ABSTRACT
Background
The impact of pneumococcal conjugate vaccines (PCVs) on the burden of invasive pneumococcal disease (IPD) and serotype distribution was examined across age groups from data collected by the Lebanese Inter-Hospital Pneumococcal Surveillance Program.
Methods
Between 2005 and 2020, 593 invasive Streptococcus pneumoniae isolates were collected from 79 hospitals throughout Lebanon. Serotypes and antimicrobial resistance (AMR) profiles were identified, and trends compared over 3 eras: PCV7, post-PCV7/ pre-PCV13, and PCV13 eras.
Results
The prevalence of PCV7 serotypes decreased significantly from 43.6% in the PCV7 era to 17.8% during the PCV13 era (p<0.001). PCV13-only serotypes remained stable in the PCV13 compared to the post-PCV7 eras, especially serotypes 1 and 3, whereas non-vaccine types (NVT) increased throughout the study period, especially 24 and 16F. The mortality rate increased substantially from 12.5% (PCV7 era) to 24.8% (PCV13 era). A significant decrease in AMR was observed across the three study eras.
Conclusion
PCVs substantially impacted IPD and AMR in vaccinated and unvaccinated populations despite an increase in mortality driven by NVT. Broadening the recommendation of vaccination to include older age-groups, using higher valency vaccines, and implementing stringent antimicrobial stewardship are likely to further impact the burden of IPD.
1. Introduction
Invasive pneumococcal disease (IPD) constitutes a major global threat across age groups particularly among children and the elderly [Citation1,Citation2]. S. pneumoniae is responsible for significant global morbidity and mortality in childhood causing more than 45 million incidents of pneumonia and more than 300,000 deaths annually in those under 5 years of age [Citation3,Citation4].
The polysaccharide capsule of S. pneumoniae determines the serotype and is a major virulence factor where it serves multiple functions during colonization, adherence, and evasion of the host immune system [Citation5,Citation6]. The capsular antigens of the common pathogenic serotypes were used to develop the original 23-valent pneumococcal polysaccharide vaccine (PPV23), which is used in the elderly and high-risk populations but is not sufficiently immunogenic in children younger than 5 years of age [Citation7,Citation8]. The substantially more immunogenic pneumococcal conjugate vaccines (PCVs) that target the most common disease-causing serotypes were later developed and successfully implemented globally [Citation9–17]. Recently, the FDA approved the 15-valent and 20-valent PCVs for adults 18 years and older that target an expanded number of IPD-causing serotypes [Citation18–23]. The large-scale use of PCVs has affected both the disease and carriage prevalence of vaccine serotypes over time and across regions [Citation24,Citation25]. However, this has been partially hampered by the global emergence of non-vaccine types (NVT) [Citation26] associated with high rates of antimicrobial resistance (AMR) [Citation27,Citation28].
In Lebanon, PCV7 was introduced in 2006 in the private sector, where about half of the children receive their vaccinations, followed by PCV10 and PCV13 in 2010. Uptake of these vaccines in the private sector was variable. PCV13 was added to the expanded program of immunization (EPI) using the 2 + 1 schedule (4, 6, and 12 months) in January 2016, making it available for all children who receive their vaccines in the public sector but without a recommendation for adult immunization, which remains at the discretion of the treating physician. Data from the Lebanese Inter-Hospital Pneumococcal Surveillance Program (LIPSP), which was established in 2005, was instrumental in making the decision to add PCV13 to the Lebanese EPI in 2016. In the previous publication from LIPSP, we reported that the majority of IPD serotypes collected between 2005 and 2011 were included in the available PCVs and that the AMR rates were high (17.4% for penicillin) with mortality highest in those older than 60 years or younger than 2 years of age [Citation29]. Since then, there is no published data from Lebanon about the impact of PCV introduction on the burden of IPD, the change in the IPD serotype landscape, the emergence of NVT similar to other countries, and AMR rates [Citation30]. The aim of the current study is to describe the impact of PCV introduction in Lebanon over a 15-year period extending from 2005 till 2020.
2. Materials and methods
2.1. Study design
LIPSP is an ongoing voluntary national surveillance program established in 2005 and headquartered at the Center for Infectious Diseases Research (CIDR) at the American University of Beirut (AUB) and supported by the Epidemiological Surveillance Unit of the Ministry of Public Health (ESU-MOPH) of Lebanon.
2.2. Target population and study site
The current study reports on 593 S. pneumoniae isolates (blood, cerebrospinal fluid, or other normally sterile sites) collected from 79 out of a total of 165 hospitals across Lebanon [Citation31] ( S) including patients of all age groups with IPD. The study was approved by the AUB Institutional Review Board (IRB) (PED.GD.01).
Figure 1. A. Overall distribution of invasive S. pneumoniae serotypes (N = 549) from 2005–2020. B. Changes in vaccine and non-vaccine serotypes in the three different age groups across the three vaccine eras of the study. PCV7 refers to isolates with serotypes 4, 6B, 9 V, 14, 18C, 19 F, and 23 F. PCV13 refers to the additional serotypes not included in PCV7: 1, 3, 5, 6A, 7 F, 19A. NVT refers to all other serotypes including those that were not typeable. Multinomial logistic regression was used to compare the percentage of VT and NVT serotypes across the 3 eras. The PCV7 era was the reference category. The bars refer to statistically significant differences in the indicated eras. C. Changes in vaccine and non-vaccine serotypes among subjects ≤5 years of age by year. PCV7 refers to isolates with serotypes 4, 6B, 9 V, 14, 18C, 19 F, and 23 F. PCV13 refers to the additional serotypes not included in PCV7: 1, 3, 5, 6A, 7 F, 19A. NVT refers to all other serotypes including those that were not typeable.

2.3. Case definition
As per the CDC, IPD was defined by the isolation of S. pneumoniae from a normally sterile body site e.g. blood, cerebrospinal fluid, or, less commonly, joint, pleural or pericardial fluid, or urine in a person of any age [Citation32].
De-identified epidemiological data was collected for each sample including age, gender, source of the sample, clinical syndrome, comorbidities and outcome. All isolates were stored at −80°C at CIDR until processing.
2.4. Identification and antibiotic susceptibility testing
Bacterial isolates were cultured on Mueller-Hinton blood sheep agar (MHSB) plates and incubated overnight in 5% CO2 at 37°C. S. pneumoniae isolates were identified by colony morphology and optochin susceptibility. Antibiotic susceptibility testing was determined using disk diffusion to oxacillin, erythromycin, clindamycin, trimethoprim-sulfamethoxazole (TMP-SMX), chloramphenicol, tetracycline, vancomycin, levofloxacin, as well as E-test (Biomerieux, France) for both penicillin (PEN) and ceftriaxone (CRO) following the Clinical and Laboratory Standards Institute (CLSI) guidelines [Citation33]. For PEN, non-meningitis breakpoints were considered susceptible (MIC ≤ 2 µg/ml), intermediate (MIC = 4 µg/ml) or resistant (MIC ≥ 8 µg/ml). In meningitis cases, MIC ≤ 0.06 µg/ml were categorized as susceptible and ≥ 0.12 µg/ml as resistant. For CRO, non-meningitis breakpoint was considered susceptible (MIC ≤ 1 µg/ml), intermediate (MIC = 2 µg/ml) or resistant (MIC ≥ 4 µg/ml). For meningitis, MIC ≤ 0.5 µg/ml were categorized as susceptible, whereas MIC ≥ 2 µg/ml was considered resistant. Non-susceptible isolates include all isolates with an intermediate and/or resistant phenotype.
2.5. Serotyping
Bacterial DNA was extracted from bacterial cells freshly grown overnight on MHSB agar using the InstaGene matrix (BIO-RAD) according to the manufacturer’s protocol. Briefly, we picked up one isolated bacterial colony, resuspended it in 200 µl of the InstaGene Matrix and vortexed, followed by heating at 56°C for one hour, then at 100°C for 8 minutes. The samples were then centrifuged at 10,000 g, and the supernatant with the extracted DNA was transferred to a new tube and stored at −20°C for later analysis.
Serotyping was performed using a sequential multiplex PCR method as previously described (available at https://www.cdc.gov/streplab/downloads/pcr-us-clinical-specimens.pdf) [Citation29]. Seven reactions composed of different primers sets were performed to determine serotypes/serogroups of pneumococcal isolates as earlier defined. In addition to each reaction-specific set of primers, an internal positive control for a conserved region in the pneumococcal cps operon (cpsA) and a negative control were added to each reaction. Due to high genotypic similarities between the capsule loci of certain serotypes and the unavailability of specific primers, we were unable to discriminate some serotypes within a particular serogroup; these included 9 V/9A, 11A/11D, 15A/15 F, 15B/15C, 18A/18B/18C/18 F; 9 N/9 L; 7 F/7A; 7C/7B/40 F, 10 F/10C/33, 24A/B/F, 35A/35C/42, 35 F/47 and 38/25. To differentiate serogroup 6 isolates, we used a serotype-specific PCR assay to identify serotypes 6A, 6B, 6C, and 6D based on a combination of three PCRs, using specific primers as previously described [Citation34,Citation35]. Some of the isolates were serotyped initially by Quellung reaction such as 6, 9 N/9 L, 9 V/9A and 18 as previously published [Citation29]. Later, as identification of serogroups by Quellung reaction became unavailable to us, we used multiplex PCR exclusively and these latter serotypes were identified as 6A/B/C/D, 9 N/9 L, 9 V/9A or 18. If the serotype was not detected by the seven reactions, the strain was classified as non-typeable (NT). Moreover, we divided the serotypes into vaccine types (VT) and non-vaccine types (NVT). VT represent those targeted by conjugate vaccines available in Lebanon (PCV7, PCV10, PCV13) whereas NVT represent all the remaining serotypes.
2.6. Statistical analysis
Continuous variables were presented as means ± Standard Deviation (SD) and categorical variables as numbers and percentages. Univariate binomial and multinomial regression analyses were performed to study the burden of IPD and impact of vaccination, the changes of serotype distribution, the serotype coverage by PCV, the changes in the trends of antibiotic resistance of S. pneumoniae, and the comparison of the antibiotic resistance rates among different age groups, during the three study eras (PCV7, post-PCV7/pre-PCV13, and PCV13 eras). The strength of association was interpreted using the Odds ratio (OR) with 95% confidence Interval (CI). A p-value ≤ 0.05 was considered statistically significant. All these analyses were carried out using the SPSS software, version 25 (SPSSTM Inc., Chicago, IL USA).
2.7. Ethical considerations
The study was approved by the AUB IRB (PED.GD.01) and the directors of participating hospitals, in addition to a legal decree that was signed with the Lebanese Ministry of Health. Ethical clearance was obtained through a formal letter granted in line with the World Medical Association Declaration of Helsinki in 2013 [Citation36]. The IRB granted a waiver of the requirement to obtain informed consent from subjects since our research involved materials already collected. There were no risks to subjects as the isolates have already been collected as part of medical care. No identifiers were collected as part of the case report form, specimens were assigned a code that reflected the origin (participating hospital) and the sequence of collection, e.g. AUBMC001, AUBMC002, etc. A logbook was kept in each hospital to keep record of the samples that were being shared with us and that liaised the serial numbers that we had to the patients’ records in the hospitals. This logbook was important to allow us to communicate the results back to the physician in charge of the patient. As for our records, all the data that were collected with each specimen were kept on an SPSS database that was accessed only by the research team allowed by the IRB, updated regularly and saved on password-protected computers at the CIDR office. To maintain confidentiality, data will be destroyed once the legal retention period expires.
3. Results
3.1. Demographics and clinical spectrum
A total of 593 isolates of S. pneumoniae were identified in patients diagnosed with IPD (). The majority of samples were collected from Beirut (63.6%), followed by the North Lebanon and Mount Lebanon regions (27.1% and 4.6%, respectively). Overall, 57.1% were collected from males. The mean age at diagnosis (±SD) was 34.3 ± 32.5 years. Age groups were distributed as follows: 21.8% (n = 128) < 2 years, 16.5% (n = 97) between 2 and < 6 years, 11.1% (n = 65) between 6 and < 21 years, 18.2% (n = 107) between 21 and < 60 years, and 32.5% (n = 191) ≥ 60 years. Age was unknown for 5 samples. The isolates were collected from the following sources: blood (84.7%, n = 502), cerebrospinal fluid (CSF) (12.5%, n = 74), pleural fluid (3.5%, n = 21), and other normally sterile sites (1.5%, n = 9). The latter sources included synovial fluid (n = 1), pericardial fluid (n = 1), mastoid abscess (n = 2), parasternal abscess (n = 1), abscess (n = 1), urine (n = 2), and peritoneal fluid (n = 1). A total of 593 isolates were collected from 606 different sources with 13 isolates identified simultaneously from 2 different sources in the same patient (11 cases from blood and CSF and 2 cases from blood and pleural fluid).
Table 1. Demographic features and clinical burden of patients with IPD from the LIPSP.
About half of the IPD patients were diagnosed with pneumonia (47.5%, n = 280). The remaining were diagnosed with bacteremia (32.4%, n = 191), meningitis (18.2%, n = 107), or other diagnoses (1.9%, n = 11).
3.2. Changes of serotype distribution during three study eras
Serotyping was attempted for 549 isolates of S. pneumoniae. We were unable to process 44 isolates due to technical difficulties resulting from lack of optimal storage or regrowth failure and these were excluded from the analyses. As shown in , the distribution of isolates during the three study eras was as follows: 172 isolates were collected between 2005–2009 (PCV7 era), 202 isolates between 2010–2015 (post-PCV7/pre-PCV13 era), and 175 isolates between 2016–2020 (PCV13 era). A specific serotype/serogroup was assigned for 517 isolates (94.2%) with the available primers whereas 32 were considered NT.
Table 2. Distribution of PCV7, PCV10, PCV13, PPV23, and other serotypes among invasive S. pneumoniae isolates recovered from all age groups during the PCV7, post-PCV7/pre-PCV13, and PCV13 eras.
When all age groups were studied together (), the predominant serotypes were all VT (19 F, 3, 1, 14, 19A). In total, 63.4% of the identified serotypes were VT (). Current NVT that will be included in PCV15 and/or PCV20 such as 33 F/33A/37, 22 F/22A, 15B/15C, 11A/11D, 12 F/12A, 10A, and 8 were detected at low rates in our surveillance but accounted for an additional 4.6% (PCV15) or 8.2% (PCV20) (). The total current NVT accounted for 36.6%, representing all serotypes not covered by the available conjugate vaccines. A majority of NVT were included in the PPV23 with the notable exceptions of serogroup 24 (2.2%), where we separately reported eight of the 12 isolates identified by whole genome sequencing as serotype 24 F [Citation28], serotype 16 F (1.6%), and the likely heterogeneous NT isolates (5.8%).
The leading serotypes detected in children ≤ 5 years were 14, 19 F, 1, and 19A, whereas among adults, they were 3, 19 F, 22 F/22A and 9 V/9A (in descending order). Serotype 14 was significantly more common in children (P-value = 0.044), whereas serotypes 3, 22 F/22A, and 9 V/9A were significantly more common in adults (P-value = 0.014, 0.007, 0.049, respectively) (Figure 2S. and Table S4).
Figure 2. Changes in antimicrobial resistance of S. pneumoniae over the three study eras. The percentage of penicillin, erythromycin and clindamycin non susceptible S. pneumoniae isolates are indicated in the PCV7, post-PCV7/Pre-PCV13 and PCV13 eras. Binomial logistic regression was used to compare the antimicrobial resistance within the 3 eras. The PCV7 era was the reference category. *p = 0.002, OR = 0.269, 95%CI = [0.119–0.612].
![Figure 2. Changes in antimicrobial resistance of S. pneumoniae over the three study eras. The percentage of penicillin, erythromycin and clindamycin non susceptible S. pneumoniae isolates are indicated in the PCV7, post-PCV7/Pre-PCV13 and PCV13 eras. Binomial logistic regression was used to compare the antimicrobial resistance within the 3 eras. The PCV7 era was the reference category. *p = 0.002, OR = 0.269, 95%CI = [0.119–0.612].](/cms/asset/4a513fd3-4846-4441-acb7-4f6e216f8e05/ierv_a_2143349_f0002_b.gif)
The clinical syndromes were distributed similarly between VT and NVT. Regarding the association of serotypes with specific clinical syndromes (N = 535), some serotypes were more likely to be associated with pneumonia such as serotypes 3 (66% of all serotype 3 isolates were from patients with pneumonia), 1 (57.1%), 19A (57.1%), 24 (54.5%), and 22 F/22A (52.9%). Other serotypes were more likely to be associated with bacteremia such as serotypes 14 (39.5% of all serotype-14 isolates were from patients with bacteremia) and 19 F (39.1%). Finally, the serotypes more likely to be associated with meningitis were: 23 F (38.1%) and 19 F (30.4%).
Out of the 77 patients who died, the most frequent serotypes associated with mortality were 19 F and 3 with each serotype representing 10.4% of the total mortalities, followed by serotype 1, 16 F, 19A and 6A (5.2%, each).
3.3. Serotype coverage by pneumococcal vaccines
The percentage of the serotypes covered by available vaccines, PCV7, PCV10 and PCV13, among all age groups were 33.2%, 45.6% and 63.4%, respectively. In children ≤ 5 years of age, they were distributed as follows: 41.1% for PCV7, 54.1% for PCV10, 68.6% for PCV13, 71% for PCV15, 77.3% for PCV20 and 77.8% for PPV23 vaccine.
In adults more than 60 years of age, the serotypes covered by available vaccines were distributed as follows: 30.1% for PCV7, 40.5% for PCV10, 62.4% for PCV13, 71.1% for PCV15, 79.2% for PCV20 and 81.9% for PPV23.
More important than the cumulative coverage of the serotypes by available vaccines, was the change in VT over the 15-year study period reflecting the impact of the introduction of PCV7 then PCV10 and PCV13. and display the changes of VT and NVT over 3 periods of time: 2005–2009 (PCV7 era), 2010–2015 (post-PCV7 era), and 2016–2020 (PCV13 era) across all age groups. There was a significant increase of PCV13/non-PCV7 VT in the Post-PCV7 era compared to the PCV7 era (OR = 1.581; 95%CI = [1.079–2.317]; p-value = 0.019). A further increase of 6.2%, although not statistically significant, was observed in the PCV13 era, mainly driven by the increase of serotypes 3 and 1 despite the adoption of the PCV13 in the national immunization schedule in 2016. In contrast, the proportion of PCV7 serotypes across age groups decreased significantly from 43.6% (75 isolates) during the PCV7 era to 17.8% (31 isolates) during the PCV13 era (p-value <0.001; OR = 0.547, 95% CI = [0.432–0.691]). Serotype 6B significantly dropped from the PCV7 era to the PCV13 era (p-value = 0.011, OR = 0.071, 95%CI = [0.009–0.543]). Similarly, serotypes 14 and 19 F decreased significantly from the PCV7 era to the PCV13 era (p-value = 0.040, OR = 0.375, 95%CI = [0.147–0.958] and p-value = 0.002, OR = 0.217, 95%CI = [0.083–0.572], respectively). The net result was an overall decline of 19.5% in PCV13 VT driven by the decrease in PCV7 serotypes. Moreover, the proportion of PPV23/non-PCV13 VT remained static whereas the NVT not included in PPV23 increased by 19.2% from PCV7 era to PCV13 era (from 22 isolates (12.8%) to 56 isolates (32%), respectively) led by serogroup 24. Among the NVT, NT isolates represented a total of 29.4% (32 isolates) throughout the study period with an increase of 5.1% from PCV7 era to PCV13 era (from 9 isolates (5.2%) to 18 isolates (10.3%), respectively).
The leading serotypes in the PCV7 era were 19 F, 14, 6B, and 3. In addition to these serotypes, serotype 1 also predominated in the post-PCV7/pre-PCV13 era, while serotypes 3 and 1 predominated in the PCV13 era. However, NVT expanded and became the leading serotypes in this era. A statistically significant increase in the number of NVT-IPD across the three study eras was observed (p-value < 0.001) (specifically from PCV7 era to PCV13 era, p-value = 0.004) ( and ). The largest increase in the number of NVT was observed in the age group 6–60 years in the PCV13 era (from 18 isolates (37.5%) in the PCV7 era to 31 isolates (59.6%) in the PCV13 era) (p-value = 0.028) ().
Since vaccination with PCV13 in Lebanon is limited to children, we examined the impact of vaccination in the age groups separately (). There was a significant decrease in PCV7 serotypes in the age groups < 5 years and 6–60 years when the PCV13 era was compared to the PCV7 era (p = 0.001 and p = 0.02, respectively) but in the >60 years’ age group, the decrease was not statistically significant. When analyzed by age-group, the PCV13-only serotypes did not change significantly over the examined eras ().
3.4. Burden of IPD and the impact of vaccination
Whereas there are multiple variables that can be used for measuring the burden of IPD, we focused on the duration of hospital stay, as a measure of morbidity, and mortality as these variables could be captured by our surveillance program. As shown in , the mean length of hospital stay was 8.3 ± 8.6 days among all patients (n = 370). Among the diagnostic groups, the highest mean hospital stay was 11.9 ± 10.1 for patients with meningitis ( S-A). Among patients with IPD, the most prevalent comorbidities were cardiovascular disease (24%), malignancy (18.1%) and respiratory disease (12.7%) (). These comorbidities were significantly less common in children <6 years of age compared to the elderly >60 years of age (Table S1-B). Of the 69 patients with known data who died, 75.4% had at least one comorbidity and this was significantly associated with fatal IPD (p-value = 0.001; OR = 2.6, 95% CI = [1.436–4.706]). Indeed, a significantly higher risk of death was found among subjects with one comorbidity (p-value = 0.024, OR = 2.182, 95%CI = [1.109–4.292]) and those with ≥ 2 comorbidities (p-value = 0.001, OR = 3.111, 95%CI = [1.597–6.061]) (Table S1-C). Following the introduction of PCV, IPD was more likely to be fatal in those with at least one comorbidity in the post-PCV7/pre-PCV13 era (one comorbidity: p-value = 0.043, OR = 3.559, 95%CI = [1.041–12.161]; ≥ 2 comorbidities: p-value = 0.021, OR = 4.321, 95%CI = [1.252–14.912]) and in those with ≥ 2 comorbidities in the PCV13 era (p-value = 0.044, OR = 3.238, 95%CI = [1.035–10.135]) (Table S1D). Additionally, in supplementary tables S1-E, 1S-F, and 1S-G, we further analyzed the relationship of mortality rate to comorbidities across eras and age groups but found no significant associations. However, we found that cardiovascular disease (36.2%), malignancy (30.4%), and neurological disease (14.5%) were significantly associated with mortality among patients with IPD (p-value = 0.009; OR = 2.101, 95% CI = [1.195–3.695]; p-value = 0.004; OR = 2.378, 95% CI = [1.303–4.339]; and p-value = 0.012; OR = 2.782, 95% CI = [1.213–6.380], respectively).
As shown in , out of the 444 patients with known outcome data, 77 patients died (overall mortality of 17.3%). The mortality rate, calculated as the (Number of IPD mortalities in a specific age group and PCV era/Total Number of IPD in the corresponding age group and PCV era) X 100, increased substantially from 12.5% in the PCV7 era (2005–2009), to 19.2% in the post-PCV7 era (2010–2015), reaching 24.8% in the PCV13 era (2016–2020) (). There was a statistically significant increase of mortality in the PCV13 era compared to the PCV7 era (OR = 2.310, 95%CI = [1.234–4.326], p = 0.009). This surprising finding led us to study whether the increase in mortality was attributed to a specific age-group (). We found that, even in the vaccinated age-group (≤ 5 years), mortality had increased from 6.6% to 17.8%. On the other hand, the risk of mortality increased in the second two vaccine eras in association with increased comorbidities (Table S1-C, 1S-D, 1S-E, 1S-F). Mortality rates differed among age groups, with the highest rate (42%) in > 60 years’ age group during the PCV13 era. Elderly (> 60 years), who have significantly higher rates of comorbidities, were almost 4 times more likely to die from IPD as compared to children ≤ 5 years (p < 0.001, OR = 3.804, 95% CI = [2.067–7.002]) ().
Table 3. Changes in the mortality rates in the three different age groups across the three vaccine eras of the study.
Table 4. Antimicrobial resistance profiles of major S. pneumoniae serotypes, 2005–2020.
IPD diagnoses were associated with different mortality rates, the highest being among those who had pneumonia or bacteremia (47.4% and 33.3%, respectively) (Table S1-A). The younger age groups were 5 times more likely to develop meningitis as compared to > 60 years age group (p < 0.001, OR = 5.778 [2.847–11.724]; p < 0.001, OR = 5.111 [2.502–10.442], for ≤ 5 years and 6–60 years age groups, respectively). Meningitis was associated with a 4-fold increase of risk of death compared to bacteremia among subjects ≤5 years (p-value = 0.026, OR = 4.296, 95%CI = [1.190–15.517]) (Table S2-A, 2S-B).
When looking at the overall mortality due to meningitis across the three eras, the mortality rate increased from 10.5% to 16.1% to 34.8%, respectively, but this finding was not statistically significant.
3.5. Serotype-specific mortality
We analyzed which serotypes were identified as major contributors to mortality across the three study eras. In the PCV7 era, VT accounted for 60% of all mortalities (12 out of 20) with 19 F being the predominant serotype (3 out of 20) (15%) distributed equally across all age groups. In the post-PCV7/pre-PCV13 era, VT accounted for 64.3% of mortalities (18 out of 28), where serotypes 19 F (4 out of 28) (13.8%) and 1 (3 out of 28) (10.3%) were predominant across all age groups. In the PCV13 era, the VT mortality burden declined to 44.8% (13 out of 29), with serotype 3 being predominant (4 out of 29) (13.8%), whereas NVT mortality was about 55.2% (16 out of 29). There was no predominant NVT associated with higher mortality. The 34 fatal NVT serotypes are listed in Table S2-C.
When comparing VT and NVT related mortality in association with comorbidities and diagnoses, there was no significant difference in the case fatality rate across eras except in the PCV13 era where we found an increase of case fatality rate due to NVT in subjects with one comorbidity (p-value = 0.031, OR = 7.917, 95%CI = [1.209–51.841]) (Table S2D, 2S-E, and 2S-F).
3.6. Changing trends of S. pneumoniae antimicrobial resistance
We examined the antimicrobial resistance trends of S. pneumoniae for the commonly used antibiotics (penicillin, erythromycin, clindamycin, and others) over the three eras (). The proportion of penicillin-resistant S. pneumoniae isolates was 18.6% in the PCV7 era, decreased to 15.4% in the post-PCV7/pre-PCV13 era, with a further significant decrease reaching 5.8% in the PCV13 era (OR = 0.269, 95%CI = [0.119–0.612], p-value = 0.002, for the PCV13 era). The erythromycin resistance rates increased initially from 28% to 37.5% over the first two eras then declined to 27% in the PCV13 era. The clindamycin resistance decreased from 38.5% to 27.7% in the post-PCV7 era, followed by a further decrement in the PCV13 era (). Non-susceptibility to TMP/SMX was high in the PCV7 (69%) era but decreased significantly in the post-PCV7 era (53%) (OR = 0.520, 95% CI = [0.334–0.810], p-value = 0.004) plateauing in the PCV13 era (OR = 0.503, 95% CI = [0.320–0.792], p-value = 0.002) (Figure 3 S).The rate of ceftriaxone non-susceptibility, dominated by 3 PCV7 VT (14, 19 F, and 9 V/9A), decreased from 15.8% in the PCV7 era to 8% in the post-PCV7/pre-PCV13 era (OR = 0.464, 95% CI = [0.219–0.983], p-value = 0.045) and none in the PCV13 era (OR = 0.040, 95% CI = [0.005–0.297], p-value = 0.002). On the contrary, non-susceptibility to tetracycline remained considerably stable in the PCV7 era through the post-PCV7/pre-PCV13 era (35.9% to 34.7%) with a significant decrease by around 15% in the PCV13 era (18.8%) (OR = 0.412, 95%CI = [0.248–0.684], p-value = 0.001) (Figure 3S). Serotype 24 F emerged as an important contributor to tetracycline resistance in the PCV13 era (Figure 4S). Similarly, Chloramphenicol resistance rates decreased significantly from 12.9% in the PCV7 era to 4.5% in the PCV13 era (OR = 0.314, 95%CI = [0.128–0.772], p-value = 0.012). On the other hand, Low rates of levofloxacin resistance were observed through the 3 study eras, (5.4% in the PCV7 era, 2.7% in the post-PCV7/pre-PCV13 era and 3.9% in the PCV13 era). No predominant resistant serotype emerged with sporadic resistance detected among serotypes 18C, 16 F, 19 F, 12 F/12A, 1, 15B/15C, 3, 23A, 14, 9 V/9A, 8, 5, 24 F and 6A.
Figure 3. Comparison of antibiotic resistance rates among different age groups over the three study eras. The percentage of Penicillin (A), Erythromycin (B) and Clindamycin (C)-non susceptible S. pneumoniae isolates are indicated in the PCV7, post-PCV7/Pre-PCV13 and PCV13 eras across three age groups. Clindamycin sensitivity was not performed for isolates from the 6-60 years age group in the PCV7 era due to technical reasons.

Figure 4. Impact of vaccination on antimicrobial resistance by serotypes across age groups. The contribution of vaccine and non-vaccine S. pneumoniae serotypes to penicillin (A), erythromycin (B), and clindamycin (C) resistance is indicated in the PCV7, post-PCV7/Pre-PCV13 and PCV13 eras across three age groups. Clindamycin sensitivity was not performed for isolates from the 6-60 years age group in the PCV7 era due to technical reasons. PCV7 refers to isolates with serotypes 4, 6B, 9V, 14, 18C, 19F, and 23F. PCV13 refers to the additional serotypes not included in PCV7: 1, 3, 5, 6A, 7F, 19A. NVT refers to all other serotypes including those that were not typeable.
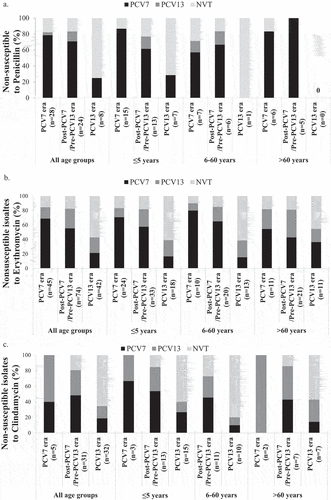
Importantly, no resistance to levofloxacin was observed in the ≤5 years age group in the PCV7 era. However, few levofloxacin-resistant isolates (19 F, 12 F/12A, and 16 F) were encountered in this age group in the post-PCV7 and PCV13 eras (Figures 3S and 4S). No resistance to vancomycin was encountered.
When comparing the AMR of PCV13 serotypes across the 3 study eras (Figure 5S.), Clindamycin and Penicillin showed a significant decline in resistance from 50% and 21.7% in the PCV7 era to 15% and 3.1% in the PCV13 era, respectively (p-value = 0.014, OR = 0.176, 95%CI = [0.044–0.704], p-value = 0.004, OR = 0.115, 95% CI = [0.026–0.504], respectively). Similarly, resistance to Tetracycline also decreased significantly from 38.2% in the PCV7 era to 16.5% in the PCV13 era (p-value = 0.002, OR = 0.319, 95%CI = [0.157–0.648]). Moreover, a significant decrease of resistance to TMP/SMX was observed in the post-PCV7/pre-PCV13 era (59.7%) and PCV13 era (51.9%) compared to the PCV7 era (73%) (p-value = 0.033, OR = 0.548, 95% CI = [0.316–0.952]; p-value = 0.003, OR = 0.400, 95% CI = [0.217–0.734]). As for the NVTs (Fig. 6S.), they had a significant increasing resistance to Erythromycin from 13.7% in the PCV7 era to 30.4% in the PCV13 era (p-value = 0.034, OR = 2.743, 95%CI = [1.082–6.956]). On the other hand, a significant decrease in the resistance to Chloramphenicol was observed across the 3 study eras (post-PCV7/pre-PCV13 era: p-value = 0.050, OR = 0.286, 95%CI = [0.082–0.998], PCV13 era: p-value = 0.002, OR = 0.120, 95%CI = [0.031–0.467]).
3.7. Comparison of the antibiotic resistance rates among different age groups over the three eras
We explored the changes in antimicrobial resistance trends over the three eras across the different age groups. We first analyzed the change in penicillin resistance and found that it decreased significantly across all three age groups over the study eras (p-value = 0.002, OR = 0.567, 95%CI = [0.396–0.813]) (). When the PCV13 and the PCV7 eras were compared, there was a pronounced decrease in penicillin resistance among the 6–60 and the > 60 years age groups. (). Whereas 14% of isolates from the age group ≤ 5 were penicillin resistant, only 2.4% were resistant in the 6–60 years and none in the >60 years age groups in the PCV13 era (p-value = 0.038, OR = 0.376, 95% CI = [0.149–0.949]) (). The proportion of isolates resistant to penicillin remained the highest in the ≤ 5 years age group across the three eras with 25.4%, 23.2% and 14% respectively. In contrast, the resistance rates to erythromycin in the ≤ 5 years age group were somewhat stable over the three study eras unlike the other age groups where a fluctuating trend was evident in the 6–60 and > 60 years age groups (). Resistance rates to clindamycin decreased significantly in the oldest age group across the 3 eras (p-value = 0.047, OR = 0.390, 95%CI = [0.154–0.988]) but in the youngest age group they remained steady (27–30%) across all eras. ().
3.8. Impact of vaccination on antimicrobial resistance
In our earlier report, we found that the majority of penicillin-resistant isolates were VT [Citation29]. Therefore, it was important to study the impact of PCV vaccination on the serotype distribution of resistant isolates. Because the introduction of PCV7, then PCV10 and PCV13, led to a significant decrease in IPD caused by PCV7 VT (), we reasoned that PCV7 VT would constitute a minority of the resistant isolates in the PCV13 era. Indeed, we found that penicillin-resistant PCV7 VT declined significantly across age groups in the PCV13 era compared to the PCV7 era although they remained a substantial minority, almost a third, in the ≤ 5 age group (compare ). Additionally, the PCV13-only VT accounted for a minor proportion of penicillin-resistant isolates across the PCV7 and Post-PCV7/Pre-PCV13 eras. (). Of the VT, 14 was the only penicillin-resistant serotype observed in the PCV13 era in the ≤ 5 years age group accounting for 2 out of the 7 (28.6%) penicillin-resistant isolates in this age group. NVT serotypes emerging in the PCV13 era included 11A/11D, 9 N/9 L, 24 F, 16 F, and 35B.
Similar observations were made regarding erythromycin resistance where the proportion of erythromycin-resistant PCV7 serotypes decreased consistently across the three eras among all age groups (). This was more evident in the PCV13 era for the ≤ 5 years and 6–60 years age groups. The proportion of erythromycin-resistant PCV13-only VT increased over the first and second study eras, with a slight decrease in the last study era mostly in the >60 years age group. (). The latter observations were not statistically significant. On the other hand, the erythromycin-resistant NVT increased significantly in the PCV13 era among all age groups (p-value = 0.034, OR = 2.743, 95% CI = [1.082–6.956]).
Similarly, clindamycin-resistant PCV7 VT decreased over the study eras in the age group ≤ 5 years. However, unlike resistance to erythromycin, the proportion of PCV13-only VT resistant to clindamycin decreased in this age group. Most of the clindamycin-resistant isolates in the PCV13 era were NVT ().
We observed that several VT were commonly associated with AMR throughout the three study eras according to age groups (, Figure 4S). Serotypes 14, 19A and 19 F were prevalent throughout the study eras. Among PCV7 VT, 14, 19 F and 23 F showed the highest AMR rates to all antibiotics except to levofloxacin and chloramphenicol (). Importantly, serotype 14 was persistently identified among those ≤ 5 years of age. Among PCV13-only VT, 19A remained to be of particular concern exhibiting multidrug resistance such as for erythromycin among all age groups, as well as to clindamycin and tetracycline mainly in the ˃ 60 years age group. In addition to 19A, serotype 3 contributed mainly to clindamycin resistance across all age groups. Among the NVT, high AMR rates were observed among serotypes 11A/11D, 12 F/12A, 24 and 34 that were resistant against most antibiotics with high proportions of 12 F/12A and 24 F being resistant to both clindamycin and erythromycin. All NVT exhibited high resistance against TMP/SMX. (, Figure 4S).
4. Discussion
In this study, we aimed to describe the disease burden, serotype distribution and AMR patterns of invasive S. pneumoniae isolates, collected over a period of 15 years and the impact of PCV introduction in Lebanon.
The highest proportions of IPD were seen at the extremes of age, in children under 2 years of age (21.8%) and in ≥ 60 years (32.5%) with male predominance (57.1%) as previously reported [Citation37]. Blood and CSF were the most common sources of invasive pneumococcal isolates [Citation29,Citation38,Citation39]. The predominant pneumococcal serotypes were 19 F (8.9%), 3 (8.7%), 1 (7.7%), and 14 (7.1%). These four PCV13 VT accounted for 33% of the total isolates. Interestingly, among the leading serotypes in the PCV7 era, 6B almost disappeared in the PCV13 era. Furthermore, a statistically significant increase in NVT-IPD across the three study eras was observed mainly in the age group less than 5 years. Studies in the MENA region described similar results in terms of serotype/serogroup prevalence with 6, 14, 19 F, and 23 F reported most [Citation39–42]. For instance, 23 F, 6B, 19 F, 4, 14 and 19A were prevalent among pneumococcal strains from children under 15 years of age, collected between 2009–2012 in KSA covering 77% of total isolates [Citation40]. Similarly, 19 F, 19A, 6A, 8 and 15B were the most prevalent serotypes among Kuwaiti children under the age of 5; whereas 14, 3, 1, 19 F and 8 were prevalent among adults >50 [Citation39]. Interestingly, a similar serotype distribution among Omani adults ≥ 51 years was observed with 3 (13.3%), 15 (8.3%), 19A (8.3%), 19 F (6.7%), and 22 (6.7%) being the most prevalent. Furthermore, circulating serotypes varied among geographical areas and between pediatric and adult cases. When comparing the frequency of serotypes causing IPD among children ≤ 5 years of age and adults > 60 years, serotype 14 was significantly more prevalent in children less than 5 years while serotypes 3, 22 F/22A, and 9 V/9A were significantly more prevalent in adult IPD cases. Among pediatric IPD cases, serotypes 6B, 14 and 19 F were frequent in Africa-East Mediterranean countries [Citation43]. Among adult cases, serotypes 1, 4, 14 and 19A were commonly detected in these regions while serotype 3 was predominant in North America. The differences in serotype frequency have been explained by natural selection or vaccine-induced pressure [Citation44]. Some of these variations are likely due to differences in geographic regions and socio-economic factors. Other confounding factors could interfere including study differences in the age groups, local levels of antibiotic consumption and resistance, and local blood culture practices [Citation45].
The impact of vaccine introduction was strongest on PCV7 serotypes but was not substantial for PCV13-only serotypes (). Similar observations were seen following PCV13 introduction in KSA where PCV13-only serotypes remained unchanged and in Canada where the major breakthrough serotypes were 3 and 19A [Citation46,Citation47]. As in Canada, the 3-dose schedule of PCV13 given in the Lebanese public sector as opposed to the 4-dose schedule given in private clinics may have played a role in lower vaccine effectiveness against these serotypes. Serotypes 3, 19A, and 7 F are the least immunogenic among the PCV13 serotypes achieving the lowest geometric mean concentrations of specific antibodies, perhaps impacting protection with lower vaccine doses [Citation48].
Over the three study eras, overall mortality increased from 12.5% in the PCV7 era, to 19.2% in the post-PCV7 era, reaching 24.8% in the PCV13 era. The overall mortality was 17% with the elderly (> 60 years) four times more likely to die from IPD as compared to children ≤5 years. This unexpected increase occurred in all age groups including those ≤ 5 years of age who were presumably vaccinated. The apparent increase in the latter group in the PCV13 era may be multifactorial. Three of the mortalities were in infants younger than 5 months of age, 2 of whom with PCV7 serotypes, who may have been unvaccinated or had received a single dose of PCV13 according to the Lebanese EPI schedule (Table S3D). In addition, three of four mortalities from NVT in this age group were due to meningitis, higher than the other two eras, with most dying within 24–72 hours of hospital admission (Table S3-C, 3S-D). According to the Lebanese Ministry of Public Health, the rate of infants receiving their first PCV13 dose at 4 months of age ranged from 91.55% to 93.68% between 2017 and 2019 but dropped to 80.99% in 2020, and those receiving their third and final dose at 12–15 months ranged from 82% to 85.64% between 2017 and 2019 but dropped to 78.99% in 2020. It remains possible that more unvaccinated children were not reported to the ministry especially among the large Syrian refugee population. Therefore, some of the mortalities from VT could have been in unvaccinated children. No data on PPV23 and PCV13 uptake in the elderly is available from Lebanon. We had expected to see, at least in the younger age group, a drop in the mortality rate since VT accounted for most fatal infections in the PCV7 era. A possible explanation is that the increased mortality correlated with a relative increase in IPD caused by NVT in all age groups accounting for a higher proportion of observed cases. The overall mortality rate is higher than those reported in different MENA countries such as Oman (15.2%) [Citation42] and the Kingdom of Saudi Arabia (KSA) (12%) [Citation49]. As expected, the highest overall mortality rate was observed in adults aged ≥ 60 years (31.7%) [Citation50] and having at least one comorbidity was significantly associated with fatal IPD.
The rates of S. pneumoniae AMR vary globally and are highest in Asia [Citation51], followed by the Middle Eastern and African countries where they are, in turn, higher than European and North American countries [Citation52]. This could be driven by the overuse of antibiotics, that are widely available without prescription, specifically in outpatient settings and in rural regions. The penicillin resistance rate of S. pneumoniae showed a decreasing trend in Lebanon over the three study eras. Serotype 19 F, a major contributor to penicillin resistance in the PCV7 and Post-PCV7/Pre-PCV13 eras, was successfully suppressed in the PCV13 era. On the other hand, serotype 14 remained as a contributor to penicillin resistance in the ≤5 years age group. The incidence of penicillin non-susceptibility varies substantially among MENA countries [Citation53]. This is evident in previously reported rates from KSA (36% to 66%), Tunisia (51%-75.3%) [Citation41], UAE (43%) [Citation54], Oman (40.9%) [Citation42] and Turkey (61.9%) [Citation55]. The current study is the first from the region to show the impact of vaccination on decreasing the rate of penicillin resistance. In the current study, resistance rates to erythromycin showed an overall decreasing trend across the age groups over the three study eras. In some MENA countries, higher resistance rates have been observed. For instance, in Turkey, around 51% of S. pneumoniae isolates collected through 2011–2013 were erythromycin-resistant [Citation55]. In KSA, 62% of invasive S. pneumoniae isolated in children were erythromycin-resistant between 2005 and 2009 [Citation56]. In the current study we observed that clindamycin resistance rates decreased from 38% to 21% over the study eras. Other studies from the region reported 35.1% of resistance in Turkey [Citation55] and 23% in the UAE [Citation54]. In our study, levofloxacin susceptibility dropped to 96.1% compared to 98% reported in previous studies from Lebanon [Citation57,Citation58], KSA (100%) [Citation40], Oman (99.8%), and Turkey (98.2%) [Citation55]. Our findings are somewhat worrisome as levofloxacin features prominently in guidelines for the treatment of infections caused by penicillin-resistant S. pneumoniae or in patients allergic to penicillin [Citation59,Citation60]. No resistance to vancomycin was documented neither in Lebanon nor in MENA countries except for Iran, which reported resistance to vancomycin among healthy and sick individuals [Citation61].
Changing AMR patterns in S. pneumoniae isolates have broad implications for both the prevention and treatment of IPD. Pneumococcal vaccination has reduced antibiotic-resistant infections [Citation62]. Several of the PCV7 and PCV13 serotypes exhibited high levels of AMR in our study. Among these serotypes, 19 F, 23 F, 14 and 19A serotypes showed the highest resistance rates to all antibiotics except to levofloxacin. The impact of PCV13 is evident from our data as the overall rates of AMR decreased substantially after its introduction. Interestingly, no single serotype with high levels of AMR emerged among the NVT. Instead, the prevalent NVT included a wide range of serotypes with high levels of AMR but low proportion such as 11A/11D, 15B/15C, 16 F, 23B, 34, 35 F/47, 9 N/9 L, 12 F/12A and 24 [Citation28]. Thus, AMR will likely continue to be problematic in Lebanon and should be addressed by substantially enhancing national antimicrobial stewardship programs in addition to optimizing vaccine uptake across age groups.
4.1. Limitations
The current study has several limitations that are dictated by the nature of the surveillance program, which is based on laboratory isolation of S. pneumoniae and the incompletely available clinical information. Firstly, there is no clear distinction between eras as defined in this study when it comes to vaccine introduction. The introduction of PCVs in the private sector was gradual since 2000 with no data available about extent of coverage. Thus, the ‘PCV7 era’ merely indicates that PCV7 was available to some unknown extent in the private sector. Similarly, the ‘post-PCV7/pre-PCV13’ era indicates the period when PCV10 and PCV13 replaced PCV7 in the private sector without an accurate estimate about the extent of coverage. Finally, the ‘PCV13’ era indicates the period when PCV13, at least theoretically became available to all infants. The second important limitation was the impossible calculation of an approximate incidence rate due to the difficulty in clearly defining the population served by each participating hospital because of the nature of the Lebanese health care system with significantly overlapping catchment areas, the variable practice of obtaining cultures prior to commencing antibiotics across the country, the easy over the counter access to antibiotics, which are taken without prescription rendering cultures negative, and the lack of reporting all of the S. pneumoniae isolates by the participating hospitals. Thus, any calculation of the incidence rate would have been misleading and severely underestimated. The third limitation is that we were unable to determine the vaccination status of subjects from whom the isolates were collected, rendering us unable to determine vaccine effectiveness and, especially for vaccine eligible young children who died, whether mortalities were due to breakthrough infection or due to lack of vaccination to start with. Given that non-encapsulated pneumococci rarely cause invasive diseases; another limitation was that 32 isolates were non-typable. Our planned incorporation of genome sequencing for S. pneumoniae isolates will remedy this problem in the future. Moreover, a further limitation in the present study was the small sample size, which may result in displaying higher percentages of AMR while absolute numbers of isolates are low. Lastly, it is possible that high rates of AMR and mortality that we observed were a result of selection bias as patients are frequently treated with antibiotics prior to obtaining cultures, making it more likely to isolate resistant organisms from very sick patients. These limitations should be considered when interpreting our findings.
5. Conclusion
The introduction of PCVs in Lebanon led to a significant decline in IPD caused by PCV7 but not by PCV13-only serotypes that was demonstrable in vaccinated and unvaccinated age-groups. This was associated with a relative increase in IPD caused by NVT. Surprisingly, the mortality rates increased significantly over the study period and were driven mostly by NVT and, to a lesser extent, PCV13-only types, particularly in the older age-groups with high rates of comorbidities. These findings support a combined approach that includes implementing the use of higher valency conjugate vaccines, or preferably universal pneumococcal vaccines, especially in older age groups, along with strict adherence to antimicrobial stewardship efforts in the hospital and in the community. These efforts should be supported by ongoing surveillance programs that continue to measure the impact of vaccination and to determine the circulating serotypes causing IPD and their AMR profiles.
Declaration of interest
G.S. Dbaibo reports receiving research grants to his institution, serving on advisory boards, and receiving honoraria for lectures from Pfizer, MSD, Sanofi, and GSK. The authors have no other relevant affiliations or financial involvement with any organization or entity with a financial interest in or financial conflict with the subject matter or material discussed in the manuscript.
Reviewer disclosures
Peer reviewers on this manuscript have no relevant financial or other relationships to disclose.
Author contributions
G.S.D was involved in the conception and design of the study. C.F.B., A.Z., M.L., T.J., R.F., G.K., M.N., M.H., S.S., E.K., R.S., M.O., E.BR., H.A., I.A., H.A., M.C., F.C., A.B., M.H., A.H., G.AN., J.E.M., Z.D., M.Z., E.S., G.F.A., and Lebanese Inter-Hospital Pneumococcal Surveillance Program investigators were involved in the data and samples collection. L.R., N.Y., C.F.B., A.AC., D.F., S.K., F.A., M.F., A.A.R., B.H., and MB.M. were involved in the analysis and interpretation of the data. G.S.D., L.R., N.Y., and C.F.B. were involved in the drafting of the paper and revising it critically for intellectual content. All authors approved the submitted version; and agreed to be accountable for all aspects of the work.
Supplemental Material
Download MS Word (492.1 KB)Acknowledgments
We acknowledge the LIPSP participating hospitals, i.e. laboratory directors and technicians for help in sample processing and data collection.
Supplementary material
Supplemental data for this article can be accessed online at https://doi.org/10.1080/14760584.2022.2143349
Additional information
Funding
References
- Collaborators GLRI, Blacker B, Khalil IA. Estimates of the global, regional, and national morbidity, mortality, and aetiologies of lower respiratory infections in 195 countries, 1990-2016: a systematic analysis for the Global Burden of Disease Study 2016. Lancet Infect Dis. 2018Nov;18(11):1191–1210. PubMed PMID: 30243584; PubMed Central PMCID: PMCPMC6202443.
- Bonnave C, Mertens D, Peetermans W, et al. Adult vaccination for pneumococcal disease: a comparison of the national guidelines in Europe. Eur J Clin Microbiol Infect Dis. 2019Apr;384:785–791. PubMed PMID: 30778705; eng
- CENTERS FOR DISEASE CONTROL AND PREVENTION. Global Pneumococcal Disease and Vaccination 2019 [ updated 27/01/2022; cited 2022 19 OCT 2022]. Available from: https://www.cdc.gov/pneumococcal/global.html
- Wasserman MD, Perdrizet J, Grant L, et al. Clinical and Economic Burden of Pneumococcal Disease Due to Serotypes Contained in Current and Investigational Pneumococcal Conjugate Vaccines in Children Under Five Years of Age. Infect Dis Ther. 2021Dec;10 4:2701–2720. PubMed PMID: 34633639; PubMed Central PMCID: PMCPMC8503717. eng
- Croucher NJ, Lochen A, Bentley SD. Pneumococcal Vaccines: Host Interactions, Population Dynamics, and Design Principles. Annu Rev Microbiol. 2018 Sep 8;72(1):521–549. PubMed PMID: 30200849.
- Nelson AL, Roche AM, Gould JM, et al. Capsule enhances pneumococcal colonization by limiting mucus-mediated clearance. Infect Immun. 2007Jan;751:83–90. PubMed PMID: 17088346; PubMed Central PMCID: PMCPMC1828419
- Kraicer-Melamed H, O’Donnell S, Quach C. The effectiveness of pneumococcal polysaccharide vaccine 23 (PPV23) in the general population of 50 years of age and older: A systematic review and meta-analysis. Vaccine. 2016 Mar 18;34(13):1540–1550. PubMed PMID: 26899372.
- Moberley S, Holden J, Tatham DP, et al. Vaccines for preventing pneumococcal infection in adults. Cochrane Database Syst Rev. CD000422. PubMed PMID: 23440780; PubMed Central PMCID: PMCPMC7045867. 2013 Jan;31(1).
- Prato R, Fortunato F, Cappelli MG, et al. Effectiveness of the 13-valent pneumococcal conjugate vaccine against adult pneumonia in Italy: a case-control study in a 2-year prospective cohort. BMJ Open. 2018;2018 Mar 25;8(3):e019034. PubMed PMID: 29581200; PubMed Central PMCID: PMCPMC5875676.
- Feldman C, Anderson R. Recent advances in the epidemiology and prevention of Streptococcus pneumoniae infections. F1000Res. 2020;9:338. PubMed PMID: 32411353; PubMed Central PMCID: PMCPMC7212261. eng.
- Rodgers GL, Whitney CG, Klugman KP. Triumph of Pneumococcal Conjugate Vaccines: Overcoming a Common Foe. J Infect Dis. 2021;224(Supplement_4):S352–S359.
- Hanquet G, Krizova P, Valentiner-Branth P, et al. Effect of childhood pneumococcal conjugate vaccination on invasive disease in older adults of 10 European countries: implications for adult vaccination. Thorax. 2019;74(5):473.
- Geno KA, Gilbert GL, Song JY, et al. Pneumococcal Capsules and Their Types: Past, Present, and Future. Clin Microbiol Rev. 2015Jul;283:871–899. PubMed PMID: 26085553; PubMed Central PMCID: PMCPMC4475641
- Masomian M, Ahmad Z, Gew LT, et al. Development of Next Generation Streptococcus pneumoniae Vaccines Conferring Broad Protection. Vaccines (Basel). 2020 Mar 17;8(1). PubMed PMID: 32192117; PubMed Central PMCID: PMCPMC7157650.
- Daniels CC, Rogers PD, Shelton CM. A Review of Pneumococcal Vaccines: Current Polysaccharide Vaccine Recommendations and Future Protein Antigens. J Pediatr Pharmacol Ther. 2016Jan-Feb;21(1):27–35. PubMed PMID: 26997927; PubMed Central PMCID: PMCPMC4778694.
- Pitsiou GG, Kioumis IP. Pneumococcal vaccination in adults: does it really work? Respir Med. 2011Dec;105(12):1776–1783. PubMed PMID: 21816596.
- Song JY, Moseley MA, Burton RL, et al. Pneumococcal vaccine and opsonic pneumococcal antibody. J Infect Chemother. 2013Jun;193:412–425. PubMed PMID: 23657429; PubMed Central PMCID: PMCPMC3692352
- Hurley D, Griffin C, Young M, et al. Safety, Tolerability, and Immunogenicity of a 20-Valent Pneumococcal Conjugate Vaccine (PCV20) in Adults 60 to 64 Years of Age. Clin infect Dis. 2020 Jul 27. PubMed PMID: 32716500.
- Peterson JT, Stacey HL, MacNair JE, et al. Safety and immunogenicity of 15-valent pneumococcal conjugate vaccine compared to 13-valent pneumococcal conjugate vaccine in adults >/=65 years of age previously vaccinated with 23-valent pneumococcal polysaccharide vaccine. Hum Vaccin Immunother. 2019;15(3):540–548. PubMed PMID: 30427749; PubMed Central PMCID: PMCPMC6605723.
- Greenberg D, Hoover PA, Vesikari T, et al. Safety and immunogenicity of 15-valent pneumococcal conjugate vaccine (PCV15) in healthy infants. Vaccine. 2018 Oct 29;36(45):6883–6891. PubMed PMID: 30244873.
- McFetridge R, Meulen AS, Folkerth SD, et al. Safety, tolerability, and immunogenicity of 15-valent pneumococcal conjugate vaccine in healthy adults. Vaccine. 2015 Jun 4;33(24):2793–2799. PubMed PMID: 25913828.
- U.S. Food and Drug Administration. Vaxneuvance. 2021 [cited 2021 Oct 5]. https://www.fda.gov/vaccines-blood-biologics/vaccines/vaxneuvance
- U.S. Food and Drug Administration. Prevnar 20. 2021 [cited 2021 Oct 5]. https://www.fda.gov/vaccines-blood-biologics/vaccines/prevnar-20
- Grabenstein JD. Effectiveness and serotype coverage: key criteria for pneumococcal vaccines for adults. Clinical Infectious Diseases. 2012 Jul;55(2):255–258. DOI:10.1093/cid/cis354. PubMed PMID: 22495543.
- Grabenstein JD, Weber DJ. Pneumococcal serotype diversity among adults in various countries, influenced by pediatric pneumococcal vaccination uptake. Clin infect Dis. 2014Mar;58(6):854–864. PubMed PMID: 24344141.
- Weinberger DM, Malley R, Lipsitch M. Serotype replacement in disease after pneumococcal vaccination. Lancet. 2011 Dec 3;378(9807):1962–1973. PubMed PMID: 21492929; PubMed Central PMCID: PMCPMC3256741.
- Liu Z, Nachamkin I, Edelstein PH, et al. Serotype emergence and genotype distribution among macrolide-resistant invasive Streptococcus pneumoniae isolates in the postconjugate vaccine (PCV-7) era. Antimicrob Agents Chemother. 2012Feb;562:743–750. PubMed PMID: 22123697; PubMed Central PMCID: PMCPMC3264211
- Reslan L, Finianos M, Bitar I, et al. The Emergence of Invasive Streptococcus pneumoniae Serotype 24F in Lebanon: Complete Genome Sequencing Reveals High Virulence and Antimicrobial Resistance Characteristics. Front Microbiol. 2021;12:637813. PubMed PMID: 33746930; PubMed Central PMCID: PMCPMC7967862.
- Hanna-Wakim R, Chehab H, Mahfouz I, et al. Epidemiologic characteristics, serotypes, and antimicrobial susceptibilities of invasive Streptococcus pneumoniae isolates in a nationwide surveillance study in Lebanon [Research Support, Non-U.S. Gov’t]. Vaccine. 2012;30(Suppl 6):G11–7. PubMed PMID: 23228352.
- Palmu AA, De Wals P, Toropainen M, et al. Similar impact and replacement disease after pneumococcal conjugate vaccine introduction in hospitalised children with invasive pneumococcal disease in Europe and North America. Vaccine. 2021 Mar 12;39(11):1551–1555. PubMed PMID: 33610373.
- International LRCAoSC, Labour Organization (ILO); European Union (EU). Labour Force and Household Living Conditions Survey 2020 [cited 2022 19 OCT 2022]. Available from: http://www.cas.gov.lb/images/Publications/Labour%20Force%20and%20Household%20Living%20Conditions%20Survey%202018-2019.pdf
- CENTERS FOR DISEASE CONTROL AND PREVENTION. Invasive Pneumococcal Disease (IPD) (Streptococcus pneumoniae) 2010 [ updated 16/04/2021; cited 2022 19 OCT 2022]. Available from: https://ndc.services.cdc.gov/case-definitions/invasive-pneumococcal-disease-2010/
- CLSI. Performance standards for antimicrobial susceptibility testing. 30thed. Wayne PA:Clinical and Laboratory Standards Institute;2020. CLSI supplement M100.
- Jin P, Kong F, Xiao M, et al. First report of putative Streptococcus pneumoniae serotype 6D among nasopharyngeal isolates from Fijian children. J Infect Dis. 2009 Nov 1;200(9):1375–1380. PubMed PMID: 19803727.
- Jin P, Xiao M, Kong F, et al. Simple, accurate, serotype-specific PCR assay to differentiate Streptococcus pneumoniae serotypes 6A, 6B, and 6C. J Clin Microbiol. 2009Aug;478:2470–2474. PubMed PMID: 19535528; PubMed Central PMCID: PMCPMC2725661
- General Assembly of the World Medical Association. World Medical Association Declaration of Helsinki: ethical principles for medical research involving human subjects. J Am Coll Dent. 2014 Summer;81(3):14–18. PubMed PMID: 25951678; eng
- Wagenvoort GH, Sanders EA, Vlaminckx BJ, et al. Sex differences in invasive pneumococcal disease and the impact of pneumococcal conjugate vaccination in the Netherlands, 2004 to 2015. Euro Surveill. 2017 Mar 9;22(10). PubMed PMID: 28300529; PubMed Central PMCID: PMCPMC5356421.
- de St Maurice A, Schaffner W, Griffin MR, et al. Persistent Sex Disparities in Invasive Pneumococcal Diseases in the Conjugate Vaccine Era. J Infect Dis. 2016 Sep 1;214(5):792–797. PubMed PMID: 27247342; PubMed Central PMCID: PMCPMC4978370.
- Mokaddas E, Albert MJ. Impact of pneumococcal conjugate vaccines on burden of invasive pneumococcal disease and serotype distribution of Streptococcus pneumoniae isolates: an overview from Kuwait. Vaccine. 2012 Dec 31;30(Suppl 6):G37–40. PubMed PMID: 23228356.
- Al-Sheikh YA, KG L, Mohammed Ali MM, et al. Distribution of serotypes and antibiotic susceptibility patterns among invasive pneumococcal diseases in Saudi Arabia. Ann Lab Med. 2014May;343:210–215. PubMed PMID: 24790908; PubMed Central PMCID: PMCPMC3999319
- Ktari S, Jmal I, Mroua M, et al. Serotype distribution and antibiotic susceptibility of Streptococcus pneumoniae strains in the south of Tunisia: A five-year study (2012-2016) of pediatric and adult populations. Int J Infect Dis. 2017 Dec;65:110–115. PubMed PMID: 29111412.
- Al-Jardani A, Al Rashdi A, Al Jaaidi A, et al. Serotype distribution and antibiotic resistance among invasive Streptococcus pneumoniae from Oman post 13-valent vaccine introduction. Int J Infect Dis. 2019 Aug;85:135–140. PubMed PMID: 31150842
- Cui YA, Patel H, O’Neil WM, et al. Pneumococcal serotype distribution: A snapshot of recent data in pediatric and adult populations around the world. Hum Vaccin Immunother. 2017 Jun 3;13(6):1–13. PubMed PMID: 28125317; PubMed Central PMCID: PMCPMC5489298.
- Hausdorff WP, Feikin DR, Klugman KP. Epidemiological differences among pneumococcal serotypes. Lancet Infect Dis. 2005Feb;5(2):83–93. PubMed PMID: 15680778
- Rendi-Wagner P, Georgopoulos A, Kundi M, et al. Prospective surveillance of incidence, serotypes and antimicrobial susceptibility of invasive Streptococcus pneumoniae among hospitalized children in Austria. J Antimicrob Chemother. 2004May;535:826–831. PubMed PMID: 15073163
- Mokaddas EM, Shibl AM, Elgouhary A, et al. Effect of the introduction of pneumococcal conjugate vaccines on serotype prevalence in Kuwait and Saudi Arabia. Vaccine. 2018 Oct 15;36(43):6442–6448. PubMed PMID: 30194003; eng.
- Ricketson LJ, Bettinger JA, Sadarangani M, et al. Vaccine effectiveness of the 7-valent and 13-valent pneumococcal conjugate vaccines in Canada: An IMPACT study. Vaccine. 2022;40(19):2733–2740.
- Bryant KA, Block SL, Baker SA, et al. Safety and immunogenicity of a 13-valent pneumococcal conjugate vaccine. Pediatrics. 2010May;1255:866–875. PubMed PMID: 20435707; eng
- Memish ZA, El-Saed A, Al-Otaibi B, et al. Epidemiology of invasive pneumococcal infection in children aged five years and under in Saudi Arabia: a five-year retrospective surveillance study. Int J Infect Dis. 2010Aug;148:e708–12. PubMed PMID: 20627645
- Torres A, Blasi F, Dartois N, et al. Which individuals are at increased risk of pneumococcal disease and why? Impact of COPD, asthma, smoking, diabetes, and/or chronic heart disease on community-acquired pneumonia and invasive pneumococcal disease. Thorax. 2015Oct;70 1:984–989. PubMed PMID: 26219979; PubMed Central PMCID: PMCPMC4602259
- Belman S, Chaguza C, Kumar N, et al. A new perspective on ancient Mitis group streptococcal genetics. Microb Genom. 2022 Feb;8(2). PubMed PMID: 35225216; PubMed Central PMCID: PMCPMC8942026. eng.
- Cillóniz C, Garcia-Vidal C, Ceccato A, et al. Antimicrobial Resistance Among Streptococcus pneumoniae. Antimicrobial Resistance in the 21st Century. 2018;7:13–38. eng DEP - 20180307.
- El Moujaber G, Osman M, Rafei R, et al. Molecular mechanisms and epidemiology of resistance in Streptococcus pneumoniae in the Middle East region. J Med Microbiol. 2017Jul;667:847–858. PubMed PMID: 28650313
- Jamsheer A, Rafay AM, Daoud Z, et al. Results from the Survey of Antibiotic Resistance (SOAR) 2011-13 in the Gulf States. J Antimicrob Chemother. 2016May;71 Suppl 1suppl 1:i45–61. PubMed PMID: 27048582; PubMed Central PMCID: PMCPMC4890347
- Soyletir G, Altinkanat G, Gur D, et al. Results from the Survey of Antibiotic Resistance (SOAR) 2011–13 in Turkey. J Antimicrob Chemother. 2016May;Suppl 71suppl 1:i71–83. PubMed PMID: 27048584; PubMed Central PMCID: PMCPMC4890354
- Shibl AM, Memish ZA, Al-Kattan KM. Antibiotic resistance and serotype distribution of invasive pneumococcal diseases before and after introduction of pneumococcal conjugate vaccine in the Kingdom of Saudi Arabia (KSA). Vaccine. 2012 Dec 31;30(Suppl 6):G32–6. PubMed PMID: 23228355.
- Moghnieh R, Araj GF, Awad L, et al. A compilation of antimicrobial susceptibility data from a network of 13 Lebanese hospitals reflecting the national situation during 2015-2016. Antimicrob Resist Infect Control. 2019;8(1):41. PubMed PMID: 30828445; PubMed Central PMCID: PMCPMC6381724.
- Chamoun K, Farah M, Araj G, et al. Surveillance of antimicrobial resistance in Lebanese hospitals: retrospective nationwide compiled data. Int J Infect Dis. 2016 May;46:64–70. PubMed PMID: 26996458
- Lieberthal AS, Carroll AE, Chonmaitree T, et al. The diagnosis and management of acute otitis media. Pediatrics. 2013Mar;1313:e964–99. PubMed PMID: 23439909
- Metlay JP, Waterer GW, Long AC, et al. Diagnosis and Treatment of Adults with Community-acquired Pneumonia. An Official Clinical Practice Guideline of the American Thoracic Society and Infectious Diseases Society of America. Am J Respir Crit Care Med. 2019 Oct 1;200(7):e45–e67. PubMed PMID: 31573350; PubMed Central PMCID: PMCPMC6812437.
- Habibian S, Mehrabi-Tavana A, Ahmadi Z, et al. Serotype distribution and antibiotics susceptibility pattern of Streptococcus pneumonia in Iran. Iran Red Crescent Med J. 2013Oct;1510:e8053. PubMed PMID: 24693373; PubMed Central PMCID: PMCPMC3950785
- Buchy P, Ascioglu S, Buisson Y, et al. Impact of vaccines on antimicrobial resistance. Int J Infect Dis. 2020 Jan;90:188–196. PubMed PMID: 31622674