ABSTRACT
Atg11 is an adaptor protein required for the induction of selective autophagy via receptor binding. However, our understanding of the molecular mechanisms by which it regulates selective autophagy remains incomplete. Here, we show that Atg11 is phosphorylated by Atg1. Rapamycin treatment or starvation conditions induced slower electrophoretic mobility of Atg11 in an Atg1 kinase activity-dependent manner. Through in vitro kinase assays combined with mutagenesis, we determined that Atg1 phosphorylates S949, S1057, and S1064 residues in CC4 domain of Atg11. Replacing the three residues with alanine suppressed the cleavage of selective autophagy substrates for the cytoplasm-to-vacuole targeting (Cvt) pathway, mitophagy, reticulophagy, and pexophagy. The Atg11 mutant was defective in binding to related selective autophagy receptors. These results demonstrate a previously unknown function of Atg1 in regulation of selective autophagy via Atg11 phosphorylation.
Abbreviations: AMPK: AMP-activated protein kinase; ATG: autophagy-related; Cvt: cytoplasm-to-vacuole targeting; FUNDC1: FUN14 domain-containing protein 1; GFP: green fluorescent protein; MTOR: mechanistic target of rapamycin kinase; PAS: phagophore assembly site; PIK3C3: phosphatidylinositol 3-kinase catalytic subunit type 3; PRKAC/PKA: protein kinase cAMP-activated; SD-G: glucose starvation; SD-N: nitrogen starvation; ULK1: unc-51 like autophagy activating kinase 1; λ-PPase: lambda protein phosphatase.
Introduction
Autophagy is a highly conserved catabolic process among eukaryotes wherein double-membraned autophagosomes enclose damaged organelles or superfluous cellular components and deliver them to vacuoles or lysosomes for degradation [Citation1]. In the light of its delivery mechanisms and cargo specificity, autophagy can be divided into either nonselective autophagy, in which cytoplasmic components are degraded in a relatively nonselective manner, or selective autophagy, which requires the recognition of autophagic substrates by specific receptors that transport the sorted cargos to autophagosomes for degradation within vacuoles or lysosomes [Citation2]. Many types of selective autophagy have been described in literature, such as ribophagy, reticulophagy, mitophagy, and xenophagy [Citation2]. Autophagy is thus essential for the maintenance of cellular homeostasis, and dysfunction in autophagy has been closely linked to the onset and development of numerous major human diseases [Citation3].
In yeast, the formation of autophagosomes requires synergistic activity of autophagy-related (ATG) proteins at the phagophore assembly site (PAS) [Citation4]. The Atg1 complex, comprised of Atg1, Atg13, Atg17, Atg29, and Atg31, is a key regulator of autophagosome formation [Citation1]. In particular, the activity of the protein kinase Atg1 is essential for both selective and nonselective autophagy, and is activated under rapamycin treatment or starvation conditions [Citation1,Citation5]. Both Atg1 and its homolog in mammals, ULK1 (unc-51 like autophagy activating kinase 1), have been found to initiate autophagy via phosphorylating a series of Atg proteins. In yeast, Atg1 phosphorylates Atg9, which is a key step in the recruitment of Atg18 and Atg8 to the PAS [Citation6]. Recently, Atg1 was shown to also phosphorylate Atg4 to specifically inhibit its Atg8 deconjugating activity [Citation7,Citation8]. In mammals, ULK1 also phosphorylates several ATG proteins as part of its regulation of autophagy. For example, BECN1/beclin1 and ATG14, two subunits of the PIK3C3-containing complex, can be phosphorylated by ULK1 to activate the complex, thereby promoting autophagosome formation [Citation9,Citation10]. ATG16L1, an essential autophagic protein that forms a trimeric E3-like enzyme with ATG12–ATG5, has also been reported to serve as an important phosphorylation substrate of ULK1 in bacterial clearance via ATG16L1 recruitment to Salmonella infection sites [Citation11]. However, compared with nonselective autophagy, the regulatory mechanisms through which Atg1 controls selective autophagy remain elusive.
Different from Atg1, Atg11 functions as a scaffold protein that associates with a variety of receptors to regulate different types of selective autophagy [Citation12]. Atg11 has four coil-coiled domains including CC1, CC2, CC3 and CC4. The CC2 and CC3 domains are responsible for the binding of Atg11 to Atg1, which facilitates the transport of cargos to autophagosomes [Citation12]. The CC4 domain binds to selective receptor proteins such as Atg19 to mediate the delivery of various substrates to vacuoles [Citation13–17]. Based on this role, Atg11 serves as a marker protein for selective autophagy, although the molecular mechanisms regulating its activity in selective autophagy remain unclear.
In this study, we show that Atg11 is a direct phosphorylated substrate of Atg1. Our results demonstrate a strong link between Atg11 phosphorylation by Atg1 and Atg11 association with selective autophagy receptors, leading us to propose that Atg1-mediated phosphorylation of Atg11 is required for the induction of selective autophagy via regulation of Atg11 binding with its cognate receptor proteins.
Results
Atg11 is phosphorylated under autophagy induction conditions
In order to better understand the molecular mechanisms by which Atg11 contributes to selective autophagy, we fused a 3×HA tag to the C terminus of Atg11 in the yeast genome to detect its expression under autophagy induction and nutrient-rich conditions. Western blot analysis showed that under nutrient-rich conditions, Atg11 protein in cells treated with the TOR inhibitor rapamycin exhibited an obvious upward shift (), indicating that the Atg11 protein had increased in size possibly due to post-translational modification. Subsequently, we explored whether this modification also occurs under other autophagy-inducing conditions. We found that, in addition to rapamycin treatment, either nitrogen or glucose starvation resulted in comparable upward shifts of the Atg11 protein band (), suggesting it to be a general phenomenon triggered by various autophagy-inducing conditions, rather than a rapamycin-specific induction.
Figure 1. Atg11 is phosphorylated under autophagy induction conditions. (A) Yeast cells expressing Atg11-3×HA were grown to log phase in nutrient-rich medium (full medium), and then were treated with rapamycin for 30 min, Atg11-3×HA was detected by anti-HA antibody. (B) Yeast cells expressing Atg11-3×HA were subjected to rapamycin treatment, nitrogen starvation (SD-N), or glucose starvation (SD-G), Atg11-3×HA was detected by anti-HA antibody. (C) Yeast cells expressing Atg11-3×HA were subjected to rapamycin treatment or SD-N. Cell lysates were treated with λ protein phosphatase (λ-PPase) in the presence or absence of phosphatase inhibitors for 0.5 h at 30°C. Atg11-3×HA was detected by anti-HA antibody. Pgk1 served as a loading control.
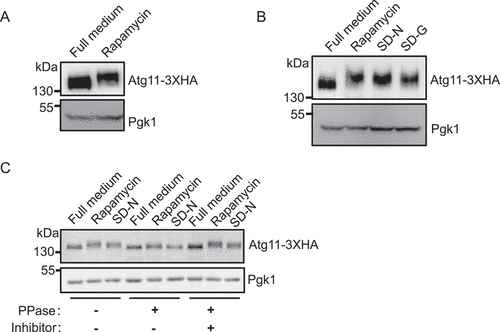
Based on previous literature that showed that phosphorylation could lead to the upward movement of protein bands and is a common regulatory mechanism for autophagic processes [Citation18], we hypothesized that the observed upward migration of Atg11 protein bands was likely due to phosphorylation. To test this possibility, cells expressing Atg11-3×HA were cultured in a nutrient-rich medium with or without rapamycin or under nitrogen starvation conditions. Since Lambda protein phosphatase (λ-PPase) can cleave phosphate moieties from phosphorylated serine, threonine, and tyrosine residues [Citation19], cell lysates were then treated or untreated with λ-PPase. The results showed that λ-PPase treatment largely abolished the shift of Atg11 bands induced by rapamycin and nitrogen starvation (SD-N), suggesting that phosphorylation was the causative modification responsible for the band shift of Atg11 (). Taken together, these results led us to conclude that Atg11 is phosphorylated under autophagy induction conditions.
Atg11 is a direct phosphorylated substrate of Atg1
We next sought to identify the protein kinase(s) that phosphorylates Atg11. Previous reports have shown that several protein kinases, including Snf1/AMPK, MTORC1, PRKA/PKA (protein kinase cAMP-activated), Atg1/ULK1, and Hrr25, all participate in the regulation of autophagy by phosphorylating different autophagy-related proteins [Citation6,Citation19–22]. The size increase of the Atg11 protein under SD-G, SD-N, and rapamycin treatment conditions indicated that the protein kinase was activated under autophagy-inducing conditions. Because MTORC1 and PRKA are known to be inactivated under nitrogen starvation, we excluded MTORC1 and PRKA as candidates for mediating upward migration of the Atg11 protein band [Citation20,Citation22]. Similarly, we found that Snf1/AMPK is inactive under exposure to rapamycin or nitrogen-starvation conditions, which indicated that Snf1 was also not involved in the size increase of the Atg11 protein (Figure S1A). Because Hrr25 was reported to phosphorylate Atg19, Atg34, and Atg36 to regulate corresponding selective autophagy [Citation19,Citation23], we examined whether Hrr25 could also phosphorylate Atg11 during the induction of autophagy. Because Hrr25 protein kinase activity is essential for yeast cell growth, we used an auxin-inducible degron system to degrade Hrr25 [Citation24]. As shown in Figure S1B, C, the degradation of Hrr25 protein severely impaired cell growth, but did not block the upward movement of the Atg11 protein band under the rapamycin treatment conditions.
In the light of previous findings that Atg1 can directly associate with Atg11, and that Atg1 is activated under SD-N, SD-G, and rapamycin treatment conditions [Citation12,Citation21], we speculated that Atg1 could serve as a likely candidate protein kinase for Atg11 phosphorylation. To test this hypothesis, we knocked out the ATG1 gene in yeast cells with endogenous expression of Atg11-3×HA. Western blots showed that the upward shift in Atg11 electrophoretic mobility under rapamycin treatment was lost in atg1∆ cells (). To further confirm that the Atg11 size increase was caused by Atg1 kinase activity, we generated an Atg1 kinase dead (KD) mutant construct and then individually introduced the empty vector, wild-type (WT) Atg1, or KD vector into atg1∆ yeast cells expressing Atg11-3×HA. The results indicated that WT Atg1, but not the Atg1 KD variant, could restore the upward shift in the Atg11 protein mobility under rapamycin treatment (). To further assess whether Atg1 kinase activity was required for phosphorylation of Atg11 during the induction of autophagy, we deleted ATG13 in yeast cells expressing Atg11-3×HA, since Atg13 has been shown to be necessary for Atg1 kinase activity under autophagy induction conditions [Citation20]. Consistent with our observations in atg1∆ cells expressing the Atg1 KD mutant, no upward shift of Atg11 was observed in the absence of ATG13 under rapamycin treatment condition. By contrast, western blot analysis showed that Atg11 was modified under rapamycin treatment in WT cells expressing Atg11-3×HA with knockout of three genes, ATG5, ATG9, and ATG14, which do not have known effects on Atg1 kinase activity but are essential for autophagy (), S1D). These cumulative results thus provided strong evidence that Atg1 phosphorylates Atg11 under autophagy induction conditions.
Figure 2. Atg11 is a direct phosphorylated substrate of Atg1. (A) Wild type (WT) or atg1∆ cells expressing Atg11-3×HA were grown to log phase in a nutrient-rich medium (full medium), and then were treated with or without rapamycin for 30 min. Atg11-3×HA was detected by anti-HA antibody. (B) atg1∆ yeast cells co-expressing Atg11-3×HA and empty vector, Wild-type Atg1-3×FLAG (WT), or Atg1 D211A-3×FLAG (kinase dead, KD) was subject to rapamycin treatment for 30 min. Atg11-3×HA and Atg1-3×FLAG proteins were detected by the corresponding antibody. (C) Wild type (WT), atg1∆, or atg13∆ yeast cells expressing Atg11-3×HA were subject to rapamycin treatment for 30 min. Atg11-3×HA was detected by anti-HA antibody. (D) Wild-type (WT), atg1∆, atg5∆, and atg14∆ yeast cells expressing Atg11-3×HA were subject to rapamycin treatment for 30 min. Atg11-3×HA was detected by anti-HA antibody. Pgk1 served as a loading control.
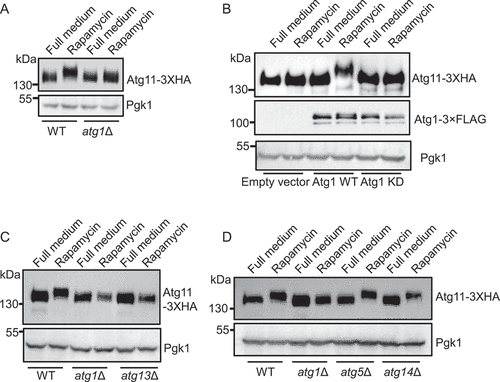
S949, S1057, and S1064 residues on Atg11 are phosphorylated by Atg1
We next investigated which site(s) on Atg11 are phosphorylated by Atg1. It has been reported that Atg11 protein contains four conserved functional domains, namely CC1, CC2, CC3, and CC4 () [Citation12]. Since the molecular weight of Atg11 is approximately 135kDa, we divided it into four segments (R1, R2, R3, and R4) according to the regions containing these secondary structures: 1–321aa, 322–576aa, 577–859aa, and 860–1178aa, respectively. Subsequently, we separately cloned each segment into an E. coli expression vector and used in vitro kinase assays to detect which region of Atg11 could be phosphorylated by Atg1. The results showed that the Atg11 R4 region was specifically phosphorylated by Atg1 (). To further demonstrate that Atg1 kinase activity was essential for phosphorylation of the Atg11 R4 region, we used immunoprecipitated WT or KD Atg1 (D211A) protein kinases from yeast cells with R4 as a substrate in in vitro kinase assays. In line with our above results, we found that WT Atg1, but not KD Atg1 could phosphorylate the Atg11 R4 (), further confirming that Atg11 is a direct phosphorylation substrate of Atg1.
Figure 3. S949, S1057 and S1064 residues on Atg11 are phosphorylated by Atg1. (A) Secondary structure diagram of Atg11 protein. (B) GST, GST-Atg11-R1, GST-Atg11-R2, GST-Atg11-R3, and GST-Atg11-R4 were purified from E. coli via a GST column. Atg1-3×FLAG were purified from rapamycin-treated yeast cells with anti-FLAG agarose beads. in vitro kinase assays were performed with GST, GST-Atg11-R1, GST-Atg11-R2, GST-Atg11-R3, or GST-Atg11-R4 as substrates and with Atg1-3×FLAG as protein kinase. The phosphorylation level of Atg11-R1, R2, R3, or R4 was detected using anti-thioP antibody. (C) in vitro kinase assays were performed with GST -Atg11-R4 purified from E. coli as substrates and purified Atg1-3×FLAG WT or Atg1-3×FLAG KD from rapamycin-treated yeast cells as protein kinase. The phosphorylation level of Atg11 R4 was detected using anti-thioP antibody. (D) Six typical Atg1 phosphorylation sites on Ag11 R4 domain. (E) in vitro kinase assays were performed using GST, GST-Atg11-R4. The six indicated GST-Atg11 R4 variants were purified from E. coli as substrates and purified Atg1-3×FLAG from rapamycin-treated yeast cell was used as a protein kinase. The phosphorylation level of Atg11 R4 and these variants was detected using anti-thioP antibody. (F) The phosphorylation levels of Atg11 R4 were quantified and presented as mean ± SD (n = 3). ***p < 0.001; **p < 0.01; NS, no significance; two-tailed Student’s t tests were used. (G) in vitro kinase assays were performed with Atg11 R4 WT or S949A S1057A S1064A (3A) protein as substrates, which were purified from E. coli, and purified Atg1-3×FLAG from rapamycin-treated yeast cell as a protein kinase. The phosphorylation level of Atg11 R4 WT and 3A was detected using anti-thioP antibody. (H) Atg11-3×HA wild type (WT) or 3A yeast cells, and atg1∆ yeast cells expressing Atg11-3×HA were grown to log phase in nutrient-rich medium (full medium), and then were subjected to rapamycin treatment, SD-N, or SD-G for 1 h. Atg11-3×HA was detected using anti-HA antibody. Pgk1 served as a loading control.
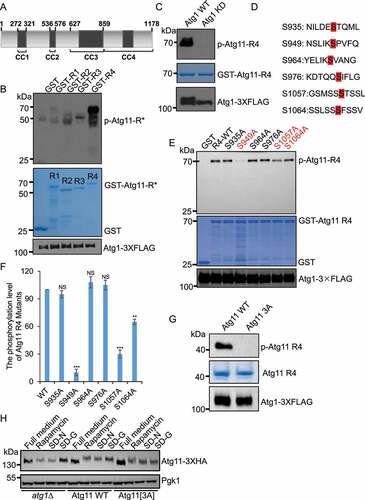
Next, to identify the specific phosphorylation site(s) of Atg1 on the Atg11 R4 region, we examined previous reports related to Atg1 which demonstrated the features of its phosphorylated substrates. These substrates were revealed to usually harbor a specific motif in which both aliphatic and aromatic residues are typically located at positions +1 and +2, with an aliphatic residue at position −3 [Citation6]. Searching for this peptide motif, we found six potential target motifs in the R4 region of Atg11 (). We then generated serine to alanine conversion mutants for each of these six sites. In vitro kinase assays showed that S949A, S1057A, and S1064A mutations led to decreased phosphorylation of Atg11, to varying degrees. In particular, the cells carrying the Atg11 S949A mutation exhibited severely impaired phosphorylation of Atg11, suggesting that S949 may serve as a primary site for phosphorylation by Atg1 ()). Furthermore, in vitro kinase assays revealed that an S949A S1057A S1064A (3A) triple mutant of the Atg11 R4 region completely abolished phosphorylation ().
Next, we introduced an S949A S1057A S1064A (3A) mutation into the genome of WT yeast cells to determine whether this variant affected the electrophoretic mobility of Atg11 under autophagy induction conditions. As shown in , the same as atg1∆, the Atg11[3A] mutant largely lost the upward shift in Atg11 electrophoretic mobility under rapamycin treatment, SD-N and SD-G conditions. Taken together, we concluded that S949, S1057 and S1064 residues in the CC4 region of Atg11 are phosphorylated by Atg1.
Atg11 phosphorylation by Atg1 is required for selective autophagy
As Atg11 is known to be required for selective autophagy [Citation12], we tested whether its phosphorylation by Atg1 is also required for selective autophagy. To this end, we examined whether the activation of the Cvt pathway requires the phosphorylation of Atg11 by Atg1 in atg11∆, WT Atg11, and Atg11[3A] yeast cells. Then, by using Ape1 precursor cleavage assays [Citation12], we found that Atg11[3A] cells decreased cleavage of Ape1 precursor at 2 h and 4 h of nitrogen starvation ()). Ams1, a vacuolar hydrolase, is another substrate of the Cvt pathway [Citation25]. We found that the accumulation of free GFP from an Ams1-GFP fusion reporter was significantly attenuated with increasing starvation time in the Atg11[3A] strain, compared with that of WT Atg11 strain ()).
Figure 4. Atg11 phosphorylation by Atg1 is required for selective autophagy. (A) atg11∆ yeast cells, Atg11-3×HA wild type (WT) or 3A yeast cells were grown to log phase in nutrient-rich medium (full medium), and then were subjected to nitrogen starvation for 0, 2 or 4 h. The maturation of Ape1 precursor was detected using anti-Ape1 antibody. (B) Quantification of the maturation of Ape1 from (A). (C-J) atg11∆ yeast cells, Atg11-3×HA wild type (WT) or 3A yeast cells expressing Ams1 (vacuolar α-mannosidase, another the Cvt pathway substrate), Sec63 (ER protein), Pex14 (peroxisomal protein), or Om45 (mitochondrial protein) fused with GFP tag were grown to log phase, and were then subjected to nitrogen starvation for 0, 4 or 8 h. The cleavage of the indicated fusion proteins was detected using anti-GFP antibody. Pgk1 served as a loading control. The degradation rates from corresponding image were quantified and presented as mean ± SD (n = 3). ***p < 0.001; **p < 0.01; *p < 0.05; NS, no significance; two-tailed Student’s t tests were used.
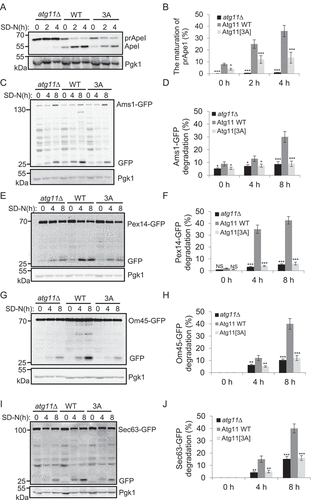
We next examined whether other selective autophagy pathways required Atg1-mediated phosphorylation of Atg11. Indeed, we observed selective autophagy processes including mitophagy, lipophagy, reticulophagy, ribophagy, and pexophagy through adding GFP tag to the C-terminal of the relevant degradation substrate in atg11∆, WT Atg11, and Atg11[3A] yeast cells [Citation14–17,Citation26,Citation27]. We found that except for ribophagy (where ribophagy does not seem to require Atg11), other detected types of selective autophagy were blocked to varying degrees by the Atg11[3A] mutation, among which pexophagy and mitophagy were the most affected (), S2A-D). Conversely, Atg11[3A] was confirmed as not being involved in nonselective autophagy (Figure S2E). Taken together, these results indicated that phosphorylation of Atg11 by Atg1 is generally required for Atg11-dependent selective autophagy.
Atg11 phosphorylation by Atg1 is required for its association with selective autophagy receptors
Given that the Atg11 CC4 domain was shown to be responsible for the association of Atg11 with selective autophagy receptors prompted us to check whether Atg1 phosphorylation of Atg11 is involved in their interaction. Atg19 is a receptor for the Cvt pathway and transports Ape1 into the autophagosome via Atg11 binding [Citation12]. We therefore introduced a plasmid expressing 3×FLAG-Atg19 into yeast cells with chromosomal expression of HA-Atg11or HA-Atg11[3A]. Immunoprecipitation and quantitative results showed that Atg19 association with Atg11 was impaired in the HA-Atg11[3A] mutant cells, regardless of growth on either nutrient-rich or nitrogen starvation conditions (, S3A).
Figure 5. Atg11 phosphorylation by Atg1 regulates its association with selective receptors. (A-F) atg11∆ yeast Cells co-expressing HA-Atg11 wild type (WT) or 3A with the indicated receptor proteins fused with 3×FLAG tag was grown to log phase, then subject to SD-N for 0, 1, or 2 h. Cell lysates were immunoprecipitated with anti-FLAG agarose beads and then analyzed by western blot using an anti-HA antibody.
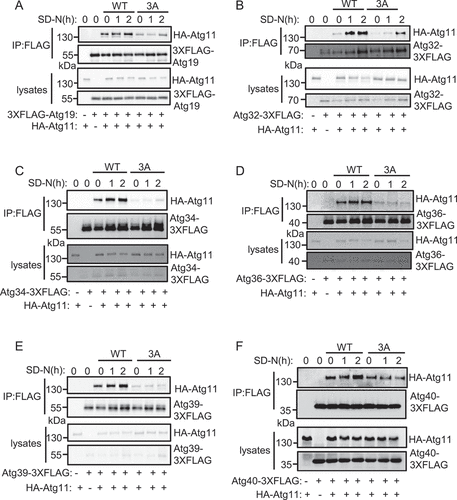
Next, to assess whether the Atg11 phosphorylation is necessary for its association with other selective autophagy receptors, we checked for Atg11 coimmunoprecipitation with the reticulophagy receptors Atg39 and Atg40, pexophagy receptor Atg36, mitophagy receptor Atg32, and another Cvt pathway receptor Atg34 in the Atg11[3A] mutant [Citation14–17,Citation28]. The results showed that interaction between these receptor proteins and Atg11 was severely limited in the HA-Atg11[3A] mutant under nitrogen starvation conditions (), S3B-F).
Since Atg1 phosphorylates Atg11, we also examined whether the kinase activity of Atg1 was required for the association of Atg11 with selective receptors. A previous report has shown that the Atg1 kinase dead mutant diminishes the interaction between Atg19 and Atg11 [Citation23]. Here, we selected other selective autophagy receptors including Atg32, Atg34, Atg36, Atg39, and Atg40 to detect their binding to Atg11 in the presence of WT or KD Atg1 plasmids. Immunoprecipitation results showed that Atg11 association with these receptors was severely impaired in the Atg1 KD yeast cells (Figure S4). Taken together, we concluded that the kinase activity of Atg1 is required for the association of Atg11 with selective autophagy receptors.
Discussion
In this study, we identified Atg11 as a direct phosphorylation substrate of Atg1. By demonstrating that the Atg1 phosphorylation of Atg11 at S979, S1046, and S1054 residues is required for interaction with different selective autophagy receptor proteins, our results reveal a close link between the Atg1 regulation of Atg11-mediated induction of selective autophagy ().
Figure 6. Model for the regulation of selective autophagy by Atg1-mediated Atg11 phosphorylation. In response to nitrogen starvation, Atg1 is activated and then phosphorylates Atg11. Phosphorylated Atg11 is required for selective autophagy by regulating the association of Atg11 with selective receptor proteins. P, phosphorylated.
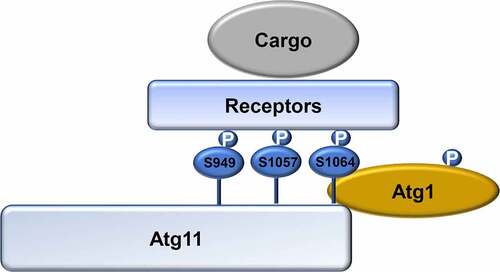
Compared with the mechanisms of nonselective autophagy, it has remained relatively unclear what specific functions of Atg1 have on the regulation of selective autophagy. Previous studies have shown that ULK1 phosphorylates the mitophagy receptor FUNDC1 to regulate mitophagy induced by hypoxia or the mitochondrial damage-inducing agent CCCP [Citation29]. ULK1 was also found to phosphorylate P62 to promote selective autophagy under proteotoxic stress [Citation30]. Atg11 serves as a scaffold protein that plays a major role in selective autophagy via direct binding with different autophagy-related proteins at specific recognition sites [Citation12]. However, the role of the post-translational modification of Atg11 in the regulation of selective autophagy has not been previously reported. Here, we found that Atg1 phosphorylates Atg11 under autophagy-inducing conditions, and that this phosphorylation occurs in the Atg11 CC4 domain specifically, which is responsible for its binding with selective autophagy receptors. This finding deepens our understanding of how Atg11 regulates selective autophagy.
In yeast, Atg11 association with receptor proteins is a key step in initiating selective autophagy [Citation12]. Previous studies have shown that the protein kinase Hrr25 phosphorylates the selective receptors Atg19, Atg34, and Atg36 to regulate their interactions with Atg11, thus enhancing selective autophagy. However, Hrr25 does not regulate mitophagy [Citation19]. It has been reported that the yeast mitophagy receptor Atg32 can be phosphorylated by casein kinase 2 (CK2) to stabilize Atg32-Atg11 binding. This leads to the assembly of core ATG protein machinery and thus activates mitophagy. However, CK2 is noted as not required for other targets of selective autophagy or bulk autophagy [Citation31]. Recently, some studies have found that the activation of Atg1 kinase requires the participation of Atg11. The activity of Atg1 kinase requires a multi-subunit complex containing protein aggregates, its autophagy receptor, and Atg11 under nutrient-rich conditions [Citation32]. In fission yeast, Atg1 kinase activity also requires Atg11 and is activated by Atg11-mediated dimerization [Citation33]. Naturally, the question arises whether the activity of Atg1 kinase regulates the function of Atg11. In this study, we found that Atg1 regulates selective autophagy by phosphorylating Atg11. This discovery expands our understanding of the regulatory mechanism underlying the binding between Atg11 and selective receptors. Despite these advances, it remains unknown whether there are other post-translational modifications in Atg11 that also affect its activity, or whether it is the substrate of other protein kinases. Atg11 performs multiple functions in selective autophagy, and our future and ongoing studies will further examine its functions in driving selective autophagy.
Materials and methods
Yeast strains, constructs and growth conditions
All yeast strains used in this work are listed in Table S1. The wild type (BY4741) and related deletion strains were purchased from Invitrogen (95,401.H2). The related strains were generated as described previously [Citation34], and verified by polymerase chain reaction (PCR) (Vazyme, P505-d1) or western blot analysis with the corresponding antibody. All mutant plasmids and strains in this work were sequenced. Yeast cells were cultured at 30°C in synthetic medium (SD; 0.17% yeast nitrogen base without amino acids and ammonium sulfate, 0.5% ammonium sulfate, 2% glucose, and corresponding auxotrophic amino acids and vitamins), or YPD (1% yeast extract, 2% peptone, and 2% glucose). For autophagy induction, cells were grown to mid-log phase in corresponding selective medium, and then were subjected to 0.2 µg/ml rapamycin (Selleck, AY-22989) treatment, nitrogen starvation (SD−N; 0.17% yeast nitrogen base without amino acids and ammonium sulfate, 2% glucose), or glucose starvation (SD-G; 0.17% yeast nitrogen base without amino acids and ammonium sulfate, 0.5% ammonium sulfate, 0.5% casamino acid) for the indicated time.
Antibodies
Antibodies were used at the indicated dilutions in this work as follows: anti-HA (Abmart, M20003L; 1:3000), anti-Pgk1 (Nordic Immunology, NE130/7S; 1:10,000), anti-FLAG (Sigma, F1804; 1:2500), anti-thiophosphate ester (Abcam, ab133473; 1:10,000), anti-GFP (Roche, 11,814,460,001; 1:2500), anti-phospho-PRKAA/AMPKα (Thr172; Cell Signaling Technology, 2535S; 1:2000), anti-Ape1 antibody was obtained from Dr. Zhiping Xie Lab (Shanghai Jiaotong University, China), goat anti-mouse IgG1, human ads-HRP (SouthernBiotech, 1070–05; 1:10,000), and goat anti-rabbit, human ads-HRP (SouthernBiotech, 4010–05; 1:10,000).
Western blots and immunoprecipitation
Yeast protein extraction, immunoblotting and immunoprecipitation experiments were performed as described methods previously [Citation21].
Auxin treatment
The corresponding yeast strains were grown to the early logarithmic phase, and then treated with 0.5 mM IAA (indole-3-acetic acid; Sigma, I2886) for 2 h to induce protein degradation.
In vitro phosphorylation assay and alkylation protocol
Cells expressing Atg1 WT-3ΧFLAG or Atg1 KD-3ΧFLAG in atg1∆ yeast strains were grown to the log phase, then treated with glucose starvation for 1 h. 50OD600 yeast cells were harvested and cell lysates were immunoprecipitated with anti-FLAG agarose beads (Sigma, A2220). Purified Atg1 WT-3×FLAG or Atg1 KD-3×FLAG were incubated with 5 µg purified protein from E. coli and 1.5 µl 10 mM ATP-γ-S (Sigma, A1388) in Atg1 kinase buffer (30 mM HEPES.KOH, pH 7.4, 5 mM NaF, 10 mM MgCl2, 1 mM DTT) at 30°C for 30 min, and then 2 µl 50 mM p-nitrobenzyl mesylate/PNBM (Abcam, ab138910) was added and incubated for another hour at 30°C. Finally, the reactions were stopped by boiling in protein loading buffer for 5 min. The phosphorylation level of substrate was detected by anti-thiophosphate ester (Abcam, ab92570).
Construction and purification of GST-Atg11 R1, R2, R3 and R4
The fragments of R1, R2, R3 and R4 were in positions aa 1–321, aa 322–576, aa 577–859 and aa 860–1178, respectively. Primers for the construction of R1, R2, R3 and R4 were designed and these fragments were cloned into pGEX4T-1 (Cytiva,71,002–626) by cutting BamHI and SalI. These constructions were identified by sequencing. The purification of GST-Atg11 R1, R2, R3 and R4 proteins were performed as described methods previously [Citation35].
Quantitative and statistical analysis
Western-blot images from three independent experiments were quantified using ImageJ software. Maturation of Ape1 was quantified by the ratio of band intensities of Ape1 to that of total Ape1 (Ape1 + prApe1). GFP intensities were divided by the sum of GFP and the GFP-fused protein intensities to calculate the degradation rate of the fused protein. Statistical analyses were presented as mean ± SD (n = 3). ***p < 0.001; **p < 0.01; *p < 0.05; NS, no significance; two-tailed Student’s t tests were used.
Supplemental Material
Download MS Word (779.3 KB)Acknowledgments
We are grateful to Prof. Y. Ohsumi, Prof. H. Nakatogawa and Prof. Zhiping Xie for the plasmids, antibodies and yeast strains. We also thank for the support of the Protein facility, Core facilities, Zhejiang University School of Medicine. The research was supported by National Natural Science Foundation of China (Grant No: 32122028, 32070739, 31771528 and 91754107), Zhejiang Provincial Natural Science Foundation of China under Grant No.LR21C070001 and the National Basic Research Program of China (2017YFA0503402) to Cong Yi, and National Natural Science Foundation of China (Grant No: 32100600) to Weijing Yao.
Disclosure statement
No potential conflict of interest was reported by the authors.
Supplementary material
Supplemental data for this article can be accessed here.
Additional information
Funding
References
- Ohsumi Y. Historical landmarks of autophagy research. Cell Res. 2014;24(1):9–23.
- Feng Y, He D, Yao Z, et al. The machinery of macroautophagy. Cell Res. 2014;24(1):24–41.
- Levine B, Kroemer G. Biological functions of autophagy genes: a disease perspective. Cell. 2019;176(1–2):11–42.
- Shibutani ST, Yoshimori T. A current perspective of autophagosome biogenesis. Cell Res. 2014;24(1):58–68.
- Matsuura A, Tsukada M, Wada Y, et al. Apg1p, a novel protein kinase required for the autophagic process in Saccharomyces cerevisiae. Gene. 1997;192(2):245–250.
- Papinski D, Schuschnig M, Reiter W, et al. Early steps in autophagy depend on direct phosphorylation of Atg9 by the Atg1 kinase. Mol Cell. 2014;53(3):471–483.
- Sanchez-Wandelmer J, Kriegenburg F, Rohringer S, et al. Atg4 proteolytic activity can be inhibited by Atg1 phosphorylation. Nat Commun. 2017;8(1):295.
- Pengo N, Agrotis A, Prak K, et al. A reversible phospho-switch mediated by ULK1 regulates the activity of autophagy protease ATG4B. Nat Commun. 2017;8(1):294.
- Park JM, Seo M, Jung CH, et al. ULK1 phosphorylates Ser30 of BECN1 in association with ATG14 to stimulate autophagy induction. Autophagy. 2018;14(4):584–597.
- Park JM, Jung CH, Seo M, et al. The ULK1 complex mediates MTORC1 signaling to the autophagy initiation machinery via binding and phosphorylating ATG14. Autophagy. 2016;12(3):547–564.
- Alsaadi RM, Losier TT, Tian W, et al. ULK1-mediated phosphorylation of ATG16L1 promotes xenophagy, but destabilizes the ATG16L1 Crohn’s mutant. EMBO Rep. 2019;20(7):e46885.
- Yorimitsu T, Klionsky DJ. Atg11 links cargo to the vesicle-forming machinery in the cytoplasm to vacuole targeting pathway. Mol Biol Cell. 2005;16(4):1593–1605.
- Leber R, Silles E, Sandoval IV, et al. Yol082p, a novel CVT protein involved in the selective targeting of aminopeptidase I to the yeast vacuole. J Biol Chem. 2001;276(31):29210–29217.
- Okamoto K, Kondo-Okamoto N, Ohsumi Y. Mitochondria-anchored receptor Atg32 mediates degradation of mitochondria via selective autophagy. Dev Cell. 2009;17(1):87–97.
- Kanki T, Wang K, Cao Y, et al. Atg32 is a mitochondrial protein that confers selectivity during mitophagy. Dev Cell. 2009;17(1):98–109.
- Motley AM, Nuttall JM, Hettema EH. Pex3-anchored Atg36 tags peroxisomes for degradation in Saccharomyces cerevisiae. EMBO J. 2012;31(13):2852–2868.
- Mochida K, Oikawa Y, Kimura Y, et al. Receptor-mediated selective autophagy degrades the endoplasmic reticulum and the nucleus. Nature. 2015;522(7556):359–362.
- Kamada Y, Yoshino K-I, Kondo C, et al. Tor directly controls the Atg1 kinase complex to regulate autophagy. Mol Cell Biol. 2010;30(4):1049–1058.
- Tanaka C, Tan L-J, Mochida K, et al. Hrr25 triggers selective autophagy-related pathways by phosphorylating receptor proteins. J Cell Biol. 2014;207(1):91–105.
- Kamada Y, Funakoshi T, Shintani T, et al. Tor-mediated induction of autophagy via an Apg1 protein kinase complex. J Cell Biol. 2000;150(6):1507–1513.
- Yi C, Tong J, Lu P, et al. Formation of a Snf1-Mec1-Atg1 module on mitochondria governs energy deprivation-induced autophagy by regulating mitochondrial respiration. Dev Cell. 2017;41(1):59–71 e4.
- Filteau M, Diss G, Torres-Quiroz F, et al. Systematic identification of signal integration by protein kinase A. Proc Natl Acad Sci U S A. 2015;112(14):4501–4506.
- Pfaffenwimmer T, Reiter W, Brach T, et al. Hrr25 kinase promotes selective autophagy by phosphorylating the cargo receptor A tg19. EMBO Rep. 2014;15(8):862–870.
- Nishimura K, Fukagawa T, Takisawa H, et al. An auxin-based degron system for the rapid depletion of proteins in nonplant cells. Nat Methods. 2009;6(12):917–922.
- Hutchins MU, Klionsky DJ. Vacuolar localization of oligomeric alpha-mannosidase requires the cytoplasm to vacuole targeting and autophagy pathway components in Saccharomyces cerevisiae. J Biol Chem. 2001;276(23):20491–20498.
- van Zutphen T, Todde V, de Boer R, et al. Lipid droplet autophagy in the yeast Saccharomyces cerevisiae. Mol Biol Cell. 2014;25(2):290–301.
- Kraft C, Deplazes A, Sohrmann M, et al. Mature ribosomes are selectively degraded upon starvation by an autophagy pathway requiring the Ubp3p/Bre5p ubiquitin protease. Nat Cell Biol. 2008;10(5):602–610.
- Suzuki K, Kondo C, Morimoto M, et al. Selective transport of alpha-mannosidase by autophagic pathways: identification of a novel receptor, Atg34p. J Biol Chem. 2010;285(39):30019–30025.
- Wu W, Tian W, Hu Z, et al. ULK 1 translocates to mitochondria and phosphorylates FUNDC 1 to regulate mitophagy. EMBO Rep. 2014;15(5):566–575.
- Lim J, Lachenmayer ML, Wu S, et al. Proteotoxic stress induces phosphorylation of p62/SQSTM1 by ULK1 to regulate selective autophagic clearance of protein aggregates. PLoS Genet. 2015;11(2):e1004987.
- Kanki T, Kurihara Y, Jin X, et al. Casein kinase 2 is essential for mitophagy. EMBO Rep. 2013;14(9):788–794.
- Kamber RA, Shoemaker CJ, Denic V. Receptor-bound targets of selective autophagy use a scaffold protein to activate the Atg1 kinase. Mol Cell. 2015;59(3):372–381.
- Pan ZQ, Shao G-C, Liu X-M, et al. Atg1 kinase in fission yeast is activated by Atg11-mediated dimerization and cis-autophosphorylation. eLife. 2020;9:e58073.
- Janke C, Magiera MM, Rathfelder N, et al. A versatile toolbox for PCR-based tagging of yeast genes: new fluorescent proteins, more markers and promoter substitution cassettes. Yeast. 2004;21(11):947–962.
- Yao W, Li Y, Wu L, et al. Atg11 is required for initiation of glucose starvation-induced autophagy. Autophagy. 2020;16(12):2206–2218.