ABSTRACT
This study aimed to investigate the relationship between ZNF582 promoter methylation (ZNF582m) level and radiosensitivity of cervical cancer and its biological basis. This was a prospective multicenter clinical study, comprising two independent cohorts of locally advanced cervical cancer patients. Exfoliated cervical cells were collected at 0, 24, 30, 36, 48, and 64 Gy to test ZNF582m levels. Radiotherapy response was evaluated according to RECIST Version. RT-PCR and WT were used to detect the mRNA and protein expression levels; MTT and flow cytometry were used to detect the cell viability and cell cycle, respectively. While clone formation and subcutaneous tumorigenesis in nude mice were used to detect the growth of HeLa cells with/without ZNF582 overexpression. In the first cohort, 22 cases achieved complete remission (CR) or partial response (PR), and the other 28 cases exhibited stable disease (SD). Radiotherapy reduced ZNF582m levels among all patients. Initial lever of ZNF582m was significantly higher in the Responder (CR + PR) group than in the SD group. Also, patients with higher initial lever ZNF582m were more sensitive towards radiotherapy than ZNF582m-low patients. The second cohort confirmed the above results. The amplitude of ZNF582m levels were related to the radiotherapeutic response; some patients of ZNF582m-low showed a transient increase in ZNF582m, and present greater radiosensitivity than other ZNF582m-low patients. In vitro, ZNF582 protein overexpression promoted cell cycle arrest in S phase. These results suggested that higher ZNF582m levels predicted greater radiosensitivity in clinical cervical cancer cases. Overexpressed ZNF582 conferred radioresistance by cell cycle arrest in vitro.
Introduction
Invasive cervical cancer is a leading cause of cancer death in women worldwide [Citation1,Citation2]. More than 85% of new cases and 90% deaths occurred in developing countries [Citation3,Citation4]. A large proportion of new cases in developing countries are already at an advanced stage (IB2 or more) at diagnosis [Citation5,Citation6]. Most advanced cervical cancer cases receive external beam radiotherapy and brachytherapy with or without chemotherapy as standard treatment [Citation6–8]. Radiotherapy has been used as a cancer treatment for more than a century, and with continued progress in precision delivery, the tumour-targeted dose has greatly increased. However, adverse reactions such as radiation vaginitis, cystitis, and proctitis seriously affect the quality of life of patient [Citation9]. These side effects may be exacerbated by radiotherapy resistance, indeed, resistance to radiotherapy accounts for most therapeutic failures in cervical cancer patients [Citation10,Citation11]. These failures highlight the necessity of developing personalized radiotherapy treatments for cervical cancer and identify reliable biomarkers for predicting treatment response.
Zinc Finger Protein 582 (ZNF582) is located on chromosome 19 that encodes a nuclear protein which contains one KRAB-A-B domain and nine zinc-finger motifs. ZNF582 protein is predicted to be an intracellular protein transcription factor, and ZNF582 gene may also be involved in DNA damage response, proliferation, cell cycle control, and tumour transformation [Citation12,Citation13]. In 2012, ZNF582 promoter was found to be hypermethylated in cervical cancer tissues for the first time [Citation14]. Our previous studies have shown that ZNF582 promoter was hypermethylated in cervical cancer [Citation15–19] and ZNF582 promoter hypermethylated cervical adenocarcinoma patients demonstrated better prognosis [Citation16]. We also found that ZNF582 promoter methylation levels were reduced in concurrent chemoradiotherapy (CCRT) patients compared with that in non-CCRT patients in cervical adenocarcinoma patients [Citation19]. However, the relationship between ZNF582 promoter methylation level and chemoradiotherapy sensitivity in cervical cancer and its potential mechanism are still unclear.
Materials and methods
Study design and patients cohort
This was a prospective multicenter study conducted between October 2017 and May 2019. The subject was composed of two cohorts with newly diagnosed invasive cervical cancer. The inclusion criteria were as follows: (1) histologically confirmed cervical cancer, (2) clinical stages IB2-IVA (FIGO 2009), (3) no prior anti-cancer treatment, and (4) available pretreatment computed tomography (CT) scan. Exclusion criteria were as follows: (1) patients with a history of previous chemotherapy or radiotherapy, (2) patients with a diagnosis of other cancers, or (3) patients with distant metastatic disease (para-aortic nodes involvement was not included).
All patients received image-guided external beam radiotherapy (EBRT) and brachytherapy (BT) with a total dose of 85–90 Gy (EQD2, equivalent dose in 2 Gy single-dose fractions). EBRT used 3D conformal technology at a dose of 1.8–2.0 Gy/fraction with a dose range of 45–50 Gy, while BT boost was volumetrically planned and delivered as weekly high-dose-rate fractions of 8 Gy EQD2 each after 15 times of EBRT. If feasible, cisplatin (40 mg/m2) chemotherapy or carboplatin (AUC = 2) were given simultaneously every week for 4–6 weeks. Cases were divided into the following two stages:
The first stage: cervical exfoliated cells were collected at 0, 24, 30, 36, 48, and 64 Gy, respectively. The cells were centrifuged and stored in phosphate-buffered saline (PBS) at −20°C immediately.
The response to radiotherapy was evaluated by two skilled radiologists based on Response Evaluation Criteria in Solid Tumours (RECIST) Version 1.1 with the following specific standards: 1. complete remission (CR), defined as disappearance of all target foci; 2. partial response (PR), defined as a ≥30% reduction in total lesion diameter from baseline; 3. stable disease (SD), defined as an increase of <20% or a reduction of <30% in total lesion diameter from baseline; 4. progressive disease (PD), defined as a ≥20% increase in total lesion diameter from baseline. ROC curve analysis to determine the best cut-off value of ZNF582 methylation levels for discerning Responder (CR + PR) group from SD and PD groups.
In the second stage, we confirmed the research result by an independent cohort at three centres (Hunan Cancer Hospital, Shandong Cancer Hospital, and Liaoning Cancer Hospital).
The study protocol was approved by the Institutional Review Board of Hunan Cancer Hospital.
DNA preparation
Genomic DNA (gDNA) was extracted from the collected cells using the QIAamp DNA Mini Kit (Qiagen GmbH, Hilden, Germany). A BioSpec-nano spectrophotometer (Shimadzu Corporation, Tokyo, Japan) was used to quantify the amount of extracted DNA.
ZNF582 promoter methylation tests
DNA was subjected to bisulphite conversion using the EZ DNA Methylation-Gold™ Kit (Zymo Research, CA, USA). The methylation levels of ZNF582 were determined using the qPCR kit (Hongya Gene Technology Co., Ltd.) and calculated the methylation index (M-index) using the formula: 10,000 × 2 [(Cp of COL2A) – (Cp of ZNF582)], the type II collagen gene (COL2A) was designed as the internal reference and tested with each specimen. The crossing point (Cp) value for COL2A, which is also the validity indicator of the test, should not be >35. For each sample, two Cp values were obtained: one from ZNF582 and another from COL2A [Citation16,Citation19].
Establishment of ZNF852 overexpression cell line
HeLa (CLS Cat# 300,194/p772_HeLa, RRID:CVCL_0030) cells were transfected with 20 μg of ZNF582 adenoviral vector (Vigene Biosciences, Rockville, MD, USA; NM_144690) or controlled adenoviral vector (Vigene Biosciences, Rockville, MD, USA; pLent-GFP-Puro-CMV). The transferred cells were then selected with puromycin.
Cell viability assay
Cultured cells in the logarithmic growth phase were harvested using trypsin and seeded onto 96-well plates at 5000 cells/well. The culture medium was aspirated off, and 100 μL XTT solution (20-300-1000, Biological Industries) was added at the indicated times, and incubated at 37°C for 3 hours. Optical density values were measured at 450 nm as an estimate of viable cell number using a microplate reader.
Cell cycle analysis by flow cytometry
Cells were collected and washed with cold PBS, fixed in 70% ethanol, and stored at 4°C overnight. The fixed cells were washed with PBS and stained with 500 μL propidium iodide (PI; BD Biosciences, Franklin Lakes, NJ, USA) in dark conditions for 30 minutes. The distribution of cell cycle stages was measured by flow cytometry within 1 hour. Cell debris and fixation artefacts were gated out.
Quantitative RT-PCR (Q-PCR)
Total RNA was extracted from cultured cells using Trizol (Invitrogen, Carlsbad, CA, USA) and reverse transcribed into cDNA using oligo (dT) primers and a cDNA synthesis kit (Invitrogen, USA) according to the manufacturer’s protocol. Gene expression was assessed by qRT-PCR using SYBR Premix Dimer Eraser (Perfect Real-Time) assay kits and the following primers:
GAPDH-forward, 5';-GAAGGTGAAGGTCGGAGTC-3';
GAPDH-reverse, 5'-GAAGATGGTGATGGGATTTC-3';
ZNF582-forward 5'-GAGGAGGCGGCAGCTCTACC;
ZNF582-reverse 5'-GAAACGGCAAGACCCAGTGAGAC.
Real-time PCR was performed using the Roche LC480 PCR System, and results were analysed using the comparative Cp method.
Western blotting and immunofluorescence assays
Crude cellular proteins were separated by SDS-PAGE and transferred to nitrocellulose membranes. Membranes were blocked in 5% non-fat milk and probed with primary antibodies against ZNF582 (ABclonal, A14453; 1:500), p21/Waf (Cell Signaling Technology, 9932kit, 1:1000), p27(KIP1) (Cell Signaling Technology, 9932kit, 1:1000), p21 (Cell Signaling Technology, 9932kit, 1:1000), CyclinD1 (Cell Signaling Technology, 9932kit, 1:1000), CDK2 (Cell Signaling Technology, 9932kit, 1:1000), CDK4 (Cell Signaling Technology, 9932kit, 1:1000), PI3K (Abcam, ab191606; 1:1000), Akt (Cell Signaling Technology, 9272, 1:1000), and α-tubulin (Abcam, ab7291, 1:5000) overnight at 4°C. Membranes were incubated with secondary antibodies for 1.5 hours and detected using a Bio-Rad imaging system.
Animal experiments
Female BALB/c nude mice (RRID:IMSR_ORNL:BALB/cRl) at 6 weeks of age were obtained from SLA Laboratory Animal (Changsha, China) and housed in a specific pathogen-free facility. Individual mice was subcutaneously injected with 5 × 106 HeLa cells. Four weeks after the inoculation, the mice were sacrificed and the tumours dissected out. The experimental protocol was approved by the Animal Care Committee of Hunan Cancer Hospital.
Statistical analysis
Statistical analyses were performed using SPSS (RRID:SCR_002865)18.0. Continuous variables are presented as mean ± standard deviation. Qualitative variables are described by frequencies and percentages. The comparison between groups was conducted by t-test, ANOVA, Chi-squared test, or non-parametric test (Mann–Whitney Test) (selected according to the data type) and a P-value of <0.05 was considered to indicate significance.
Results
Radiotherapy reduced ZNF582 promoter methylation levels
The first cohort included 50 patients with locally advanced cervical cancer who received concurrent chemoradiotherapy (CCRT) (n = 37) or radiotherapy alone (n = 13) (). The patients’ characteristics are summarized in . Cervical exfoliated cells were collected at 0, 24, 30, 36, 48, and 64 Gy doses (). All patients revealed a significant decline in ZNF582 promoter methylation levels (ZNF582m) during radiotherapy (). The tendency of ZNF582m levels during radiotherapy was consistent with squamous cell carcinoma antigen (SCC-Ag) ().
Figure 1. Study design and patient cohort for this study.
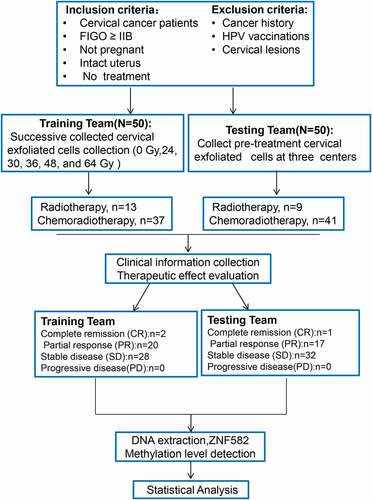
Figure 2. Changes in ZNF582 methylation level (ZNF582m) during radiotherapy.
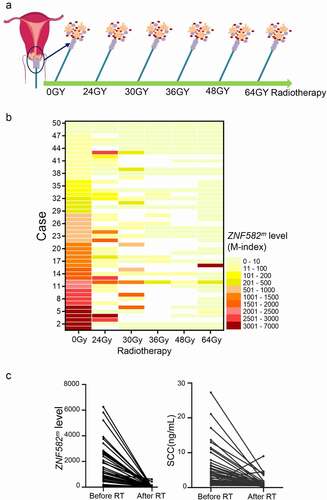
Table 1. Characteristics of ZNF582m-High and ZNF582m-Low subjects in the first cohort.
Higher ZNF582m predicted greater radiosensitivity
In the first group, 22 cases achieved a CR or PR (Responder group), whereas 28 exhibited SD (); ZNF582m was significantly higher in the Responder group than in the SD group, both in patients receiving radiotherapy only or chemoradiotherapy (). In ROC curve, a cut-off value of 1182 stratified Responder patients and SD patients with area under the curve (AUC) of 81.01%, sensitivity of 82.14%, and specificity of 72.73% ().
Figure 3. Patients with higher initial lever of ZNF582m were more sensitive towards radiotherapy than ZNF582m-low patients.
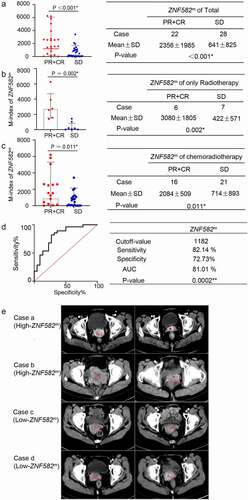
Among the 50 patients, 21 were defined as high-level ZNF582 methylation (ZNF582m-high) cases, while 29 defined as low-level ZNF582 methylation (ZNF582m-low) case according to ROC curve (). The ZNF582m-high patients were more sensitive to radiotherapy than ZNF582m-low patients, both in the entire cohort (76.2% vs. 20.7%) and in patients receiving radiotherapy only (85.7% vs. 0%) (). Representative computed tomography (CT) images are shown in .
Table 2. Comparison of the efficacy of radiotherapy in the first cohort with ZNF582m-High or ZNF582m-Low.
The above results were confirmed by an independent cohort; ZNF582m was significantly higher in the Responder group than in the SD group, not only in the entire cohort (n = 50, ) but also in the patients receiving radiotherapy only (n = 9, ) or chemoradiotherapy (n = 41, ). ROC curve acquired 84.38% sensitivity and 83.33% specificity (). The ZNF582m-high patients were more sensitive to radiotherapy than the ZNF582m-low patients (). The characteristics of the first cohort and second cohort are presented in
Figure 4. An independent cohort confirmed the patients with ZNF582m higher initial lever achieving better response than low baseline ZNF582m patients.
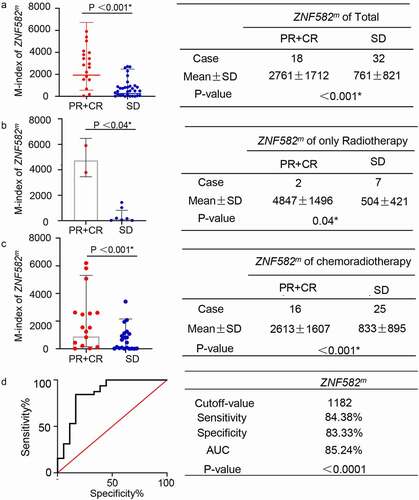
Table 3. Comparison of the efficacy of radiotherapy in the second cohort with ZNF582m-High or ZNF582m-Low.
Table 4. Characteristics of first cohort and second cohort subjects.
A transient increase of ZNF582m levels during radiotherapy predicted greater radiosensitivity in the ZNF582m-low cases
A subset of patients (5 of 29 cases) in ZNF582m-low group showed a transient increase of ZNF582m levels after receiving 24 Gy radiotherapy, representative cases are shown in . This suggested that radiation could increase ZNF582m levels and synergistically enhance the radiosensitivity. In the ZNF582m-high levels group, ZNF582m levels decreased rapidly in some cases (12 of 21 cases), while decreased slowly in others (). There was no significant difference in radiosensitivity between these two subgroups.
Figure 5. The amplitude of ZNF582m levels were related to radiotherapeutic response.
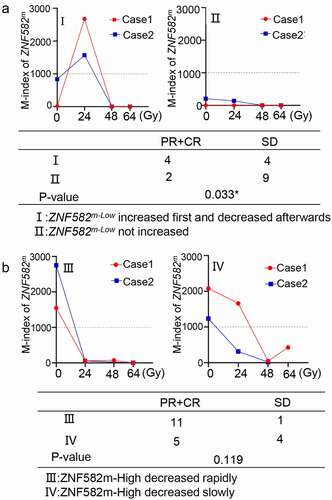
ZNF582 protein overexpression induced cell cycle arrest and radioresistance in HeLa cells
Our previous study demonstrated that ZNF582m-negative status was correlated with high ZNF582 protein expression, and ZNF582 overexpression increased the radiation and chemotherapy resistance of cervical cancer cells [Citation16]. To investigate the underlying mechanisms, we transfected ZNF582 into HeLa cells (). ZNF582 protein overexpressing significantly reduced cell proliferation (), colony formation () and tumour growth () of HeLa cell. Transcriptome sequencing showed that cell cycle-related pathways were significantly up-regulated in HeLa cells after ZNF582 protein overexpression (). The flow cytometry showed that the proportion of S phase was significantly increased and G1 phase decreased in the ZNF582 overexpressing HeLa cells (), suggesting that ZNF582 overexpression arrested the cell cycle in the S phase. Further, ZNF582 overexpressing HeLa cells demonstrated resistance to radiotherapy compared to control cells (). Western blotting revealed that ZNF582 overexpressing enhanced p27(KIP1), p21, CDK2, and CDK4 expression, and attenuated PI3K and Akt expression in HeLa cells (); and immunohistochemical analysis of mice tumour tissues confirmed these phenomena (), suggesting ZNF582 protein overexpression may induce S phase arrest by stimulating p27(KIP1)/p21/CDK2/4 signalling in HeLa cells. Cell cycle stage is associated with radiation sensitivity, with the highest sensitivity in G2/M phase and the lowest in S phase [Citation20–24]. Overexpressed ZNF582 may reduce cervical cancer radiosensitivity by arresting the cell cycle in S phase.
Figure 6. ZNF582 overexpression induced S phase arrest in Hela cells.
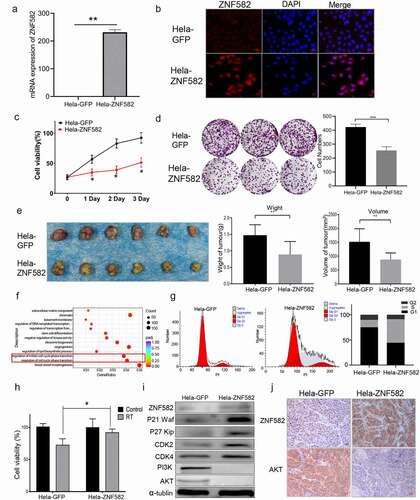
Discussion
Cervical cancer is one of the most common gynaecological malignancies in the world. It is estimated that 94 million cases will increase in low- and middle-income countries requiring external beam radiotherapy from 2015 to 2035, of which 70 million will also require treatment with brachytherapy [Citation25]. Chemoradiotherapy (CRT) is the standard treatment for locally advanced cervical cancer [Citation4], and radiotherapy provides the greatest survival benefit for cervical cancer patients. However, neither radiotherapy nor chemotherapy is effective for a substantial proportion of cases, and the 5-year survival rate remains at only 40–50% [Citation26].One possible explanation for these unsatisfactory results is the radioresistance at baseline. Therefore,continued efforts are required to improve CRT and identify biomarkers for patients at risk of poor therapeutic response.
Our previous studies have shown that ZNF582m-positive cervical cancer patients have a better prognosis, but the underlying mechanisms are undefined. Chemoradiotherapy resistance is associated with poor prognosis of cervical cancer, especially in advanced cases, suggesting that ZNF582m status may regulate chemo- and/or radiosensitivity. In this study, we confirmed that patients with high levels of ZNF582 promoter methylation were more sensitive to radiation therapy ( and ). SCC-Ag is most commonly used to monitor the therapeutic effect, recurrence, metastasis, and prognosis of cervical cancer [Citation8,Citation27–29]. Similar to SCC, ZNF582m was significantly reduced after radiotherapy ().
Another major finding of this study was that radiation therapy could induce an initial transient increase in ZNF582−low group, and these patients presented greater sensitivity compared to other hypomethylated patients. Further studies are needed to explore predictors and the mechanisms underlying this unusual response to expand the clinical application of radiotherapy.
We also explored the underlying mechanisms of ZNF582m levels and radiotherapy sensitivity of cervical cancer. Methylation is a negative regulator of gene expression. We reported in our previous study that ZNF582 promoter hypermethylation correlated with low ZNF582 protein expression, and similarly, low ZNF582m levels correlated with high protein expression [Citation16]. The expression levels of ZNF582 were extremely low in HeLa cells (). ZNF582 protein overexpression delayed S/G2-phase in HeLa cells, inducing p27/p21 accumulation and inhibition of the PI3K/Akt pathway, and ZNF582 protein overexpression increased resistance to radiation (). Tumour cells in different cell cycle stages showed different sensitivity to radiation, with the highest sensitivity in G2/M phase and the lowest in S phase [Citation21–23]. Accumulation of p27 [Citation30–35], p21 [Citation36–38], CDK2 [Citation39], and CDK4 [Citation40] and inhibition of PI3K/Akt/CyclinD1 [Citation41] may contribute to S/G2 cell cycle arrest. Our data suggested that ZNF582 protein overexpression delayed S/G2-phase in HeLa cells and increased resistance to radiation. These findings may explain why ZNF582m-low patients were resistant to radiotherapy and conferred poorer prognosis [Citation16].
This study demonstrated that ZNF58m levels in exfoliated cervical cells are a reliable biomarker for predicting the response to radiotherapy and monitoring therapeutic efficacy. As a biomarker, ZNF582m has several advantages: (1) it could be measured in non-invasively exfoliated cells; (2) it provides not only a pre-treatment predictor of response but also allows therapeutic monitoring during the course of treatment; and (3) it could be accurately and inexpensively detected with conventional reagents. We concluded that ZNF58m measurement is helpful for early screening, diagnosis, prediction of radiotherapy response, and prognosis of cervical cancer.
Abbreviations
ZNF582, zinc finger protein 582;ZNF582m, methylated ZNF582 gene; RECIST, Response Evaluation Criteria in Solid Tumours; ROC, receiver operating characteristic; CCRT, concurrent chemoradiotherapy; PBS, phosphate-buffered saline; CR, complete remission; PR, partial response; PD, Progressive disease; SD, Stable disease; M-index, the methylation index; HPV, human papillomavirus; gDNA, genomic DNA; QMSP, quantitative methylation-specific PCR; COL2A, The type II collagen gene; Cp, The crossing point; SR, Satisfied response; MR, Modest response; AUC, area under the curve; CT, computed tomography; SCC-Ag, Squamous cell carcinoma antigen; CRT, Chemoradiotherapy; FIGO, Federation of Gynecology and Obstetrics; RT, radiation treatment; SCC, Squamous cell carcinoma; SD, standard deviation; ORR, objective remission rate.
Authors’ contributions
XYZ, MCZ, JTC, TH, XTL, and CZQ contributed to sample collection. NYW contributed to the writing of this manuscript. XYZ, NYW, and MCZ contributed to the clinical data evaluation and the molecular experiments. JTC, JW, NYW, and QJL contributed to the conception, design, and final approval of the submitted version. JW and NYW provide financial support. XYZ, YW, CF, SYL, and HL contributed to the technical support for the data analysis. All authors read and approved the final manuscript.
Ethics approval and consent to participate
The study protocol was approved by the Institutional Review Board of Hunan Cancer Hospital and the Animal Ethics Committee of Hunan Cancer Hospital. Informed consent was obtained from all participants in accordance with the Declaration of Helsinki.
Consent for publication availability of data and material
The data that support the findings of this study are available on request from the corresponding author. The data are not publicly available due to privacy or ethical restrictions.
Financial support
This work was supported by the Department of Science and Technology of Hunan Province (2018SK2121, 2020SK2120, 2020JJ5338 and 2021RC2104), the National Natural Science Foundation of China (82,003,050), the Foundation of Social Development Science and Technology Division (kq2004138), and Scientific Research Project of Hunan Health Commission (C2019066).
Authors’ information
1. Hunan Cancer Hospital, the Affiliated Cancer Hospital of Xiangya School of Medicine, Central South University, Changsha, Hunan, China. 2. University of South China, Hengyang, Hunan, China. 3. The Second People’s Hospital of Yueyang City, Hunan, China. 4. The Second People’s Hospital of Hunan Province. 5. Department of Pharmacy, the First Affiliated Hospital of Zhengzhou University, Zhengzhou, China.
Acknowledgments
The authors thank all the members of Jing Wang laboratory, Hunan Hongya Gene Technology Co., Ltd., and Enago (www.enago.cn) for the English embellishment.
Disclosure statement
No potential conflict of interest was reported by the author(s).
Additional information
Funding
References
- Siegel RL, Miller KD, Jemal A. Cancer statistics, 2019. CA Cancer J Clin. 2019;69(1):7–34.
- Miller KD, Nogueira L, Mariotto AB, et al. Cancer treatment and survivorship statistics, 2019. CA Cancer J Clin. 2019;69(5):363–385.
- Bray F, Ferlay J, Soerjomataram I, et al. Global cancer statistics 2018: GLOBOCAN estimates of incidence and mortality worldwide for 36 cancers in 185 countries. CA Cancer J Clin. 2018;68(6):394–424.
- Bermudez A, Bhatla N, Leung E. Cancer of the cervix uteri. Int J Gynaecol Obstet. 2015;131(2):S88–95.
- Wang SM, Qiao YL. Implementation of cervical cancer screening and prevention in China–challenges and reality. Jpn J Clin Oncol. 2015;45(1):7–11.
- Kokka F, Bryant A, Brockbank E, et al. Hysterectomy with radiotherapy or chemotherapy or both for women with locally advanced cervical cancer. Cochrane Database Syst Rev. 2015; (4):Cd010260. DOI:10.1002/14651858.CD010260.pub2.
- Yang J, Cai H, Xiao Z-X, et al. Effect of radiotherapy on the survival of cervical cancer patients: an analysis based on SEER database. Medicine (Baltimore). 2019;98(30):e16421.
- Fu J, Wang W, Wang Y, et al. The role of squamous cell carcinoma antigen (SCC Ag) in outcome prediction after concurrent chemoradiotherapy and treatment decisions for patients with cervical cancer. Radiat Oncol. 2019;14(1):146.
- Sabulei C, Maree JE. An exploration into the quality of life of women treated for cervical cancer. Curationis. 2019;42(1):e1–e9.
- Li Q, Zhang Y, Jiang Q. SETD3 reduces KLC4 expression to improve the sensitization of cervical cancer cell to radiotherapy. Biochem Biophys Res Commun. 2019;516(3):619–625.
- Huang C, Lu H, Li J, et al. SOX2 regulates radioresistance in cervical cancer via the hedgehog signaling pathway. Gynecol Oncol. 2018;151(3):533–541.
- Figueroa ME, Lugthart S, Li Y, et al. DNA methylation signatures identify biologically distinct subtypes in acute myeloid leukemia. Cancer Cell. 2010;17(1):13–27.
- Huntley S, Baggott DM, Hamilton AT, et al. A comprehensive catalog of human KRAB-associated zinc finger genes: insights into the evolutionary history of a large family of transcriptional repressors. Genome Res. 2006;16(5):669–677.
- Huang RL, Chang -C-C, Su P-H, et al. Methylomic analysis identifies frequent DNA methylation of zinc finger protein 582 (ZNF582) in cervical neoplasms. PLoS One. 2012;7(7):e41060.
- Liou YL, Zhang T-L, Yan T, et al. Combined clinical and genetic testing algorithm for cervical cancer diagnosis. Clin Epigenetics. 2016;8(1):66.
- Wu N-Y-Y, Zhang X, Chu T, et al. High methylation of ZNF582 in cervical adenocarcinoma affects radiosensitivity and prognosis. Ann Transl Med. 2019;7(14):328.
- Lin H, Chen T-C, Chang T-C, et al. Methylated ZNF582 gene as a marker for triage of women with Pap smear reporting low-grade squamous intraepithelial lesions - a Taiwanese Gynecologic Oncology Group (TGOG) study. Gynecol Oncol. 2014;135(1):64–68.
- Tian Y, Wu NYY, Liou YL, et al. Utility of gene methylation analysis, cytological examination, and HPV-16/18 genotyping in triage of high-risk human papilloma virus-positive women. US: Oncotarget; 2017.
- Liou YL, Zhang Y, Liu Y, et al. Comparison of HPV genotyping and methylated ZNF582 as triage for women with equivocal liquid-based cytology results. Clin Epigenetics. 2015;7(1):50.
- Sinclair WK, Morton RA. X-ray sensitivity during the cell generation cycle of cultured Chinese hamster cells. Radiat Res. 1966;29(3):450–474.
- Sinclair WK, Morton RA. Variations in X-ray response during the division cycle of partially synchronized Chinese hamster cells in culture. UK: Nature. 1963; 199: 1158–1160.
- Terasima T, Tolmach LJ. Variations in several responses of HeLa cells to x-irradiation during the division cycle. Biophys J. 1963;3(1):11–33.
- Terasima T, Tolmach LJ. X-ray sensitivity and DNA synthesis in synchronous populations of HeLa cells. Science. 1963;140(3566):490–492.
- Carlson JG. X-ray-induced prophase delay and reversion of selected cells in certain avian and mammalian tissues in culture. Radiat Res. 1969;37(1):15–30.
- Rodin D, Burger EA, Atun R, et al. Scale-up of radiotherapy for cervical cancer in the era of human papillomavirus vaccination in low-income and middle-income countries: a model-based analysis of need and economic impact. Lancet Oncol. 2019;20(7):915–923.
- Wright JD, Chen L, Tergas AI, et al. Population-level trends in relative survival for cervical cancer. Am J Obstet Gynecol. 2015;213(5):670.e1–7.
- Salvatici M, Achilarre MT, Sandri MT, et al. Squamous cell carcinoma antigen (SCC-Ag) during follow-up of cervical cancer patients: role in the early diagnosis of recurrence. Gynecol Oncol. 2016;142(1):115–119.
- Wen YF, Cheng -T-T, Chen X-L, et al. Elevated circulating tumor cells and squamous cell carcinoma antigen levels predict poor survival for patients with locally advanced cervical cancer treated with radiotherapy. PLoS One. 2018;13(10):e0204334.
- Shen Q, Lin W, Luo H, et al. Differential expression of aquaporins in cervical precursor lesions and invasive cervical cancer. Reprod Sci. 2016;23(11):1551–1558.
- Liu Z, Li J, Chen J, et al. MCM family in HCC: MCM6 indicates adverse tumor features and poor outcomes and promotes S/G2 cell cycle progression. BMC Cancer. 2018;18(1):200.
- Wang A, Zeng R, Huang H. Retinoic acid and sodium butyrate as cell cycle regulators in the treatment of oral squamous carcinoma cells. Oncol Res. 2008;17(4):175–182.
- Lin CB, Lin CC, Tsay GJ. 6-gingerol inhibits growth of colon cancer cell LoVo via induction of G2/M arrest. Evid Based Complement Alternat Med. 2012;2012:326096.
- Sun XL, Zhang X-W, Zhai H-J, et al. Magnoflorine inhibits human gastric cancer progression by inducing autophagy, apoptosis and cell cycle arrest by JNK activation regulated by ROS. Biomed Pharmacother. 2020;125:109118.
- Auld CA, Fernandes KM, Morrison RF. Skp2-mediated p27(Kip1) degradation during S/G2 phase progression of adipocyte hyperplasia. J Cell Physiol. 2007;211(1):101–111.
- Zhong S, Ji D-F, Li Y-G, et al. Activation of P27kip1-cyclin D1/E-CDK2 pathway by polysaccharide from phellinus linteus leads to S-phase arrest in HT-29 cells. Chem Biol Interact. 2013;206(2):222–229.
- Barr AR, Cooper S, Heldt FS, et al. DNA damage during S-phase mediates the proliferation-quiescence decision in the subsequent G1 via p21 expression. UK: Nat Commun. 2017; 8:14728.
- Huang B, Mu P, Chen X, et al. Aflatoxin B(1) induces S phase arrest by upregulating the expression of p21 via MYC, PLK1 and PLD1. Biochem Pharmacol. 2019;166:108–119.
- Beckerman R, Donner AJ, Mattia M, et al. A role for Chk1 in blocking transcriptional elongation of p21 RNA during the S-phase checkpoint. Genes Dev. 2009;23(11):1364–1377.
- Zhong S, Li YG, Ji DF, et al. Protocatechualdehyde induces S-phase arrest and apoptosis by stimulating the p27(KIP1)-cyclin A/D1-CDK2 and mitochondrial apoptotic pathways in HT-29 cells. Switzerland: Molecules; 2016; 21(7):934.
- Ravanko K, Järvinen K, Paasinen-Sohns A, et al. Loss of p27Kip1 from cyclin E/cyclin-dependent kinase (CDK) 2 but not from cyclin D1/CDK4 complexes in cells transformed by polyamine biosynthetic enzymes. Cancer Res. 2000;60(18):5244–5253.
- Ahn H, Im E, Lee DY, et al. Antitumor effect of pyrogallol via miR-134 mediated S phase arrest and inhibition of PI3K/AKT/Skp2/cMyc signaling in hepatocellular carcinoma. Int J Mol Sci. 2019;20(16):3985.