ABSTRACT
Fusobacterium nucleatum, an anaerobic Gram-negative bacterium primarily residing in the oral cavity, has garnered significant attention for its emerging role in cancer progression and prognosis. While extensive research has revealed mechanistic links between Fusobacterium nucleatum and colorectal cancer, a comprehensive review spanning its presence and metastatic implications in cancers beyond colorectal origin is conspicuously absent. This paper broadens our perspective from colorectal cancer to various malignancies associated with Fusobacterium nucleatum, including oral, pancreatic, esophageal, breast, and gastric cancers. Our central focus is to unravel the mechanisms governing Fusobacterium nucleatum colonization, initiation, and promotion of metastasis across diverse cancer types. Additionally, we explore Fusobacterium nucleatum‘s adverse impacts on cancer therapies, particularly within the domains of immunotherapy and chemotherapy. Furthermore, this paper underscores the clinical research significance of Fusobacterium nucleatum as a potential tumor biomarker and therapeutic target, offering a novel outlook on its applicability in cancer detection and prognostic assessment.
1. Introduction
Fusobacterium nucleatum (F. nucleatum), a member of the genus Fusobacterium, is a gram-negative, non-spore-forming, obligate anaerobic bacterium with a slender shape and fusiform ends.Citation1 Initially considered a commensal bacterium primarily inhabiting the oral cavity of humans and animals, its frequent isolation during disease activity has led to the recognition of F. nucleatum as an opportunistic pathogen. Current understanding positions F. nucleatum as a potential contributor to the pathogenesis of several human diseases.Citation1 In disease contexts, including gastrointestinal tumors, breast cancer, and other malignancies, F. nucleatum is emerging as a prevalent species outside the oral environment ().Citation11 Furthermore, mounting evidence implicates F. nucleatum in the development of various gastrointestinal tumors, breast cancer, and other malignancies (). While earlier research has firmly established F. nucleatum as a catalyst for the progression and severity of colorectal cancer (CRC), recent investigations have unveiled its presence in a spectrum of gastrointestinal and extragastrointestinal tumors, often associated with a poor prognosis.Citation12 F. nucleatum engages in tumorigenesis and metastasis across diverse cancer types through multifaceted mechanisms, including immune response modulation, virulence factors, oncogenic microRNAs, intestinal metabolite interactions, DNA damage, and epithelial-mesenchymal transition.Citation13–15 Additionally, compelling research suggests that F. nucleatum may exert detrimental effects on cancer treatment outcomes.Citation16 For instance, in patients with esophageal squamous cell carcinoma, a high abundance of F. nucleatum has been linked to adverse responses to neoadjuvant chemotherapy.Citation17
Table 1. F. nucleatum and tumor.
In this comprehensive review, we systematically elucidate the pathogenic mechanisms through which F. nucleatum promotes the development of various tumors, including CRC, oral cancer, pancreatic cancer, breast cancer, esophageal squamous cell carcinoma cancer(ESCC), and gastric cancer(GC). We also explore its utility as a predictive marker for early detection and as a therapeutic target for the aforementioned cancers. Furthermore, we delve into the intricate mechanisms by which F. nucleatum interferes with the efficacy of chemotherapeutic agents, compromising treatment outcomes. Additionally, we investigate its impact on anti-PD-1 inhibitors, shedding light on the complex interplay between F. nucleatum and immunotherapy. These insights shed light on the intricate mechanisms and clinical implications of F. nucleatum in tumor progression, metastasis, and therapeutic resistance.
2. Origin, colonization, and metastasis
The prevalence of Fusobacterium species in healthy tissues, such as the human oral cavity and various mucosal regions, and the paramount presence of F. nucleatum within the local microbiota in these domains. Notably, F. nucleatum emerges as the preeminent species within the oral cavity. Its significance stems from two pivotal factors: Firstly, F. nucleatum assumes a pivotal role in orchestrating the development of biofilms, intricately weaving connections with both the oral microbiota and adjacent tissues. In doing so, it exerts a profound influence over the state of periodontal health and the dynamics of disease. Through the expression of adhesion proteins like radiation-sensitive DNA adhesins(RadD), F. nucleatum acts as a bridge between early colonizers (e.g., Streptococci) and subsequent colonizers (e.g., Porphyromonas gingivalis). This facilitates the formation of biofilms that firmly adhere to tooth surfaces.Citation15 Furthermore, F. nucleatum adheres to the biofilm and, through various adhesion mechanisms such as RadD, Fusobacterium autotransporter protein 2(Fap2), and Fusobacterium outer membrane protein A(FapA), residues 68–123 to 68–125, facilitates the aggregation of secondary colonies. Such a nuanced interplay enables F. nucleatum to mediate intricate interactions, not only within its realm but also with actinomycetes and the fungus Candida albicans. As a consequence, it assumes the role of a structural support system, robustly withstanding the cleansing forces exerted by saliva and the gingival sulcus fluid.Citation1,Citation15
While F. nucleatum is a frequent inhabitant of the oral cavity, its presence within the gut microbiota is conspicuously limited. Given the anatomical connection between the oral cavity and the intestinal tract, and the identification of identical F. nucleatum strains in both oral and gastrointestinal cancer samples, it is reasonable to postulate that F. nucleatum found in gut tumors may originate from the oral cavity.Citation18 Recent investigations have elucidated F. nucleatum‘s capacity to endure acidic environments and navigate the gastrointestinal tract, particularly in the presence of Fusobacterium adhesin A (FadA).Citation19 Continuous swallowing of saliva, rich in bacteria, provides a potential pathway for gastrointestinal transmission. This is corroborated by the higher prevalence of F. nucleatum and FadA in fecal samples from CRC patients.Citation20 Additionally, the identification of F. nucleatum strains within colon cancer tissues subsequent to intravenous injection hints at the prospect of systemic colonization through circulation.Citation21 Furthermore, the localization of F. nucleatum within tumors can be attributed to glycan-lectin interactions. As a galactose-adhesive hemagglutinin, Fap2 has been shown to mediate F. nucleatum colonization by binding to the tumor-overexpressed host factor Gal-GalNAc.Citation22 Gal-GalNAc is overexpressed in various tumor types, including ovarian cancer, prostate cancer, CRC, pancreatic cancer, and breast cancer.Citation15,Citation23 Concurrently, the DNA load of F. nucleatum significantly escalates within these tumors, implying that F. nucleatum may accumulate in malignancies exhibiting elevated levels of Gal-GalNAc.Citation7,Citation10 Although research confirming the Fap2/Gal-GalNAc mechanism for F. nucleatum translocation in OSCC tissues is lacking, peanut agglutinin staining has unveiled preferential F. nucleatum binding to OSCC tissue, suggestive of Gal-GalNAc expression in OSCC tissues.Citation24,Citation25 Collectively, these findings posit a plausible mechanism for F. nucleatum translocation.
3. F. nucleatum and tumorigenesis tumor metastasis
Recent research has increasingly illuminated the pivotal role played by F. nucleatum in the pathogenesis of various malignancies. Advances in microbial multi-omics technology have profoundly enriched our comprehension of the intricate mechanisms underpinning F. nucleatum‘s contributions to oncogenesis and metastasis(). In the following sections, we shall embark on an exploration of the potential mechanisms through which F. nucleatum actively orchestrates its participation in tumor development.
Table 2. Summary of F. nucleatum pro-tumorigenic and pro-metastasis mechanisms.
3.1. Colorectal cancer
CRC stands as the second most prominent cause of cancer-related mortality worldwide, ranking third in terms of overall cancer incidence.Citation21,Citation70 The etiology of cancer is profoundly multifactorial, influenced by a myriad of factors including dietary habits, lifestyle, genetic predispositions (e.g., familial polyposis syndrome, family history of CRC, hereditary non-polyposis syndromes), chronic inflammatory bowel diseases (such as Crohn’s disease and ulcerative colitis), and the complex interplay with the gut microbiota.Citation12,Citation71 Additionally, Furthermore, a significant contributor to high mortality in CRC is the dissemination of cancer cells to distant organs and tissues.Citation72 Through a number of processes (EMT, immunosuppression, synthesis of matrix metalloproteinases (MMPs), etc.), F. nucleatum acts a crucial part in the metastasis of CRC. Recent advancements in microbiological detection techniques have yielded compelling evidence highlighting the involvement of F. nucleatum in the progression of CRC.Citation1,Citation71
Previous investigations have revealed that F. nucleatum may contribute to CRC development and metastasis by actively subduing the host’s immune response against tumors, particularly in the context of CRC liver metastasis(). Statistically, patients grappling with CRC liver metastases (CRCLM) face significantly diminished 5-year overall survival rates (16.9%) compared to their counterparts without such metastases (70.4%).Citation73 In the ensuing exploration, we shall dissect the intricate involvement of F. nucleatum in CRC. Notably, F. nucleatum selectively fosters the expansion of myeloid-derived suppressor cells (MDSCs), which, in turn, nurture tumor growth and abet tumor immunosuppression while concurrently promoting angiogenesis.Citation26,Citation27 In the realm of CRC, F. nucleatum exerts its influence by precipitating T cell apoptosis, stifling T cell proliferation, thereby orchestrating a reconfiguration of the tumor microenvironment (TME) conducive to tumorigenesis and metastasis.Citation74,Citation75 Additionally, F. nucleatum interacts with cellular TLR4, instigating the expression of S100A9, a damage-associated molecular pattern protein, and triggering the activation of M2 macrophages through nuclear factor-κB (NF-κB) activation. This cascade, in turn, engenders tumor cell proliferation and migration.Citation28,Citation76 Furthermore, Gram-negative bacteria, including F. nucleatum, release outer membrane vesicles (OMVs), extracellular vesicles containing cytoplasmic and periplasmic components enclosed within a protein-lipid bilayer.Citation77 F. nucleatum has been substantiated as an activator of TLR4 through the discharge of OMVs, instigating a sequence that involves NF-κB, CREB, extracellular signal-regulated kinases, and inflammatory cytokines within human cell lines and colon-like monolayers. This intricate cascade culminates in inflammation and fosters intestinal carcinogenesis, as exemplified by the APCMin/+ CRC model.Citation31,Citation32 In addition, Yin et al. discovered that pro-inflammatory cytokines from the bloodstream enter the liver, resulting in the recruitment of hepatic MDSCs, attraction of CD8+ T cells, polarization of tumor-associated macrophages, and accumulation of regulatory T cells (Tregs). T These processes give rise to the establishment of a chronic inflammatory and immunosuppressive milieu, which, in turn, propels the development of CRCLM. Notably, the presence of F. nucleatum was associated with a reduction in the diversity of intestinal bacteria, ultimately leading to dysbiosis. Yin et al. also observed that F. nucleatum amplifies the relative abundance of Aspergillus intestinalis while diminishing the relative abundance of the Methanobacterium phylum.Citation33 Furthermore, the burgeoning population of intestinal microorganisms may yield metabolically deleterious substances like prostaglandins and bile acids. These substances can reach the liver through the portal vein and the hepatic intestinal circulation, thereby facilitating further hepatic metastasis of CRC.Citation78,Citation79 Recent studies have unveiled the intricate mechanisms through which F. nucleatum thwarts the anti-tumor immune response, encompassing the promotion of Foxp3+ regulatory T cell proliferation and the concurrent inhibition of effector T cell proliferation and function.Citation29,Citation80 Fathi et al. illuminated a scenario in which the binding of Fap2 protein from F. nucleatum within the gastrointestinal tract to the T cell immunoreceptor with Ig and ITIM domains (TIGIT) can shield tumors from immune cell assault. This interaction effectively inhibits the function of natural killer (NK) cells in releasing a myriad of chemokines and cytokines.Citation30,Citation81 Another analogous mechanism revolves around the binding of F. nucleatum to carcinoembryonic antigen-related cell adhesion molecule 1 (CEACAM1), subsequently triggering the suppression of immune cell activity. This exertion of a protective influence against tumor development is driven by the stimulation of CEACAM1, a carcinoembryonic antigen-related cell adhesion molecule ubiquitously expressed on various tumor surfaces. The stimulation, in turn, leads to the attenuation of immune cell activity and a subsequent reduction in their capacity to produce interferon-γ. These collective events contribute to the promotion of CRC development.Citation82
Figure 1. F. nucleatum promotes tumorigenesis and metastasis by influencing the immune response. Fn, fusobacterium nucleatum. Figure created with BioRender.com.

Moreover, F. nucleatum wields influence over the expression of oncogenic RNAs, a factor that potentially contributes to the initiation and progression of CRC(). Recent studies have discovered that F. nucleatum stimulates the proliferation and tumorigenesis of CRC cells by triggering TLR4 signaling, as shown by an increase in the expression of microRNA-21 or miR21.Citation34,Citation83 Employing CRC modeling and metabolic scrutiny involving 33 CRC patients, Hong et al. unearthed that F. nucleatum amplifies the binding of SP1 to the promoter region of long non-coding RNA (lncRNA) ENO1-IT1, thereby kindling transcription. This upsurge in ENO1 expression propels glycolysis, resulting in an accumulation of lactic acid, which in turn diminishes the pH of the TME and fosters the proliferation of malignant cells.Citation35 Furthermore, through an examination of exosomes in the sera of 82 patients afflicted with primary CRC, Guo et al. have underscored that F. nucleatum has the capacity to elevate the levels of miR-1246/92b-3p/27a-3p within circulating exosomes. These exosomes are then transported into uninfected cells, amplifying tumor invasion and metastasis.Citation36 Recent in vitro co-culture experiments and analytical assays conducted by Zheng et al. have elucidated that F. nucleatum engenders the enrichment of hypoxia-inducible factor-1α (HIF-1α) and acetylation of histone H3 lysine 27 (H3K27Ac) within the gene promoter region. Both H3K27Ac and HIF-1α collaborate to upregulate the expression of angiopoietin-like protein 4, thus promoting glycolysis and angiogenesis. These molecular orchestration events not only sustain F. nucleatum colonization but also advance CRC development.Citation37 F. nucleatum doesn’t solely impact tumorigenesis by modulating RNA and protein expression; it also plays a significant role in promoting tumor metastasis. The ensuing mechanisms elucidate how F. nucleatum facilitates CRC metastasis. One integral component of the cytoskeleton is Keratin 7, which not only upholds cellular structural integrity but also augments cell motility. Chen et al.‘s study showcased that F. nucleatum spurs in vitro migration and in vivo metastasis of CRC cells by elevating the expression of long non-coding RNA Keratin7-antisense and Keratin7.Citation38 During epithelial-mesenchymal transition (EMT), epithelial cells lose their ability to adhere to the extracellular matrix (ECM) and neighboring cells, giving rise to the unique invasive and migratory capacity of mesenchymal stem cells.Citation84 Emerging evidence suggests that both in vivo and in vitro metastatic capacity of CRC cells are associated with increased expression of the long noncoding RNA EVADR, a process linked to F. nucleatum infection.Citation39,Citation40 EMT-associated variables such as Slug, Zeb1, and Snail have been found to be mitigated through the increased presence of EVADR, indicating that F. nucleatum-induced EVADR might facilitate CRC dissemination. Moreover, at the protein transcription level, Kong et al. identified that F. nucleatum promotes CYP2J2 transcription through activation of the TLR4/AKT/NRF2 signaling pathway, ultimately promoting EMT and CRC metastasis.Citation41 Cancer cells embark on their journey from the primary tumor site during the intricate process of metastasis, with their adherence to endothelial cells representing a pivotal step. Thus, tumor metastasis closely correlates with alterations in the function or expression of cell adhesion molecules. For instance, Zhang et al. demonstrated that F. nucleatum binds to the receptor ALPK1, activates the NF-κB pathway, and induces the expression of intercellular adhesion molecule 1 (ICAM1), thereby facilitating tumor metastasis. This suggests that F. nucleatum enhances the adhesion of CRC cells to endothelial cells, consequently promoting extravasation and metastasis.Citation42,Citation85 Additionally, a carefully regulated lysosomal management process known as cellular autophagy has been associated with the emergence of tumor metastasis. In advanced tumor stages, the activation of autophagy promotes tumor cell invasion and migration. Chen et al. found that F. nucleatum activates Nod-like receptors by upregulating the expression of a related protein gene, recruitment structural domain 3 (CARD3), subsequently inducing cellular autophagy and CRC metastasis.Citation43 N6-methyladenine (m6A) is a prevalent RNA modification that plays a pivotal role in regulating various aspects of RNA functionality, including stability, translocation, translation, and degradation. Kinesin Family Member 26B (KIF26B) is a gene encoding a protein with multifaceted involvement in essential biological processes, notably cell migration and cell division. Consequently, any aberrant expression or mutations in KIF26B may have significant implications, particularly in the context of tumorigenesis.Citation86 Current research has linked dysregulated m6A modifications and elevated KIF26B expression to the progression of breast, hepatocellular, and GC.Citation87 In a recent study, F. nucleatum was observed to activate the Hippo-Yes-associated protein (YAP) signaling pathway by up-regulating Yamaguchi sarcoma viral oncogene homolog 1-associated transcription factors, leading to the suppression of forkhead box (FOXD3) expression. Furthermore, FOXD3 was identified as a positive transcription factor for methyltransferase-like 3 (METTL3), and its inhibition resulted in the downregulation of METTL3 expression. The reduction in METTL3 expression subsequently promoted invasion and metastasis in CRC through two distinct pathways: the first pathway involved decreased KIF26B degradation, while the second pathway resulted in reduced m6A modification. These findings underscore the intricate interplay between m6A modifications, KIF26B, and the regulatory effects of F. nucleatum on the YAP signaling pathway, shedding light on their collective contribution to CRC progression.Citation44 In conclusion, F. nucleatum plays a multifaceted role in tumor metastasis, impacting cellular motility, EMT, adhesion to endothelial cells, autophagy, and mRNA modification. These complex mechanisms underscore the significant contribution of F. nucleatum to CRC metastasis.
Figure 2. F. Nucleatum promotes tumorigenesis metastasis through affecting RNA expression. Figure created with BioRender.com.
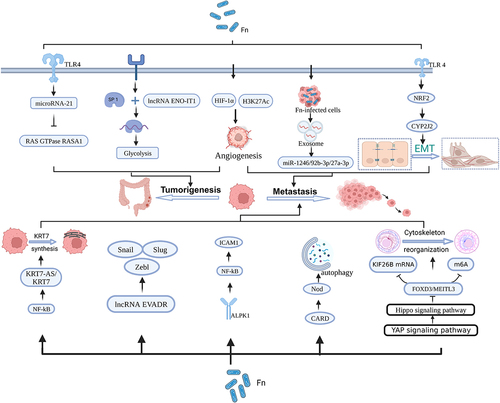
Extensive DNA damage can ensue due to chemical exposure, exogenous physical agents such as UV light, or cellular metabolism.Citation88,Citation89 Prolonged exposure to these detrimental DNA factors results in substantial damage that surpasses the human system’s capacity for repair. Consequently, damaged DNA instigates mutations in proto-oncogenes, cell cycle control genes, and tumor suppressor genes, ultimately leading to tumorigenesis ().Citation90 Recent publications have established connections between higher levels of F. nucleatum in CRC tissue and the CpG island methylator phenotype, as well as widespread microsatellite instability at a high frequency (MSI-H). In their analysis of tumor tissue from 304 CRC patients, Okita et al. revealed that a high load of F. nucleatum could induce DNA damage and DNA mismatch repair, thereby contributing to the association between cancer progression and MSI.Citation45,Citation91 Sayed et al. discovered that F. nucleatum downregulates the levels of NEIL2 (an oxidized base-specific DNA glycosylase) in colonic epithelial cells, resulting in increased inflammation and inducing the accumulation of DNA double-strand breaks (DSBs), leading to the development of CRC.Citation47 In addition, F. nucleatum-induced reactive oxygen species (ROS)-mediated DNA damage may exacerbate CRC progression. Koi et al. experimentally found that ROS generated by F. nucleatum infection caused DNA damage while inducing the associated MSH2 and MSH6 to form a heterodimer MutSα, for repair of damaged DNA. The mechanism of repair is that MutSα and DNA methyltransferase and the polycomb repressive complex 4 work together to repair DNA methylation at CpG-island, which in turn inhibits gene transcription and thus plays a role in repair. However, concomitantly, CIMP suppresses the expression of oncogenes, thus promoting tumorigenesis.Citation46
Metabolites of microbial origin, including compounds like succinic acid, lactic acid, fumaric acid, L-2-hydroxyglutaric acid, and others, can accumulate within tumors and actively participate in the development of malignancies ().Citation49,Citation92 It is reported that cancer stem cells possess the capability to regenerate tumor heterogeneity and self-renew, contributing to tumor initiation and progression.Citation93 Ternes et al. revealed that the F. nucleatum-derived metabolite formate enhances the self-renewal capacity of colorectal tumor cells to increase tumor formation and cancer stem cells.Citation94 Recent investigations have shown that F. nucleatum releases succinate, which influences the inflammatory response and facilitates CRC tumorigenesis by interacting with succinate receptors expressed on immune cells.Citation49,Citation50 F. nucleatum utilizes peptides and amino acids as nutritional sources to generate short-chain fatty acids (SCFAs) within the TME. SCFAs and the signaling pathways involved in their detection play intricate parts in gut defense and carcinogenesis. Under certain conditions, SCFAs, such as butyrate, have also been shown to stimulate colonic epithelial and immunosuppressive cell proliferation which in turn promotes tumor development.Citation51 Overall, F. nucleatum metabolites can contribute to tumor progression and growth by suppressing antitumor immune cells, boosting tumor cell proliferation, and enhancing tumor angiogenesis.
Virulence factor-mediated mechanisms employed by F. nucleatum also wield considerable influence in the realm of tumorigenesis(). FadA, a primary virulence factor of F. nucleatum, facilitates bacterial adhesion to the host mucosal surface and induces damage to endothelial or epithelial cells.Citation72 In addition, FadA promotes β-catenin signaling and regulates E-cadherin, which increases the expression of Wnt genes, inflammatory genes, transcription factors, and oncogenes as well as DNA damage-mediated growth stimulation of CRC cells.Citation52,Citation95,Citation96 Another pivotal virulence factor, Fap2, adheres to the Gal-GalNAc polysaccharide generated by CRC cells, inducing lymphocyte demise, or it binds to immunoglobulins and immunoreceptor tyrosine-based inhibition motifs (ITIMs), thereby suppressing the activity of immune cells. These actions foster the formation and progression of CRC.Citation15,Citation53,Citation97 One of the most prevalent virulence agents of F. nucleatum, LPS, stimulates the TLR4/p-PAK1/p-catenin S675-cascade signaling pathway, which in turn propels CRC cell proliferation and carcinogenesis.Citation32
F. nucleatum intricately contributes to CRC development through a multitude of mechanisms encompassing immune response modulation, DNA damage induction, the generation of carcinogenic metabolites, dysregulated RNA expression, and the influence of virulence factors. Furthermore, there exists a noteworthy interplay between these mechanisms, wherein F. nucleatum not only incites inflammation by modulating immune responses but also fuels inflammation through the production of aberrant metabolites.
3.2. Oral cancer
Head and neck cancer constitutes a pervasive global health challenge, ranking as the sixth most frequently diagnosed malady on a worldwide scale.Citation98,Citation99 Among the array of head and neck cancer subtypes, oral cancer emerges as the most prevalent, casting a substantial shadow over human well-being and health. It bears the hallmark of a dire prognosis and notably meager survival rates, primarily stemming from its innate proclivity for metastasis.Citation24 OSCC, a major form of oral cancer, arises from the complex interplay of diverse factors, including lifestyle choices (such as alcohol consumption, tobacco smoking, and betel quid usage), genetic predispositions, and intricate oral microbiome composition.Citation100,Citation101 Recent advancements in sequencing and microbial culture technologies have unveiled a staggering diversity in the human oral cavity, housing over 700 bacterial species from more than 20 phyla.Citation102 Amongst the denizens of this oral milieu, F. nucleatum has assumed a prominent role as a consequential instigator in the genesis and metastasis of oral cancer ().
Figure 4. Mechanism of F. Nucleatum for oral cancer, pancreatic cancer, and breast cancer. Figure created with BioRender.com.
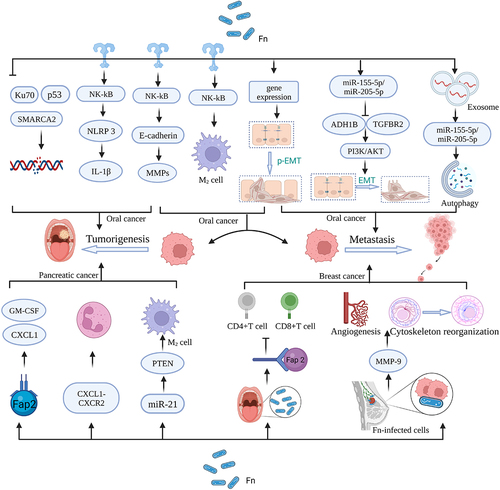
A pivotal mechanism underpinning potential tumorigenesis revolves around the regulation of protein expression. MMPs, colloquially known as matrix metalloproteinases, represent endopeptidases hinging on the presence of zinc ions for their catalytic activity. They play a crucial role in degrading ECM proteins, including collagen, laminin, elastin, and fibronectin, facilitating ECM remodeling.Citation21,Citation103 In a trailblazing endeavor, Gallimidi et al. established a murine model for the inception of oral carcinogenesis and unearthed a momentous revelation: F. nucleatum possesses the capacity to instigate the activation of the STAT3 signaling pathway within OSCC, achieved through its direct interaction with oral epithelial cells via Toll-like receptors.Citation54 STAT3, the key signaling molecule responsible for regulating the synthesis of various proteins, significantly upregulates the expression of cell cycle proteins, cell survival markers, and MMPs upon activation, stimulating OSCC cell proliferation and invasion and metastasisCitation10,Citation104
In terms of shaping the tumor microenvironment, F. nucleatum becomes an important inducer in tumorigenesis and metastasis. F. nucleatum directly elevates the expression of pro-interleukin (IL)-1β via the NF-κB pathway. Activation of the NLRP3 inflammasome leads to the conversion of the cytokine precursor interleukin into its mature, biologically active form. This, in turn, fosters tumor cell proliferation and malignant transformation.Citation55,Citation105 Additionally, the release of OMVs by F. nucleatum promotes the polarization of macrophages toward the pro-inflammatory M1 phenotype. These polarized macrophages secrete cytokines, creating an inflammatory microenvironment that contributes to tumorigenesis in adjacent tissues.Citation106 In addition, the abundant presence of F. nucleatum in tumor tissues can promote head and neck squamous cell carcinomas (HNSCC) metastasis by activating TLR4 leading to M2-type macrophage polarization.Citation56
DNA damage, a recurrent mechanism in carcinogenesis, also constitutes a vital component in the potential mechanisms underlying this process. Recently, Geng et al. have noted that the presence of F. nucleatum downregulates the expression of DNA repair proteins Ku70 and p53, as evidenced by Western blot techniques. This downregulation results in DSBs and DNA damage, compromising the stability and integrity of the genome and elevating the susceptibility to OSCC development.Citation57 The process of DNA replication and repair receives substantial support from the ATP-dependent chromatin remodeling complex, known as SMARCA2. Chen et al.‘s analysis of 68 HNSCC tumor tissues revealed a correlation between increased F. nucleatum presence and hyper-methylation of tumor suppressor genes SMARCA2 and LXN. This hyper-methylation inhibits DNA repair processes, thus fostering HNSCC growth.Citation13
EMT, a complex biological phenomenon, involves the transformation of cells from an epithelial phenotype characterized by cell-cell adhesion and polarity to a mesenchymal phenotype marked by enhanced motility and invasiveness.Citation107 Partial EMT (p-EMT) denotes a transitional state where cells exhibit both epithelial and mesenchymal characteristics.Citation108 These processes, EMT and p-EMT, play crucial roles in various biological phenomena, with particular relevance in the context of cancer metastasis.Citation58 In addition to the aforementioned processes involved in OSCC carcinogenesis, EMT and p-EMT further contribute to the intricate landscape of tumor progression.Citation109–111 OSCC cells exposed to F. nucleatum undergo a shift toward a mesenchymal state, as indicated by the downregulation of epithelial markers such as E-cadherin and the upregulation of mesenchymal markers like N-cadherin and Vimentin.Citation112 Furthermore, F. nucleatum-derived OMVs have been implicated in the modulation of autophagy-related genes (such as Beclin-1, ATG3, and ATG7) within oral cancer cells, thereby influencing the process of EMT and facilitating lung metastasis.Citation58 Additionally, the promotion of tumor metastasis is associated with the upregulation of genes related to p-EMT.Citation110 It is important to note that in laryngeal squamous cell carcinoma (LSCC), F. nucleatum exhibits contrasting effects. Here, it inhibits the expression of alcohol dehydrogenase 1B and transforming growth factor-β receptor 1 through the up-regulation of miR-155-5p and miR-205-5p, ultimately leading to the activation of EMT.Citation59 These findings underscore the context-dependent nature of F. nucleatum‘s impact on tumor progression.
3.3 Pancreatic cancer
Pancreatic cancer holds the 12th position in terms of overall cancer incidence, but it is a significant contributor to cancer-related mortality, ranking seventh in lethality.Citation113 Despite not being among the most commonly diagnosed cancers, pancreatic cancer presents a dire prognosis due to its insidious and highly lethal nature.Citation114 Several factors contribute to the development of pancreatic cancer, encompassing population aging, tobacco use, obesity, alcohol consumption, diabetes, and genetic predispositions.Citation115 Additionally, emerging research has highlighted the intricate relationship between pancreatic cancer and microorganisms.Citation45,Citation116,Citation117 In a particular study, F. nucleatum was identified within pancreatic tumor cells at significantly elevated levels compared to controls, suggesting its potential association with pancreatic cancer development().Citation60 Furthermore, recent findings have shed light on F. nucleatum‘s interaction with pancreatic cancer cells, primarily through the Fap2 adhesin. This interaction paves the way for F. nucleatum infection in pancreatic cancer, wherein infected tumor cells release specific cytokines like GM-CSF, CXCL1, IL-8, and MIP-3α, thereby promoting further tumor progression. For instance, GM-CSF significantly accelerates the proliferation of pancreatic cancer cells, while CXCL1 plays a pivotal role in metastasis and chemotherapy resistance in pancreatic cancer. Moreover, F. nucleatum has been found to stimulate tumor cell proliferation and migration by invading normal pancreatic epithelial cells.Citation118 Additionally, recent research has revealed that F. nucleatum within pancreatic tumors can influence pancreatic cancer development by reshaping the tumor immune microenvironment. CXCL1, a chemokine, functions as an immune cell chemoattractant and is involved in inflammatory and immune responses. Its binding to CXCR2, a specific receptor, triggers various signaling events, particularly affecting tumor proliferation and migration. Experimental evidence has shown that intratumoral F. nucleatum promotes tumor growth by enhancing the secretion of CXCL1 from pancreatic cancer cells in an autocrine manner. Furthermore, intratumoral F. nucleatum recruits MDSCs and suppresses CD8+ T cells in the tumor microenvironment via the CXCL1-CXCR2 axis in a paracrine manner, facilitating immune evasion by the tumor.Citation119 Immune evasion mechanisms are further exemplified by the role of PTEN, a crucial tumor suppressor. Dysfunction or mutations in the PTEN gene lead to an immune-suppressive tumor microenvironment characterized by the modulation of M2 macrophages, MDSCs, and Tregs. F. nucleatum has been shown to downregulate PTEN expression by upregulating miR-21 levels, promoting immune evasion by pancreatic cancer cells.Citation15 In summary, while pancreatic cancer may not be among the most frequently diagnosed cancers, it presents a formidable challenge due to its poor prognosis. The interplay between F. nucleatum and pancreatic cancer, as evidenced by its presence within tumor cells and its impact on the tumor microenvironment, underscores the need for further research into the mechanisms underlying its involvement in cancer development and immune evasion.Citation45
3.4. Breast cancer
Breast cancer, the most prevalent malignancy among women, has witnessed a concerning surge in incidence over the past four decades.Citation120 Accumulating evidence indicates a close relationship between microbial dysbiosis and breast cancer.Citation121 In recent years, the presence of F. nucleatum has been unequivocally established in human breast cancer tissue, and its capacity to expedite breast cancer progression has been substantiated in murine models().Citation60 Hieken et al. also inferred F. nucleatum as a risk factor for breast cancer development through experiments as well as a series of risk meta-analyses.Citation122 While several postulated causative factors have been proposed, the precise mechanisms underpinning F. nucleatum‘s colonization and its promotion of breast cancer progression remain, as yet, not fully elucidated.Citation10 Concerning the mechanisms entailed in F. nucleatum‘s colonization of breast tumors, firstly, it is essential to acknowledge that breast cancer fosters a distinct TME that provides a conducive milieu for the proliferation of F. nucleatum.Citation123 Secondly, the virulence factor FapA, which possesses an affinity for and binds to Gal-GalNAc, serves as a critical mediator in the colonization of F. nucleatum, thereby instigating tumorigenesis.Citation21,Citation30 Fap2, another protein produced by F. nucleatum, operates through the same colonization pathway to suppress CD4+ and CD8+ T cells, ultimately inducing lymphocyte death. This process contributes to breast cancer metastasis and expedites the growth of breast cancer metastatic lesions.Citation10 Furthermore, the up-regulation of MMP9, also triggered by F. nucleatum colonization, may enhance breast cancer metastasis by promoting angiogenesis and facilitating cytoskeletal rearrangement.Citation10
3.5. Esophageal cancer
Esophageal cancer, characterized by a dismal prognosis and elevated mortality rates, ranks as the seventh leading cause of cancer-related fatalities in men. Risk factors implicated in esophageal disease encompass alcohol consumption, tobacco use, acid reflux, obesity, esophageal stricture, and cardia incontinence.Citation120 In recent years, it has been found that there is a direct correlation between elevated levels of F. nucleatum in esophageal cancer tissues and decreased overall survival as well as a high degree of tumor malignancy.Citation4,Citation5,Citation16 While some evidence has been gathered, the mechanisms of how F. nucleatum affects this disease remain uncertain. This paper therefore outlines a preliminary mechanism for the role of F. nucleatum in esophageal carcinogenesis.
Moreno et al. experimentally proposed that F. nucleatum binds to ESCC cells utilizing Fad A and activates chemokines such as CCL20, thereby promoting aggressive tumor behavior.Citation61 In addition, co-culture experiments have revealed that F. nucleatum may increase CD8+ T cell, KIR2DL1 expression over time, thereby weakening the immune system’s response to cancer and reducing the sensitivity of tumor cells to cisplatin.Citation62 Pertaining to immunological aspects, a congruence has been discerned between F. nucleatum infiltration and the recruitment of Treg cells. Consequently, chronic F. nucleatum infection may contribute to the malignant progression of esophageal cancer by attracting Treg cells, thereby stifling immune responses, fostering tumor cell proliferation, facilitating immune escape, and ultimately engendering long-term colonization.Citation63 It has also been found that F. nucleatum promotes tumor invasiveness by affecting lymphocyte density in esophageal cancer.Citation64 Furthermore, the overexpression of inducible nitric oxide synthase (iNOS) stands as another conceivable mechanism linking F. nucleatum to esophageal carcinogenesis.Citation124 The expression of iNOS can escalate due to the presence of lipopolysaccharides in Gram-negative bacteria. This can result in the reduction of esophageal sphincter relaxation and an associated rise in the risk of esophageal cancer.Citation65 Augmented COX-2 expression, Toll-like receptor activation, and the effects of secreted metabolites are additional potential conduits through which F. nucleatum exerts its influence on esophageal cancer.Citation125 In a 2023 study, Ding et al. reported that F. nucleatum infection led to an increase in the concentration of compounds such as putrescine and spermine, which are known to have favorable effects on the metastasis of ESCC.Citation66 Moreover, nascent evidence suggests a plausible association between the presence of F. nucleatum and ESCC metastasis, although the precise underlying mechanisms necessitate further elucidation.Citation126
3.6. Gastric cancer
GC is one of the most common malignancies worldwide and the fourth leading cause of cancer-related death. GC is a multifactorial disease in which both environmental and genetic factors influence its occurrence and progression.Citation127 Within the realm of the gastric microbiota, a diverse array of guest bacteria, originating from the oral cavity and establishing residence in the lower intestine, coexist with native bacterial inhabitants. Notably, an array of studies have shed light on the pivotal role of the microbiota in the genesis of GC.Citation128,Citation129 Helicobacter pylori (H. pylori) is now widely recognized as a major risk factor for GC.Citation130 In addition to H. pylori, the entire complex microbiome in the gastrointestinal tract may trigger more aggressive oncogenic effects.Citation1,Citation67 However, our understanding of F. nucleatum‘s role in GC and precancerous gastric lesions remains limited, necessitating in-depth exploration of the implicated mechanisms. The putative mechanisms underpinning F. nucleatum‘s involvement in gastric carcinoma development are expounded upon below. It has been reported that F. nucleatum utilizes FadA to bind to E-cadherin and activates β-catenin signaling, thereby promoting various inflammatory and oncogenic properties of cells.Citation131 Given the close association between the diffuse type of GC and E-cadherin dysregulation, one may conjecture a potential molecular mimicry of the diffuse GC subtype and its specific prognostic implications.Citation67 According to a study from Taiwan, the colonization of F. nucleatum was able to alter the dynamics of actin filaments and promote the mobility and invasiveness of GC cells. In a recent study, it was discovered that GC cells infected with F. nucleatum produced exosomes, leading to an increase in HOTTIP expression and promoting GC invasion via the miR-885-3p/EphB2/PI3K/AKT axis.Citation132 These revelations underscore the plausible role of F. nucleatum in instigating the metastatic progression of GC. Moreover, H. pylori is acknowledged for its contribution to cancer metastasis, primarily through mechanisms that instigate epithelial-mesenchymal transition and cell invasion. A cohort analysis revealed that GC patients co-infected with F. nucleatum and H. pylori experienced a worse prognosis, indicating a synergistic effect of these two pathogens in promoting GC invasiveness.Citation68 Another study proposed that F. nucleatum-induced transcriptional activation in neutrophils might be linked to gastric carcinogenesis through various candidate genes, with suggested functional mechanisms including organelle dysfunction and intracellular transport dysregulation.Citation69 Intriguingly, the impact of F. nucleatum appears to differ among GC subtypes, with F. nucleatum-positive patients displaying a poorer prognosis in the context of Lauren diffuse GC, while no substantial difference was noted in intestinal GC patients. This observation hints at the prospect that F. nucleatum may exert disparate modes of action within the domain of Lauren diffuse GC,Citation67 although further research is required to elucidate the precise mechanisms involved.
In summary, there is an urgent need for further investigations to comprehensively understand the role of F.nucleatum in the development of GC and its potential impact on patient outcomes.
4. F. nucleatum and tumor resistance
F. nucleatum frequently assumes a role in undermining tumor therapy, posing substantial challenges for both patients and healthcare providers. In addition to the aforementioned arguments, it is imperative to delve into the potential mechanisms through which F. nucleatum may contribute to a better understanding of the nexus between F. nucleatum and resistance to tumor treatment. At present, the fulcrum of tumor therapy revolves around immunotherapy, with a central focus on targeting the PD-1/PD-L1 axis. PD-1, a programmed death molecule expressed on T-cells, encounters its immunosuppressive ligand, PD-L1, residing on the surface of tumor cells. This interaction has the capacity to neutralize T-cell activity against cancer cells.Citation17 Inhibitors targeting these receptors hold promise in restoring the immune system’s ability to recognize and effectively eliminate cancer cells. However, the presence of F. nucleatum can impede the action of anti-PD-1 inhibitors. Recent research illuminates that succinic acid, a derivative produced by F. nucleatum, disrupts the GMP-AMP synthase-interferon-β pathway, consequently dampening the body’s responsiveness to anti-PD-1 monoclonal antibodies and diminishing immune efficacy in CRC.Citation133 Additionally, chemotherapeutic agents like oxaliplatin and 5-fluorouracil exert their therapeutic effects by disrupting the cell cycle.Citation134 Intriguingly, experimental evidence underscores F. nucleatum‘s propensity to induce cancer autophagy, accomplished through the selective suppression of miR-18a* and miR-4802 expression via the TLR4 and MYD88 pathways, thereby impacting resistance to chemotherapy in CRC.Citation135 Nevertheless, F. nucleatum can also instigate resistance to these drugs by upregulating the expression of the apoptosis inhibitor protein BIRC3 or the chloride channel protein ANO1.Citation136 This resistance pathway has been observed not only in CRC but also in ESCC. Additionally, studies have uncovered that F. nucleatum significantly diminishes the therapeutic efficacy of cisplatin chemotherapy and enhances tumor resistance by activating NLRP3 in ESCC cells, consequently augmenting MDSCs.Citation137 Another avenue through which F. nucleatum engenders drug resistance in ESCC is by inciting DNA damage in senescent tumor cells following chemotherapy, subsequently activating the DNA damage repair pathway. This process may prompt tumor cells to secrete senescence-associated phenotypes, further reinforcing their resistance to chemotherapy.Citation138 In OSCC, cisplatin resistance also correlates with the presence of F. nucleatum. F. nucleatum reduces the expression levels of p53 and E-cadherin, predominantly through the Wnt/NFAT pathway, thereby promoting cisplatin resistance in tumor cells.Citation139
5. The potential clinical value of F. nucleatum in tumors
The primary method of reducing tumor mortality is early detection. As previously elucidated, the heightened presence of F. nucleatum in individuals correlates with their tumor status, positioning F. nucleatum as a plausible biomarker for tumor screening, particularly as macroeconomic technologies have advanced.Citation140 A noninvasive CRC screening approach that uses a fecal occult blood test and a fecal immunochemical test to evaluate F. nucleatum burden in feces is currently suggested.Citation15 Furthermore, immunoassays based on F. nucleatum antibodies in serum and saliva are useful tools for CRC screening.Citation22 Indeed, F. nucleatum manifests in significantly greater concentrations in the feces of tumor-afflicted individuals compared to healthy controls. In response to the substantial F. nucleatum presence in CRC patients, the immune system produces IgA and IgG antibodies against F. nucleatum. Quantitative evaluation of these antibodies within circulating serum, facilitated by enzyme-linked immunosorbent assay (ELISA), has unveiled markedly elevated levels of IgA and IgG in CRC patients relative to their healthy counterparts.Citation141 Additionally, F. nucleatum encodes a distinctive amyloid adhesin complex known as FadA (FadAc), which plays a contributory role in tumorigenesis. Similar to anti-F. nucleatum antibodies, circulating serum antibodies targeting FadAc are predominantly of the IgA class. In the conducted study, ELISA was employed to detect anti-FadAc-IgA antibodies in the blood of healthy subjects, early-stage CRC patients, and advanced-stage CRC patients. Significantly higher antibody titers were observed in both early and advanced CRC patients when compared to those in the normal population. This compelling evidence suggests that circulating anti-FadAc-IgA antibodies may hold promise as a potential novel biomarker for the early diagnosis of CRC.Citation142 Elevated concentrations of F. nucleatum were also identified in tumor tissues and saliva of individuals afflicted with oral cancer.Citation143 Another investigation reported F. nucleatum to be twice as prevalent in the mouthwash of HNSCC patients compared to the control group.Citation144 In the realm of stomach cancer screening, where the invasiveness of gastroscopy and the limited specificity and sensitivity of conventional serum tumor markers hinder early detection, the heightened levels of F. nucleatum in patients’ saliva relative to controls offer a promising avenue for the development of a novel early diagnosis model for gastric cancer.Citation145 Notably, chemotherapy and immunotherapy serve as conventional modalities for cancer treatment; however, their efficacy is often compromised due to the interaction of F. nucleatum within tumors with these therapeutic agents or alterations in the immunological milieu of malignant tumors. These interactions contribute to the attenuation of drug sensitivity.Citation14
Moreover, building upon the preceding discourse regarding the pivotal role of F. nucleatum in carcinogenesis and metastasis, it is anticipated that F. nucleatum-targeted therapies will herald a novel paradigm in tumor treatment.Citation146 After receiving antibiotic treatment in animal models, F. nucleatum abundance was noticeably low in the tissues of CRC mice, and tumor growth was slowed. Phages armed with nanomaterial payloads exhibited the capability to selectively target F. nucleatum and impede CRC progression. Significantly, reshaping the composition of the gut microbiota stands as a pivotal strategy for CRC prevention and treatment. Probiotics and fecal microbiota transplantation have shown promise in mitigating CRC development through the reduction of F. nucleatum colonization and enhancement of gut barrier integrity. In addition to the aforementioned treatments, antimicrobial peptides (AMPs) have emerged as promising candidates in the realm of novel antimicrobial drugs. In a recent study, an amphiphilic AMP known as Jelleine-I (J-I) displayed remarkable anti-F. nucleatum activity, particularly its halogenated J-I derivatives, which exhibited significantly enhanced efficacy.Citation147 Mechanistically, these J-I derivatives not only suppressed F. nucleatum-induced inflammation but also acted as bactericidal agents by directly disrupting the F. nucleatum cell membrane. These dual actions played a significant role in inhibiting the progression of CRC. Furthermore, it’s worth noting that these antimicrobial peptides possess a unique advantage – they exhibit minimal cytotoxicity to colon epithelial cells at high doses. This characteristic positions them as a potential focal point in future anti-tumor research and development. FadA mutations are being used in breast cancer to decrease F. nucleatum colonization and cancer-promoting capacity, either alone or in combination with Fap2 antibodies.Citation148
In addition to its potential clinical significance as a diagnostic tool and therapeutic target in cancer, F. nucleatum may also serve as a predictive factor for patient prognosis. A clinical analysis revealed that stage IV CRC tissues exhibited significantly higher F. nucleatum levels than stage I-III CRC, suggesting a potential association between F. nucleatum abundance and unfavorable tumor prognosis.Citation149 Furthermore, the presence of F. nucleatum has been linked to the ability to predict patient survival in CRC cohorts. Elevated levels of F. nucleatum in CRC tissues were associated with decreased overall patient survival compared to cases with lower bacterial loadsCitation150 The higher abundance of F. nucleatum DNA in tumor tissues, along with poor clinical outcomes, may be attributed to its role in promoting mutation-related alterations in tumor molecular properties, such as high microsatellite instability (MSI).Citation151 Additionally, the F. nucleatum load has an impact on the prognosis of patients with metastatic colon cancer. A study analyzing DNA in tumor tissues from patients with metastatic colon cancer revealed that those with F. nucleatum-enriched tumors had significantly worse progression-free survival rates.Citation152 F. nucleatum also demonstrates its potential as a prognostic biomarker in pancreatic cancer. Long-term follow-up of pancreatic cancer patients showed significantly lower survival rates in the group with F. nucleatum-positive tumors compared to the control group.Citation153 In esophageal cancer, the F. nucleatum DNA content was notably higher in cancer tissues compared to normal esophageal mucosa, and this increase in DNA levels correlated with advanced tumor stages. Clinical studies further revealed that F. nucleatum positivity in esophageal cancer tissues was associated with shorter overall survival.Citation5 In esophageal squamous cell carcinoma, the primary subtype of esophageal cancer, the presence of F. nucleatum in tumor tissues was also associated with a higher risk of tumor metastasis, underscoring its prognostic significance.Citation126
F. nucleatum holds great promise in the fields of tumor diagnosis and prognostic biomarkers. However, its full potential is yet to be realized due to a shortage of extensive clinical samples and data for more in-depth exploration. Nevertheless, it is anticipated that advancements in technology and the utilization of multi-omics techniques will gradually unlock the untapped clinical value of F. nucleatum.
6. Discussion
This comprehensive review delves into the multifaceted mechanisms through which F. nucleatum contributes to carcinogenesis and metastasis, providing a nuanced understanding for researchers invested in unraveling the intricacies of F. nucleatum‘s role in malignancies. The spotlight is cast upon F. nucleatum‘s oncogenic impact, spanning realms of immunity, virulence factors, DNA damage, and metabolite mediation. Beyond the realms of immunological and virulence-driven carcinogenesis, F. nucleatum propels tumor metastasis through orchestration of EMT, exosome secretion, orchestration of intestinal flora dysbiosis, and cytoskeletal remodeling. In addition, the possible role of F. nucleatum in resistance in immunotherapy and chemotherapy of tumors has been described.
Additionally, the review underscores the pivotal role of F. nucleatum in the landscape of tumor diagnosis and treatment. The evolving landscape of PCR and sequencing technologies holds promise in harnessing alterations in F. nucleatum as prospective biomarkers for tumor diagnosis and prognostication. This promise emanates from the integral role F. nucleatum plays in the intricate tapestry of tumorigenesis and metastasis.
However, it is imperative to acknowledge the limitations inherent in the current body of research. F. nucleatum, as a prevalent oral flora, stands vulnerable to a plethora of external and intrinsic host influences. These multifarious factors may introduce biases in investigations pertaining to tumor pathways, as well as in the arena of tumor diagnosis and prognostication. Furthermore, the localization of F. nucleatum within the tumor milieu – whether it resides within the microvascular lumen, the extracellular matrix, or intracellularly within tumor cells – potentially yields distinct regulatory effects on tumor behavior. These divergent influences may potentially reshape our comprehension of the mechanistic underpinnings of F. nucleatum within tumors and its amenability to future targeting strategies – an aspect sometimes overlooked in current research. Moreover, it is essential to underscore that F. nucleatum has yet to yield tumor diagnostic results that attain both sensitivity and specificity. Hence, an imperative mandate beckons for comprehensive multigenic research endeavors that delve deeper into the multifarious roles microbes play in the genesis and progression of cancer. In conclusion, the exploration of F. nucleatum‘s intricate relationship with tumors has endured over a considerable span of time, primarily constrained to correlation studies owing to the paucity of comprehensive clinical datasets. Nonetheless, the quest to unravel the multifaceted roles enacted by F. nucleatum in the realms of carcinogenesis and metastasis remains an ongoing endeavor. Recent strides in multi-omics technologies have ushered in a transformative era, unveiling a treasure trove of cancer-related biomarkers originating from both tumor cells and microorganisms within the intricate tapestry of the T. These advancements have served as a formidable catalyst in sculpting innovative diagnostic and therapeutic paradigms. Notable examples encompass the application of metabolomics investigations, harnessing single-cell methodologies to delve into the intricacies of tumor microbial proteomics, and the relentless evolution of genomics and transcriptomics, with a pronounced emphasis on the intricate dance of nucleic acids – namely, RNA and DNA – in the domain of carcinoma-microbe interplay.Citation154 Current research priorities include the discovery of biomarkers for tumor diagnosis and prognosis, as well as the development of tailored therapeutics, both of which offer novel directions for future clinical applications.
7. Conclusion
In summation, it is unequivocally established that microorganisms entwined with tumorous entities assume a pivotal role in the intricate orchestration of tumorigenesis, metastatic propagation, and the dynamic responses to cancer therapeutics. TME, a veritable crucible of molecular interactions, undergoes profound reconfiguration through the intricate interplay among immune constituents, specifically F. nucleatum, and malignant cells. Notably, immune effectors wield direct influence over the multifaceted impact of F. nucleatum on the genesis of malignancies, thereby shaping the intricate landscape in which microorganisms interface with anti-cancer modalities. This accentuates the burgeoning significance of scrutinizing F. nucleatum‘s contributory role in the oncogenic narrative, a realm progressively expanded by the burgeoning domain of multi-omics research dedicated to this microorganism’s enigma. In this context, the convergence of F. nucleatum-targeted therapeutic strategies with conventional anti-cancer pharmacopoeia beckons as a propitious avenue within the panorama of cancer management. Indeed, this review delineates the specific mechanisms by which F. nucleatum orchestrates and potentiates tumorigenesis, metastatic cascade, and resistance to conventional therapeutic regimens. It also underscores contemporary strides in the associated disciplines of study. Furthermore, the pivotal role played by F. nucleatum in the precincts of cancer diagnosis and therapeutic interventions assumes merited prominence. It furnishes discerning scholars with a comprehensive comprehension of this intricate terrain. Foreseeing the horizon, one anticipates that robust database sharing, the amalgamation of diverse indicators into a cohesive framework, and the aggregation of clinical datasets shall expedite the elucidation of the nuanced mechanistic underpinnings and the translation of F. nucleatum‘s clinical applicability within the panorama of tumorigenesis
Abbreviations
F. nucleatum | = | Fusobacterium nucleatum |
CRC | = | Colorectal cancer |
OSCC | = | Oral squamous cell carcinoma |
GC | = | Gastric cancer |
Gal-GalNAc | = | galactose-N-acetyl-D-galactosamine |
RadD | = | radiation-sensitive DNA adhesins |
Fap2 | = | Fusobacterium autotransporter protein 2 |
FadA | = | Fusobacterium adhesin A |
MDSCs | = | Myeloid-derived suppressor cells |
TME | = | tumor microenvironment |
TLR4 | = | Toll-like receptor 4 |
NF-κB | = | nuclear factor-κB |
OMVs | = | outer membrane vesicles |
Tregs | = | regulatory T cells |
CRCLM | = | CRC liver metastases |
TIGIT | = | T cell immunoreceptor with Ig and ITIM domains |
NK | = | natural killer |
CEACAM1 | = | carcinoembryonic antigen-related cell adhesion molecule 1 |
ITIM | = | immunoreceptor tyrosine-based inhibition motif |
LPS | = | lipopolysaccharides |
lncRNA | = | long non-coding RNA |
HIF-1α | = | hypoxia-inducible factor-1α |
H3K27Ac | = | histone H3 lysine 27 |
MSI-H | = | microsatellite instability at high frequency |
ECIM | = | extracellular matrix |
ICAM1 | = | intercellular adhesion molecule 1 |
m6A | = | N6-methyladenine |
KIF26B | = | Kinesin Family Member 26B |
YAP | = | Hippo-Yes-associated protein |
YES1 | = | Yamaguchi sarcoma viral oncogene homolog 1 |
FOXD3 | = | forkhead box |
METTL3 | = | methyltransferase-like 3 |
CARD3 | = | recruitment structural domain 3 |
SFAs | = | short-chain fatty acids |
MMPs | = | matrix metalloproteinases |
IL | = | interleukin |
HNSCC | = | head and neck squamous cell carcinomas |
EMT | = | Epithelial-mesenchymal transition |
FapA | = | Fusobacterium outer membrane protein A |
ESCC | = | esophageal squamous cell carcinoma |
iNOS | = | inducible nitric oxide synthase |
H. pylori | = | Helicobacter pylori |
ECM | = | extracellular matrix |
p-EMT | = | partial EMT |
LSCC | = | Laryngeal squamous cancer |
ELISA | = | enzyme-linked immunosorbent assay |
AMPs | = | antimicrobial peptides |
J-l | = | Jelleine-I |
CRediT authorship contribution statement
Haitao Sun: Conceptualization, Supervision. Chun Ye and Xiao Liu: Investigation, Writing – original draft. Zilun Liu and Chuxuan Pan: Writing – Review & Editing. Xiaowei Zhang and Zhanyi Zhao: visualization. All authors reviewed and approved the final manuscript to be published.
Acknowledgments
Thanks to the editors and reviewers for their hard work and important comments. We thank all those involved in writing and revising this manuscript. Also, we thank www.biorender.com for providing graphic design software.
Disclosure statement
No potential conflict of interest was reported by the author(s).
Additional information
Funding
Notes on contributors
Chun Ye
Chun Ye, studied at Southern Medical University for clinical medicine. Chun Ye has been involved in the research as a leader of a project on student innovation and entrepreneurship, focusing on the relationship between microorganisms and tumors.
Xiao Liu
Xiao Liu, studied at Southern Medical University for her clinical medical discipline. As a member of the university students’ innovation and entrepreneurship project, focusing on the relationship between microorganisms and tumor immunity.
Zilun Liu
Zilun Liu, attended Southern Medical University and brought a wealth of oncology knowledge from clinical internship at ZhuJiang Hospital. Zilun Liu’s research focuses on the relationship between tumors and microbial cultivation.
Chuxuan Pan
Chuxuan Pan, studied at Southern Medical University for clinical anesthesia. As a member of the university students’ innovation and entrepreneurship project, focusing on the relationship between related microorganisms and tumor therapy.
Xiaowei Zhang
Xiaowei Zhang, studied at Southern Medical University for clinical medicine. Xiaowei Zhang has been involved in a research project as the leader of a student innovation project, focusing on the relationship between microorganisms and tumor metastasis.
Zhanyi Zhao
Zhanyi Zhao received Master’s Degree in Neurosurgery from Zhujiang Hospital, Southern Medical University, focusing on the pathogenesis of brain tumors and tumor markers.
Haitao Sun
Haitao Sun, received M.D. & Ph.D. degree from Southern Medical University, and is currently working as the Director of Biospecimen Bank, Deputy Chief Physician, and Associate Researcher at Zhujiang Hospital, Southern Medical University. Currently, Haitao Sun main research interests are: precision detection and diagnosis, mechanism and intervention of major brain diseases (brain tumors, cerebrovascular diseases, etc.).
References
- Brennan CA, Garrett WS. Fusobacterium nucleatum - symbiont, opportunist and oncobacterium. Nat Rev Microbiol. 2019;17(3):156–19. doi:10.1038/s41579-018-0129-6.
- Zhang L, Liu Y, Zheng HJ, Zhang CP. The Oral Microbiota May Have Influence on Oral Cancer, Front Cell Infect Microbiol. Front Cell Infect Microbiol. 2019. 9:476. doi:10.3389/fcimb.2019.00476.
- Zhao H, Chu M, Huang Z, Yang X, Ran S, Hu B, Zhang C, Liang J. Variations in oral microbiota associated with oral cancer. Sci Rep. 2017;7(1):11773. doi:10.1038/s41598-017-11779-9.
- Shao D, Vogtmann E, Liu A, Qin J, Chen W, Abnet CC, Wei W. Microbial characterization of esophageal squamous cell carcinoma and gastric cardia adenocarcinoma from a high-risk region of China. Cancer. 2019;125(22):3993–4002. doi:10.1002/cncr.32403.
- Yamamura K, Baba Y, Nakagawa S, Mima K, Miyake K, Nakamura K, Sawayama H, Kinoshita K, Ishimoto T, Iwatsuki M, et al. Human Microbiome Fusobacterium Nucleatum in Esophageal Cancer Tissue Is Associated with Prognosis. Clin Cancer Res. 2016;22(22):5574–5581. doi:10.1158/1078-0432.Ccr-16-1786.
- Lee SA, Liu F, Riordan SM, Lee CS, Zhang L. Global Investigations of Fusobacterium nucleatum in Human Colorectal Cancer. Front Oncol. 2019;9:566. doi:10.3389/fonc.2019.00566.
- Castaño-Rodríguez N, Goh KL, Fock KM, Mitchell HM, Kaakoush NO. Dysbiosis of the microbiome in gastric carcinogenesis. Sci Rep. 2017;7(1):15957. doi:10.1038/s41598-017-16289-2.
- Hsieh YY, Tung SY, Pan HY, Yen CW, Xu HW, Lin YJ, Deng YF, Hsu WT, Wu CS, Li C. Increased abundance of Clostridium and Fusobacterium in gastric microbiota of patients with gastric cancer in Taiwan. Sci Rep. 2018;8(1):158. doi:10.1038/s41598-017-18596-0.
- Alkharaan H, Lu L, Gabarrini G, Halimi A, Ateeb Z, Sobkowiak MJ, Davanian H, Fernández Moro C, Jansson L, Del Chiaro M, et al., Circulating and salivary antibodies to Fusobacterium nucleatum are associated with cystic pancreatic neoplasm malignancy, Front Immunol 11 (2020) 2003. 10.3389/fimmu.2020.02003
- Parhi L, Alon-Maimon T, Sol A, Nejman D, Shhadeh A, Fainsod-Levi T, Yajuk O, Isaacson B, Abed J, Maalouf N, et al. Breast cancer colonization by Fusobacterium nucleatum accelerates tumor growth and metastatic progression. Nat Commun. 2020;11(1):3259. doi:10.1038/s41467-020-16967-2.
- Fan Z, Tang P, Li C, Yang Q, Xu Y, Su C, Li L. Fusobacterium nucleatum and its associated systemic diseases: epidemiologic studies and possible mechanisms. J Oral Microbiol. 2023;15(1):2145729. doi:10.1080/20002297.2022.2145729.
- Hashemi Goradel N, Heidarzadeh S, Jahangiri S, Farhood B, Mortezaee K, Khanlarkhani N, Negahdari B. Fusobacterium nucleatum and colorectal cancer: a mechanistic overview. J Cell Physiol. 2019;234(3):2337–2344. doi:10.1002/jcp.27250.
- Chen Z, Wong PY, Ng CWK, Lan L, Fung S, Li JW, Cai L, Lei P, Mou Q, Wong SH, et al. The intersection between oral microbiota, host gene methylation and patient outcomes in head and neck squamous cell carcinoma. Cancers Basel. 2020;12(11):3425. doi:10.3390/cancers12113425.
- Zhao K, Hu Y. Microbiome harbored within tumors: a new chance to revisit our understanding of cancer pathogenesis and treatment. Signal Transduct Target Ther. 2020;5(1):136. doi:10.1038/s41392-020-00244-1.
- Alon-Maimon T, Mandelboim O, Bachrach G. Fusobacterium nucleatum and cancer. Periodontol 2000. 2022;89(1):166–180. doi:10.1111/prd.12426.
- Yamamura K, Izumi D, Kandimalla R, Sonohara F, Baba Y, Yoshida N, Kodera Y, Baba H, Goel A. Intratumoral Fusobacterium Nucleatum levels predict therapeutic response to neoadjuvant chemotherapy in esophageal squamous cell carcinoma. Clin Cancer Res. 2019;25(20):6170–6179. doi:10.1158/1078-0432.CCR-19-0318.
- Ai L, Xu A, Xu J. Roles of PD-1/PD-L1 pathway: signaling, cancer, and beyond. Adv Exp Med Biol. 2020;1248:33–59. doi:10.1007/978-981-15-3266-5_3.
- Abed J, Maalouf N, Manson AL, Earl AM, Parhi L, Emgård JEM, Klutstein M, Tayeb S, Almogy G, Atlan KA, et al. Colon cancer-associated Fusobacterium nucleatum may originate from the oral cavity and reach colon tumors via the circulatory system. Front Cell Infect Microbiol. 2020;10:400. doi:10.3389/fcimb.2020.00400.
- Meng Q, Gao Q, Mehrazarin S, Tangwanichgapong K, Wang Y, Huang Y, Pan Y, Robinson S, Liu Z, Zangiabadi A, et al. Fusobacterium nucleatum secretes amyloid-like FadA to enhance pathogenicity. EMBO Rep. 2021;22(7):e52891. doi:10.15252/embr.202152891.
- Wirbel J, Pyl PT, Kartal E, Zych K, Kashani A, Milanese A, Fleck JS, Voigt AY, Palleja A, Ponnudurai R, et al. Meta-analysis of fecal metagenomes reveals global microbial signatures that are specific for colorectal cancer. Nat Med. 2019;25(4):679–689. doi:10.1038/s41591-019-0406-6.
- Abed J, Emgård JE, Zamir G, Faroja M, Almogy G, Grenov A, Sol A, Naor R, Pikarsky E, Atlan KA, et al. Fap2 Mediates Fusobacterium nucleatum Colorectal Adenocarcinoma Enrichment by Binding to Tumor-Expressed Gal-GalNAc. Cell Host & Microbe. 2016;20(2):215–225. doi:10.1016/j.chom.2016.07.006.
- Wang N, Fang JY. Fusobacterium nucleatum, a key pathogenic factor and microbial biomarker for colorectal cancer. Trends Microbiol. 2023;31(2):159–172. doi:10.1016/j.tim.2022.08.010.
- Abed J, Maalouf N, Parhi L, Chaushu S, Mandelboim O, Bachrach G. Tumor Targeting by Fusobacterium nucleatum: A Pilot Study and Future Perspectives. Front Cell Infect Microbiol. 2017;7:295. doi:10.3389/fcimb.2017.00295.
- McIlvanna E, Linden GJ, Craig SG, Lundy FT, James JA, Fusobacterium nucleatum and oral cancer: a critical review, BMC Cancer 21 (1) (2021) 1212. 10.1186/s12885-021-08903-4
- Amer A, Galvin S, Healy CM, Moran GP. The microbiome of potentially malignant oral leukoplakia exhibits enrichment for Fusobacterium, Leptotrichia, Campylobacter, and Rothia Species. Front Microbiol. 2017;8:2391. doi:10.3389/fmicb.2017.02391.
- Chen T, Li Q, Wu J, Wu Y, Peng W, Li H, Wang J, Tang X, Peng Y, Fu X. Fusobacterium nucleatum promotes M2 polarization of macrophages in the microenvironment of colorectal tumours via a TLR4-dependent mechanism. Cancer Immunol Immunother. 2018;67(10):1635–1646. doi:10.1007/s00262-018-2233-x.
- OuYang LY, Wu XJ, Ye SB, Zhang RX, Li ZL, Liao W, Pan ZZ, Zheng LM, Zhang XS, Wang Z, et al. Tumor-induced myeloid-derived suppressor cells promote tumor progression through oxidative metabolism in human colorectal cancer. J Transl Med. 2015;13(1):47. doi:10.1186/s12967-015-0410-7.
- Hu L, Liu Y, Kong X, Wu R, Peng Q, Zhang Y, Zhou L, Duan L. Fusobacterium nucleatum facilitates M2 macrophage polarization and colorectal carcinoma progression by activating TLR4/NF-κB/S100A9 cascade. Front Immunol. 2021;12:658681. doi:10.3389/fimmu.2021.658681.
- Mima K, Sukawa Y, Nishihara R, Qian ZR, Yamauchi M, Inamura K, Kim SA, Masuda A, Nowak JA, Nosho K, et al. Fusobacterium nucleatum and T cells in colorectal carcinoma. JAMA Oncol. 2015;1(5):653–661. doi:10.1001/jamaoncol.2015.1377.
- Gur C, Ibrahim Y, Isaacson B, Yamin R, Abed J, Gamliel M, Enk J, Bar-On Y, Stanietsky-Kaynan N, Coppenhagen-Glazer S, et al. Binding of the Fap2 protein of Fusobacterium nucleatum to human inhibitory receptor TIGIT protects tumors from immune cell attack. Immunity. 2015;42(2):344–355. doi:10.1016/j.immuni.2015.01.010.
- Engevik MA, Danhof HA, Ruan W, Engevik AC, Chang-Graham AL, Engevik KA, Shi Z, Zhao Y, Brand CK, Krystofiak ES, et al. Fusobacterium nucleatum Secretes Outer Membrane Vesicles and Promotes Intestinal Inflammation. mBio. 2021;12(2). doi:10.1128/mBio.02706-20.
- Wu Y, Wu J, Chen T, Li Q, Peng W, Li H, Tang X, Fu X. Fusobacterium nucleatum Potentiates Intestinal Tumorigenesis in Mice via a Toll-Like Receptor 4/p21-Activated Kinase 1 Cascade. Dig Dis Sci. 2018;63(5):1210–1218. doi:10.1007/s10620-018-4999-2.
- Yin H, Miao Z, Wang L, Su B, Liu C, Jin Y, Wu B, Han H, Yuan X. Fusobacterium nucleatum promotes liver metastasis in colorectal cancer by regulating the hepatic immune niche and altering gut microbiota, aging (Albany NY. 2022.14 (4). 1941–1958.10.18632/aging.203914
- Yang Y, Weng W, Peng J, Hong L, Yang L, Toiyama Y, Gao R, Liu M, Yin M, Pan C, et al. Fusobacterium nucleatum increases proliferation of colorectal cancer cells and tumor development in mice by activating toll-like receptor 4 signaling to nuclear factor-κB, and up-regulating expression of MicroRNA-21. Gastroenterology. 2017;152(4):851–866.e24. doi:10.1053/j.gastro.2016.11.018.
- Hong J, Guo F, Lu SY, Shen C, Ma D, Zhang X, Xie Y, Yan T, Yu T, Sun T, et al. F.Nucleatum targets lncRNA ENO1-IT1 to promote glycolysis and oncogenesis in colorectal cancer. Gut. 2021;70(11):2123–2137. doi:10.1136/gutjnl-2020-322780.
- Guo S, Chen J, Chen F, Zeng Q, Liu WL, Zhang G. Exosomes derived from Fusobacterium nucleatum-infected colorectal cancer cells facilitate tumour metastasis by selectively carrying miR-1246/92b-3p/27a-3p and CXCL16. Gut. 2020;70(8):1507–1519. doi:10.1136/gutjnl-2020-321187.
- Zheng X, Liu R, Zhou C, Yu H, Luo W, Zhu J, Liu J, Zhang Z, Xie N, Peng X, et al. ANGPTL4-mediated promotion of glycolysis facilitates the colonization of Fusobacterium nucleatum in colorectal cancer. Cancer Res. 2021;81(24):6157–6170. doi:10.1158/0008-5472.Can-21-2273.
- Chen S, Su T, Zhang Y, Lee A, He J, Ge Q, Wang L, Si J, Zhuo W, Wang L. Fusobacterium nucleatum promotes colorectal cancer metastasis by modulating KRT7-AS/KRT7. Gut Microbes. 2020;11(3):511–525. doi:10.1080/19490976.2019.1695494.
- Mohan CD, Rangappa S, Nayak SC, Sethi G, Rangappa KS. Paradoxical functions of long noncoding RNAs in modulating STAT3 signaling pathway in hepatocellular carcinoma. Biochimica Et Biophysica Acta (BBA) - Reviews On Cancer. 2021;1876(1):188574. doi:10.1016/j.bbcan.2021.188574.
- Lu X, Xu Q, Tong Y, Zhang Z, Dun G, Feng Y, Tang J, Han D, Mao Y, Deng L, et al. Long non-coding RNA EVADR induced by Fusobacterium nucleatum infection promotes colorectal cancer metastasis. Cell Rep. 2022;40(3):111127. doi:10.1016/j.celrep.2022.111127.
- Kong C, Yan X, Zhu Y, Zhu H, Luo Y, Liu P, Ferrandon S, Kalady MF, Gao R, He J, et al. Fusobacterium Nucleatum Promotes the Development of Colorectal Cancer by Activating a Cytochrome P450/Epoxyoctadecenoic Acid Axis via TLR4/Keap1/NRF2 Signaling. Cancer Res. 2021;81(17):4485–4498. doi:10.1158/0008-5472.Can-21-0453.
- Zhang Y, Zhang L, Zheng S, Li M, Xu C, Jia D, Qi Y, Hou T, Wang L, Wang B, et al. Fusobacterium nucleatum promotes colorectal cancer cells adhesion to endothelial cells and facilitates extravasation and metastasis by inducing ALPK1/NF-κB/ICAM1 axis. Gut Microbes. 2022;14(1):2038852. doi:10.1080/19490976.2022.2038852.
- Chen Y, Chen Y, Zhang J, Cao P, Su W, Deng Y, Zhan N, Fu X, Huang Y, Dong W. Fusobacterium nucleatum promotes metastasis in colorectal cancer by activating autophagy signaling via the upregulation of CARD3 expression. Theranostics. 2020;10(1):323–339. doi:10.7150/thno.38870.
- Chen S, Zhang L, Li M, Zhang Y, Sun M, Wang L, Lin J, Cui Y, Chen Q, Jin C, et al. Fusobacterium nucleatum reduces METTL3-mediated m(6)A modification and contributes to colorectal cancer metastasis. Nat Commun. 2022;13(1):1248. doi:10.1038/s41467-022-28913-5.
- Li R, Hu Y, Hou S. An Exploration of Oral-Gut Pathogens Mediating Immune Escape of Pancreatic Cancer via miR-21/PTEN Axis. Front Microbiol. 2022;13:928846. doi:10.3389/fmicb.2022.928846.
- Koi M, Okita Y, Carethers JM. Fusobacterium nucleatum Infection in Colorectal Cancer: Linking Inflammation, DNA Mismatch Repair and Genetic and Epigenetic Alterations. J Anus Rectum Colon. 2018;2(2):37–46. doi:10.23922/jarc.2017-055.
- Sayed IM, Chakraborty A, Abd El-Hafeez AA, Sharma A, Sahan AZ, Huang WJM, Sahoo D, Ghosh P, Hazra TK, Das S. The DNA glycosylase NEIL2 suppresses fusobacterium-infection-induced inflammation and DNA damage in colonic epithelial cells. Cells. 2020;9(9):1980. doi:10.3390/cells9091980.
- Ternes D, Tsenkova M, Pozdeev VI, Meyers M, Koncina E, Atatri S, Schmitz M, Karta J, Schmoetten M, Heinken A, et al. The gut microbial metabolite formate exacerbates colorectal cancer progression. Nat Metab. 2022;4(4):458–475. doi:10.1038/s42255-022-00558-0.
- Ternes D, Karta J, Tsenkova M, Wilmes P, Haan S, Letellier E. Microbiome in colorectal cancer: how to get from meta-omics to mechanism? Trends Microbiol. 2020;28(5):401–423. doi:10.1016/j.tim.2020.01.001.
- Connors J, Dawe N, Van Limbergen J. The Role of Succinate in the Regulation of Intestinal Inflammation. Nutrients. 2018;11(1):25. doi:10.3390/nu11010025.
- Brennan CA, Clay SL, Lavoie SL, Bae S, Lang JK, Fonseca-Pereira D, Rosinski KG, Ou N, Glickman JN, Garrett WS. Fusobacterium nucleatum drives a pro-inflammatory intestinal microenvironment through metabolite receptor-dependent modulation of IL-17 expression. Gut Microbes. 2021;13(1):1987780. doi:10.1080/19490976.2021.1987780.
- Rubinstein MR, Wang X, Liu W, Hao Y, Cai G, Han YW. Fusobacterium nucleatum promotes colorectal carcinogenesis by modulating E-cadherin/β-catenin signaling via its FadA adhesin. Cell Host & Microbe. 2013;14(2):195–206. doi:10.1016/j.chom.2013.07.012.
- Kaplan CW, Ma X, Paranjpe A, Jewett A, Lux R, Kinder-Haake S, Shi W. Fusobacterium nucleatum outer membrane proteins Fap2 and RadD induce cell death in human lymphocytes. Infect Immun. 2010;78(11):4773–4778. doi:10.1128/iai.00567-10.
- Binder Gallimidi A, Fischman S, Revach B, Bulvik R, Maliutina A, Rubinstein AM, Nussbaum G, Elkin M. Periodontal pathogens Porphyromonas gingivalis and Fusobacterium nucleatum promote tumor progression in an oral-specific chemical carcinogenesis model. Oncotarget. 2015;6(26):22613–22623. doi:10.18632/oncotarget.4209.
- Vyhnalova T, Danek Z, Gachova D, Linhartova PB. The role of the oral microbiota in the etiopathogenesis of oral squamous cell carcinoma. Microorganisms. 2021;9(8):1549. doi:10.3390/microorganisms9081549.
- Metsäniitty M, Hasnat S, Salo T, Salem A. Oral microbiota—A new frontier in the pathogenesis and management of head and neck cancers. Cancers Basel. 2021;14(1):46. doi:10.3390/cancers14010046.
- Geng F, Zhang Y, Lu Z, Zhang S, Pan Y. Fusobacterium nucleatum caused DNA damage and promoted cell proliferation by the Ku70/p53 pathway in oral cancer cells. DNA Cell Biol. 2020;39(1):144–151. doi:10.1089/dna.2019.5064.
- Chen G, Gao C, Jiang S, Cai Q, Li R, Sun Q, Xiao C, Xu Y, Wu B, Zhou H. 2023. Fusobacterium nucleatum outer membrane vesicles activate autophagy to promote oral cancer metastasis. J Adv Res. doi:10.1016/j.jare.2023.04.002.
- Hsueh CY, Huang Q, Gong H, Shen Y, Sun J, Lau HC, Zhang D, Tang D, Wu C, Guo Y, et al. A positive feed-forward loop between Fusobacterium nucleatum and ethanol metabolism reprogramming drives laryngeal cancer progression and metastasis. iScience. 2022;25(2):103829. doi:10.1016/j.isci.2022.103829.
- Nejman D, Livyatan I, Fuks G, Gavert N, Zwang Y, Geller LT, Rotter-Maskowitz A, Weiser R, Mallel G, Gigi E, et al. The human tumor microbiome is composed of tumor type-specific intracellular bacteria. Sci. 2020;368(6494):973–980. doi:10.1126/science.aay9189.
- Liu Y, Baba Y, Ishimoto T, Tsutsuki H, Zhang T, Nomoto D, Okadome K, Yamamura K, Harada K, Eto K, et al. Fusobacterium nucleatum confers chemoresistance by modulating autophagy in oesophageal squamous cell carcinoma. Br J Cancer. 2021;124(5):963–974. doi:10.1038/s41416-020-01198-5.
- Wang X, Liu Y, Lu Y, Chen S, Xing Y, Yang H, Wang X, Zhang Y, Pan T, Li J, et al. Clinical impact of Fn-induced high expression of KIR2DL1 in CD8 T lymphocytes in oesophageal squamous cell carcinoma. Ann Med. 2022;54(1):51–62. doi:10.1080/07853890.2021.2016942.
- Zhang N, Liu Y, Yang H, Liang M, Wang X, Wang M, Kong J, Yuan X, Zhou F. Clinical Significance of Fusobacterium nucleatum Infection and Regulatory T Cell Enrichment in Esophageal Squamous Cell Carcinoma. Pathol Oncol Res. 2021;27:1609846. doi:10.3389/pore.2021.1609846.
- Kosumi K, Baba Y, Yamamura K, Nomoto D, Okadome K, Yagi T, Toihata T, Kiyozumi Y, Harada K, Eto K, et al. Intratumour Fusobacterium nucleatum and immune response to oesophageal cancer. Br J Cancer. 2023;128(6):1155–1165. doi:10.1038/s41416-022-02112-x.
- Park H, Clark E, Cullen JJ, Koland JG, Kim MS, Conklin JL. Expression of inducible nitric oxide synthase in the lower esophageal sphincter of the endotoxemic opossum. J Gastroenterol. 2002;37(12):1000–1004. doi:10.1007/s005350200169.
- Ding N, Cheng Y, Liu H, Wu Y, Weng Y, Cui H, Cheng C, Zhang W, Cui Y, Doucet-Populaire FC. Fusobacterium nucleatum infection induces malignant proliferation of esophageal squamous cell carcinoma cell by putrescine production. Microbiol Spectr. 2023;11(2):e0275922. doi:10.1128/spectrum.02759-22.
- Boehm ET, Thon C, Kupcinskas J, Steponaitiene R, Skieceviciene J, Canbay A, Malfertheiner P, Link A. Fusobacterium nucleatum is associated with worse prognosis in Lauren’s diffuse type gastric cancer patients. Sci Rep. 2020;10(1):16240. doi:10.1038/s41598-020-73448-8.
- Hsieh YY, Tung SY, Pan HY, Chang TS, Wei KL, Chen WM, Deng YF, Lu CK, Lai YH, Wu CS, et al. Fusobacterium nucleatum colonization is associated with decreased survival of helicobacter pylori-positive gastric cancer patients. World J Gastroenterol. 2021;27(42):7311–7323. doi:10.3748/wjg.v27.i42.7311.
- Zhou T, Meng X, Wang D, Fu W, Li X, Acharya A. Neutrophil Transcriptional Deregulation by the Periodontal Pathogen Fusobacterium nucleatum in Gastric Cancer: A Bioinformatic Study, Dis Markers 2022 (2022) 9584507. Dis Markers. 2022. 2022:1–10. doi:10.1155/2022/9584507.
- Baidoun F, Elshiwy K, Elkeraie Y, Merjaneh Z, Khoudari G, Sarmini MT, Gad M, Al-Husseini M, Saad A. Colorectal cancer epidemiology: recent trends and impact on outcomes. Curr Drug Targets. 2021;22(9):998–1009. doi:10.2174/18735592MTEx9NTk2y.
- Li R, Zhou R, Wang H, Li W, Pan M, Yao X, Zhan W, Yang S, Xu L, Ding Y, et al. Gut microbiota-stimulated cathepsin K secretion mediates TLR4-dependent M2 macrophage polarization and promotes tumor metastasis in colorectal cancer. Cell Death Differ. 2019;26(11):2447–2463. doi:10.1038/s41418-019-0312-y.
- Kashani N, Bezmin Abadi AT, Rahimi F, Forootan M. FadA-positive Fusobacterium nucleatum is prevalent in biopsy specimens of Iranian patients with colorectal cancer. New Microbes New Infect. 2020;34:100651. doi:10.1016/j.nmni.2020.100651.
- Xu Z, Zhou Z, Zhang J, Xuan F, Fan M, Zhou D, Liuyang Z, Ma X, Hong Y, Wang Y, et al. Targeting BMI-1-mediated epithelial-mesenchymal transition to inhibit colorectal cancer liver metastasis. Acta Pharm Sin B. 2021;11(5):1274–1285. doi:10.1016/j.apsb.2020.11.018.
- Sieminska I, Baran J, Myeloid-derived suppressor cells in colorectal cancer, Front Immunol 11 (2020) 1526. 10.3389/fimmu.2020.01526
- Al-Mterin MA, Elkord E. Myeloid-derived suppressor cells in colorectal cancer: prognostic biomarkers and therapeutic targets. Explor Target Antitumor Ther. 2022;3(4):497–510. doi:10.37349/etat.2022.00097.
- Wu Z, Ma Q, Guo Y, You F. 2022. The Role of Fusobacterium nucleatum in Colorectal Cancer Cell Proliferation and Migration. Cancers Basel. 1421: 10.3390/cancers14215350.
- Zhao P, Zhang Z. TNF-alpha promotes colon cancer cell migration and invasion by upregulating TROP-2. Oncol Lett. 2018;15(3):3820–3827. doi:10.3892/ol.2018.7735.
- Hayes CL, Dong J, Galipeau HJ, Jury J, McCarville J, Huang X, Wang XY, Naidoo A, Anbazhagan AN, Libertucci J, et al. Commensal microbiota induces colonic barrier structure and functions that contribute to homeostasis. Sci Rep. 2018;8(1):14184. doi:10.1038/s41598-018-32366-6.
- Loo TM, Kamachi F, Watanabe Y, Yoshimoto S, Kanda H, Arai Y, Nakajima-Takagi Y, Iwama A, Koga T, Sugimoto Y, et al. Gut microbiota promotes obesity-associated liver cancer through PGE(2)-mediated suppression of antitumor immunity. Cancer Discov. 2017;7(5):522–538. doi:10.1158/2159-8290.Cd-16-0932.
- Nosho K, Sukawa Y, Adachi Y, Ito M, Mitsuhashi K, Kurihara H, Kanno S, Yamamoto I, Ishigami K, Igarashi H, et al. Association of Fusobacterium nucleatum with immunity and molecular alterations in colorectal cancer. World J Gastroenterol. 2016;22(2):557–566. doi:10.3748/wjg.v22.i2.557.
- Fathi M, Pustokhina I, Kuznetsov SV, Khayrullin M, Hojjat-Farsangi M, Karpisheh V, Jalili A, Jadidi-Niaragh F. T-cell immunoglobulin and ITIM domain, as a potential immune checkpoint target for immunotherapy of colorectal cancer. Iubmb Life. 2021;73(5):726–738. doi:10.1002/iub.2461.
- Gur C, Maalouf N, Shhadeh A, Berhani O, Singer BB, Bachrach G, Mandelboim O. Fusobacterium nucleatum supresses anti-tumor immunity by activating CEACAM1. Oncoimmunology. 2019;8(6):e1581531. doi:10.1080/2162402x.2019.1581531.
- Yang Y, Weng W, Peng J, Hong L, Yang L, Toiyama Y, Gao R, Liu M, Yin M, Pan C, et al. Fusobacterium nucleatum increases proliferation of colorectal cancer cells and tumor development in mice by activating toll-like receptor 4 signaling to nuclear Factor−κB, and up-regulating expression of MicroRNA-21. Gastroenterology. 2017;152(4):851–866.e24. doi:10.1053/j.gastro.2016.11.018.
- Manfioletti G, Fedele M. Epithelial–mesenchymal transition (EMT) 2021. Int J Mol Sci. 2022;23(10):5848. doi:10.3390/ijms23105848.
- Xu C, Fan L, Lin Y, Shen W, Qi Y, Zhang Y, Chen Z, Wang L, Long Y, Hou T, et al. Fusobacterium nucleatum promotes colorectal cancer metastasis through miR-1322/CCL20 axis and M2 polarization. Gut Microbes. 2021;13(1):1980347. doi:10.1080/19490976.2021.1980347.
- Li H, Shen S, Chen X, Ren Z, Li Z, Yu Z. miR-450b-5p loss mediated KIF26B activation promoted hepatocellular carcinoma progression by activating PI3K/AKT pathway. Cancer Cell Int. 2019;19(1):205. doi:10.1186/s12935-019-0923-x.
- Gu S, Liang H, Qi D, Mao L, Mao G, Qian L, Zhang S. Knockdown of KIF26B inhibits breast cancer cell proliferation, migration, and invasion. Onco Targets Ther. 2018;11:3195–3203. doi:10.2147/ott.S163346.
- Basu AK. DNA damage, mutagenesis and cancer. Int J Mol Sci. 2018;19(4):970. doi:10.3390/ijms19040970.
- Liu Y, Li X, Zhang B, Fu Y, Yang A, Zhang H, Zhang H, Niu Y, Nie J, Yang J. CYP1A1 methylation mediates the effect of smoking and occupational polycyclic aromatic hydrocarbons co-exposure on oxidative DNA damage among Chinese coke-oven workers. Environ Health. 2019;18(1):69. doi:10.1186/s12940-019-0508-0.
- Huang R, Zhou PK. DNA damage repair: historical perspectives, mechanistic pathways and clinical translation for targeted cancer therapy. Signal Transduct Target Ther. 2021;6(1):254. doi:10.1038/s41392-021-00648-7.
- Okita Y, Koi M, Takeda K, Ross R, Mukherjee B, Koeppe E, Stoffel EM, Galanko JA, McCoy AN, Keku TO, et al. Fusobacterium nucleatum infection correlates with two types of microsatellite alterations in colorectal cancer and triggers DNA damage. Gut Pathog. 2020;12(1):46. doi:10.1186/s13099-020-00384-3.
- Qu R, Zhang Y, Ma Y, Zhou X, Sun L, Jiang C, Zhang Z, Fu W. Role of the gut microbiota and its metabolites in tumorigenesis or development of colorectal cancer. Adv Sci (Weinh). 2023;10(23):e2205563. doi:10.1002/advs.202205563.
- Najafi M, Farhood B, Mortezaee K. Cancer stem cells (CSCs) in cancer progression and therapy. J Cell Physiol. 2019;234(6):8381–8395. doi:10.1002/jcp.27740.
- Ternes D, Tsenkova M, Pozdeev VI, Meyers M, Koncina E, Atatri S, Schmitz M, Karta J, Schmoetten M, Heinken A, et al. The gut microbial metabolite formate exacerbates colorectal cancer progression. Nat Metabolism. 2022;4(4):458–475. doi:10.1038/s42255-022-00558-0.
- Guo P, Tian Z, Kong X, Yang L, Shan X, Dong B, Ding X, Jing X, Jiang C, Jiang N, et al. FadA promotes DNA damage and progression of Fusobacterium nucleatum-induced colorectal cancer through up-regulation of chk2. J Exp Clin Cancer Res. 2020;39(1):202. doi:10.1186/s13046-020-01677-w.
- Rubinstein MR, Baik JE, Lagana SM, Han RP, Raab WJ, Sahoo D, Dalerba P, Wang TC, Han YW. 2019. Fusobacterium nucleatum promotes colorectal cancer by inducing Wnt/β-catenin modulator Annexin A1. EMBO Rep. 204: 10.15252/embr.201847638.
- Ganesan K, Guo S, Fayyaz S, Zhang G, Xu B. Targeting programmed Fusobacterium nucleatum Fap2 for colorectal cancer therapy. Cancers Basel. 2019;11(10):1592. doi:10.3390/cancers11101592.
- Bray F, Ferlay J, Soerjomataram I, Siegel RL, Torre LA, Jemal A. Global cancer statistics 2018: GLOBOCAN estimates of incidence and mortality worldwide for 36 cancers in 185 countries. CA Cancer J Clin. 2018;68(6):394–424. doi:10.3322/caac.21492.
- Yang G, Yang Y, Tang H, Yang K. Loss of the clock gene Per1 promotes oral squamous cell carcinoma progression via the AKT/mTOR pathway. Cancer Sci. 2020;111(5):1542–1554. doi:10.1111/cas.14362.
- Blahak J, Zelinka J, Gumulec J, Machacek C, Danek Z, Bulik O. HPV, protein p16 and squamous cell carcinoma of the oral cavity. Biomed Pap Med Fac Univ Palacky Olomouc Czech Repub. 2020;164(3):292–299. doi:10.5507/bp.2019.026.
- Capote-Moreno A, Brabyn P, Muñoz-Guerra MF, Sastre-Pérez J, Escorial-Hernandez V, Rodríguez-Campo FJ, García T, Naval-Gías L. Oral squamous cell carcinoma: epidemiological study and risk factor assessment based on a 39-year series. Int J Oral Maxillofac Surg. 2020;49(12):1525–1534. doi:10.1016/j.ijom.2020.03.009.
- Mark Welch JL, Ramírez-Puebla ST, Borisy GG. Oral Microbiome Geography: Micron-Scale Habitat and Niche. Cell Host & Microbe. 2020;28(2):160–168. doi:10.1016/j.chom.2020.07.009.
- Mondal S, Adhikari N, Banerjee S, Amin SA, Jha T. Matrix metalloproteinase-9 (MMP-9) and its inhibitors in cancer: a minireview. Eur J Med Chem. 2020;194:112260. doi:10.1016/j.ejmech.2020.112260.
- Harrandah AM, Chukkapalli SS, Bhattacharyya I, Progulske-Fox A, Chan EKL. Fusobacteria modulate oral carcinogenesis and promote cancer progression. J Oral Microbiol. 2020;13(1):1849493. doi:10.1080/20002297.2020.1849493.
- Kelley N, Jeltema D, Duan Y, He Y. The NLRP3 inflammasome: an overview of mechanisms of activation and regulation. Int J Mol Sci. 2019;20(13):3328. doi:10.3390/ijms20133328.
- Chen G, Sun Q, Cai Q, Zhou H. Outer membrane vesicles from fusobacterium nucleatum switch M0-like macrophages toward the M1 phenotype to destroy periodontal tissues in mice. Front Microbiol. 2022;13:815638. doi:10.3389/fmicb.2022.815638.
- Dongre A, Weinberg RA. New insights into the mechanisms of epithelial-mesenchymal transition and implications for cancer. Nat Rev Mol Cell Biol. 2019;20(2):69–84. doi:10.1038/s41580-018-0080-4.
- Puram SV, Tirosh I, Parikh AS, Patel AP, Yizhak K, Gillespie S, Rodman C, Luo CL, Mroz EA, Emerick KS, et al. Single-cell transcriptomic analysis of primary and metastatic tumor ecosystems in head and neck cancer. Cell. 2017;171(7):1611–1624.e24. doi:10.1016/j.cell.2017.10.044.
- Ling Z, Cheng B, Tao X. Epithelial-to-mesenchymal transition in oral squamous cell carcinoma: challenges and opportunities. Int J Cancer. 2021;148(7):1548–1561. doi:10.1002/ijc.33352.
- Shao W, Fujiwara N, Mouri Y, Kisoda S, Yoshida K, Yoshida K, Yumoto H, Ozaki K, Ishimaru N, Kudo Y. Conversion from epithelial to partial-EMT phenotype by Fusobacterium nucleatum infection promotes invasion of oral cancer cells. Sci Rep. 2021;11(1):14943. doi:10.1038/s41598-021-94384-1.
- Stasiewicz M, Karpiński TM. The oral microbiota and its role in carcinogenesis. Semin Cancer Biol. 2022;86(Pt 3):633–642. doi:10.1016/j.semcancer.2021.11.002.
- Weng S, Ruan H. Multi-omics characterization of synthetic lethality-related molecular features: implications for SL-based therapeutic target screening. FEBS J. 2022;290(6):1477–1480. doi:10.1111/febs.16692.
- Huang J, Lok V, Ngai CH, Zhang L, Yuan J, Lao XQ, Ng K, Chong C, Zheng Z-J, Wong MCS. Worldwide burden of risk factors for, and trends in pancreatic cancer. Gastroenterology. 2021;160(3):744–754. doi:10.1053/j.gastro.2020.10.007.
- Cai J, Chen H, Lu M, Zhang Y, Lu B, You L, Zhang T, Dai M, Zhao Y. Advances in the epidemiology of pancreatic cancer: trends, risk factors, screening, and prognosis. Cancer Lett. 2021;520:1–11. doi:10.1016/j.canlet.2021.06.027.
- Klein AP. Pancreatic cancer epidemiology: understanding the role of lifestyle and inherited risk factors. Nat Rev Gastroenterol Hepatol. 2021;18(7):493–502. doi:10.1038/s41575-021-00457-x.
- Riquelme E, Zhang Y, Zhang L, Montiel M, Zoltan M, Dong W, Quesada P, Sahin I, Chandra V, San Lucas A, et al. Tumor microbiome diversity and composition influence pancreatic cancer outcomes. Cell. 2019;178(4):795–806.e12. doi:10.1016/j.cell.2019.07.008.
- Pushalkar S, Hundeyin M, Daley D, Zambirinis CP, Kurz E, Mishra A, Mohan N, Aykut B, Usyk M, Torres LE, et al. The pancreatic cancer microbiome promotes oncogenesis by induction of innate and adaptive immune suppression. Cancer Discov. 2018;8(4):403–416. doi:10.1158/2159-8290.Cd-17-1134.
- Udayasuryan B, Ahmad RN, Nguyen TTD, Umaña A, Monét Roberts L, Sobol P, Jones SD, Munson JM, Slade DJ, Verbridge SS. Fusobacterium nucleatum induces proliferation and migration in pancreatic cancer cells through host autocrine and paracrine signaling. Sci Signal. 2022;15(756):eabn4948. doi:10.1126/scisignal.abn4948.
- Hayashi M, Ikenaga N, Nakata K, Luo H, Zhong P, Date S, Oyama K, Higashijima N, Kubo A, Iwamoto C, et al. Intratumor Fusobacterium nucleatum promotes the progression of pancreatic cancer via the CXCL1-CXCR2 axis. Cancer Sci. 2023;114(9):3666–3678. doi:10.1111/cas.15901.
- Morgan E, Soerjomataram I, Rumgay H, Coleman HG, Thrift AP, Vignat J, Laversanne M, Ferlay J, Arnold M. The Global Landscape of Esophageal Squamous Cell Carcinoma and Esophageal Adenocarcinoma Incidence and Mortality in 2020 and Projections to 2040: New Estimates From GLOBOCAN 2020. Gastroenterology. 2022;163(3):649–658 e2. doi:10.1053/j.gastro.2022.05.054.
- Plaza-Diaz J, Alvarez-Mercado AI, Ruiz-Marin CM, Reina-Perez I, Perez-Alonso AJ, Sanchez-Andujar MB, Torne P, Gallart-Aragon T, Sanchez-Barron MT, Reyes Lartategui S, et al. Association of breast and gut microbiota dysbiosis and the risk of breast cancer: a case-control clinical study. BMC Cancer. 2019;19(1):495. doi:10.1186/s12885-019-5660-y.
- Gaba FI, Gonzalez RC, Martinez RG, Isola G. The role of oral Fusobacterium nucleatum in female breast cancer: a systematic review and meta-analysis. Int J Dent. 2022. 2022:1–12. doi:10.1155/2022/1876275.
- Wei MQ, Ellem KA, Dunn P, West MJ, Bai CX, Vogelstein B. Facultative or obligate anaerobic bacteria have the potential for multimodality therapy of solid tumours. Eur J Cancer. 2007;43(3):490–496. doi:10.1016/j.ejca.2006.10.005.
- Gillespie MR, Rai V, Agrawal S, Nandipati KC. 2021. The role of microbiota in the pathogenesis of esophageal adenocarcinoma. Biology (Basel). 108: 10.3390/biology10080697.
- Muszynski D, Kudra A, Sobocki BK, Folwarski M, Vitale E, Filetti V, Dudzic W, Kazmierczak-Siedlecka K, Polom K. Esophageal cancer and bacterial part of gut microbiota - a multidisciplinary point of view. Front Cell Infect Microbiol. 2022;12:1057668. doi:10.3389/fcimb.2022.1057668.
- Li Z, Shi C, Zheng J, Guo Y, Fan T, Zhao H, Jian D, Cheng X, Tang H, Ma J. Fusobacterium nucleatum predicts a high risk of metastasis for esophageal squamous cell carcinoma. BMC Microbiol. 2021;21(1):301. doi:10.1186/s12866-021-02352-6.
- Machlowska J, Baj J, Sitarz M, Maciejewski R, Sitarz R. Gastric cancer: epidemiology, risk factors, classification, genomic characteristics and treatment strategies. Int J Mol Sci. 2020;21(11):4012. doi:10.3390/ijms21114012.
- Stewart OA, Wu F, Chen Y. The role of gastric microbiota in gastric cancer. Gut Microbes. 2020;11(5):1220–1230. doi:10.1080/19490976.2020.1762520.
- Wang L, Zhou J, Xin Y, Geng C, Tian Z, Yu X, Dong Q. Bacterial overgrowth and diversification of microbiota in gastric cancer. Eur J Gastroenterol Hepatol. 2016;28(3):261–266. doi:10.1097/MEG.0000000000000542.
- Liatsos C, Papaefthymiou A, Kyriakos N, Galanopoulos M, Doulberis M, Giakoumis M, Petridou E, Mavrogiannis C, Rokkas T, Kountouras J. Helicobacter pylori, gastric microbiota and gastric cancer relationship: unrolling the tangle. World J Gastrointest Oncol. 2022;14(5):959–972. doi:10.4251/wjgo.v14.i5.959.
- Rubinstein MR, Wang X, Liu W, Hao Y, Cai G, Han YW. Fusobacterium nucleatum promotes colorectal carcinogenesis by modulating E-cadherin/beta-catenin signaling via its FadA adhesin. Cell Host & Microbe. 2013;14(2):195–206. doi:10.1016/j.chom.2013.07.012.
- Xin Y, Li X, Zhang M, Shang Z, Luo Z, Wang Y, Gui X, Liu Q, Li T, Zeng S, et al. Fusobacterium nucleatum-induced exosomal HOTTIP promotes gastric cancer progression through the microRNA-885-3p/EphB2 axis. Cancer Sci. 2023;114(6):2360–2374. doi:10.1111/cas.15781.
- Jiang S-S, Xie Y-L, Xiao X-Y, Kang Z-R, Lin X-L, Zhang L, Li C-S, Qian Y, Xu P-P, Leng X-X, et al. Fusobacterium nucleatum-derived succinic acid induces tumor resistance to immunotherapy in colorectal cancer. Cell Host & Microbe. 2023;31(5):781–797.e9. doi:10.1016/j.chom.2023.04.010.
- LaCourse KD, Zepeda-Rivera M, Kempchinsky AG, Baryiames A, Minot SS, Johnston CD, Bullman S. The cancer chemotherapeutic 5-fluorouracil is a potent Fusobacterium nucleatum inhibitor and its activity is modified by intratumoral microbiota. Cell Rep. 2022;41(7):111625. doi:10.1016/j.celrep.2022.111625.
- Yu T, Guo F, Yu Y, Sun T, Ma D, Han J, Qian Y, Kryczek I, Sun D, Nagarsheth N, et al. Fusobacterium nucleatum promotes chemoresistance to colorectal cancer by modulating autophagy. Cell. 2017;170(3):548–563.e16. doi:10.1016/j.cell.2017.07.008.
- Alon-Maimon T, Mandelboim O, Bachrach G. Fusobacterium nucleatum and cancer. Periodontology. 2022;89(1):166–180. doi:10.1111/prd.12426.
- Liang M, Liu Y, Zhang Z, Yang H, Dai N, Zhang N, Sun W, Guo Y, Kong J, Wang X, et al. Fusobacterium nucleatum induces MDSCs enrichment via activation the NLRP3 inflammosome in ESCC cells, leading to cisplatin resistance. Ann Med. 2022;54(1):989–1003. doi:10.1080/07853890.2022.2061045.
- Zhang J-W, Zhang D, Yin H-S, Zhang H, Hong K-Q, Yuan J-P, Yu B-P. Fusobacterium nucleatum promotes esophageal squamous cell carcinoma progression and chemoresistance by enhancing the secretion of chemotherapy-induced senescence-associated secretory phenotype via activation of DNA damage response pathway. Gut Microbes. 2023;15(1):2197836. doi:10.1080/19490976.2023.2197836.
- Da J, Wang X, Li L, Xu Y. Fusobacterium nucleatum promotes cisplatin-resistance and migration of oral squamous carcinoma cells by up-regulating Wnt5a-mediated NFATc3 expression. Tohoku J Exp Med. 2021;253(4):249–259. doi:10.1620/tjem.253.249.
- Liu K, Yang X, Zeng M, Yuan Y, Sun J, He P, Sun J, Xie Q, Chang X, Zhang S, et al. The role of fecal Fusobacterium nucleatum and pks+ Escherichia coli as early diagnostic markers of colorectal cancer, disease markers 2021. Dis Markers. 2021. 2021:1–11. doi:10.1155/2021/1171239.
- Kurt M, Yumuk Z, Diagnostic accuracy of Fusobacterium nucleatum IgA and IgG ELISA test in colorectal cancer, Sci Rep 11 (1) (2021) 1608. 10.1038/s41598-021-81171-1
- Baik JE, Li L, Shah MA, Freedberg DE, Jin Z, Wang TC, Han YW. Circulating IgA antibodies against Fusobacterium nucleatum amyloid adhesin FadA are a potential biomarker for colorectal neoplasia. Cancer Res Commun. 2022;2(11):1497–1503. doi:10.1158/2767-9764.Crc-22-0248.
- Chattopadhyay I, Verma M, Panda M. Role of Oral Microbiome Signatures in Diagnosis and Prognosis of Oral Cancer. Technol Cancer Res Treat. 2019;18:1533033819867354. doi:10.1177/1533033819867354.
- Chen Z, Wong PY, Ng CWK, Lan L, Fung S, Li JW, Cai L, Lei P, Mou Q, Wong SH, et al. The intersection between oral microbiota, host gene methylation and patient outcomes in head and neck squamous cell carcinoma. Cancers. 2020;12(11):3425. doi:10.3390/cancers12113425.
- Chen WD, Zhang X, Zhang MJ, Zhang YP, Shang ZQ, Xin YW, Zhang Y. Salivary Fusobacterium nucleatum serves as a potential diagnostic biomarker for gastric cancer. World J Gastroenterol. 2022;28(30):4120–4132. doi:10.3748/wjg.v28.i30.4120.
- Ting NL-N, Lau HC-H, Yu J. Cancer pharmacomicrobiomics: targeting microbiota to optimise cancer therapy outcomes. Gut. 2022;71(7):1412–1425. doi:10.1136/gutjnl-2021-326264.
- Jia F, Yu Q, Wang R, Zhao L, Yuan F, Guo H, Shen Y, He F. Optimized Antimicrobial Peptide Jelleine-I Derivative Br-J-I Inhibits Fusobacterium Nucleatum to Suppress Colorectal Cancer Progression. Int J Mol Sci. 2023;24(2):1469. doi:10.3390/ijms24021469.
- Van der Merwe M, Van Niekerk G, Botha A, Engelbrecht A-M. The onco-immunological implications of Fusobacterium nucleatum in breast cancer. Immunol Lett. 2021. 232:60–66. doi:10.1016/j.imlet.2021.02.007.
- Yamaoka Y, Suehiro Y, Hashimoto S, Hoshida T, Fujimoto M, Watanabe M, Imanaga D, Sakai K, Matsumoto T, Nishioka M, et al. Fusobacterium nucleatum as a prognostic marker of colorectal cancer in a Japanese population. J Gastroenterol. 2018;53(4):517–524. doi:10.1007/s00535-017-1382-6.
- Kunzmann AT, Proença MA, Jordao HW, Jiraskova K, Schneiderova M, Levy M, Liska V, Buchler T, Vodickova L, Vymetalkova V, et al. Fusobacterium nucleatum tumor DNA levels are associated with survival in colorectal cancer patients. Eur J Clin Microbiol Infect Dis. 2019;38(10):1891–1899. doi:10.1007/s10096-019-03649-1.
- Mima K, Nishihara R, Qian ZR, Cao Y, Sukawa Y, Nowak JA, Yang J, Dou R, Masugi Y, Song M, et al., Fusobacterium nucleatum in colorectal carcinoma tissue and patient prognosis, Gut 65 (12) (2016) 1973–1980. 10.1136/gutjnl-2015-310101
- Lee JB, Kim KA, Cho HY, Kim D, Kim WK, Yong D, Lee H, Yoon SS, Han DH, Han YD, et al. Association between Fusobacterium nucleatum and patient prognosis in metastatic colon cancer. Sci Rep. 2021;11(1):20263. doi:10.1038/s41598-021-98941-6.
- Mitsuhashi K, Nosho K, Sukawa Y, Matsunaga Y, Ito M, Kurihara H, Kanno S, Igarashi H, Naito T, Adachi Y, et al. Association of Fusobacterium species in pancreatic cancer tissues with molecular features and prognosis. Oncotarget. 2015;6(9):7209–7220. doi:10.18632/oncotarget.3109.
- Zhang N, Kandalai S, Zhou X, Hossain F, Zheng Q. Applying multi-omics toward tumor microbiome research. iMeta. 2023;2(1):e73. doi:10.1002/imt2.73.