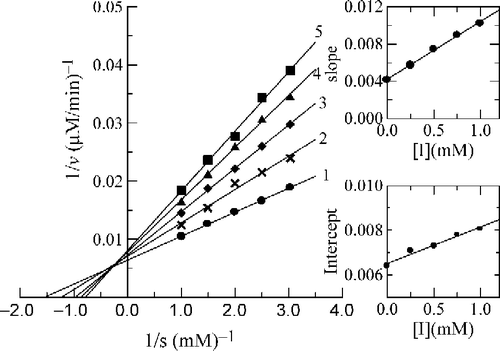
Discussion
Tyrosinase has two distinct catalytic functions: the hydroxylation of monophenol and the oxidation of o-diphenol. Here, we report the effects of fluorobenzaldehydes on the hydroxylation of Tyr and the oxidation of l-DOPA by mushroom tyrosinase. The results show that these three fluorobenzaldehydes have inhibitory effects on both the monophenolase and the diphenolase activity of the enzyme. For diphenolase activity, fluorobenzaldehydes displayed reversible inhibition. The inhibitory mechanism for the ortho-substitued fluorobenzaldehyde was a competitive type, but the meta- and the para-substitued fluorobenzaldehydes displayed a mixed-type inhibitory mechanism, indicating a different inhibitory mechanism. During catalysis, tyrosinase has exists in three forms, Emet, Eoxy and Edeoxy. Both the Emet form and the Eoxy form can catalyze the diphenol substrate (l-DOPA); the Eoxy form can also catalyze the monophenol substrate (Tyr) but the Emet form cannot. The Edeoxy form can bind with oxygen. If inhibitor only binds with free enzyme molecule in the Emet form and Eoxy form, to become EmetI and EoxyI, respectively, it will be a competitive inhibitor for the diphenolase. However if the inhibitor only binds with enzyme-substrate complex, in the EmetD form and EoxyD form, it will be an uncompetitive inhibitor for the diphenolase. From the results, it can be seen that compound (a) can bind with the free enzyme forms (Emet and Eoxy) but cannot bind the enzyme-substrate complexes (EmetD or EoxyD), and so displayed a competitive type of inhibition. The meta- and para-substituted fluorobenzaldehydes, however, can bind with both free enzyme and enzyme-substrate complexes, and they displayed a mixed-type. The above conclusion was supported by another experiment using Tyr as substrate. When the enzymatic reaction was started by the action of tyrosinase on Tyr, a marked lag period was observed simultaneously with the appearance of the first stable product, dopachrome. The system reached a constant rate (the steady-state rate) after the lag period. If the inhibitor binds with the free enzyme, it can not only lengthen the lag period but also decrease the steady-state rate. If the inhibitor just binds with the enzyme-substrate complex, it can only decrease the steady-state rate but not lengthen the lag time. Chen et al. reported that o-tolualdehyde and m-tolualdehyde could bind both free enzyme and enzyme-substrate complex, and displayed a mixed-type inhibitory mechanism for the diphenolase activity [Citation11]. o-Tolualdehyde and m-tolualdehyde could lengthen the lag period for the monophenolase. p-Isopropylbenzaldehyde was a uncompetitive inhibitor for the diphenolase and could not lengthen the lag period for the monophenolase activity [Citation11].
Walker and Wilson suggested the existence of two distinct sites on tyrosinase: one for the binding of the substrate and another, adjacent, site for binding the inhibitor [Citation15]. The results from the inhibitory type studies lead us to suppose that 2-fluorobenzaldehyde attached to the active site and hindered the binding of substrate to the enzyme, but 3-fluorobenzaldehyde and 4-fluorobenzaldehyde could attach to both the active site and the binding site of the inhibitor. It also suggested that substitution by a fluorine atom in the para-position improves the inhibitory character of the molecule and the inhibitory mechanism for fluorine substitution is different from that of hydroxy or alkyl group substitution, because para-hydroxy benzaldehyde was a competitive inhibitor [Citation16] and para-alkylbenzaldehydes were uncompetitive inhibitors [Citation11]. The inhibition potency among fluorobenzaldehydes used here follows the order: 4-fluorobenzaldehyde (c) > 3-fluorobenzaldehyde (b) > 2-fluorobenzaldehyde (a). This difference may be explained by the different electron-donating capacity of the substitution at different positions. However, irrespective of substitution position the fluorobenzaldehydes used here were effective inhibitors on mushroom tyrosinase.
Acknowledgements
The present investigation was supported by Grant 2002AA245011 from the National High Technology Research and Development of China (863 Program) and by Grant 2004N002 of the Science and Technology Foundation of Fujian Province.
References
- Martínez MV, Whitaker JR. The biochemistry and control of enzymatic browning. Trends Food Sci Technol 1995; 6: 195–200
- Espín JC, Jolivet S, Overeem A, Wichers HJ. Agaritine from Agaricus bisporus is capable of preventing melanin formation. Phytochemistry 1999; 50: 555–563
- Whitaker JR. Food enzymes, structure and mechanism, DWS Wong, New York 1995; 271–307
- Friedman M. Food browning and its prevention: An overview. J Agric Food Chem 1996; 44: 631–653
- Mosher DB, Pathak MA, Fitzpatrick TB, et al. Vitiligo, etiology, pathogenesis, diagnosis, and treatment, TB Fitzpatrick, New York 1983; 205–225
- Nerya O, Musa RM, Khatib S, Tamir S, Vaya J. Chalcones as potent tyrosinase inhibitors: The effect of hydroxyl positions and numbers. Phytochemistry 2004; 65: 1389–1395
- Chen QX, Song KK, Wang Q, Huang HJ. Inhibitory effects of mushroom tyrosinase by some alkylbenzaldehydes. J Enz Inhib Med Chem 2003; 18: 491–496
- Song KK, Chen QX, Wang Q, Qiu L, Huang H. Inhibitory effects of 4-vinylbenzaldehyde and 4-vinylbenzoic acid on the activity of mushroom tyrosinase J Enz Inhib Med Chem 2004. In press.
- Espín JC, Wichers HJ. Slow-binding inhibition of mushroom (Agaricus bisporus) tyrosinase isoforms by tropolone. J Agric Food Chem 1999; 47: 2638–2644
- Kahn V, Ben-Shalom N, Zakin V. Effect of kojic acid on the oxidation of N-acetyldopamine by mushroom tyrosinase. J Agric Food Chem 1997; 45: 4460–4465
- Chen Q, Chen QX, Qiu L, Song KK, Huang H. Inhibitory effect of 4-cyanobenzaldehyde and 4-cyanobenzoic acid on mushroom (Agaricus bisporus) tyrosinase. J Protein Chem 2003; 22(7)607–612
- Jiménez M, Chazarra S, Escribano J, Cabanes J, Garcia-Carmína F. Competitive inhibition of mushroom tyrosinase by 4-substituted benzaldehydes. J Agric Food Chem 2001; 49: 4060–4063
- Chen QX, Zhou HM. Inhibitors of green crab (Scylla serrata) alkaline phosphatase. J Enz Inhib 1999; 14: 251–257
- Sánchez-Ferrer A, Rodríguez-López JN, García-Cánovas F, García-Carmona F. Tyrosinase: A comprehensive review of its mechanism. Biochim Biophys Acta 1995; 1247: 1–11
- Walker JRL, Wilson EL. Studies on the enzymatic browning of apples. Inhibition of apple o-diphenol oxidase by phenolics acid. J Sci Food Agric 1975; 26: 1825–1831
- Huang H, Liu XD, Chen QX. Studies on mushroom tyrosinase activity inhibited by benzaldehyde family compounds. J Xiamen Univ 2003; 42(1)97–101