ABSTRACT
The roles of exonuclease 1 (EXO1) in hepatocellular carcinoma (HCC) tumorigenesis and progression remain unclear. This study aimed to assess the prognostic value and therapeutic potential of EXO1 in HCC. Exo1 gene copy numbers were obtained from three Oncomine microarray datasets (n = 447). EXO1 mRNA expression was validated by semi-quantitative PCR and QuantiGene® 2.0 assays. Cell growth curve and colony formation were performed to asses the cell proliferation. Clonogenic assay, flow cytometry, and immunofluorescence were adopted to acess the effects of EXO1 knockdown and radiation on cell survival, cell cycle distribution and DNA repair. Western blots were performed to reveal the related mechanism. A significant copy number variation (CNV) of the Exo1 gene was found in HCC specimens in three separate sets of published microarray data. In the 143 cases treated by our team, EXO1 expression levels were elevated (86.71%, 124/143). In addition, EXO1 overexpression was correlated with larger tumor size (P = 0.002), increased lymph node metastasis (P=0.033) and lower Edmondson grade (P = 0.018). High EXO1 expression unfavorably affected overall survival (OS) (P = 0.009). Both univariate and multivariate Cox regression analyses identified EXO1 as an independent predictor of OS (univariate, P = 0.012; multivariate, P = 0.039). Silencing of EXO1 in vitro reduced cell proliferation. EXO1 knockdown further suppressed clonogenic cell survival, abrogated radiation-induced G2/M phase arrest, and enhanced γ-H2AX foci after exposure to irradiation. The accumulation of ataxiatelangiectasia mutated (ATM) might partially regulate the EXO1 related radiosensitivity. In summary, EXO1 could be a promising prognostic marker, with a potential therapeutic value in HCC.
Introduction
Hepatocellular carcinoma (HCC) is one of the most common cancers worldwide, with the highest incidence rate in East Asia and Africa, and an increasing prevalence in Western countries [Citation1]. Multiple risk factors result in poor prognosis of HCC patients, e.g. abnormal expression or mutation of susceptibility genes [Citation2]. In China, HCC is the fourth most prevalent cancer and the third leading cause of cancer-related deaths [Citation3]. Due to late diagnosis, high incidence of tumor recurrence after curative resection, and resistance to radiotherapy and chemotherapy, HCC prognosis remains poor [Citation4]. Biomarkers to predict and treat HCC are required for timely invention.
As a member of the RAD2/XPG nuclease family, the exonuclease 1 (Exo1) gene is located at chromosome 1q42–43 and encodes an 846 amino acid protein [Citation5,Citation6]. The coding protein comprises the N-terminal nuclease and interaction domains [Citation7]. Two subdomains of the N-terminal region, namely the DNA (N-domain) and Mg 2+ (I-domain) binding domains, facilitate EXO1 binding to DNA [Citation8]. The C-terminal domain directly interacts with the mismatch repair proteins MLH1 and MSH2, forming a ternary complex of EXO1-MLH1-PMS2 or EXO1-MSH2-MSH6 [Citation6,Citation9–Citation12]. These functional domains, at least partly, contribute to Exo1 associated 5′ exonuclease, 5′ flap, and intrinsic 5′ RNase H activities in several biological processes, including DNA mismatch repair (MMR), micro-mediated end-joining, homologous recombination (HR), and replication [Citation6,Citation7,Citation9,Citation13–Citation16].
Since EXO1 plays a key role in DNA repair, contributes to maintaining chromatin stability, and modulates DNA recombination, the relationship between polymorphisms of this gene and risk of cancer has been evaluated, with at least 9 genetic variants identified in cancer. A widely assessed non-synonymous single nucleotide polymorphism (SNP) at codon 589 (rs1047840KE) is closely associated with increased susceptibility to lung cancer [Citation17–Citation19], gastric cancer [Citation20], cervical cancer [Citation21], breast cancer [Citation22], oral cancer [Citation23], colorectal cancer [Citation24], glioma [Citation25] and HCC [Citation26]. Such SNP could be used as a biomarker of carcinogenesis [Citation27]. Besides, partly due to the heterogeneity of different cancer types, it remains an open question whether other moderate genetic polymorphisms induce or inverse cancer susceptibility. In Turkish HCC patients, no association was found of the rs4149963TM polymorphism with genetic susceptibility to hepatocarcinogenesis [Citation28]. In a Chinese cohort, the rs3754093AG genotype was shown to exhibit reduced HCC cancer risk [Citation29].
Compared with the numerous molecular epidemiological studies assessing EXO1 polymorphisms, reports on its expression in carcinogenesis and prognosis are limited. EXO1 transcription is enhanced by FOXM1 after treatment with cisplatin, with increased survival of ovarian cancer cells after cisplatin treatment [Citation30]. In astrocytoma, EXO1 is remarkably overexpressed and correlated with unfavorable patient prognosis [Citation31]. However, the expression and prognostic value of EXO1 in HCC remains undefined.
This study demonstrated a significant copy number variation (CNV) occurring in the Exo1 gene. We then validated EXO1 expression in 143 cases of HCC with long-term follow-up, to identify a biomarker associated with clinical significance and unfavorable prognosis. In addition, in vitro functional analyses revealed the potential roles of EXO1 in radiation-induced double strained breaks (DSBs) and DNA repair.
Results
CNV and mRNA expression of EXO1 are elevated in HCC specimens
First, we assessed the copy number profiles of EXO1 in human HCC in multiple patient datasets available in the Oncomine database, including Guichard Liver, Guichard Liver 2 and TCGA Liver, with a total of 222 HCC and 225 non-HCC cases. Compared with the normal DNA copy number in blood and liver tissue samples, a significant elevation of DNA copy number of EXO1 was observed in all three profiles (). Fold changes of average DNA copy number in the three HCC groups were 1.112, 1.096 and 1.238, separately. This finding indicated a possible overexpression of EXO1 mRNA in HCC specimens.
To verify this possibility, we then analysed EXO1 expression levels in 20 HCC paired hepatic tissues by semi-quantitative PCR. EXO1 was overtly overexpressed in 18 of 20 HCC specimens compared with the respective normal adjacent liver tissues (). Then, EXO1 mRNA expression levels were analysed using QuantiGene Plex 2.0 assay in 143 additional paired HCC specimens. EXO1 was also significantly (P < 0.0001) overexpressed (HCC/non-HCC> 2) in 124 HCC tumor tissues compared with the corresponding non-tumor counterparts, with average relative mRNA expression levels of 0.148 ± 0.01 (HCC) and 0.018 ± 0.002 (non-HCC), respectively ().
Figure 1. EXO1 gene copy number is increased in HCC as assessed by data-mining of the Oncomine database. Box-whiskers Plot of EXO1 gene copy number between normal and cancer tissue samples in (a) TCGA Liver, (b) Guichard Liver, and (c) Guichard Liver 2 data sets. B, blood; N, normal liver; T, HCC. Numbers below the horizontal axis indicate total cases in different groups. The line within each box represents the median value; lower and upper edges of each box represent the 25th and 75th percentiles, respectively; lower and upper whiskers represent 5th and 95th percentiles, respectively. P values were calculated by the Oncomine database.
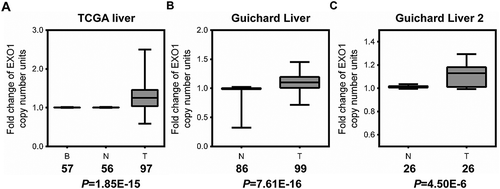
Figure 2. EXO1 mRNA expression levels in HCC and paired adjacent non-tumorous liver tissues. (a) Semi-quantitative RT-PCR data for EXO1 in 20 liver cancer cases and paired normal tissues. (b) EXO1 mRNA levels in 143 paired HCC and adjacent non-tumorous liver tissues measured by the QuantiGene Plex assay. Statistical significance was determined by paired two-tailed Student’s t test. ****, P < 0.0001. Lines from the top represent the 75th, 50th, and 25th of EXO1 mRNA values, respectively. N, normal liver; T, HCC.
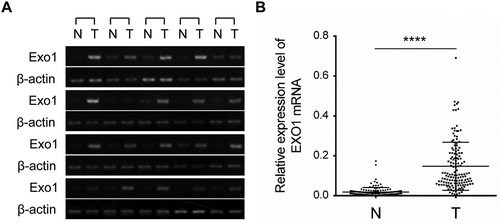
Clinical and prognostic significance of EXO1 expression in HCC patients
Since EXO1 overexpression in HCC tissues was consistently observed, we then assessed the associations of EXO1 expression with clinicopathological parameters in the 143 above HCC patients (). High EXO1 expression was associated with larger tumor size (P = 0.002), increased lymph node metastasis (P = 0.033) and higher Edmondson grade (P = 0.018).
Table 1. Analyses of the EXO1 gene in 143 HCC tissues.
Further, we observed that there was no consistency between EXO1 mRNA levels and serum AFP protein in HCC tissues. EXO1 was upregulated in 47 cases of HCC specimens (47/143, 32.87%) with no serum AFP protein (< 200μg/l). The combined use of EXO1 and serum AFP would improve the diagnostic sensitivity from 48.95% (70/143) to 81.82% (117/143, ).
The relationship between EXO1 expression and patient prognosis was also estimated. Mean overall survival (OS) rates in the EXO1-high and EXO1-low groups were 17.6 and 25.3 months, respectively. In the Kaplan-Meier survival analysis model, the 1-, 3-, and 5-year OS rates were 52.69%, 15.05%, and 3.23% in patients with high EXO1 expression, respectively, and 66.0%, 26.0%, and 8.0% in those with low EXO1 expression (P = 0.009, ). Although EXO1 expression was not correlated with age (≤ 55), AFP (≤ 20µg/L) and tumor number (n = 1), high EXO1 expression showed unfavorable effects within the subgroups of patients with no serum AFP (P = 0.012), under 55 years old (P = 0.013), and with single tumor in the liver (P = 0.036) (). Further subgroup analyses revealed that among patients with no lymph node metastasis and Edmonson grade I cases, high EXO1 expression also showed unfavorable effects on OS (P = 0.002 and P = 0.003, ).
Figure 3. Prognostic value of EXO1 mRNA expression in HCC. (a) Distribution of EXO1 mRNA and serum AFP expression levels in 143 HCC patients. The numbers on the pie chart represent the percentages of various groups. EXO1 high, EXO1 mRNA levels ≥ 0.075 in HCC specimens; EXO1 low, EXO1 mRNA levels < 0.075 in HCC specimens; AFP high, AFP protein levels ≥ 200μg/l in peripheral blood; AFP low, AFP protein levels < 200μg/l in peripheral blood. (B to G) Kaplan-Meier analyses of EXO1 mRNA expression levels in 143 HCC patients (b), cases with serum AFP ≤ 20µg/L(c), patients with age ≤ 55 years (d), cases with single tumor (e), patients with no lymph node metastasis (f) and Edmonson stage I cases (G). High EXO1 expression was significantly correlated with poor prognosis (P < 0.05).
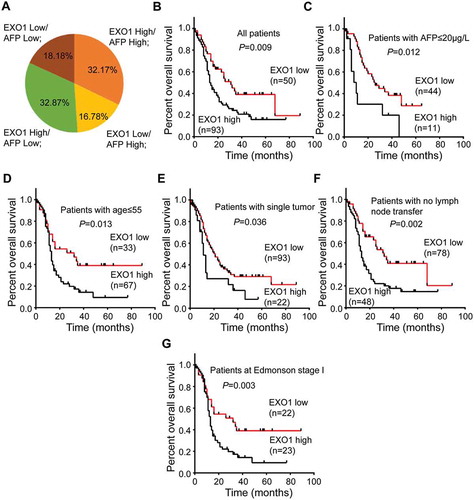
Univariate and multivariate Cox regression analyses were performed to evaluate the associations of EXO1 with clinical risk factors. Univariate analysis revealed that EXO1 expression (Hazard ratio [HR] = 1.805, P = 0.012), as well as two clinical pathological characteristics, including gender (HR = 4.573, P = 0.034) and tumor size (HR = 2.105, P = 0.014), showed unfavorable effects on OS (). In multivariate analysis, EXO1 expression (HR = 1.633, P = 0.039) and gender (HR = 4.57, P = 0.034) were independent predictors of OS (). Besides, tumor size tended to show an unfavorable independent effect on OS (HR = 1.817, P = 0.052). These results demonstrated the diagnostic and prognostic values of EXO1 in HCC patients.
Table 2. Univariate and multivariate Cox regression analyses of EXO1 mRNA expression and clinical variables for overall survival in HCC patients.
EXO1 regulates cell growth and colony formation
To assess the potential contribution of EXO1 inactivation in HCC, we performed the cell proliferation assay and the colony formation assay with stablely transfected BEL-7404 and YY-8103 cells in the EXO1 shRNA (sh-EXO1) and the negative control (sh-NC) groups. The EXO1 protein expression was decreased to various degrees in both HCC cells. Results showed that knockdown of EXO1 reduced the proliferation of YY-8103 at the fourth (P = 0.012) and fifth days (P = 0.003) after cell plating (), and the anchorage-dependent colony formation of both BEL-7404 (P = 0.003) and YY8103 (P = 0.001, ).
Figure 4. EXO1 knockdown descreases the cell proliferation in HCC cells. (a) Cell growth curves of BEL-7404 and YY-8103. EXO1 proteins were detected by western blots. (b) Colony formation of BEL-7404 and YY-8103. All experiments were performed in triplicates and data are shown as mean ± SD. Statistical significance was determined by two-tailed Student’s t test. *, P < 0.05; **, P < 0.01.
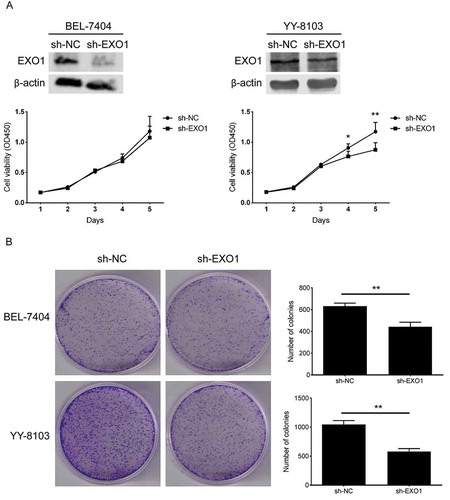
EXO1 silencing facilitates cell cycle dynamics in HCC cells
We then analysed the cell cycle distribution of stably transfected HCC cells. The proportion of cells in the G0/G1 phase was significantly increased in BEL-7404 (P = 0.0006) and YY-8103 cells (P = 0.0007), along with a starkly decreased G2/M phase in YY-8103 (P = 0.0003) cells after EXO1 silencing ().
Considering the possible role in DNA mismatch repair and chromatin stability maintenance, we also examined the cell cycle dynamics in response to ionizing radiation (IR). Along with the similar G0/G1 phase arrest, a significant reduction in G2/M phase BEL-7404 cells in the EXO1 knockdown group was observed 24h after an exposure to a 4 Gy dose of radiation (P < 0.0001, ). An additional S phase reduction was also observed in both EXO1 knockdown cells (BEL-7404, P = 0.007; YY-8103, P = 0.027; ). These results indicated the impact of EXO1 overexpression in IR-induced DNA damage repair in HCC cells.
Figure 5. EXO1 silencing dysregulates the cell cycle in HCC cells. (a) Cell cycle analysis by DNA content measured flow-cytometrically. (b) Cell cycle analysis 24h after ionizing radiation (4Gy). Cell cycle models were fit by the ModFit LT software (left). Average percentages of G0/G1, S and G2/M phase cells are shown on the right. All experiments were performed in triplicate, and data are mean± SD. Statistical significance was determined by two-tailed Student’s t test. *, P < 0.05; **, P < 0.01; ***, P < 0.001; ****, P < 0.0001.
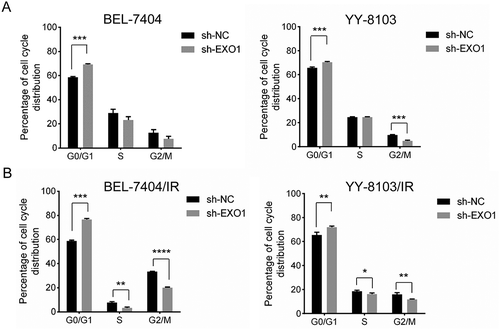
EXO1 knockdown increases radiosensitivity and inhibits DNA repair in YY-8103 cells
Clonogenic survival assay was performed to determine whether downregulation of EXO1 could affect the radioresistance of YY-8103 cells. Lower expression of EXO1 combined with radiation reduced the clonogenic growth, which indicated that EXO1 expression could significantly contribute to the cell survival after irradiation with a 2 (P = 0.042), 6 (P = 0.001) and 8 (P = 0.001) Gy dose ()).
The γ-H2AX focus formation was analysed to measure the induction of DNA-DSBs after irradiation. HCC cells with less EXO1 exhibited significantly increased γ-H2AX foci 1h post-radiation compared with control cells (P = 0.03, )). It suggested that inhibition of the DNA repair might be the molecular mechanism of the radiosensitization induced by lack of EXO1.
Figure 6. EXO1 knockdown regulates the clonogenic survival, γ-H2AX locus formation and ATM accumulation in YY-8103 cells. (a) Clonogenic survival fractions of cells irradiated with a single dose of 0, 2, 4, 6 and 8 Gy X-rays. Survival curves were obtained by means of 3 standard colony formation assay. (b) γ-H2AX loci of cells irradiated with a single dose of 2 Gy. Quantitative analysis of γ-H2AX foci per cell averaged over 50 cells per data point. (c) ATM accumulation of cells irradiated with a single dose of 2 Gy revealed by western blots. Statistical significance was determined by two-tailed Student’s t test. *, P < 0.05; **, P < 0.01.
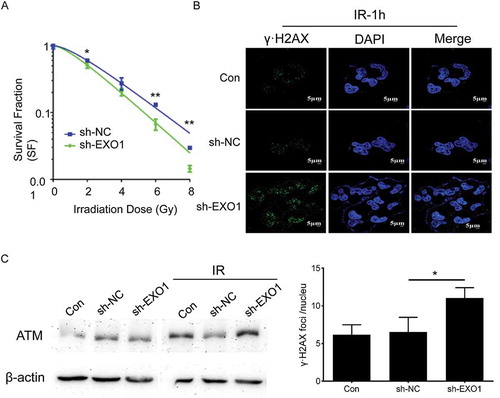
Targets of EXO1 on DSBs induced DNA repair were assessed with western blot assay. An enhanced expression of ataxiatelangiectasia mutated (ATM) was observed in irradiated HCC cells with lower EXO1 expression ()), suggesting the potential mechanism of EXO1 involved radioprotection.
Discussion
By data mining, we observed increased DNA copy number of Exo1 in HCC, in agreement with previous findings that the chromosomal region 1q, especially 1q42.12 and 1q43, has frequent copy number gains in more than 65% of HCC cases [Citation32]. This finding prompted us to assess the expression levels of EXO1 in HCC, which remain unknown, since copy number variation (CNV) is an important genetic aberration and leads to the activation of oncogenes in cancer [Citation33]. Moreover, a possible mechanism of chromosome abnormalities responsible for EXO1 gene upregulation other than modulation at the transcription and post-translation levels could be demonstrated [Citation34,Citation35].
Although the role of EXO1 polymorphism in various cancers is well reported, the expression of this gene and its clinical significance in HCC remain unclear. This study identified EXO1 upregulation in HCC, with significant associations with clinical parameters, including tumor size, Edmondson grade, lymph node metastasis, and overall survival. The complementary between EXO1 and AFP levels was also observed. These results suggested the potential role of EXO1 as a molecular biomarker of HCC.
Next, we assessed the prognostic value of EXO1. Kaplan-Meier survival analysis revealed that high EXO1 expression predicted a worse prognosis of HCC patients. In Cox regression analyses, EXO1 expression was (univariate) or tended to be (multivariate) an independent risk factor, while gender and tumor size were definite independent risk factors. As a widely used marker for routine surveillance and noninvasive diagnosis of HCC, high serum AFP levels (≥ 20µg/L) play an important role in predicting high mortality among patients with tumor size ≥ 5 cm [Citation36]. However, prognostic markers for patients with no AFP expression (< 20µg/L) are rare. In this study, EXO1 showed an unfavorable effect in patients with no AFP expression (P = 0.012). Thus, EXO1 could be used to predict mortality, especially in AFP-negative-HCC patients.
Double strand breaks (DSBs) are the most toxic DNA damage caused by IR, and could lead to genomic instability without correct repair. Using clonogenic assay, we found that EXO1 expression affects the HCC cell survival under irradiation. To identify the potential mechanism, the G2/M phase block and the phosphorylation of γ-H2AX, which are the two important markers of DNA damage induced repair, were measured in HCC cells with different EXO1 expression levels. Silencing of EXO1 significantly reduced the G2/M arrest and produced a higher amount of γ-H2AX loci under the IR pressure. However, it contradicted with the previous report that EXO1 knockdown could favor DSB restoration by inducing a G2/M phase arrest in astrocytoma [Citation31]. The conflicting phenotypes of radiosensitivity induced by EXO1 knockdown may be due to the functional diversity of the gene in the individual cancers. The details of the related signaling pathway still need more investigation. Radiation-induced G2-phase blockage is a universal event in tumor cells that makes the G2/M checkpoint a target to improve sensitivity to radiation [Citation37]. Thus, this funcional study highlighted the potential of EXO1 as a target for improving the efficiency of radiation therapy. The accumulation of ATM indicates a possible mechanism for EXO1 in DSBs induced radioprotection. Further studies should be designed to reveal more details of the related signaling pathways.
Materials and methods
Eligibility of HCC gene DNA copy number datasets
Raw DNA copy number datasets of HCC and normal control samples were selected and downloaded from the Oncomine database (https://www.oncomine.org/resource/login.html). Datasets containing DNA data of HCC tumors, liver specimens and blood samples were included. Data sets for cirrhotic liver tissue, non-human specimen and cell lines were excluded.
Tissue specimens and cell culture
A total of 143 surgically resected HCC tissue specimens and respective adjacent non-HCC tissue samples were obtained from patients in the First Affiliated Hospital of Soochow University Hospital between 2006 and 2014. The patients were diagnosed with HCC based on clinical symptoms, serological tests, imaging, and pathological evaluations according to “Primary Liver Cancer Clinical Diagnosis and Staging Criteria” [Citation38]. All samples were stored at −80°C immediately after surgical resection. The recorded clinicopathological characteristics included age, gender, hepatitis B surface antigen (HBsAg) amounts, alpha-fetoprotein (AFP) levels, tumor size and number, Edmondson’s histologic grade, and lymph node metastasis status (). Dates of surgical resection and last follow-up or HCC-related death were also recorded. The average overall survival time was 20.3 months (median, 14 months; range, 1 to 89 months).
The human hepatoma cell line BEL-7404 and YY-8103 were cultured in Dulbecco’s Modified Eagle’s medium (DMEM, Thermo Fisher, Waltham, USA) supplemented with 10% (v/v) fetal bovine serum (FBS, Thermo Fisher, Waltham, USA) at 37°C in a humidified incubator under 5% CO2.
RNA extraction and cDNA synthesis
Total RNA was extracted from 20–30 mg of tissue or 1 × 105–1 × 106 cells with TRIzol Reagent (TaKaRa, Otsu, Japan) according to the manufacturer’s instructions. Total RNA was treated with RNase-free DNase Ι (TaKaRa, Otsu, Japan) to prevent genomic DNA contamination. RNA amounts were determined on a NanoDrop 2000 (Thermo Fisher, Waltham, USA). Total RNA integrity was assessed by visualization of the 28S and 18S ribosomal RNAs by 1.2% agarose gel electrophoresis. First strand cDNA was synthesised with 1µg total RNA, with Prime Script RT Reagent Kit (TaKaRa, Otsu, Japan) according to the manufacturer’s instructions.
Semi-quantitative PCR, quantitative real-time PCR and quantigene plex assay
Both semi-quantitative RT-PCR (RT-PCR) and quantitative real-time PCR (qRT-PCR) were performed with the following primers for EXO1 (amplicon length of 266bp): 5′-TCTGAGAGGTAGTTAATTTGG-3′ (forward) and 5′-TACATCCATCAAATACGAGA-3′ (reverse). β-actin served as an internal reference, and was assessed with the following primers (amplicon length of 213bp): 5′-AGAGCCTCGCCTTTG-3′ (forward) and 5′-CATAGGAATCCTTCTGACC-3′ (reverse). RT-PCR reactions were performed at 94°C for 5 min followed by 32 cycles (EXO1) or 25 cycles (β-actin) of 94°C for 30 sec, 58°C for 30 sec and 72°C for 30 sec, and a final extension at 70°C for 5 min. The resulting products were separated by 2% agarose gel electrophoresis using ethidium bromide for detection. For qRT-PCR, amplification was performed with SYBR Premix Ex Taq (TaKaRa, Otsu, Japan) according to the manufacturer’s instructions. Each sample was assessed in triplicate. The 2−ΔΔCt method [Citation39] was used for data analysis.
QuantiGene Plex 2.0® assay (Thermo Fisher, Waltham, USA) was used to quantify EXO1 mRNA expression in HCC specimens and respective adjacent non-tumorous liver tissues, following the manufacturer’s instructions. HPRT1 was used as a reference control. The oligo nucleotide probe sets were designed by Thermo Fisher. The initial amounts of each total RNA sample was 200μg. Mean fluorescence intensity (MFI) generated from each specific probe set was captured and quantified on a Luminex instrument (Bio-Rad).
ShRNA, cell transfection and selection
Exo1 shRNA (sc-44,880-SH) and its negative control carrying an irrelevant nucleotide (sc-108,060) were purchased from Santa Cruz Biotechnology (California, USA). Then, shRNA transfection of HCC cells was performed with Lipofectamine 2000 (Invitrogen) according to the manufacturer’s instructions. After 48h of transfection, the cells were subjected to resistance selection (puromycin, working concentration at 2µg/ml) for a week. Live cells were further cultured and lysed for the following manipulation.
Ionizing radiation
A single dose of IR was delivered by a Siemens 6 MV X-ray linear accelerator at room temperature, with a dose rate of 1.177 Gy/min.
Cell proliferation assay and clonogenic cell survival analysis
For proliferation assay, cells were seeded into 96-well plates at a concentration of 2 × 103 per well and cultured for 5 days to assess the proliferation. CCK-8 were applied at a 24-hour intervals following the manuscript. The absorbance was measured at 450nm as an indicator of cell viability.
For colony formation assays, 500 cells were seeded into per 35mm dish and cultured for 2 weeks. Cells were fixed with 90% ethonal for 10min and stainned with 0.1% crystal violet for 10min. The number of clones were counted with Image J software.
To estimate the cell growth in reponse to DSBs, cells seeded into 6-well plates were exposed to various doses up to 8 Gy X-rays, and then cultured for 2 weeks before being stained with 0.1% crystal violet solution. The number of colonies per dish was counted. The plating efficiency and surviving fraction were calculated as previously described [Citation40].
Cell cycle analysis
The plated cells were irradiated with 4 Gy X-ray 24h before cell cycle analysis. Cells were harvested with trypsin and washed with phosphate-buffered saline (PBS) before fixation with cold 70% ethanol for 30 min at 4°C. The fixed cells were washed twice and re-suspended in PBS containing 50µg/ml propidium iodide (PI) and 100μg/ml DNase-free RNase A. The cell suspensions were protected from light, incubated for 30 min at room temperature, and analysed on a flow cytometer (Becton Dickinson, Franklin Lakes, USA). Cell cycle analysis was performed with the CellQuest software and ModFit LT (Verity Software House, ME, USA).
Immunofluorescence assay
To determine the residual DNA DSBs, cells grown on coverslips were irradiated with 2 Gy X-rays and the immunofluorescence assay was applied to detect the γ-H2AX foci 24h later. The primary antibody phospho-H2AX (ser139, dilution 1:500; Millipore) and the secondary FITC-conjugated antibody was used for immunostaning. The detailed procedure has been previously described [Citation40].
Western blot analysis
Western blots were performed to identify the DNA repair related proteins. The irradiated cells were released with RIPA lysate (Beyotime). The following primary antibodies were used for immunoblotting: EXO1 (11,690–1-AP, dilution, 1:1000; Proteintech), ATM (2873T) (dilution, 1:1,000; Cell Signaling Technology, Inc.), β-actin (C-4, dilution, 1:1,000; Santa Cruz), as well as the secondary antibodies goat anti- mouse and goat anti-rabbit IgG (dilution, 1:1,000; LI-COR).
Statistical analysis
Statistical analyses were performed with the SPSS21.0 software. The cut-off value was optimised with the X-tile software for defining high and low subgroups. Correlation analysis was performed by the Chi-square test. The Kaplan-Meier method with the log-rank test was used to assess overall survival. Univariate and multivariate analyses were conducted with the Cox proportional hazards regression model. Two-tailed Student’s t-test was used to assess quantitative variables. P < 0.05 was considered statistically significant.
Grant Support
This work was funded by the National Natural Science Foundation of China (81,502,059, 81,472,582 and 81,774,291), Shanghai Rising-Star Program (16QB1402900), the Science and Technology Development Foundation of Pudong New District, Shanghai, China (PKJ2015-S29) and Shanghai Sailing Program (17YF1416000).
Disclosure statement
No potential conflict of interest was reported by the authors.
Additional information
Funding
References
- Torre LA, Bray F, Siegel RL, et al. Global cancer statistics, 2012. CA Cancer J Clin. 2015;65:87–108.
- Lazarevich NL, Cheremnova OA, Varga EV, et al. Progression of HCC in mice is associated with a downregulation in the expression of hepatocyte nuclear factors. Hepatology. 2004;39:1038–1047.
- Chen W, Zheng R, Baade PD, et al. Cancer statistics in China, 2015. CA Cancer J Clin. 2016;66:115–132.
- Bang H, Ha SY, Hwang SH, et al. Expression of PEG10 Is associated with poor survival and tumor recurrence in hepatocellular carcinoma. Cancer Res Treat. 2015;47:844–852.
- Wilson DM 3rd, Carney JP, Coleman MA, et al. Hex1: a new human Rad2 nuclease family member with homology to yeast exonuclease 1. Nucleic Acids Res. 1998;26:3762–3768.
- Schmutte C, Marinescu RC, Sadoff MM, et al. Human exonuclease I interacts with the mismatch repair protein hMSH2. Cancer Res. 1998;58:4537–4542.
- Keijzers G, Bohr VA, Rasmussen LJ. Human exonuclease 1 (EXO1) activity characterization and its function on flap structures. Biosci Rep. 2015;35(3).
- Orans J, McSweeney EA, Iyer RR, et al. Structures of human exonuclease 1 DNA complexes suggest a unified mechanism for nuclease family. Cell. 2011;145:212–223.
- Jager AC, Rasmussen M, Bisgaard HC, et al. HNPCC mutations in the human DNA mismatch repair gene hMLH1 influence assembly of hMutLalpha and hMLH1-hEXO1 complexes. Oncogene. 2001;20:3590–3595.
- Schmutte C, Sadoff MM, Shim KS, et al. The interaction of DNA mismatch repair proteins with human exonuclease I. J Biol Chem. 2001;276:33011–33018.
- Liberti SE, Andersen SD, Wang J, et al. Bi-directional routing of DNA mismatch repair protein human exonuclease 1 to replication foci and DNA double strand breaks. DNA Repair (Amst). 2011;10:73–86.
- Chen ZY, Zheng SR, Zhong JH, et al. Association between three exonuclease 1 polymorphisms and cancer risks: a meta-analysis. Onco Targets Ther. 2016;9:899–910.
- Szankasi P, Smith GR. A role for exonuclease I from S. pombe in mutation avoidance and mismatch correction. Science. 1995;267:1166–1169.
- Morin I, Ngo HP, Greenall A, et al. Checkpoint-dependent phosphorylation of Exo1 modulates the DNA damage response. Embo J. 2008;27:2400–2410.
- Tran PT, Erdeniz N, Symington LS, et al. EXO1-A multi-tasking eukaryotic nuclease. DNA Repair (Amst). 2004;3:1549–1559.
- Bardwell PD, Woo CJ, Wei K, et al. Altered somatic hypermutation and reduced class-switch recombination in exonuclease 1-mutant mice. Nat Immunol. 2004;5:224–229.
- Tang J, Tang S, Liu J, et al. Genetic risk of lung cancer associated with a single nucleotide polymorphism from EXO1: a meta analysis. Int J Clin Exp Med. 2015;8:11132–11138.
- Jin G, Wang H, Hu Z, et al. Potentially functional polymorphisms of EXO1 and risk of lung cancer in a Chinese population: a case-control analysis. Lung Cancer. 2008;60:340–346.
- Hsu NY, Wang HC, Wang CH, et al. Lung cancer susceptibility and genetic polymorphisms of Exo1 gene in Taiwan. Anticancer Res. 2009;29:725–730.
- Bau DT, Wang HC, Liu CS, et al. Single-nucleotide polymorphism of the Exo1 gene: association with gastric cancer susceptibility and interaction with smoking in Taiwan. Chin J Physiol. 2009;52:411–418.
- Luo X, Hong XS, Xiong XD, et al. A single nucleotide polymorphism in EXO1 gene is associated with cervical cancer susceptibility in Chinese patients. Int J Gynecol Cancer. 2012;22:220–225.
- Wang HC, Chiu CF, Tsai RY, et al. Association of genetic polymorphisms of EXO1 gene with risk of breast cancer in Taiwan. Anticancer Res. 2009;29:3897–3901.
- Tsai MH, Tseng HC, Liu CS, et al. Interaction of Exo1 genotypes and smoking habit in oral cancer in Taiwan. Oral Oncol. 2009;45:e90–4.
- Kabzinski J, Przybylowska K, Mik M, et al. Association of polymorphism of Lys589Glu Exo1 gene with the risk of colorectal cancer in the Polish population. Pol Przegl Chir. 2014;86:370–373.
- Chang JS, Yeh RF, Wiencke JK, et al. Pathway analysis of single-nucleotide polymorphisms potentially associated with glioblastoma multiforme susceptibility using random forests. Cancer Epidemiol Biomarkers Prev. 2008;17:1368–1373.
- Bayram S, Akkiz H, Bekar A, et al. The significance of Exonuclease 1 K589E polymorphism on hepatocellular carcinoma susceptibility in the Turkish population: a case-control study. Mol Biol Rep. 2012;39:5943–5951.
- Duan F, Song C, Dai L, et al. The significance of Exo1 K589E polymorphism on cancer susceptibility: evidence based on a meta-analysis. PLoS One. 2014;9:e96764.
- Bayram S, Akkiz H, Bekar A, et al. No association of the exonuclease 1 T439M polymorphism and risk of hepatocellular carcinoma development in the Turkish population: a case-control study. Asian Pac J Cancer Prev. 2011;12:2455–2460.
- Tan S, Qin R, Zhu X, et al. Associations between single-nucleotide polymorphisms of human exonuclease 1 and the risk of hepatocellular carcinoma. Oncotarget. 2016;7:87180–87193.
- Zhou J, Wang Y, Wang Y, et al. FOXM1 modulates cisplatin sensitivity by regulating EXO1 in ovarian cancer. PLoS One. 2014;9:e96989.
- de Sousa JF, Torrieri R, Serafim RB, et al. Expression signatures of DNA repair genes correlate with survival prognosis of astrocytoma patients. Tumour Biol. 2017;39:1010428317694552.
- Chochi Y, Kawauchi S, Nakao M, et al. A copy number gain of the 6p arm is linked with advanced hepatocellular carcinoma: an array-based comparative genomic hybridization study. J Pathol. 2009;217:677–684.
- Zhou C, Zhang W, Chen W, et al. Integrated analysis of copy number variations and gene expression profiling in hepatocellular carcinoma. Sci Rep. 2017;7:10570.
- Colombo CV, Trovesi C, Menin L, et al. The RNA binding protein Npl3 promotes resection of DNA double-strand breaks by regulating the levels of Exo1. Nucleic Acids Res. 2017;45:6530–6545.
- Bologna S, Altmannova V, Valtorta E, et al. Sumoylation regulates EXO1 stability and processing of DNA damage. Cell Cycle. 2015;14:2439–2450.
- Dai CY, Lin CY, Tsai PC, et al. Impact of tumor size on the prognosis of hepatocellular carcinoma in patients who underwent liver resection. J Chin Med Assoc. 2018;81(2):155-163.
- Anastasov N, Hofig I, Vasconcellos IG, et al. Radiation resistance due to high expression of miR-21 and G2/M checkpoint arrest in breast cancer cells. Radiat Oncol. 2012;7:206.
- Verslype C, Rosmorduc O, Rougier P. Hepatocellular carcinoma: ESMO-ESDO clinical practice guidelines for diagnosis, treatment and follow-up. Ann Oncol. 2012;23(Suppl 7):vii41–8.
- Livak KJ, Schmittgen TD. Analysis of relative gene expression data using real-time quantitative PCR and the 2(-Delta Delta C(T)) Method. Methods. 2001;25:402–408.
- Sun KK, Zhong N, Yang Y, et al. Enhanced radiosensitivity of NSCLC cells by transducer of erbB2.1 (TOB1) through modulation of the MAPK/ERK pathway. Oncol Rep. 2013;29:2385–2391.