Abstract
Among the structurally related flavonoids tested on the bovine kidney low molecular weight protein tyrosine phosphatase (LMrPTP) activity, quercetin activated by about 2.6-fold the p-nitrophenyl-phosphate (p-NPP)-directed reaction, in contrast to morin that acted as a competitive inhibitor, with Ki values of 87, 73 and 50 μM for p-NPP, FMN, and tyrosine-phosphate, respectively. Other related flavonoids, such as rutin, kaempferol, catechin, narigin, phloretin and taxifolin did not significantly affect the LMrPTP activity.
The positions of the hydroxyl groups in the structures of the flavonoids were important for their distinct effects on LMrPTP activity. The hydroxyl groups at C3′ and C4′ and the presence of a double bond at C2 and C3 were essential for the activating effect of quercetin. The absence of the 3′-OH (kaempferol), absence of the double bond (taxifolin) and the presence of the sugar rutinose at the 3-OH (rutin) suppressed the effect of quercetin. The C2′- and C4′-hydroxyl groups, the presence of the double bond, and a C4-ketone group were important requirements for the inhibitory effects of morin.
Introduction
The phosphorylation of proteins at tyrosine residues is regulated by the opposing activities of protein tyrosine quinases, which catalyze tyrosine phosphorylation, and protein tyrosine phosphatases, which catalyze tyrosine dephosphorylation [Citation1,Citation2]. The protein tyrosine phosphatases (PTPs) (EC 3.1.3.48) are key regulatory enzymes that participate in signal transduction [Citation2,Citation3] and are essential for regulating cellular growth, differentiation, cell cycle, gene transcription, immune response and other processes Citation4-9. Based on structure and substrate specificity, the PTPs can be divided into four distinct subfamilies: tyrosine-specific PTP, with exclusive tyrosine specificity; dual-specific PTPs, VH1-like, which also hydrolyze phosphorylated serine and threonine residues; Cdc25 phosphatases, related to the control of cell division; and low molecular mass PTPs (LMrPTPs), with no sequence similarity to the other two groups, except for the active site signature [Citation10,Citation11]. Despite significant diversity in their primary sequences, these proteins share a signature motif, (H/V)CxAGxxR(S/T)G, which includes catalytically essential cysteine and arginine residues [Citation10].
The LMrPTPs, basic and cytosolic enzymes expressed in all eukaryotic organisms, were originally studied as low molecular mass acid phosphatases (EC 3.1.3.2) [Citation12,Citation13]. These enzymes have very similar kinetic properties when compared with the other PTPs and play important roles in cell migration and adhesion [Citation14], cell differentiation [Citation15], neurogenesis [Citation16], and in cell proliferation acting as positive regulators of tumor onset and growth [Citation17].
The purification [Citation18], kinetic properties [Citation19,Citation20] and regulation by chemical compounds [Citation21,Citation22] of mammalian LMrPTPs have been studied in our laboratory. In relation to the regulation of bovine kidney LMrPTPs, differential effects could be observed depending on the structures of the chemical compounds. Among the purine compounds, guanosine and adenosine were activators and uric acid and adenine were inhibitors [Citation21], and in contrast to the cationic surfactants, the anionic surfactants promoted the denaturation of the enzyme [Citation22]. The differential effects exerted by purine compounds on LMr acid phosphatase could be ascribed to the different isoforms used Citation23-25.
The identification and utilization of compounds that alter the activities of enzymes that control the cell cycle are important tools in the understanding of kinetic mechanisms and in this context, the flavonoids are potential candidates. These polyphenolic compounds, largely distributed in the vegetable kingdom, have demonstrated several biological efffects, such as antiinflammatory, antiallergic, antiviral, antibacterial, antitumor, antioxidant and antihemorrhagic properties Citation26-30.
Flavonoids have shown inhibitory effects on several enzyme activities, such as proteases [Citation31], metallopeptidases [Citation32], H+, K+-ATPase [Citation33], DNA and RNA polymerases [Citation34], reverse transcriptase [Citation35,Citation36], tyrosinase [Citation37], farnesyl protein transferase [Citation38] and glutathione S-transferase [Citation39].
In relation to the effect of flavonoids on the signal transduction, some authors have reported effects of such compounds on the activities of protein kinases Citation40-43. However, effects of flavonoids on protein phosphatases activities have only been sparsely reported. Lee et al. [Citation44]. reported inhibitory effects of three flavonoids, norwogonin, dihydronorwogonin and baicalein on a dual-specificity protein tyrosine phosphatase.
The aim of this work was to study the effects of structurally-related flavonoids (quercetin, morin, rutin, kaempferol, catechin, naringin, taxifolin, myricetin and phloretin) on the activities of bovine kidney LMrPTP. Kinetic studies using three different substrates, p-nitrophenylphosphate, flavin mononucleotide and tyrosine phosphate are also described.
Materials And Methods
Materials
Bovine kidney LMr PTP (17.8 kDa) was purified 1,640-fold to homogeneity with a specific activity of 100 μmol min− 1 mg− 1 as previously described [Citation18]. All the flavonoids and the other chemicals used in this work were obtained from Sigma Chemical Co. (St Louis, MO, U.S.A.).
Methods
Protein tyrosine phosphatase assay
PTP activity was determined, as previously described [Citation45], in a final volume of 1 mL containing 100 mM acetate buffer (pH 5.0) and 5 mM substrate, by measuring the inorganic phosphate released, according to the Lowry-Lopez method [Citation46]. After 10 min of incubation at 37°C, the reaction was stopped by adding 1 ml of 3% (w/v) ammonium molybdate (in 200 mM acetate buffer, pH 4.0) followed by 0.1 ml of 120 mM ascorbic acid (in 200 mM acetate buffer, pH 4.0). After 30 min at room temperature, the absorbance of the resulting color was read at 700 nm and the amount of inorganic phosphate released was calculated using a molar extinction coefficient of 4,000 M− 1 cm− 1 [Citation46]. One unit of activity was defined as the amount of enzyme that catalyzes the formation of 1 μmol of inorganic phosphate per minute. under these conditions. All the determinations were done at least in triplicate.
Effect of flavonoids on enzyme activity
The effect of flavonoids on LMrPTP activity was studied by measuring the enzyme activity at 37°C in 100 mM acetate buffer with 5 mM substrate, as described above, in the absence (control) and presence of the desired flavonoid. The I50 value corresponds to the flavonoid concentration that produces 50% inhibition.
Determination of kinetic parameters
Km and Vmax values were determined from Lineweaver-Burk double reciprocal plots [Citation47]. Values of the inhibition constants (Ki) were determined from Dixon plots [Citation48], in the presence of three different concentrations of inhibitor.
Determination of the activation energy
The activation energy value was determined from the Arrhenius plot (log k versus 1/T). This plot was determined from an assay measuring LMrPTP activity at different temperatures using pNPP as substrate, in the absence and in the presence of quercetin.
Effect of pH on LMrPTP activity in the presence of quercetin or morin
Enzyme activity was determined with pNPP as substrate as described above, at different pH values, in the absence and in the presence of quercetin or morin. The following buffers (100 mM) were used: pH 2.5 and 9.0 (glycine), pH 3.0 (citrate), pH 3.5–5.5 (acetate), pH 6.0—7.0 (bis–tris), pH 8.0 (hepes).
Results
Among the flavonoids tested (quercetin, morin, rutin, phloretin, narigin, myricetin, catechin, taxifolin and kaempferol), only quercetin and morin showed significant effects on LMrPTP activity. At a concentration of 400 μM, quercetin activated the enzyme reaction about 2.6-fold but, on the other hand, morin inhibited the same reaction by 35%. Catechin, myricetin, phloretin and rutin were less effective as inhibitors, with inhibition values of 14, 24, 26 and 25%, respectively. The IC50 (concentration that achieves 50% enzyme inhibition) values obtained for morin and phloretin were 500 and 1,000 μM, respectively; no IC50 values were reached for catechin, myricetin and rutin (results not shown), The other flavonoids tested, narigin, taxifolin and kaempferol did not show any significative effect ().
Table I. Effect of flavonoids on LMrPTP activity. The enzyme activity was determined as described in Materials and Methods with p-NPP as substrate, in the absence (control) and in the presence of 400 μM of each flavonoid. All the data were obtained in triplicate and are shown with their standard deviations.
It is interesting to note that a slight modification of the chemical structure of the flavonoid () promoted large and differential effects on the enzyme activity. For instance, a simple change of the hydroxyl group position from C2′ of the chain B (morin) to the C3′ position (quercetin) greatly modified the flavonoid effect, which behaved as an activator instead of an inhibitor of the enzyme reaction. Quercetin has been reported to promote a potent relaxation of the ileum; the absence of a hydroxyl group at the C3′ position (and presence at C2′), observed for the flavonoid morin, or the substitution of a hydroxyl group at the C3 position for a sugar rutinose, observed for rutin, drastically diminished the biological effect [Citation49].
Figure 1 Structures of the flavonoids. The hydroxyl substituents are shown on the carbon atoms of the rings A and B for each flavonoid.
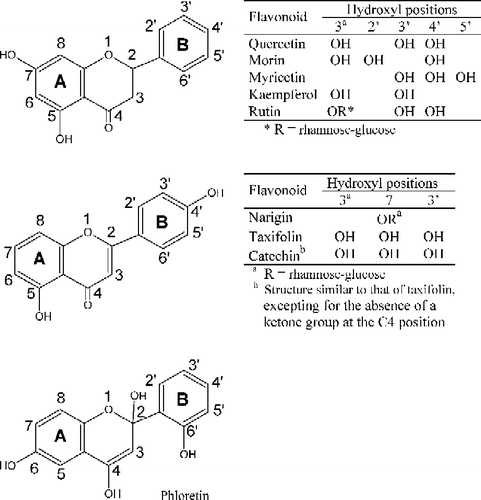
We observed that the activating effect of quercetin and inhibitory effect of morin were concentration-dependent (not shown) and that the concentration of 400 μM of flavonoids (used in ) was an adequate concentrations for studying the effects of these compounds.
Quercetin and morin, whose effects were more pronounced when compared with the other flavonoids, were selected for a more detailed kinetic study. The determination of Michaelis constant and activation energy values could give important information to explain the activating effect of quercetin. shows the effect of quercetin on the substrate pNPP concentration and the inset of this figure shows the Arrhenius plot used to determine the Km and activation energy values, respectively. summarizes the values of Vmax, Km, Vmax/Km (specificity constant) and activation energy, obtained from and inset of this figure. Despite the increase in the Vmax value in the presence of quercetin, the Km value also increased, suggesting that the activating effect of this flavonoid is not related to the increase in the enzyme-substrate affinity. The decrease in the specificity constant (Vmax/Km) value in the presence of quercetin reinforces this conclusion. On the other hand, the decrease of about 8 kJ/mol in the activation energy value obtained in the presence of quercetin could, at least partially, explain this activating effect. The activating effect of quercetin on LMrPTP was substrate-specific since no significant activations were observed with FMN and Tyr-P as substrates (not shown).
Figure 2 Effect of quercetin on LMrPTP activity with p-NPP as substrate. The enzyme activity was determined as described in Materials and methods by varying the concentrations of p-NPP as substrate, in the absence (▪) and in the presence (•) of 400 μM quercetin. Inset. Determination of the activation energy by Arrhenius plot. These data were obtained from an experiment of velocity versus temperature. Bars represent the s.e. of triplicate determinations.
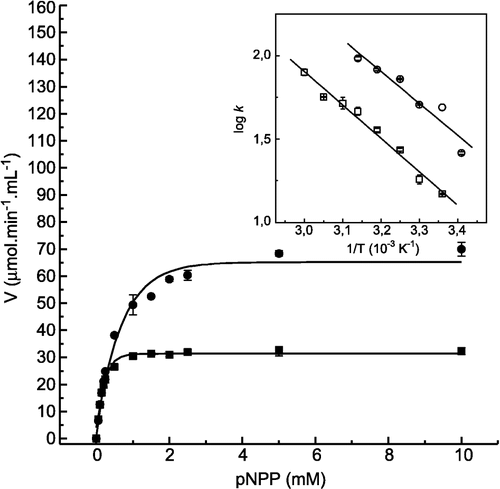
Table II. Effect of quercetin on the kinetic parameters of the reaction catalyzed by LMrPTP. The Km and Vmax values were calculated from Lineweaver-Burk plots (obtained from ). The activation energy value was determined from an Arrhenius plot (, inset).
In order to study in more detail the inhibitory effect of morin on LMrPTP activity, we determined the inhibition constant values, using as substrate p-nitrophenylphosphate (), FMN (), and tyrosine phosphate (). The Dixon plots (insets of these figures) show, for all the substrates tested, that the inhibition by morin was of the competitive type, with inhibition constant (Ki) values of 87 μM, 73 μM and 50 μM, for p-NPP, FMN and TyrP, respectively. Examining the ratio Km/Ki, which indicates the efficiency of inhibition, it was observed that the inhibition by morin using Tyr-P was stronger when compared with the other substrates (). This observation correlates well with the IC50 values which were 500 μM for FMN, and 10 μM for Tyr-P (not shown).
Figure 3 Inhibition of p-NPP hydrolysis by morin. The enzyme activity was determined as described in Materials and methods by varying the concentrations of p-NPP as substrate, in the absence (▪) and in the presence of 100 (•), 250 (▴) and 400 μM (▾) morin. Inset. Dixon plot for the determination of the Ki value for morin. Bars represent the s.e. of triplicate determinations.
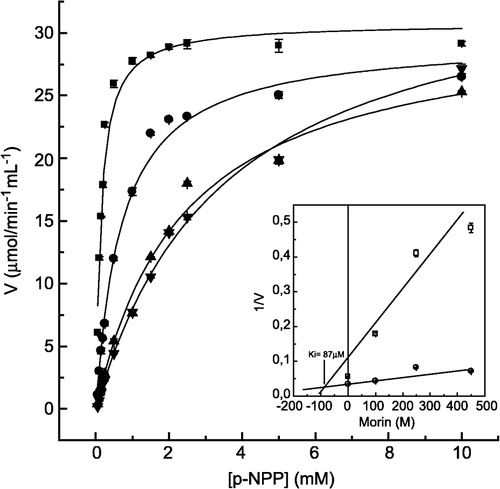
Figure 4 Inhibition of FMN hydrolysis by morin. The enzyme activity was determined as described in Materials and methods by varying the concentrations of FMN as substrate, in the absence (▪) and in the presence of 200 (•), 300 (▴) and 400 μM (▾) morin. Inset. Dixon plot for the determination of the Ki value for morin. Bars represent the s.e. of triplicate determinations.
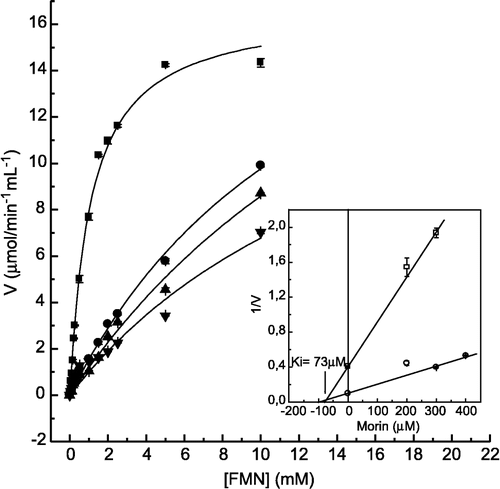
Figure 5 Inhibition of Tyr-P hydrolysis by morin. The enzyme activity was determined as described in Materials and methods by varying the concentrations of Tyr-P as substrate, in the absence (▪) and in the presence of 100 (•), 200 (▴) and 300 μM (▾) morin. Inset. Dixon plot for the determination of the Ki value for morin. Bars represent the s.e. of triplicate determinations.
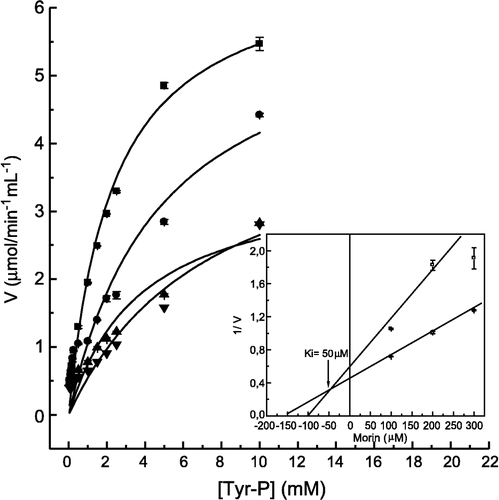
Table III. Kinetic parameters for the inhibition of LMrPTP by morin with different substrates. The Km values for FMN and Tyr-P were determined from Lineweaver-Burk plots (not shown), and the Ki values were determined as shown in the insets of the .
The activation by quercetin and the inhibition by morin were studied at different pH values of in order to evaluate the type of enzyme-flavonoid interaction. shows that the profile of the pH curve did not change in the presence of quercetin, with the maintenance of the optimum pH of enzyme catalysis (pH 5.0). However, in the presence of morin, there was a shift in the optimum pH from 5.0 to 6.0. Our results suggest that differently from the enzyme-morin interaction, the enzyme-quercetin interaction was not electrostatic and probably occurs by another kind of mechanism, for instance, a hydrophobic interaction between the aromatic ring of the flavonoid and hydrophobic specific sites of the enzyme. Another possibility is a hydrophilic interaction between the hydroxyl and ketonic groups of the rings A and B with the enzyme. Wang et al.[Citation50]. observed that adenine could lead and direct a water molecule to the LMrPTP active site, so promoting activation. Comparing the quercetin structure with the purine compound adenine, similarities could be observed in relation to the planar aromatic ring. In this case, quercetin with the hydroxyl groups at positions-3′ and-4′ could carry out the role of the purine adenine.
Figure 6 Effects of quercetin and morin on LMrPTP activity at different pH values. The enzyme activity was determined as described in Materials and methods with p-NPP as substrate, at different pH values, in the absence (▪) and in the presence of 400 μM quercetin. (•) or morin (▴). Bars represent the s.e. of triplicate determinations.
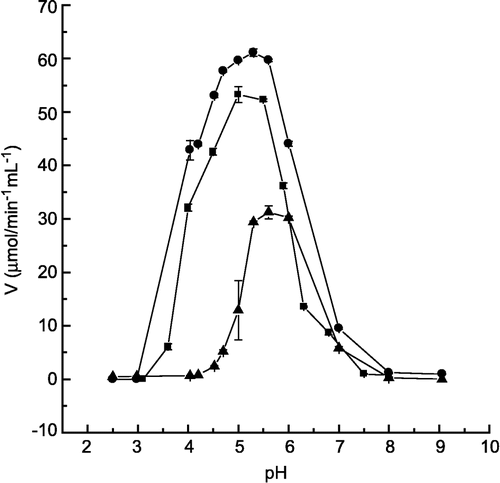
Discussion
The stucture-function relationship of flavonoids has been extensively studied in order to determine the mechanism of action of these compounds and to aid the rational drug design of potential pharmaceuticals.
Analysis of our results shows that it is possible to evaluate the effect of flavonoids on LMrPTP activity. Using p-NPP as substrate, this enzyme was activated by quercetin, and inhibited by morin and these effects were independent of the substrates p-NPP, FMN and Tyr-P used. Morin was selected as inhibitor when compared to phloretin due to its greater inhibitory effect (smaller IC50 value). Despite the inhibition shown by rutin, catechin and myricetin (), these flavonoids were not considered as inhibitors since their IC50 values could not be reached. Other flavonoids such as narigin, kaempferol and taxifolin had little or no significative effects on LMrPTP activity.
For the differential effects of the flavonoids, the positions of the hydroxyl groups in the B ring is of fundamental importance. These points possess high electron densities adequate for interactions such as intermolecular hydrogen bonds [Citation40]. Accordingly, quercetin, a very good activator, possesses hydroxyl groups at positions-3′ and-4′, appropriate to form a hydrogen bond with a water molecule. This mechanism could be similar to that proposed by Wang et al. [Citation50]. for the activation of LMrPTP by adenine, where a water molecule participated at the enzyme active site to improve catalysis. Nikolovska-Coleska et al.[Citation42]. demonstrated through classical and quantum chemical parameters that the para and meta positions in substituents at C 3′ and 4′ of the phenyl ring should have electron-donating properties and most probably this part of the flavonoid molecule could interact with the catalytic domain of the enzyme. The presence of the two hydroxyl groups in the B ring is important for the activating effect of quercetin, since kaempferol, where it lacks a hydroxyl group at C3′, has no significant effect. However, the presence of the hydroxyl groups alone is not sufficient to promote the activating effect of quercetin. The presence of the double bond between the C2 and C3 should be essential for this effect since taxifolin and catechin, where this bond is absent, presented no significant effect. In relation to the structure of taxifolin, catechin has an additional modification, in that the ketone group at C4 is absent. Finally, a free hydroxyl group at the C3 position is also important for the effect of quercetin; the presence of the sugar rutinose at this position, in the flavonoid rutin, suppressed the effect of quercetin.
Alternatively, morin, a good inhibitor, has the hydroxyl groups at positions-2′ and-4′, adequate for the formation of intramolecular hydrogen bonds, with no possibility of carrying a water molecule to the active site, but still having the ability to coordinate important enzyme functions for competition with substrates. It is worthwhile to mention that the hydroxyl groups of morin could interact with the sulfhydryl groups present at the active site of the LMrPTP, so inactivating the cysteine residues important for the catalysis.
Independently of the differential effects promoted by the flavonoids on enzyme activity, it the presence of a free hydroxyl group at C3 is essential. Glycosylation or methylation of the hydroxyl groups drastically diminished the effects of the flavonoids [Citation33]. The rhamnose-glucose sugar linked to the hydroxyl group at position-3 suppresses the effect of the double bond at carbons-2 and-3 so preventing the displacement of the electronic density responsible for the effect of the flavonoids.
It is also important to analyze the increase in the volume of the molecule which produces a steric impediment to the entry of the flavonoid into the active site of the enzyme [Citation42].
For the enzyme-flavonoid interaction the flavonoid must be in a structural conformation forming a 45° angle between the phenyl ring B and the remainder of the flavonoid structure. This is possible due to free rotation of the single bond that links the ring B to the rest of the flavonoid structure [Citation40].
Protein phosphorylation is governed by opposing activities of protein kinases and protein phosphatases, key enzymes in signal transduction. Consistently, our results with quercetin as an activator of LMrPTP correlate well with its role as an inhibitor of protein kinases, such as protein kinase C [Citation40,Citation41,Citation43]. The inhibitory effects on such enzyme activities by flavonoids could be used in studies on tumor proliferation, whose mechanism is not yet well understood.
Acknowledgements
This work was supported by grants from Fundação de Amparo à Pesquisa do Estado de São Paulo (FAPESP), Conselho Nacional de Desenvolvimento Científico e Tecnológico (CNPq), Fundo de Apoio ao Ensino e à Pesquisa (FAEP/UNICAMP) and a FAPESP scholarship to MAM. The authors are grateful to Dr. Fred Y. Fujiwara (Instituto de Química, UNICAMP) for critically reading the manuscript.
References
- Hunter T. Cell 2001; 100: 113–127
- Shade AE, Levine AD. Mol Immunol 2003; 40: 531–537
- Nell BG, Tonks NK. Curr Opin Cell Biol 1997; 9: 193–204
- Chernoff J. J Cell Physiol 1999; 180: 173–181
- Li LW, Dixon JE. Sem Immunol 2000; 12: 75–84
- Zhang ZY. Acc Chem Res 2003; 36: 385–392
- Aoyama H, Silva TMA, Miranda MA, Ferreira CV. Quim Nova 2003; 26: 896–900
- Dube N, Cheng A, Tremblay ML. Proc Natl Acad Sci USA 2004; 101: 1834–1839
- Alonso A, Sasin J, Bottini N, Friedberg I, Osterman A, Godzik A, Dixon J, Mustelin T. Cell 2004; 117: 699–711
- Wang WO, Sun JP, Zhang ZY. Curr Top Med Chem 2003; 3: 739–748
- Pils B, Schultz J. Mol Biol Evol 2004; 21: 625–631
- Ramponi G, Stefani M. Biochim Biophys Acta 1997; 1341: 137–156
- Raugei G, Ramponi G, Chiarugi P. Cell Mol Life Sci 2002; 59: 941–949
- Angers-Loustau A, Cote JF, Tremblay ML. Biochem Cell Biol 1999; 77: 493–505
- Fiaschi T, Chiarugi P, Buricchi F, Giannoni E, Taddei ML, Talini D, Cozzi G, Zecchi-Orlandini S, Raugei G, Ramponi G. J Biol Chem 2001; 276: 49156–49163
- Lucentini L, Fulle S, Ricciolini C, Lancioni H, Panara F. Int J Biochem Cell Biol 2003; 35: 1378–1387
- Chiarugi P, Taddei ML, Schiavoni N, Papucci L, Giannoni E, Fiaschi T, Capaccioli S, Raugei G, Ramponi G. Oncogene 2004; 23: 3905–3914
- Granjeiro JM, Taga EM, Aoyama H. An Acad Bras Ci 1997; 69: 451–460
- Granjeiro JM, Ferreira CV, Jucá MB, Taga EM, Aoyama H. Biochem Mol Biol Int 1997; 41: 1201–1208
- Buzalaf MAR, Taga EM, Granjeiro JM, Ferreira CV, Lourenção VA, Ortega MM, Poletto DH, Aoyama H. Exper Lung Res 1998; 24: 269–272
- Granjeiro JM, Ferreira CV, Granjeiro PA, Silva CC, Taga EM, Volpe PLO, Aoyama H. J Enz Inhib Med Chem 2002; 5: 345–350
- Granjeiro JM, Miranda MA, Maia MGST, Ferreira CV, Taga EM, Aoyama H, Volpe PLO. Mol Cell Biochem 2004; 265: 133–140
- Baxter JH, Suelter CH. Arch Biochem Biophys 1985; 239: 29–37
- Dissing J, Rangaard B, Christensen U. Biochim Biophys Acta 1993; 1162: 275–282
- Zabell AP, Corden S, Helquist P, Stauffacher CV, Wiest O. Bioorg Med Chem 2004; 12: 1867–1880
- Middleton E, Kandaswami C, Theoharides TC. Pharmacol Rev 2000; 52: 673–751
- Harborne JB, Williams CA. Phytochemistry 2000; 55: 481–504
- Havsteen BH. Pharmacol Ther 2002; 96: 67–202
- Ren WY, Qiao ZH, Wang HW, Zhang I. Med Res Rev 2003; 23: 519–534
- Williams RJ, Spencer JPE, Rice-Evans C. Free Rad Biol Med 2004; 36: 838–848
- Bormann H, Melzing MF. Pharmazie 2000; 55: 129–132
- Mantle D, Falkous G, Perry EK. Clin Chim Acta 1999; 285: 13–20
- Murakami S, Muramatsu M, Tomisawa K. J Enz Inhib 1999; 14: 151–166
- Shinozuka K, Kikuchi Y, Nishino C, Mori A, Tawata S. Experientia 1988; 44: 882–885
- Chu SC, Hsieh YS, Lin JY. J Nat Prod 1992; 55: 179–183
- Wang HK, Xia Y, Yang ZY, Natschke SL, Lee KH. Adv Exp Med Biol 1998; 439: 191–225
- Shimizu K, Kondo R, Sakai K. Planta Medica 2000; 66: 11–15
- Kang HM, Kim JH, Lee MY, Son KH, Yang DC, Baek NI, Kwon BM. Nat Prod Res 2004; 18: 349–356
- van Zanden JJ, Hamman OB, van Iersel MLPS, Boeren S, Cnubben NHP, Lo Bello M, Vervoort J, van Bladeren PJ, Rietjens IMCM. Chem Biol Interact 2003; 145: 139–148
- Ferriola CP, Cody V, Middleton E. Biochem Pharmacol 1989; 38: 1617–1624
- Cushman M, Nagarathmam D, Burg DL, Geahlen RL. J Med Chem 1991; 34: 798–806
- Nikolovska-Coleska Z, Suturkova L, Dorevski K, Krbavcic A, Solmajer T. Quant Struct-Act Relat 1998; 17: 7–13
- Gamet-Payrastre L, Manenti S, Gratacap MP, Tulliez J, Chap H, Payrastre B. Gen Pharmacol 1999; 32: 279–286
- Lee MS, Oh WK, Kim BY, Ahn SC, Kang DO, Sohn CB, Osada H, Ahn JS. Planta Medica 2002; 68: 1063–1065
- Prazeres JN, Ferreira C, Aoyama H. Plant Physiol Biochem 2004; 42: 15–20
- Lowry OH, Lopez JA. J Biol Chem 1945; 162: 421–424
- Lineweaver H, Burk D. J Am Chem Soc 1934; 56: 658–666
- Dixon M, Webb EC. Enzymes3rd Edn. Longmans, London 1979; 332–399
- Hammad HM, Abdalla SS. Gen Pharmacol 1997; 28: 767–771
- Wang S, Stauffacher CV, van Etten RL. Biochemistry 2000; 39: 1234–1242