ABSTRACT
Calorie restriction (CR) extends lifespan from yeast to mammals. SIRT6 is a member of the sirtuin family of NAD+-dependent histone deacetylases, which is responsible for mediating the effects of CR. The transcription factor NF-κB, which is involved in inflammation and aging, has been shown to be regulated by SIRT6. Here we describe the crucial role of SIRT6 in aging and inflammation. We show that CR had improved renal insufficiency and enhanced SIRT6 expression after 6-month treatment in aged mice. Culture cells in low glucose (LG) conditions also showed resistance to cell senescence and enhanced SIRT6 expression compared to normal glucose (NG) group, showing beneficial effects of the CR-mimic cultural conditions. Moreover, SIRT6 overexpression is sufficient to delay the replicative senescence of WI38 by attenuating NF-κB signaling, while SIRT6 knockdown results in accelerated cell senescence and overactive NF-κB signaling. These findings confirm the key status of CR and disclose the critical role of SIRT6 on aging and inflammation.
Introduction
Aging is the major risk factor for multiple diseases, including diabetes, cancer, renal pathologies, etc.Citation1 Calorie restriction (CR), a dietary regimen, exhibits various beneficial effects on health, including lifespan extension, organism functional improvement and cell senescence retardation.Citation2,3 However, the mechanism of CR remains unclear.
The silent information regulator 2 (SIR2) is one of the critical mediators of CR-induced lifespan extension in yeast.Citation4 In mammalian genomes, 7 homologues of yeast Sir2, SIRT1–7, have been identified.Citation5 Among them SIRT1 is the mostly studied member and it has been reported to be activated by resveratrol and play a pivotal role in CR-mediated lifespan regulation.Citation6,7 SIRT6 is another key member of the mammalian sirtuin family and it has been shown to control metabolism, DNA damage repair and aging.Citation8 SIRT6 functions as a NAD+-dependent deacetylaseCitation9 and ADP ribosyltransferase.Citation10 SIRT6-deficient mice develop severe genomic instability and metabolic defects,Citation11 while the SIRT6 transgenic mice are long-lived,Citation12 particularly via suppression of NF-κB signaling. However, whether SIRT6 can mediate beneficial effects of CR is unknown.
NF-κB, the nuclear transcription factor, is associated with inflammatory responses, aging and apoptosis.Citation13,14 Activated NF-κB translocation into the nucleus is associated with age-related pathologies.Citation15 Previous studies showed that the transcriptional activity of NF-κB is regulated by SIRT6. SIRT6 can suppress the NF-κB activity by deacetylating the histone H3K9 of NF-κB dependent genesCitation16 as well as the Lys 310 (K310) of NF-κB p65 (RelA) subunit.Citation17
In this study, we show that CR prevents age-dependent renal insufficiency and cell senescence by up-regulation of SIRT6. CR-triggered SIRT6 activation suppresses NF-κB signaling via deacetylation and preventing nuclear translocation of NF-κB. Here we identified the beneficial effects of CR and determined the crucial role of SIRT6 on lifespan regulation.
Results
CR enhances SIRT6 expression and delays age-dependent renal degeneration
To study the effects of CR on aging, 24-month old mice were fed under either Ad libitum (AL) or CR diet for 6 months. We took kidney into this investigation to test typical histologic features of organ senescence. We applied Haematoxylin-eosin (HE) staining, Periodic acid-Schiff (PAS) staining, Masson's trichrome staining and Senescent-associated β-gal (SA-β-gal) assay to evaluate age-dependent renal degeneration. First, we used HE staining to test renal histomorphology. Significantly higher number of dilated glomerulus and swelling renal tubule epithelium in AL group were observed, which are well known hallmarks of renal aging,Citation18 comparing to CR group (). PAS staining is used to detect accumulation of saccharides (polysaccharides, glycoproteins and glycolipids) in tissues.Citation19 We found that reduced amount of saccharides deposits were detected in CR group, indicated the reduced amount of renal damage ().Citation20 We also used Masson's trichrome staining to detect severity of renal fibrosis and our results displayed reduced tubulointerstitial fibrosis in CR group compared to AL group (). SA-β-gal assay is an effective method to measure cell senescenceCitation21 and significantly fewer SA-β-gal positive cells were observed in CR conditions ().
Figure 1. CR enhances SIRT6 expression and improves renal pathologies. (a) Aged male mice were fed under either Ad libitum (AL, n = 10) or caloric restriction (CR, n = 7) diet for 6 months. HE staining (×200) was applied to test renal histomorphology. (b) PAS staining (×200) was used to determine saccharides level. (c) Masson staining (×400) were applied to detect severity of renal fibrosis. (d) SA-β-gal assay (×200) was used to test the expression of senescence-associated β-galactosidase. (e) The level of renal tumor necrosis factor-α (TNF-α) and interleukin-1β (IL-1β) were measured by ELISA in both groups. (f) Immunohistochemistry (×200) and (g) Western blot analysis was applied to measure SIRT6 expression in mice kidney under AL or CR diet. *p < 0.05.
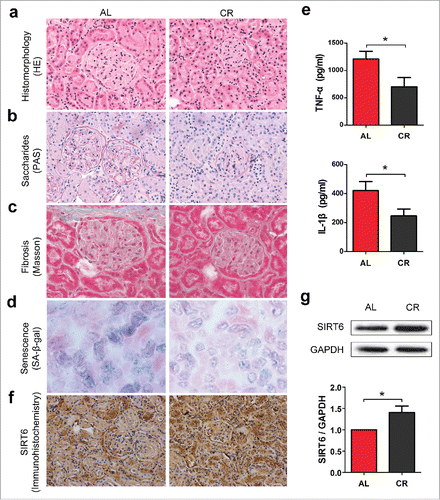
Aging is associated with increased inflammation.Citation22 To test whether CR can play anti-inflammatory effects in the process of aging, we measured the level of tumor necrosis factor-α (TNF-α) and interleukin-1β (IL-1β), 2 proinflammatory cytokines that play major roles in coordinating the inflammatory response, by enzyme-linked immunosorbent assay (ELISA). Compared with AL group, reduced production of renal TNF-α and IL-1β were observed in mice subjected to CR (), indicated that CR can reduce renal inflammatory activity effectively.
It has been shown that SIRT6 overexpression can prolong mouse lifespan.Citation12 To test whether SIRT6 is involved in renal aging, we stained SIRT6 in AL and CR mice and compared its level by immunohistochemistry and Western blot analysis. SIRT6 is a nuclear-localized protein.Citation11,23 Immunohistochemistry () and Western blot analysis () of SIRT6 indicated enhanced SIRT6 expression in CR condition. Our results strongly supports the evidence that CR can effectively prevent age-dependent renal failure and promotes in vivo SIRT6 expression.
Calorie restriction retards replicative senescence in human fibroblast WI38
It has been reported that culturing skeletal myoblastsCitation24 and human diploid fibroblastsCitation25 in low glucose (LG) medium strongly activates SIRT1 and SIRT3 expression, respectively, mimicking the effect of CR in vitro. To test whether SIRT6 expression can be stimulated under CR conditions, we cultured commonly-used human diploid fibroblast WI38 in LG or normal glucose (NG) condition. We found that culturing WI38 cells in LG condition effectively suppressed cell senescence measured by calculation of SA-β-gal positive cells, compared to NG group ().
Figure 2. Calorie restriction activates SIRT6 expression and prolongs WI38 lifespan. (a) WI38 cells were grown under normal glucose (NG) or Low glucose (LG) condition. Percentage of SA-β-gal positive cells were calculated to measure proportion of senescent cells. (b) Population doubling level (PDL) calculation was conducted by cell counting at each passage. (c) Cell cycle profiles of WI38 were analyzed at early and late stage of cell culture. (d) Western blot analysis of SIRT6 and p16INK4A expression in NG or LG condition, normalized to GAPDH. *p < 0.05, **p < 0.01 (n = 3).
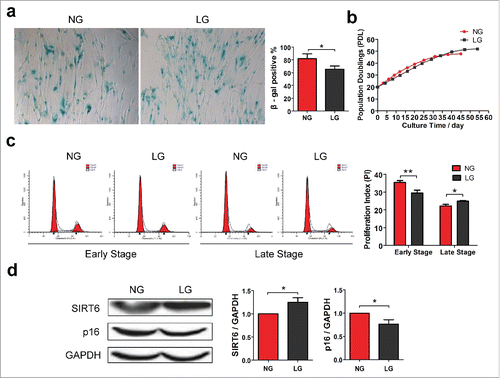
We also monitored WI38 lifespan by counting cells at each passage according to the calculation method of population doubling level (PDL) from ATCC. The proliferation of WI38 cells in NG group was increased at early passage, while the replication ability was gradually lost thereafter. After 45 d of cell culture, WI38 from NG group reached their Hayflick limitCitation26 with a finite PDL number of about 48. In contrast, cells in LG group had lower proliferation rate compared to NG group, while the proliferation ability was extended to 54 d with a finite PDL number of about 52 ().
Cell cycle distributions were determined by flow cytometry (FCM) at both early culture stage (the 20th day) and late culture stage (the 40th day), respectively. In eukaryotes, cell cycle can be divided into 5 periods: Gap 0 (G0), quiescent phase. Gap 1 (G1), the checkpoint for DNA synthesis. Synthesis (S), DNA replication. Gap 2 (G2), the checkpoint for mitosis. Mitosis (M), cell division.Citation27 We utilized the proliferation index (PI) = (S + G2/M) / (G0/G1 + S + G2/M) to measure their proliferation ability. Cell cycle profiles indicated that at the early stage of cell culture, cells under LG condition grew with lower population doubling rate, while during the late stage of cell culture, LG group revealed preserved ability of cell proliferation whereas NG group showed growth retardation ().
We also compared the levels of p16INK4A, a commonly used biomarker of cell senescence which has been confirmed a significantly increase with aging.Citation28,29 Culturing cells in LG conditions, significantly reduced the levels of p16INK4A expression, further confirming suppression of cell senescence. We further investigated the effect of CR on SIRT6 expression and found that SIRT6 expression was enhanced in LG conditions, compared with NG group. These results indicate the beneficial effects of calorie restriction on cell lifespan and potential involvement of SIRT6 in the CR-mediated senescence retardation.
Effects of SIRT6 in delaying cell senescence
Because level of SIRT6 changes according to different caloric conditions, we wondered whether the regulation of SIRT6 can affect cell senescence. First, we stably overexpressed wild-type SIRT6 (SIRT6-WT) or the catalytic H133Y SIRT6 mutant protein (SIRT6-HY, His133 → Tyr)Citation9 in WI38 (). Both wild-type and mutant SIRT6 exhibited comparable expression of SIRT6. Using SA-β-gal staining, reduced number of SA-β-gal positive cells were observed in WI38 with SIRT6-WT overexpression, but not in cells with SIRT6-HY overexpression (). Moreover, SIRT6 overexpression resulted in reduced p16INK4A expression, while the dominant negative SIRT6-HY overexpression caused slightly enhanced p16INK4A expression, although it wasn't statistical significant (). Our data indicate that SIRT6 overexpression is sufficient to delay cell senescence.
Figure 3. SIRT6 overexpression inhibits cell senescence. (a) Western blot analysis of SIRT6 in pcDNA3.1, wild-type SIRT6 (SIRT6-WT) or catalytic H133Y mutant SIRT6 (SIRT6-HY) stably overexpressed WI38 cells. (b) SA-β-gal assay indicated the rate of SA-β-gal positive cells. Positive rates were calculated by random fields' positive cells counting. (c) Western blot analysis of SIRT6 and p16INK4A in pcDNA3.1, SIRT6-WT or SIRT6-HY overexpressed WI38. *p < 0.05 (n = 3).
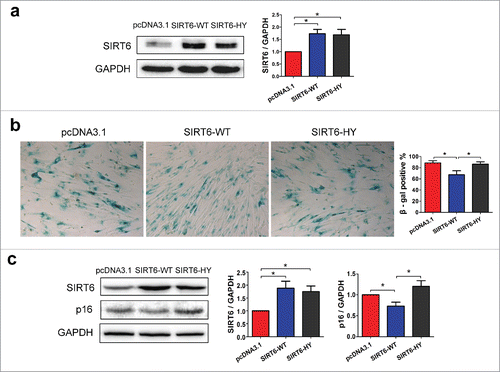
To further confirm the effects of SIRT6 on aging, we stably transfected WI38 cells with SIRT6 shRNAs. To avoid the off-target effects, 2 independent shRNAs were used to stably knockdown SIRT6 in WI38 and cells transfected with pSUPER.retro.puro (pSR) empty vectors were used as control. The knockdown efficiency was confirmed by Western blot analysis. Both shRNAs resulted in significantly decrease of the level of SIRT6 comparing to cells infected with empty vector ().
Figure 4. SIRT6 knockdown accelerates cell senescence. (a) Western blot analysis of SIRT6 in pSR (control) and SIRT6-shRNAs transfected WI38. (b) SA-β-gal assay indicated the number of SA-β-gal positive cells in the 3 groups. Positive rates were calculated by random fields' positive cells counting. (c) Western blot analysis of SIRT6 and p16INK4A in control and SIRT6-knockdown WI38. *p < 0.05 (n = 3).
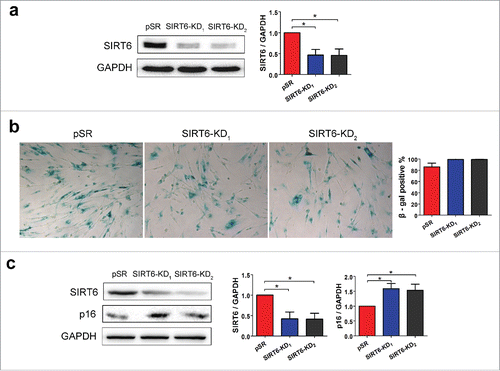
To test whether downregulation of SIRT6 can induce cells senescence we stained WI38 cells with SA-β-gal. Silencing of SIRT6 increased the percentage of SA-β-gal positive cells, comparing to cells transfected with empty vector (). In addition, SIRT6 knockdown resulted in appearance of random arranged cell clusters which exhibit the well-known fibroblast senescence hallmarks. In addition, downregulation of SIRT6 strongly upregulated expression of p16INK4A (), further confirming acceleration of cell senescence when the level of SIRT6 is decreased.
As cell replicative senescence is strongly linked to cell cycle and SIRT6 is of great importance in the process of cell senescence. So, we wandered whether SIRT6 can play a role in cell cycle regulation. We built SIRT6 transient overexpression or knockdown 293FT cells to determine whether the change of SIRT6 expression can affect the cell cycle (Fig. S1). Cell cycle was determined by flow cytometry (FCM) 24 hours after transfection. However no discernible effect was detected from SIRT6 enhanced or depleted cells indicated that SIRT6 expression had no significant effect on cell cycle. Our data indicate the pivotal role of SIRT6 in delaying cell senescence and suggested that the regulation of SIRT6 on longevity is cell cycle independent.
SIRT6 interacts with NF-κB to regulate inflammation and apoptosis
NF-κB is involved in aging and inflammatory response.Citation30 It has been reported that SIRT6 overexpression can effect osteogenesis by regulating the expression of Ac-NF-κB p65 (K310).Citation17 Here we tested whether SIRT6 can promote K310 deacetylation of NF-κB p65 in WI38 cells and affect cell senescence.
Expression of SIRT6-WT strongly decreased the level of Ac-NF-κB while without changing the level of total NF-κB, compared with transfection of empty pcDNA3.1 vector or SIRT6-HY (). Our results strongly indicate that SIRT6 overexpression deacetylates K310 of NF-κB p65 subunit.
Figure 5. SIRT6 overexpression attenuates NF-κB pathway. (a) Western blot analysis of K310 Ac-NF-κB p65 and total NF-κB p65 in pcDNA3.1, SIRT6-WT or SIRT6-HY overexpressed WI38. (b) Confocal microscopy showed the distribution and expression of SIRT6 and NF-κB in the 3 groups. Green, SIRT6. Red, NF-κB p65. Blue, DAPI. *p < 0.05, **p < 0.01 (n = 3).
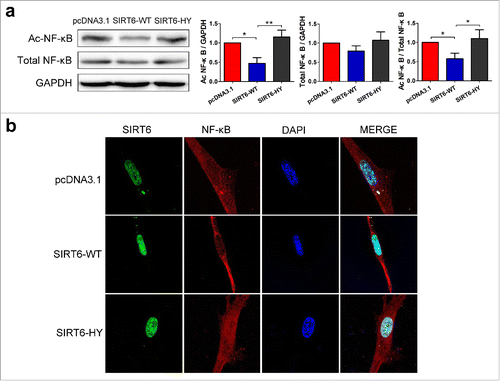
Confocal microscopy was conducted to detect the distribution and expression of SIRT6 and NF-κB. The SIRT6-WT overexpressed cells were observed in a younger cell state with elongated cell body and a relatively small nucleus, compared with other groups (). Most importantly, NF-κB are most distributed in the cytoplasm in the SIRT6-WT overexpressed cells, which means an attenuation of NF-κB signaling and a suppression of inflammation and senescence. While, the SIRT6-HY group even showed negative effects with more NF-κB translocated into the nucleus than the control group. Results demonstrated that SIRT6 overexpression is sufficient to delay cell senescence and attenuate NF-κB signaling.
To further confirm the effects of SIRT6 on age-related inflammatory response, we determined NF-κB signaling in SIRT6-deficient WI38. Downregulation of SIRT6 with different shRNAs led to a strong accumulation of acetylated NF-κB p65 but did not affect the level of total NF-κB p65 comparing to the cells with expression of empty vector, which confirmed the hyperacetylation of NF-κB in SIRT6-deficient WI38 (). To further prove that loss of SIRT6 causes NF-κB activation, we monitored subcellular localization of NF-κB p65. Compared with empty vector, SIRT6 knockdown induced the translocation of NF-κB p65 into the nucleus along with enlarged cell bodies, indicated an activation of the NF-κB signaling and accelerated cell senescence ().
Figure 6. SIRT6 knockdown activates NF-κB. (a) Western blot analysis of K310 Ac-NF-κB p65 and total NF-κB p65 in pSR and SIRT6-shRNAs transfected WI38. (b) Confocal microscopy showed the distribution and expression of SIRT6 and NF-κB p65 in 3 groups. Green, SIRT6. Red, NF-κB p65. Blue, DAPI. (c) The mechanism of how SIRT6 mediates NF-κB signaling pathway. *p < 0.05 (n = 3).
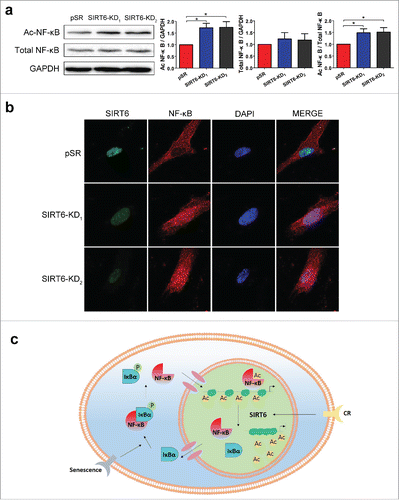
As the activity of NF-κB is affected by SIRT6, and NF-κB have been reported to be associated with increased resistance to apoptosis.Citation31 So we wandered whether SIRT6 can affect the process of apoptosis. In our research, 293FT cells were transiently transfected with pcDNA3.1, SIRT6-WT, SIRT6-HY, pSR, SIRT6-KD1 and SIRT6-KD2 plasmids respectively and induced apoptosis by 200 mmol/L H2O2 for 2 hours. Apoptosis was evaluated by FCM (Fig. S2). Horizontal axis stood for AnnexinV-FITC and vertical axis represented PI. Q1, AnnexinV-FITC−/PI+: necrotic cells. Q2, AnnexinV-FITC+/PI+: late apoptotic cells. Q3, AnnexinV-FITC+/PI−: early apoptotic cells. Q4, AnnexinV-FITC−/PI−: live cells. We took Q2+Q3 as apoptotic rate. In our results, lower apoptotic rate were detected in SIRT6 depleted cells, indicated that SIRT6 depletion elevated apoptosis resistance.
Our data provide more evidence toward the beneficail effects of CR-induced SIRT6 activtion and demonstrate that level of SIRT6 can affect NF-κB signaling via its translocation into nuclei and deacetylation, then influence inflammation, cell senescencen, and apoptosis.
Discussion
Nowadays, investigations are making efforts to elucidate the mechanisms of aging to treat age-associated diseases and promote healthy aging.Citation32 In this study, we demonstrated that the activation of SIRT6 by CR can improve the renal pathological characterizations and retard cell senescence through the attenuation of NF-κB pathway.
CR can prevent renal aging even in an old age
CR, an effective way to prevent energy excess,Citation33 has been reported to extend lifespan in diverse species including yeast, nematodes, flies and mammals.Citation4,34 Encouraging results are also achieved in Rhesus Monkeys, the close relatives to humans, in which lower age-related deaths and retardant age-associated pathologies onset were observed with CR application.Citation35 In this study, we conducted the experiment with 24-month old mice which already entered aging stage and fed them with either AL or CR diet for 6 months. These findings confirmed that CR can protect against aging even if started in an old age. Moreover, we took kidney into focus as kidney is one of the vital organs which is closely associated with aging process. This study provides more evidence toward the effects of CR on specific organ, which is rarely reported.
SIRT6 and NF-κB interaction
NF-κB plays key roles in the regulation of immune, inflammatory responses and aging.Citation13 In resting cells, the NF-κB complexes are bounded by the inhibitory protein IκB and present in cytoplasm in an inactive state.Citation36 Upon stimulation, the IκB is phosphorylated by kinases, ubiquitinated and degraded. The released NF-κB p50-p65 subunits then translocate into the nucleus and are acetylated to activate the transcription of their target genes ().
In this study, we took the method of confocal microscopy to show the cellular localization of NF-κB in SIRT6 enrichment or depleted cells, which visual displayed the effect of SIRT6 on NF-κB nuclear translocation. However, we cannot make sure whether the altered expression of SIRT6 is the direct driver of NF-κB cytoplasm - nucleus translocation. SIRT6 can target several key longevity routes and metabolism related proteins such as the IGF-Akt signaling,Citation37 AP-1,Citation38 Hif1αCitation39 and mTOR.Citation40 In this way, the alteration of SIRT6 expression may result in a synergistic action of several effectors to regulate cell state corporately, resulting in the translocation of NF-κB.
SIRT6 regulates lifespan in a cell cycle-independent manner
The effect of sirtuins on cell cycle profiles is rarely reported. Among the 7 mammalian homologs of the yeast Sir2 deacetylase, SIRT2 is the only one reported to have a role in the mitotic checkpoint function and therefore regulate cell cycle.Citation41 So, we examined that whether SIRT6 is involved in cell cycle regulation and founded out that SIRT6 regulates cell senescence in a cell cycle-independent manner.
It is not difficult to understand this conclusion. As the role of SIRT6 is quite complex, SIRT6 is not only involved in several key singnaling pathways but also associated with the function of base excision DNA repair (BER)Citation11 and telomere maintenance,Citation9 which all play pivotal roles in cellular homeostasis maintenance and senescence prevention. That is to say, there are multiple ways for SIRT6 to play the anti-aging role except participate in cell cycle regulation.
Effect of SIRT6 on apoptosis is controversial
Previous study show that the knockdown of SIRT6 resulted in the induction of antiapoptotic genes and SIRT6 depleted Hela cells were more resistant to apoptosis induced by FADD.Citation16 In addition, it has been reported that overexpression of SIRT6 induces apoptosis in cancer cell but not in non-transformed cell lines,Citation42 which may help to explore a new methods toward cancer therapy. However, there are also studies indicate that depletion of SIRT6 has no major effect on the induction of apoptotic cell death.Citation43
In this study, we detected apoptosis resistance in SIRT6 depleted cells and provided more evidence toward the effects of SIRT6 on apoptosis. Unfortunately, subsequent apoptosis associated test, such as Hoechst staining and Caspase 3 protein expression detection did not show consistent results (data not shown), leaving disputations toward the effect of SIRT6 on apoptosis. More work will be done on this subject in further research.
In summary, our study reveals the beneficial effects of CR-induced SIRT6 activation and provides more evidences toward the molecular mechanism of SIRT6-mediated NF-κB regulation. This study may provide a new therapeutic target to resist cellular dysfunction and retard aging associated diseases.
Materials and methods
Animals and treatment
Male C57BL/6 mice were obtained from the experimental animal facility of Tsinghua University, Beijing, China. Eight-week-old mice were housed under pathogen free conditions, 12/12 light/dark cycle for 22 months then divided into 2 groups. One group was fed under AL condition (control), another group were provided with 70% of the food consumed by AL group as a caloric restriction for 6 months, then sacrificed. Experiments were carried out in accordance with the approved guidelines and with the approval of the Institutional Ethical Committee of China.
Pathological section staining
The kidneys were fixed in 4% buffered paraformaldehyde. For Periodic acid-Schiff staining (Sigma, St. Louis, MO, USA) and Masson's trichrome staining (Sigma), samples were embedded in paraffin. For senescent-associated β-gal assay (Sigma), samples were OCT-embedded. Experiments were conducted according to the standard protocol.
ELISA
The kidneys (100 mg/group) were homogenized in 1 ml PBS and stored overnight at −20°C After 2 freeze-thaw cycles to break the cell membranes, the homogenates were centrifuged and the supernates were removed and assayed immediately. Experiments were conducted according to the ELISA Kit (Cusabio, Wuhan, China).
Immunohistochemical analysis
Immunohistochemical analysis was conducted according to the IHC Kit (Maxim, Fujian, China). SIRT6 antibody (Cell Signaling Technology, Danvers, MA, USA) was used as a primary antibody.
Western blot analysis
Proteins were extracted from tissues or cells by RIPA lysis buffer (Beyotime Biotechnology, Shanghai, China) then the concentration was calculated by BCA method.Citation44 Antibodies were purchased from the indicated sources: SIRT6 (Cell Signaling Technology), NF-κB p65 (Cell Signaling Technology), NF-κB p65 acetyl K310 (Abcam, Cambridge, UK), p16 (Santa Cruz Biotechnology, Santa Cruz, CA, USA), GAPDH (Santa Cruz Biotechnology), Goat anti-rabbit IgG (Santa Cruz Biotechnology). Experiments were carried out according to standard protocol. Images were quantified by ImageJ software.
Cell culture and plasmid transfection
WI-38 and 293-FT cells (ATCC) were maintained in DMEM (Corning, Manassas, VA, USA) medium with 37°C and 5% CO2. Normal glucose (NG): 25 mmol/L, Low glucose (LG): 5.5 mmol/L. Amaxa Cell Line Nucleofector Kit (LONZA, Walkersville, MD, USA) was used for the transfection. WI-38 cells were transfected by Kit R under program V-001, 293FT cells were transfected by Kit V under program Q-001.
Cell proliferation curve
We tested the population doubling level (PDL) of the WI38 cells that were kept either under NG or LG condition. Cells were cultured from 20 PDL. The method of PDL calculation was according to ATCC company: n = 3.32 (logN2 - logN1) + X; n-the final PDL, N2-total cell number of the temporal, N1-former seeding number of cells, X-former PDL of the cells.
Cell cycle and apoptosis analysis
Propidium iodide (PI) cell cycle kit (Sigma) and AnnexinV-FITC / PI apoptosis detection kit (KeyGen BioTech, Nanjing, China) were used to test cell cycle and apoptosis. Flow cytometries were performed by BD FACS Calibur and analyzed with FlowJo software.
Cell senescence associate β-Gal (SA-β-Gal) staining
Cellular SA-β-Gal assay was performed with senescence cells histochemical staining kit (Sigma) according to the standard protocol. Five random views in each group were selected to calculate the positive staining rate.
Confocal microscopy
Confocal images were obtained with a Zeiss LSM710 confocal laser scanning microscope and images were analyzed by Zen 2012 software. Antibodies for confocal microscopy were purchased from the indicated sources: Primary antibodies against SIRT6 (Cell Signaling Technology), NF-κB (Santa Cruz Biotechnology), Secondary antibodies goat anti-rabbit IgG-FITC (Santa Cruz Biotechnology) and goat anti-mouse IgG-TRITC (Santa Cruz Biotechnology) were used to indicate the content and distribution of destined proteins.
Statistical analyses
All quantitative results were obtained from at least triplicate samples. Data were analyzed with Graph Pad Prism 5.0 software. The results were expressed as mean±SEM. P values were determined by 2-tailed Student's t-test.
Abbreviations
BER | = | base excision DNA repair |
CR | = | calorie restriction |
ELISA | = | enzyme-linked immunosorbent assay |
FCM | = | flow cytometry |
H3K9 | = | histone H3 lysine 9 |
HE | = | haematoxylin-eosin |
IL-1β | = | interleukin-1β |
LG | = | low glucose |
NG | = | normal glucose |
PAS | = | periodic acid-Schiff |
PDL | = | population doubling level |
PI | = | proliferation index |
pSR | = | pSUPER.retro.puro |
SA-β-gal | = | senescent-associated β-gal; |
SIR2 | = | silent information regulator 2 |
TNF-α | = | tumor necrosis factor-α |
Disclosure of potential conflicts of interest
No potential conflicts of interest were disclosed.
Supplementary Files
Download MS Word (787.5 KB)Acknowledgments
We would like to thank Dr. Katrin F. Chua from Stanford University for the kindly share of the SIRT6-WT, SIRT6-H133Y and SIRT6-shRNAs plasmids. We also thank Dr. Andrey Parkhitko from Harvard Medical School for his efforts with manuscript modification.
Funding
This work was financially supported by the National Basic Research Program of China (973 Program, 2013CB530802) and the National Natural Science Foundation of China (No.81270425).
References
- Longo VD, Antebi A, Bartke A, Barzilai N, Brown-Borg HM, Caruso C, Curiel TJ, de Cabo R, Franceschi C, Gems D, et al. Interventions to Slow Aging in Humans: Are We Ready? Aging Cell 2015; 14:497-510; PMID:25902704; http://dx.doi.org/10.1111/acel.12338
- Guarente L. Sirtuins, aging, and metabolism. Cold Spring Harb Symp Quant Biol 2011; 76:81-90; PMID:22114328; http://dx.doi.org/10.1101/sqb.2011.76.010629
- De Cabo R, Liu L, Ali A, Price N, Zhang J, Wang M, Lakatta E, Irusta PM. Serum from calorie-restricted animals delays senescence and extends the lifespan of normal human fibroblasts in vitro. Aging 2015; 7:152-66; PMID:25855056
- Lin SJ, Defossez PA, Guarente L. Requirement of NAD and SIR2 for life-span extension by calorie restriction in Saccharomyces cerevisiae. Science 2000; 289:2126-8; PMID:11000115; http://dx.doi.org/10.1126/science.289.5487.2126
- Frye RA. Phylogenetic classification of prokaryotic and eukaryotic Sir2-like proteins. Biochem Biophys Res Commun 2000; 273:793-8; PMID:10873683; http://dx.doi.org/10.1006/bbrc.2000.3000
- Timmers S, Auwerx J, Schrauwen P. The journey of resveratrol from yeast to human. Aging 2012; 4:146-58; PMID:22436213
- Bordone L, Guarente L. Calorie restriction, SIRT1 and metabolism: understanding longevity. Nat Rev Mol Cell Biol 2005; 6:298-305; PMID:15768047; http://dx.doi.org/10.1038/nrm1616
- Nakagawa T, Guarente L. SnapShot: sirtuins, NAD, and aging. Cell Metab 2014; 20:192-e1; PMID:24988458; http://dx.doi.org/10.1016/j.cmet.2014.06.001
- Michishita E, McCord RA, Berber E, Kioi M, Padilla-Nash H, Damian M, Cheung P, Kusumoto R, Kawahara TL, Barrett JC, et al. SIRT6 is a histone H3 lysine 9 deacetylase that modulates telomeric chromatin. Nature 2008; 452:492-6; PMID:18337721; http://dx.doi.org/10.1038/nature06736
- Liszt G, Ford E, Kurtev M, Guarente L. Mouse Sir2 homolog SIRT6 is a nuclear ADP-ribosyltransferase. J Biol Chem 2005; 280:21313-20; PMID:15795229; http://dx.doi.org/10.1074/jbc.M413296200
- Mostoslavsky R, Chua KF, Lombard DB, Pang WW, Fischer MR, Gellon L, Liu P, Mostoslavsky G, Franco S, Murphy MM, et al. Genomic instability and aging-like phenotype in the absence of mammalian SIRT6. Cell 2006; 124:315-29; PMID:16439206; http://dx.doi.org/10.1016/j.cell.2005.11.044
- Kanfi Y, Naiman S, Amir G, Peshti V, Zinman G, Nahum L, Bar-Joseph Z, Cohen HY. The sirtuin SIRT6 regulates lifespan in male mice. Nature 2012; 483:218-21; PMID:22367546; http://dx.doi.org/10.1038/nature10815
- Salminen A, Huuskonen J, Ojala J, Kauppinen A, Kaarniranta K, Suuronen T. Activation of innate immunity system during aging: NF-kB signaling is the molecular culprit of inflamm-aging. Ageing Res Rev 2008; 7:83-105; PMID:17964225; http://dx.doi.org/10.1016/j.arr.2007.09.002
- Perkins ND. The importance of the p50 NF-kappaB subunit. Cell Cycle 2015; 14:2877-8; PMID:26291483; http://dx.doi.org/10.1080/15384101.2015.1010952
- Salminen A, Kaarniranta K. NF-kappaB signaling in the aging process. J Clin Immunol 2009; 29:397-405; PMID:19408108; http://dx.doi.org/10.1007/s10875-009-9296-6
- Kawahara TL, Michishita E, Adler AS, Damian M, Berber E, Lin M, McCord RA, Ongaigui KC, Boxer LD, Chang HY, et al. SIRT6 links histone H3 lysine 9 deacetylation to NF-kappaB-dependent gene expression and organismal life span. Cell 2009; 136:62-74; PMID:19135889; http://dx.doi.org/10.1016/j.cell.2008.10.052
- Sun H, Wu Y, Fu D, Liu Y, Huang C. SIRT6 regulates osteogenic differentiation of rat bone marrow mesenchymal stem cells partially via suppressing the nuclear factor-kappaB signaling pathway. Stem Cells 2014; 32:1943-55; PMID:24510807; http://dx.doi.org/10.1002/stem.1671
- Hill GS, Heudes D, Bariety J. Morphometric study of arterioles and glomeruli in the aging kidney suggests focal loss of autoregulation. Kidney Int 2003; 63:1027-36; PMID:12631084; http://dx.doi.org/10.1046/j.1523-1755.2003.00831.x
- Wang Z, Li H, Xu H, Yue XL, Cheng XQ, Hou WJ, Zhang YY, Chen DF. Beneficial effect of Bupleurum polysaccharides on autoimmune disease induced by Campylobacter jejuni in BALB/c mice. J Ethnopharmacol 2009; 124:481-7; PMID:19467314; http://dx.doi.org/10.1016/j.jep.2009.05.013
- Teoh SL, Abd Latiff A, Das S. Histological changes in the kidneys of experimental diabetic rats fed with Momordica charantia (bitter gourd) extract. Rom J Morphol Embryol 2010; 51:91-5; PMID:20191126
- Dimri GP, Lee X, Basile G, Acosta M, Scott G, Roskelley C, Medrano EE, Linskens M, Rubelj I, Pereira-Smith O, et al. A biomarker that identifies senescent human cells in culture and in aging skin in vivo. Proc Natl Acad Sci U S A 1995; 92:9363-7; PMID:7568133; http://dx.doi.org/10.1073/pnas.92.20.9363
- Metcalf TU, Cubas RA, Ghneim K, Cartwright MJ, Grevenynghe JV, Richner JM, Olagnier DP, Wilkinson PA, Cameron MJ, Park BS, et al. Global analyses revealed age-related alterations in innate immune responses after stimulation of pathogen recognition receptors. Aging Cell 2015; 14:421-32; PMID:25728020; http://dx.doi.org/10.1111/acel.12320
- Mao Z, Hine C, Tian X, Van Meter M, Au M, Vaidya A, Seluanov A, Gorbunova V. SIRT6 promotes DNA repair under stress by activating PARP1. Science 2011; 332:1443-6; PMID:21680843; http://dx.doi.org/10.1126/science.1202723
- Fulco M, Cen Y, Zhao P, Hoffman EP, McBurney MW, Sauve AA, Sartorelli V. Glucose restriction inhibits skeletal myoblast differentiation by activating SIRT1 through AMPK-mediated regulation of Nampt. Dev Cell 2008; 14:661-73; PMID:18477450; http://dx.doi.org/10.1016/j.devcel.2008.02.004
- Zhang B, Cui S, Bai X, Zhuo L, Sun X, Hong Q, Fu B, Wang J, Chen X, Cai G. SIRT3 overexpression antagonizes high glucose accelerated cellular senescence in human diploid fibroblasts via the SIRT3-FOXO1 signaling pathway. Age 2013; 35:2237-53; PMID:23494737; http://dx.doi.org/10.1007/s11357-013-9520-4
- Shay JW, Wright WE. Hayflick, his limit, and cellular ageing. Nat Rev Mol Cell Biol 2000; 1:72-6; PMID:11413492; http://dx.doi.org/10.1038/35036093
- Schafer KA. The cell cycle: a review. Vet Pathol 1998; 35:461-78; PMID:9823588; http://dx.doi.org/10.1177/030098589803500601
- Kim WY, Sharpless NE. The regulation of INK4/ARF in cancer and aging. Cell 2006; 127:265-75; PMID:17055429; http://dx.doi.org/10.1016/j.cell.2006.10.003
- Krishnamurthy J, Torrice C, Ramsey MR, Kovalev GI, Al-Regaiey K, Su L, Sharpless NE. Ink4a/Arf expression is a biomarker of aging. J Clin Invest 2004; 114:1299-307; PMID:15520862; http://dx.doi.org/10.1172/JCI22475
- Salvioli S, Monti D, Lanzarini C, Conte M, Pirazzini C, Bacalini MG, Garagnani P, Giuliani C, Fontanesi E, Ostan R, et al. Immune system, cell senescence, aging and longevity–inflamm-aging reappraised. Curr Pharm Des 2013; 19:1675-9; PMID:23589904
- Adler AS, Sinha S, Kawahara TL, Zhang JY, Segal E, Chang HY. Motif module map reveals enforcement of aging by continual NF-kappaB activity. Genes Dev 2007; 21:3244-57; PMID:18055696; http://dx.doi.org/10.1101/gad.1588507
- Stipp D. The transformative promise of aging science. Cell Cycle 2012; 11:3903-4; PMID:22983093; http://dx.doi.org/10.4161/cc.22211
- Bilinski T, Paszkiewicz T, Zadrag-Tecza R. Energy excess is the main cause of accelerated aging of mammals. Oncotarget 2015; 6:12909-19; PMID:26079722; http://dx.doi.org/10.18632/oncotarget.4271
- Rogina B, Helfand SL. Sir2 mediates longevity in the fly through a pathway related to calorie restriction. Proc Natl Acad Sci U S A 2004; 101:15998-6003; PMID:15520384; http://dx.doi.org/10.1073/pnas.0404184101
- Colman RJ, Anderson RM, Johnson SC, Kastman EK, Kosmatka KJ, Beasley TM, Allison DB, Cruzen C, Simmons HA, Kemnitz JW, et al. Caloric restriction delays disease onset and mortality in rhesus monkeys. Science 2009; 325:201-4; PMID:19590001; http://dx.doi.org/10.1126/science.1173635
- Ghizzoni M, Haisma HJ, Maarsingh H, Dekker FJ. Histone acetyltransferases are crucial regulators in NF-kappaB mediated inflammation. Drug Discov Today 2011; 16:504-11.
- Sundaresan NR, Vasudevan P, Zhong L, Kim G, Samant S, Parekh V, Pillai VB, Ravindra PV, Gupta M, Jeevanandam V, et al. The sirtuin SIRT6 blocks IGF-Akt signaling and development of cardiac hypertrophy by targeting c-Jun. Nat Med 2012; 18:1643-50; PMID:23086477; http://dx.doi.org/10.1038/nm.2961
- Min L, Ji Y, Bakiri L, Qiu Z, Cen J, Chen X, Chen L, Scheuch H, Zheng H, Qin L, et al. Liver cancer initiation is controlled by AP-1 through SIRT6-dependent inhibition of survivin. Nat Cell Biol 2012; 14:1203-11; PMID:23041974; http://dx.doi.org/10.1038/ncb2590
- Zhong L, D'Urso A, Toiber D, Sebastian C, Henry RE, Vadysirisack DD, Guimaraes A, Marinelli B, Wikstrom JD, Nir T, et al. The histone deacetylase Sirt6 regulates glucose homeostasis via Hif1alpha. Cell 2010; 140:280-93; PMID:20141841; http://dx.doi.org/10.1016/j.cell.2009.12.041
- Blagosklonny MV. Linking calorie restriction to longevity through sirtuins and autophagy: any role for TOR. Cell Death Dis 2010; 1:e12; PMID:21364614; http://dx.doi.org/10.1038/cddis.2009.17
- Inoue T, Hiratsuka M, Osaki M, Oshimura M. The molecular biology of mammalian SIRT proteins: SIRT2 in cell cycle regulation. Cell Cycle 2007; 6:1011-8; PMID:17457050; http://dx.doi.org/10.4161/cc.6.9.4219
- Van Meter M, Mao Z, Gorbunova V, Seluanov A. SIRT6 overexpression induces massive apoptosis in cancer cells but not in normal cells. Cell Cycle 2011; 10:3153-8; PMID:21900744; http://dx.doi.org/10.4161/cc.10.18.17435
- Kaidi A, Weinert BT, Choudhary C, Jackson SP. Human SIRT6 promotes DNA end resection through CtIP deacetylation. Science 2010; 329:1348-53; PMID:20829486; http://dx.doi.org/10.1126/science.1192049
- Walker JM. The bicinchoninic acid (BCA) assay for protein quantitation. Methods Mol Biol 1994; 32:5-8; PMID:7951748