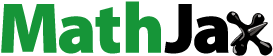
Abstract
This paper compares the application of two recently published guidance documents for risk-based assessment of hydraulic actions on bridges, namely the UK Design Manual for Roads and Bridges and the Italian Ministry of Infrastructure and Transport’s Guidelines, to two case study bridges (Staverton Bridge, UK; Borgoforte Bridge, Italy). This work is one of the first to illustrate how to apply these guidelines. Both documents present risk-based methods for the assessment of hydraulic actions, while exhibiting fundamental differences. For example, the UK method prescribes calculations for local and constriction scour, water depth, and velocity at several cross-sections; by comparison, the Italian method does not prescribe calculations to assess the risk level. For the case studies in this paper, the hydraulic risk obtained for Staverton Bridge resulted as ‘High’ using both methods. The scour score for the Borgoforte Bridge resulted higher using the Italian method (Medium-High), as compared to the UK approach (Medium). This difference is due to how the guidelines assess the vulnerability associated with the minimum clearance. The comparison of these two risk-based approaches and the resulting discussion may serve as a useful resource for those wishing to develop new risk-based methods for assessing hydraulic actions on bridges.
1. Introduction
Hydraulic actions on bridges are a significant source of damage and pose risks to the safety and stability of new and existing structures. One particular action, namely scour erosion at piers and abutments, remains a major cause of damage and collapse of bridges worldwide (Blockley, Citation2011; Lamb, Garside, Pant, & Hall, Citation2019; Maddison, Citation2012; Sasidharan, Parlikad, & Schooling, Citation2021; Selvakumaran, Plank, Geiß, Rossi, & Middleton, Citation2018). Scour occurs where flowing water leads to the removal of soil from around bridge foundations (Hamill, Citation1999), which can compromise stability. Maddison (Citation2012) provides a detailed discussion of the various types of bridge scour, namely ‘general’, ‘contraction/constriction’, and ‘local’ scour. General scour is caused by natural channel evolution, contraction (or constriction) scour is caused by water flowing through bridge openings (reduced flow area), and local scour is caused by the presence of obstacles (such as foundations) to the flow. The combination of scour types can lead to significant scour hole depths at affected bridges (Klinga & Alipour, Citation2015). Scour can be exacerbated by certain phenomena such as actions induced by debris accumulation (Panici, Kripakaran, Djordjević, & Dentith, Citation2020). Moreover, climate change is likely to increase the frequency and magnitude of flooding events (Nasr et al., Citation2020), which could further aggravate scour risk on certain structures. For these reasons, the identification of bridges at risk of scour is crucial for infrastructure operators, managers, and asset owners.
Asset owners and operators use various methods to rank and prioritise structures using available data. There is general consensus in literature that risk-based methods are the most appropriate for this task because they allow consideration of multiple elements (hazard, structure, cost, etc.) that underpin decision-making for a bridge subjected to hazards (Adey, Hajdin, & Brühwiler, Citation2003). Various European projects on bridge management under natural hazards have proposed a range of risk-based approaches (e.g., Campos, Casas, & Fernandes, Citation2016; Khakzad & van Gelde, Citation2016). Decision-making related to bridge management can be enhanced by information from monitoring systems that can capture and record scour effects during flood events (Giordano, Prendergast, & Limongelli, Citation2022; Prendergast & Gavin, Citation2014; Vardanega, Gavriel, & Pregnolato, Citation2021). For large networks of bridges, however, certain structures will inevitably succumb to flood-related damage and scour (Whitbread, Benn, & Hailes, Citation2000), and real-time monitoring of every bridge with sensors remains unachievable due to the associated costs (Farreras-Alcover, Andersen, & McFadyen, Citation2016). It is therefore essential that asset owners and operators can prioritise the most at-risk bridges in their network (Arneson, Zevenbergen, Lagasse, & Clopper, Citation2012).
Various guidelines for the management of bridges under flood hazards have been developed to date (e.g., Abé, Shimamura, & Fujino, Citation2014; FHWA, Citation2004; Mn DOT, Citation2009; TDT, Citation1993). International references include the AASHTO manuals (Citation2020), while the European regulation Eurocodes (BSI, Citation2002, Citation2005, Citation2006; CEN TC 250 N1148: 2015; EN 16991: 2018; ISO 13822: 2010) currently offer principles for assessing hydraulic actions on bridges but offer little guidance on how to prioritise bridges for scour monitoring and maintenance interventions. Moreover, how to deal with climate change effects and the influence of debris has received little attention (Takano & Pooley, Citation2021). This paper focuses on two recent risk-based guidelines for assessing hydraulic actions on bridges, namely the UK Design Manual for Roads and Bridges (CS 469) (HA, Citation2012; Takano & Pooley, Citation2021) and the Italian Ministry of Infrastructure and Transport (MIT) guidelines (CSLP, Citation2020), and demonstrates their performance against two case study bridges. This paper has the following aims:
To apply the UK and Italian guidelines to two bridges from the UK and Italy to demonstrate how the guidelines operate.
To compare the results from the two methods to demonstrate how risk-based approaches and concepts can be incorporated into scour assessment guidelines.
To offer novel insights on how future codes of practice can be further enhanced to better assess hydraulic risk for bridge structures.
2. Management of scour and hydraulic actions in practice
Transportation agencies usually manage a portfolio of hundreds or even thousands of bridges, thus they must adopt specific procedures to rank the level of risk and prioritise interventions on bridges prone to scour. A risk-based approach to prioritize interventions combines information about the hazard (hydraulic actions), the vulnerability of the bridge, and the consequences of potential damage (Pregnolato, Citation2019). Using inspections and monitoring data (when available) as inputs, risk-based approaches usually rank bridges according to different ‘risk’ classes. Pregnolato et al. (Citation2020) suggested that bridge agencies can have varying in-house definitions of the concept of risk, which is incorporated into ‘risk-based’ practice with varying degrees of refinement. The present work focuses on two risk-based national guidelines for managing hydraulic actions and scour available in the UK and Italy, which were released in 2021 and 2020 respectively.
The UK guidelines (see Sec. 2.1) introduced innovation by considering debris and climate change in assessments. Moreover, it included an updated assessment of the impact of a bridge failure on communities (which was missing in previous work, e.g., Sasidharan et al., Citation2021). The Italian guidelines (see Sec. 2.2) are the first to be issued at the national (Italian) level for the management of bridges, and contain a comprehensive system perspective and a wider assessment of consequences, which also include human and surrounding property losses.
2.1. Scour and hydraulic actions risk assessment in the UK
The risk assessment due to scour and hydraulic actions on UK bridges is regulated by National Highways’ CS 469 Management of scour and other hydraulic actions at highway structures (hereafter referred to as CS 469), which replaces BD97/12 Assessment of scour and other hydraulic actions at highway structures (Takano & Pooley, Citation2021). Informative case histories and technical guidance on scour at bridges is also contained in CIRIA (Citation2017). The main aim of CS 469 is to evaluate the risk posed by scour and other hydraulic actions to bridges and other riverine structures (e.g., retaining walls), and to prioritise and manage risk mitigation measures when required. The guideline covers crucial aspects of the whole process of monitoring, evaluating, and managing risk, including characterising scour and hydraulic risks, inspections and monitoring of scour-prone structures, and mitigation measures to reduce negative consequences from scour and hydraulic actions for both affected populations and structures.
The estimation of the risk in CS 469 is split into two levels. Level 1 is a preliminary screening that assesses scour impact based on a qualitative analysis without calculations or numerical analysis. Level 1 considers: (i) the history of scour at the bridge, (ii) visual inspections, (iii) other factors based on the bridge location (e.g., flow angle of attack, history of debris accumulation). The outcome of a Level 1 assessment can be either low risk of scour or the requirement to conduct a Level 2 assessment. Level 2 assesses the risk level after a detailed set of surveys and calculations that include a topographic survey of the cross-sections upstream and downstream of the subject bridge, as well as hydraulic calculations for water depth and velocity upstream and at the structure.
2.2. Scour and hydraulic actions risk assessment in Italy
Risk assessment on Italian bridges is addressed by the ‘Guidelines for risk classification and management, safety evaluation and monitoring of existing bridges’ which was issued by the Italian Ministry for Public Work in 2020 (CSLP, Citation2020). These guidelines are aimed at providing operators with standard procedures for bridge safety management at a national level ensuring safety by maintaining an acceptable risk level. The guidelines propose a multi-level and multi-risk procedure entailing the survey and the risk classification of existing bridges, the assessment of their safety, and the management of planned inspections and monitoring.
The procedure concerns six levels (0-5) of analysis. The complexity and the level of detail of the investigations increase with the level of the analysis, but the number of bridges examined, as well as the level of uncertainty of the results obtained, decrease. The first three levels relate to a preliminary analysis at a local scale, involving the collection of existing data (Level 0), in situ inspections (Level 1), and risk-based classification (Level 2) considering four types of risks, namely, (i) the structure-foundation risk, (ii) the seismic risk, (iii) the landslide risk, and (iv) the hydraulic risk. The structures that present criticalities are further investigated through simplified (Level 3) or accurate (Level 4) safety assessments. Level 5 is the final level and mentions a resilience analysis of the transportation network. This level of analysis is not directly detailed in the guidelines, which recommend adopting methods from international literature. Currently, the implementation of these guidelines is at an experimental phase which will lead to a preliminary review for their final adoption. Very few studies have applied this approach, with those that have mainly focused on seismic and structural risk (e.g., De Matteis, Bencivenga, & Zizi, Citation2021; Santarsiero, Masi, Picciano, & Digrisolo, Citation2021).
3. Methodology
This study applies the UK and Italian guidelines to two different case study bridges with the goal to demonstrate how the methods operate, and how they compare, in order to draw lessons for further improvement.
3.1. Methodology proposed in the UK code
For the UK assessment, the Level 2 Scour Risk Assessment of CS 469 has been employed, which evaluates risk and vulnerability that are assessed and classified independently for scour, hydraulic actions (e.g., uplift), and channel stability. shows a schematic of the hydraulic-related phenomena considered in CS 469 and its assessing criteria; interested readers can refer to Takano and Pooley (Citation2021) for further details.
Figure 1. A schematic representation of the UK method and indication assessment process for the three aspects considered; details can be found in Takano and Pooley (Citation2021).
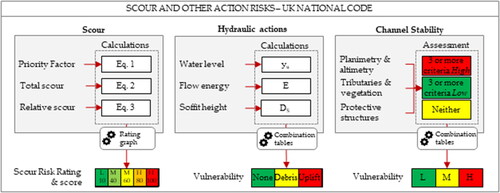
The scour risk is based on two factors, the first is the priority factor PF:
(1)
(1)
This equation is the product of seven heuristic coefficients (described in ), each of them depending on qualitative or quantitative information available (e.g., previous history of scour, river gradient, importance of road), although each term is independently defined (Takano & Pooley, Citation2021). In terms of establishing the history of scour, this can be achieved by analyzing previous visual inspection data if available. If not, methods such as Ground Penetrating Radar have shown success at detecting the depth of previous scour holes, subsequently filled in upon flood attenuation, see for example Anderson, Ismael, and Thitimakor (Citation2007).
Table 1. Factors and range of values for the Priority Factor in EquationEquation (1)(1)
(1) .
The second factor is the total scour depth DT at each structural element, whereby scour depth is given by the sum of contraction (DC) and local (DL) scour depths. The approach employed in the CS 469 makes use of formulae (CIRIA, Citation2017) for the estimation of each type of scour that are inclusive of several factors (e.g., angle of attack, pier or abutment shape, debris accumulations), according to:
(2)
(2)
where each term is described in . The computation of individual components (e.g., DC) in EquationEquation (2)
(2)
(2) (not shown here for brevity) is dependent on an input flow, that is the flood discharge for a return period of 200 years inclusive of climate change allowance (variable between 20% to 35% increase, depending on geographic location) for all types of rivers.
Table 2. Factors used in EquationEquation (2)(2)
(2) and typical ranges.
The risk assessment is evaluated by computing the relative scour depth DR (i.e., the ratio between total scour depth DT and foundation depth DF:
(3)
(3)
and then plotting against the priority factor PF. This operation needs to be repeated for all structural elements (e.g., piers, abutments), whilst the overall risk rating of the bridge will correspond to the highest among all assessed elements. depicts the graph used for this estimation, with indication of the risk category and score. The region in the graph where the interpolation between PF and DR falls will determine the risk rating for a structure.
Figure 2. Scour risk assessment chart for CS 469, interpolating the Priority factor PF on the horizontal axis and the relative scour depth DR on the vertical axis. Each score is identified by a category (i.e. High, Medium, Low) and a score (i.e. 10 to 100).
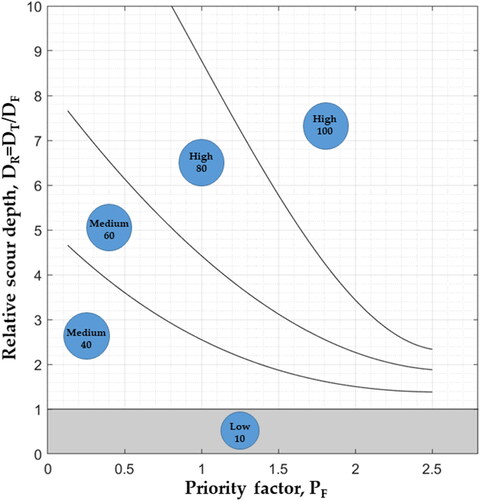
Depending on the area where the output lies in the plot, the bridge will be assigned a score and a rating for scour risk: 100 (High – immediate risk of scour), 80 (high), 60, and 40 (Medium), and 10 (Low). Structures with score 100 will be considered at immediate risk of scour and treated as substandard, i.e., requiring urgent interventions to minimise the load carried by the structure, including closure to vehicular traffic and implementation of scour mitigation measures.
In parallel with scour risk, the CS 469 requires carrying out an assessment on hydraulic actions on the bridge, such as soffit uplift, damage to bridge deck and parapets, and impact forces from debris. The assessment is based on the hydraulic calculations already carried out for scour, and only requires assessing i) whether the soffit of the bridge will be submerged by the flood flow or not, or ii) if the flow specific energy (i.e. the sum of kinetic and potential energy head of the flow relative to the channel bottom) under the bridge will be higher than the soffit height. If the hydraulic analysis estimates bridge submergence, then the guidelines require a vulnerability analysis (exposure × hazard). Associated recommended action might include bridge classification as substandard, if hydraulic actions are deemed to cause damage to the bridge. An intermediate situation is when neither the flow nor the specific energy is estimated to reach the bridge soffit, but the latter (i.e. flow specific energy) will be within 0.60 m of it. In this case, CS 469 consider the bridge at potential failure due to impact with debris and in need of further investigations (although this is outside the scope of CS 469).
Finally, a third assessment must be carried out on river channel stability upstream of the bridge. In this case, the assessment is only based on qualitative analysis, which includes observations on local river channel conditions or stream or riverbank protections. High or medium vulnerability will require actions from the overseeing organisation to reduce the impact of channel instability. Although in CS469 it is considered as a ‘risk rating’, the channel stability should be rather considered as vulnerability (as we refer to in this paper), since the approach is limited to qualitative observations, and does not consider possible consequences.
3.2. Methodology proposed in the Italian code
As introduced in Section 2.2, the risk classification of bridges at the local level is addressed in the realm of Level 2 of the multi-level procedure proposed by the Italian guidelines. The first step includes assigning an Attention Class (AC) for each risk type, i.e., the structure-foundation risk, the seismic risk, the landslide risk, and the hydraulic risk. Then, the individual ACs are combined to obtain a global AC used for risk classification purposes. There are five ACs in total, namely: Low, Medium-Low, Medium, Medium-High, and High. The global AC determines the actions to be carried out on each bridge of a given portfolio, i.e., the need for safety assessment, and/or the need for collecting detailed data by means of inspections or Structural Health Monitoring (SHM). In line with the purpose of this study, the Italian guidelines are applied up to the level relevant to Level 2 (risk classification), considering the hydraulic risk only.
The evaluation of the AC with respect to hydraulic actions considers three hydraulic-related phenomena, specifically: (i) insufficient minimum vertical clearance, (ii) general scour, and (iii) local scour. In the original manual, ‘general scour’ (erosione generalizzata, in Italian) relates to both contraction and general scour, as defined in Section 1; ‘local scour’ (erosione localizzata, in Italian) relates to both local and general scour. The AC for scour is determined by combining the AC for local and general scour by means of combination tables. The global AC for hydraulic risk is obtained by selecting the lowest AC between the AC for insufficient vertical clearance and the AC for scour (see ).
Figure 3. A schematic representation of the hydraulic risk assessment for the Italian method. Details can be found in CSLP. (Citation2020).
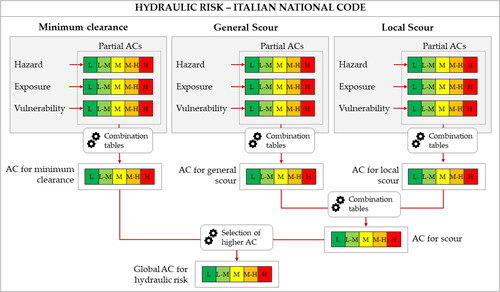
For each hydraulic-related phenomenon, a partial AC is assigned to hazard, exposure, and vulnerability, for a total of nine partial ACs (three hydraulic-related phenomena multiplied by three risk parameters, as shown in ). The partial ACs are combined by means of combination tables (see ). For instance, in case the partial ACs for hazard, vulnerability, and exposure are Low, High, and Low, respectively, the AC for that hydraulic phenomenon will be High. The guidelines provide specific rules to drive the assignment of the nine partial ACs based on the value assumed by several quantitative or qualitative variables, as briefly explained in the sequence.
Regarding the hazard, for the minimum clearance, the partial AC is estimated by considering the distance between the bridge soffit and the water level related to the specified return period; different return periods are considered but they do not depend on the type of bridge, although the importance of the bridge is considered in the consequences. With reference to general scour, the partial AC is evaluated considering: (i) the ratio between the width of the riverbed occupied by the bridge and the overall width of the riverbed, Ca, and (ii) the ratio between the width of the floodplains occupied by the bridge and the overall width of the floodplains, Cg. As for the local scour, the partial AC is determined by the ratio between the scour depth and the foundation depth (taken as 2 m if the foundation depth is unknown); the method requires the assumption that the scour depth be two times the width of the piers (CSLP, Citation2020, p. 34).
The vulnerability depends on the type of foundation, the geometry of the riverbed and the amounts of sediment/debris/floating materials. The definition of the partial ACs for the three hydraulic-related phenomena is mostly based on meeting certain conditions, i.e. the operator uses tables provided in the guidelines to verify if certain conditions are respected. The following conditions are considered:
For insufficient vertical clearance: (i) significant sediment deposition or riverbed erosion; (ii) significant transport of plant material of considerable size; (iii) dimension of the river basin higher or lower than defined thresholds. For example, a partial AC can score ‘medium’ whether at least one condition is met among the presence of (i) and (ii), and (iii) <500 km2.
For general scour: (i) superficial foundations; (ii) generalized lowering of the riverbed; (iii) curvature of the riverbed. For example, a partial AC can score ‘medium’ whether at least one condition is met among presence of (i), (ii), and (iii).
For local scour: (i) superficial foundations; (ii) generalized lowering of the riverbed; (iii) accumulations of debris or floating material; (iv) planimetric migration of the riverbed; (v) existence of scour protection devices. For example, a partial AC can score ‘medium’ whether at least one condition is met among presence of (i), (ii), (iii), and (iv).
The exposure mainly accounts for indirect consequences related to the loss of functionality of the bridge and damage to the environment induced by the bridge collapse. A single partial AC is determined for all three hydraulic phenomena considering different parameters, namely: (i) the daily traffic level; (ii) the length of the bridge span used as a proxy for the number of people on the bridge; (iii) presence (under the bridge) of people and important assets from the naturalistic, economic and social perspectives; (iv) presence of alternative routes; (v) criticality of the bridges during an emergency; and (vi) transport of dangerous goods. Based on the value assumed by these parameters, tables, and graphs guide the operator in the selection of the partial AC for the exposure, as illustrated in CSLP (Citation2020). For instance, the partial AC for bridges with a low level of traffic (≤10,000 vehicles/day) and short span length (≤20 m) is Medium-High in case: (i) alternative routes are not present, (ii) people or important assets are present under the bridge, (iii) the bridge is a strategic asset. The presence of dangerous goods is used as a prioritization factor between bridges belonging to the same AC.
3.3. Case study
This study applies the UK and Italian guidelines to two different case studies in Italy and in the UK (), which presented different characteristics and available data.
Figure 5. The selected structures for the case study: (a) Staverton Bridge in the UK; (b) Borgoforte Bridge in Italy.
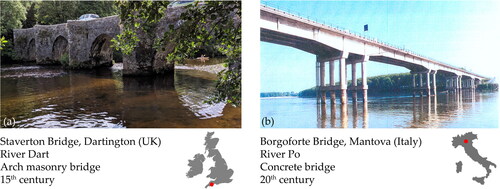
Staverton Bridge () crosses the River Dart near Totnes in Devon, in the South-West of England (UK), and is located on road C46. The bridge consists of seven arch masonry spans of varying sizes. The bridge construction date is believed to be 1413, and it is a Grade I listed structure (i.e., the bridge is of exceptional architectural and historical interest). The bridge is located in between two river bends, immediately downstream of a small island, whilst downstream of the bridge the river Dart flows around the large Dartington island. The bridge is owned by Devon County Council and scour was observed at the piers and foundations during inspections in 2018 and 2019. The River Dart at Staverton Bridge has a catchment area of 268.1 km2 after originating in Dartmoor, and the riverbed is formed mostly by coarse gravel, with a catchment bedrock of low permeable rocks. The 200-year flood peak flow is estimated to be 611.1 m3s−1, whereby the increase due to climate change allowance for peak river flow (according to CS 469) is 25% for the South-West of England. The bridge is also known for periodically accumulating large wood debris at its piers after seasonal floods.
Borgoforte bridge in Italy () is a concrete bridge built in 1961 which crosses the Po River on state road no. 62 between the Regions Lombardy and Emilia Romagna (Northern Italy). The overall length of the structure is approximately 1137 m. The bridge consists of a complex structure of three different parts, as follows:
The left access viaduct (Lombardy side) is located in the floodplain and consists of 9 simply supported spans each with a length of 18.35 m (total length 165m).
The bridge over the river Po, partly located in the floodplain and partly in the riverbed, consists of 7 pairs of piers that support cantilever beams of variable height which are connected by means of a suspended section (total length 472m). The total span between two piers is 63.50 m. The piers are composed of 3 circular section columns with a diameter of 1.5 m.
Right access viaduct (Emilia Romagna side) is located beyond the embankment and consists of 28 simply supported spans each of 18.40 m length (total length 500m).
In normal flow conditions, the width of the river is approximately 300 m. Therefore, only four of the 44 piers of the bridge are permanently in water. In the year 2000, a 15 m deep scour hole was found in the riverbed after a flood. Subsequently, the piles affected by the scour were reinforced and the hole filled (Ballio, Ballio, Franzetti, Crotti, & Solari, Citation2018). Main input data for the two bridges and the two methodologies are listed in ; data were obtained from private reports owned by Devon County Council and Italian authorities for Staverton and Borgoforte bridge, respectively.
Table 3. Input data for the two case studies and the two methodologies; data were obtained from private reports.
4. Results
The first outcome of this study includes a methodological comparison between the application of the two guidelines (CSLP, Citation2020; Takano & Pooley, Citation2021). A critical analysis and interpretation of the two methods resulted in the following list of similarities (S) and differences (D), shown in .
Table 4. Summary of methodological similarities and differences between the Italian and UK methods (authors’ critical analysis).
A more informative analysis occurs when both methods are applied to a single bridge, demonstrating how they work and comparing their performance. In the application, results are mostly homogenous, although a few differences are present. Table 6 summarizes the results obtained highlighting the actions triggered by the attained risk levels. Sec. 4.1 and 4.2 illustrate the application and explain the results in detail.
Table 5. Final risk rating for the two methods and the two bridges considered in this study (L = low, M = medium, H = high).
4.1. Results of the application of the UK method
In this section, the UK method is applied to both the UK and Italian bridges to demonstrate its operation. The application of the UK method (CS 469) to Staverton Bridge and Borgoforte Bridge showed that the two bridges attained different levels of risk, depending on the type of hydraulic action. shows a schematic comparison between the two structures. First, the scour risk rating is High (score of 100) for Staverton Bridge, indicating that the bridge is at immediate risk of scour, according to the UK criteria. On the other hand, Borgoforte Bridge scored 40, indicating a risk rating of Medium. As a result, Staverton will have to be treated as a substandard structure (where actions have to be taken immediately) by the competent authority, whilst Borgoforte Bridge will only require a monitoring plan.
Since the priority factor of the two structures is comparable (1.638 for Staverton and 1.747 for Borgoforte Bridge), the different ratings are mostly due to the relative scour depth (DR): for Borgoforte Bridge, DR is relatively low (1.14), due to the depth of foundations comparable to the scour depth. Conversely, for Staverton Bridge DR is very high (9.71), although this was calculated on an assumed foundation depth of 1 m, as per recommendation of the UK approach. Nevertheless, the type of structure (masonry arch bridge from the 15th century) suggests that the real foundation depth will be in the order of 1-2 m, resulting in high values of DR.
For the assessment of hydraulic actions (e.g., uplift) both bridges resulted in low vulnerability, meaning that the bridge deck and parapet are unlikely to be submerged in either case and thus to be at risk of hydraulic actions. Despite in both cases the clearance under the bridge deck being less than 1 m, the UK method would not require any further assessment. It should be noted that the hydraulic risk for CS 469 is not explicitly specified, since the analysis is treated as vulnerability. For the purposes of this work, the risk of hydraulic actions has been assumed low since no vulnerability was identified.
The hydraulic assessment also suggested that Staverton Bridge might be at risk from debris impact damaging or dislodging the bridge, whilst this is not the case for Borgoforte Bridge. This assessment is only based on energy considerations at the bridge opening (Section 3.1), i.e. the flow specific energy under the bridge at Staverton Bridge was ca. 0.60 m lower than the bridge soffit depth, although it should be considered that both bridges have a history of debris accumulation. Finally, the vulnerability of channel stability for this study resulted as low for Borgoforte Bridge and medium for Staverton. This difference is based on the qualitative assessment adopted in the UK method: Borgoforte Bridge had enough criteria qualifying for a low rating, whilst Staverton Bridge did not have enough criteria for either low or high risk, hence it was taken as Medium.
4.2. Results of the application of the Italian method
The results relating to the application of the Italian guidelines are shown in . Both Borgoforte and Staverton bridges are classified into the High global AC for hydraulic actions. Nevertheless, it is observed that in general, the partial ACs assume different values for the two case studies. Two major differences exist, concerning: (i) the partial AC for exposure, and (ii) the partial ACs for the hazard relating to general scour and localized scour.
As for the exposure, the Borgoforte and the Staverton bridge fall into the High and Low partial AC, respectively. Indeed, the Borgoforte bridge is one of the major bridges of the Mantua Province and it serves both local and interregional traffic. Besides, it has been estimated that the indirect costs related to the closure of the bridge (including the cost of time loss for users and additional costs of running vehicles) in 2013 are of the order of 88.7 million Euro/year (Éupolis Lombardia, Citation2013). Conversely, the Staverton bridge, although relevant from a historical point of view, does not play a significant role within the road network and therefore its closure does not imply severe consequences.
Regarding the hazard for general and local scour, the Borgoforte and the Staverton bridge fall into the Low and High partial AC, respectively. These results are mainly due to the different structural properties of the two bridges (concrete vs arch masonry bridge). As for the general scour, the Borgoforte bridge presents very low values of the ratios Ca and Cg (see Sec. 3) due to the relatively small dimensions of piers with respect to the dimensions of the river, corresponding to Low AC. Conversely, the two ratios are quite high for Staverton bridge due to its massive structure with respect to the river dimensions. Regarding local scour, the AC is strongly influenced by the value of the foundation depth, that is 18 m for the Borgoforte bridge and unknown for the Staverton bridge (thereby the reference value of 2 m is employed in the calculations).
4.3. Sensitivity analysis
A sensitivity analysis is carried out in this section to determine which are the dominant factors of the methods proposed by the two national documents. Specifically, for the UK method, the sensitivity analysis has been focused on varying individual parameters that contribute to the value of the Priority Factor PF according to EquationEquation (1)(1)
(1) . For the Italian guidelines, the sensitivity analysis is performed to assess the relative importance of each partial AC involved in the computation of the global AC for hydraulic risk.
A similar sensitivity analysis has been carried out on the factors used by the UK method for scour assessment. Since the estimation of scour depth is deterministic, the analysis has been based on the heuristic Priority Factor PF. In this case, for both Borgoforte and Staverton bridges, the individual factors that contribute to PF have been varied across the whole range of factors available and consequently, the risk rating has been re-assessed for each new combination, based on the scour risk rating graph in . Results are reported in for Staverton Bridge at piers and abutments. On the vertical axis the individual elements of the priority factor are reported, whilst on the horizontal axis the values that contribute to
for each individual factor (see EquationEquation (1)
(1)
(1) ) are displayed.
Figure 8. Sensitivity analysis for Staverton bridge considering the UK method for the Priority factor PF for piers and abutments. For Borgoforte Bridge no variation was observed.
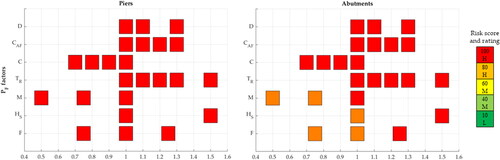
In the case of Borgoforte Bridge, since the relative scour depth is low (i.e., DR = 1.14) any variation of PF does not produce any difference. From , any DR value in the range 1-1.4 will always result in a rating Medium and score 40, irrespective of the Priority factor PF. A similar situation is also observed for Staverton bridge at the piers, although for the opposite reason. In this case, the relative scour depth is High (DR=9.71), whereby the minimum value of priority factor to decrease from a score of 100 to a score of 80 is 0.85, therefore any variation of the priority factor would not affect the final risk rating, that will remain High (score 100).
Regarding the abutments of Staverton Bridge, DR is lower (resulting in 4.92), so the variation of PF produces a change of one risk class for the variation of the factors F (i.e., foundation type) and HS (i.e., history of scour). When F is varied, values of 1 and 0.75 would still result in High risk class, but with a lower score (80) than for its highest value (that is, 1.25). For the history of scour factor HS, a value of 1 (instead of 1.5) would lower the score to 80 (although still being considered at High risk). It should be noted that according to CS 469 the overall bridge risk rating should be considered as the highest among all structural elements, therefore, even if the risk rating of the abutments were to be reduced, the bridge rating will be unchanged.
Overall, the analysis showed that the approach proposed by CS 469 tends to have a low sensitivity to variations of the priority factor PF, although the two cases analysed here had relative scour depths lying on two opposite ends of the spectrum, whereby intermediate values might show a slightly higher sensitivity to changes in PF. For variations of the relative scour depth DR (and, consequently, of the total scour depth DT), a change in risk score only occurs when increase (or decrease) of the estimated maximum scour depth is significant: for Borgoforte bridge, a 46% increase of total scour depth is necessary to increase the scour risk rating to a score of 60. On the other hand, a reduction of 14% of the total scour depth would be required to reduce the risk rating to Low and a score of 10. This means that it is highly unlikely that the bridge will achieve a higher risk level, even with a more conservative approach. For Staverton bridge, the situation is the opposite: a change in risk rating from a score of 100 to a score of 80 is only possible when the total scour depth is reduced by a factor of 3 for the piers (1.5 for the abutments). Thus, Staverton bridge is likely to be assessed at the highest risk level even with less conservative scour depth estimations.
The method proposed by the Italian guidelines entails seven partial ACs in total (see Sec. 3.2): the hazard and vulnerability ACs for the three hydraulic-related phenomena (insufficient Minimum vertical Clearance (MC), General Scour (GS), and Local Scour (LS) plus one AC for the exposure. In this sensitivity analysis, the ACs are modified one by one, keeping the other ACs constant and equal to the value obtained in the analysis.
The results of the sensitivity analysis for the Borgoforte Bridge are displayed in , where the horizontal axis shows the values of the partial ACs for factors (indicated on the vertical axis) that contribute to risk, and the different colours indicate the value of the resulting global AC. The Exposure has greater importance with respect to vulnerability and hazard for the three hydraulic-related phenomena (MC, GS, LS): a decrease in the range M-H to M-L of the partial AC of Exposure entails a decrease from H to M-H of the global AC while a decrease of Exposure to L entails a decrease of the global AC from H to M. Changes of the partial ACs of Hazard and Vulnerability for GS and LS do not affect the global AC whereas the decrease of the partial AC for minimum clearance (MC) in the range M to L leads to a decrease of the global AC from H to M-H.
Figure 9. Sensitivity analysis for the Italian method applied to Borgoforte Bridge; for Staverton Bridge no variation was observed. MC = Minimum Clearance, GS = General Scour, LS = Local Scour.
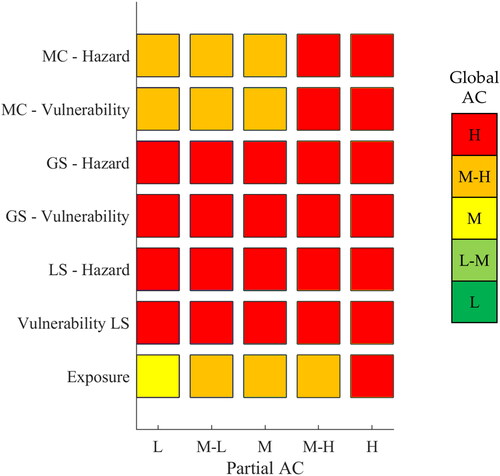
The most important hydraulic-related phenomenon appears to be the minimum vertical clearance. This depends on the combination rule considered in the guidelines where the partial ACs for general and local scour are further combined to obtain the global AC for scour (see ) whereas the partial AC for minimum clearance contributes directly to the evaluation of the global AC. As for the Staverton Bridge, any variation of a single AC does not modify the Global AC. The main reason for this result is that the ACs for minimum clearance and scour are both H: even if one of them lowers, the Global AC is H (note that the global AC is assigned as the most severe AC between the AC for minimum clearance and the AC for scour).
5. Discussion on compatibility of approaches
This work is one of the first to illustrate and show how to apply the latest UK (2021) and Italian (2020) guidelines to bridges subjected to scour and other hydraulic actions. Both methods are risk-based and produce compatible results, when comparing the approaches directly against each bridge. The scour risk obtained for Staverton Bridge resulted as High using both methods; the score for Borgoforte Bridge resulted higher for the Italian method, as compared to the UK method. The UK method categorised Borgoforte bridge as medium (40), which means that only standard monitoring is required; the Italian method assessed Borgoforte bridge as Medium-High risk, but it is necessary to finalize Level 2 of the analysis to understand which actions to take (and therefore combine the ACs relevant to all risk types). Additional national codes are available worldwide and future research could advance additional case studies for comparison.
In general, it is the hydraulic action assessment that showed significant differences between both methods: the Italian approach assessed Staverton as Medium-High hazard for minimum clearance, whilst the UK approach considers this bridge unlikely to be submerged. The main difference can be identified in the way the two methods assess the vulnerability of this particular hydraulic phenomenon: the UK method will consider bridge submergence only if the upstream water depth is higher than the bridge soffit (or the specific energy of the flow through the bridge is higher than the bridge soffit height). On the other hand, for the Italian approach, even if the design flow will not reach the bridge soffit, minimum clearance is required before lowering the rating, making the Italian method more conservative than the UK approach.
It can be observed that the combination procedure for ACs proposed by the Italian guidelines adopts a conservative approach, which accounts for the uncertainties due to the qualitative level of its method. For instance, the highest value between the AC for insufficient vertical clearance and the AC for scour is selected as the global AC. However, a High AC for hydraulic actions does not necessarily imply that the global AC of the bridge would be High overall. Indeed, in the Italian guidelines, the global AC for hydraulic risk must be combined with the ACs for the structure-foundation risk, seismic risk, and landslide risk, and often it is the AC for structure-foundation risk which has the highest impact on the evaluation of the global AC, as highlighted in Santarsiero et al. (Citation2021).
It should also be noted that the Italian method accounts for contraction and local scour in the hazard computation of the partial AC, whilst general scour is considered for the assessment of the vulnerability. The UK approach instead calculates the depth of scour due to each phenomenon and estimates the risk as a compound value. Whilst the UK method also considers channel stability around a bridge, this is not included in the Italian approach, making it a potential candidate for future implementation in further iterations. Another interesting observation relates to the sensitivity of the two approaches to the variations of the factors that affect the risk evaluation. The UK method seems to have a low sensitivity to the variation of the assessment factors, whereby the scour risk rating does not change for either bridge, despite varying all heuristic factors across the existing range. The same behaviour could not be observed for the Italian procedure as the AC for hydraulic risk is affected by the partial ACs, as highlighted in Sec. 4.3. Thus, it can be inferred that the response from the UK method is less sensitive than the Italian one, as it is unlikely that the risk rating would change with changing values within the priority factor. It should be noted, though, that the two bridges reside at two opposite ends of the scour spectrum, i.e., Staverton bridge with a very high relative scour depth and Borgoforte bridge with a low one. Therefore, other structures with intermediate conditions might display a more marked sensitivity than witnessed in these two case studies.
This work focuses on two specific case studies; however, national agencies manage thousands of bridges in their portfolios. In this respect, the main drawback of both methods is that the final classification includes five risk classes without a ranking. The lack of a ranking means that a prioritisation process among structures is challenging (e.g., which bridges are at most risk in the high-risk category?). The Italian method was applied considering scour and hydraulic risk only, and future studies should verify the landslide, seismic, and structure-foundation risk as well, according to the complete multi-risk procedure. In the UK, the guideline is not multi-risk: despite the UK not being a seismic region, the landslides and structural risk would be relevant and could be considered. Both the UK and Italy are countries with relevant heritage (e.g., the 40% of UK bridges are historical assets, Sasidharan et al., Citation2021); however, neither approach considers this aspect. This could be an additional element to integrate into the evaluation of the ‘importance’ of the bridge, by updating how exposure is evaluated. In recent years, increasing emphasis has been put on vertical contraction scour, i.e. the erosion depth caused by pressurised flow under bridges (Majid & Tripathi, Citation2021). Nevertheless, neither the Italian nor the UK method consider vertical contraction scour, which could be included for further estimation of the scour depth. Finally, the UK method could also be improved by reviewing the Priority Factor, e.g., including direct costs such as the cost of repairing/replacement or costs associated with affected people.
The availability of data (e.g., both the UK and Italian method requests a visual inspection at Level 1) could be a general barrier to the application of a methodology, especially in a context of fragmented bridge ownership and lack of national bridge databases. For example, the UK method requires a topographical survey of an affected structure, which could be difficult to achieve in large rivers where sonar (and other resources) might be required. Some automated data collection strategies, for example, automated ground penetrating radar using remote surveys, could supply additional information for the procedures, but this would require significant additional resources for each asset.
In line with the increasing role of monitoring for vulnerable structures (Giordano, Prendergast, & Limongelli, Citation2020), both methods recommend monitoring in their guidance. The last part of the Italian method (Sec. 7) is dedicated to inspection procedures and monitoring. Specifically, in addition to scheduled inspection, the installation of monitoring systems is recommended for structures classified in the Medium-High or High ACs in Level 2 or strategic structures. The guidelines describe the general principles of SHM and refer to UNI TR 11634:Citation2016 for technical details. For the UK, a new part about a ‘monitoring plan’ for all structures is present, requiring regular monitoring for all structures falling within the Medium or High scour risk categories. The monitoring plan is required to periodically review the condition of the bridge, any change to existing scour holes, debris accumulations, or change in the river morphology next to the bridge. Also, neither of the analysed methods treat different bridge types (materials/static system) in the definition of failure modes; failure mode analysis would provide a more comprehensive risk-based outlook on potentially endangered bridges (e.g. to plan preventative maintenance or monitoring). However, both methods omit clearly relating monitoring and risk assessment, i.e. the practice of integration between monitoring data, failure analysis, and risk assessment could be addressed by future studies.
6. Conclusions
This paper demonstrates the application of two recently published guidance documents for assessing hydraulic actions (including scour) on bridges, namely the UK Design Manual for Roads and Bridges and the Italian Ministry of Infrastructure and Transport Guidelines. These guidelines were explained and applied to two bridges, the Staverton Bridge in the UK and the Borgoforte Bridge in Italy. This paper is the first to compare both of these guidelines, and the comparison of the methodologies and the results provides novel insights into the benefits and drawbacks of each approach. For example, the methodologies could be further improved by considering the relevance of heritage, which is currently neglected in each method.
The application and comparison of the two guidelines may support practitioners or researchers wishing to develop their own risk-based methods, and this paper could serve as a reference for authorities wishing to incorporate risk into existing codes of practice and guidance documents for scour assessment. Future work will consider expanding the comparison to other national best-practices or other relevant procedures.
Disclosure statement
No potential conflict of interest was reported by the authors.
Additional information
Funding
References
- AASHTO. (2020). LRFD Bridge Design Specifications. American Association of State Highway and Transportation Officials 9th Edition, Washington, DC. ISBN: 978-1-56051-738-2
- Abé, M., Shimamura, M., & Fujino, Y. (2014). Risk management and monitoring of Japanese railway bridges. Proceedings of the Institution of Civil Engineers - Forensic Engineering, 167(2), 88–98. doi:10.1680/feng.13.00022
- Adey, B., Hajdin, R., & Brühwiler, E. (2003). Risk-based approach to the determination of optimal interventions for bridges affected by multiple hazards. Engineering Structures, 25(7), 903–912. doi:10.1016/S0141-0296(03)00024-5
- Anderson, N. L., Ismael, A. M., & Thitimakor, T. (2007). Ground-penetrating radar: A tool for monitoring bridge scour. Environmental and Engineering Geoscience, 13(1), 1–10. doi:10.2113/gseegeosci.13.1.1
- Arneson, L. A., Zevenbergen, L. W., Lagasse, P. F., & Clopper, P. E. (2012). Evaluating scour at bridges (5th ed.). Report No. FHWA-HIF-12-003 HEC-18. Washington, DC: Federal Highway Administration, US Department of Transportation. Retrieved from https://www.fhwa.dot.gov/engineering/hydraulics/pubs/hif12003.pdf
- Ballio, F., Ballio, G., Franzetti, S., Crotti, G., & Solari, G. (2018). Actions monitoring as an alternative to structural rehabilitation: Case study of a river bridge. Structural Control and Health Monitoring, 25(11), e2250. doi:10.1002/stc.2250
- Blockley, D. I. (2011). Engineering safety. Proceedings of the Institution of Civil Engineers - Forensic Engineering, 164(1), 7–13. doi:10.1680/feng.2011.164.1.7
- BSI. (2002). BS EN 1990: Eurocode basis of design. London, UK: BSI.
- BSI. (2005). BS EN 1991-1-6: 2005 Eurocode 1. General actions. Actions during execution. London, UK: BSI.
- BSI. (2006). BS EN 1990: BS EN 1991-1-7: Eurocode 1: Actions on structures – Part 1-7: General actions – accidental actions. London, UK: BSI.
- Campos, J. C., Casas, J. R., & Fernandes, S. (2016). COST Action TU1406 quality specifications for roadway bridges (BridgeSpec). In Maintenance, Monitoring, Safety, Risk and Resilience of Bridges and Bridge Networks: Proceedings of the 8th International Conference on Bridge Maintenance, Safety and Management (IABMAS 2016), Foz do Iguazu, Brazil. The Netherlands: CRC Press/Balkema Taylor & Francis Group.
- CIRIA. (2017). Manual on scour at bridges and other hydraulic structures (C742) (2nd ed.). London, UK: CIRIA.
- CSLP. (2020). Linee Guida per la Classificazione e Gestione del Rischio, la Valutazione della Sicurezza ed il Monitoraggio dei Ponti Esistenti. Ministero delle Infrastrutture e dei Trasporti. Consiglio Superiore dei Lavori Pubblici. Retrieved from https://bit.ly/3qiwRDR (In Italian)
- D.Lgs. (2010, February 23). n. 49. Attuazione della direttiva 2007/60/CE relativa alla valutazione e alla gestione dei rischi di alluvioni. . Retrieved from https://bit.ly/309dL8K.
- De Matteis, G., Bencivenga, P., & Zizi, M. (2021, August 29– September). Structural risk assessment of existing road bridges according to Italian Guidelines based on a territorial case study. In EUROSTRUCT 2021, Padova, Italy.
- Éupolis Lombardia. (2013). Valutazione del ciclo di vita delle infrastrutture sensibili con selezione degli interventi necessari - Allegato B. Schede analitiche sui ponti – analisi economico-territoriale e trasportistica. Istituto superiore per la ricerca, la statistica e la formazione. Retrieved from https://bit.ly/3wo6qhc. (in Italian).
- Farreras-Alcover, I., Andersen, J. E., & McFadyen, N. (2016). Assessing temporal requirements for SHM campaigns. Proceedings of the Institution of Civil Engineers – Forensic Engineering, 169(2), 61–71. doi:10.1680/jfoen.15.00015
- FHWA. (2004). National bridge inspection standards. Washington, DC: 23 CFR Part 650, Federal Highway Administration (FHWA), U.S. Department of Transportation.
- Giordano, P. F., Prendergast, L. J., & Limongelli, M. P. (2020). A framework for assessing the value of information for health monitoring of scoured bridges. Journal of Civil Structural Health Monitoring, 10(3), 485–496. doi:10.1007/s13349-020-00398-0
- Giordano, P. F., Prendergast, L. J., & Limongelli, M. P. (2022). Quantifying the value of SHM information for bridges under flood-induced scour. Structure and Infrastructure Engineering (Online), 1–17. doi:10.1080/15732479.2022.2048030
- Hamill, L. (1999). Bridge hydraulics. London: E.& F.N. Spon.
- Highways Agency (HA). (2012). The assessment of scour and other hydraulic actions at highway structures BD97/12. Design manual for roads & bridges. London, UK: HMSO. Retrieved from https://www.standardsforhighways.co.uk/prod/attachments/8ff7a31b-1ce0-4e34-9e94-b2372f125f34?inline=true
- Khakzad, N., & van Gelde, P. (2016). Re-Gen risk assessment of ageing infrastructure. Risk optimization in road infrastructure elements. Deliverable No. 4.2, January 2016. CEDR Transnational Road Research Programme. Retrieved from https://www.cedr.eu/download/other_public_files/research_programme/call_2013/ageing_infrastructure/re-gen/D4.2-Risk-Optimisation-in-Road-Infrastructure-Elements.pdf
- Klinga, J. V., & Alipour, A. (2015). Assessment of structural integrity of bridges under extreme scour conditions. Engineering Structures, 82, 55–71. doi:10.1016/j.engstruct.2014.07.021
- Lamb, R., Garside, P., Pant, R., & Hall, J. W. (2019). A probabilistic model of the economic risk to Britain's railway network from bridge scour during floods. Risk Analysis : An Official Publication of the Society for Risk Analysis, 39(11), 2457–2478. doi:10.1111/risa.13370
- Maddison, B. (2012). Scour failure of bridges. Proceedings of the Institution of Civil Engineers – Forensic Engineering, 165(1), 39–52. doi:10.1680/feng.2012.165.1.39
- Majid, S. A., & Tripathi, S. (2021). Pressure-flow scour due to vertical contraction: A review. Journal of Hydraulic Engineering, 147(12), 03121002. doi:10.1061/(ASCE)HY.1943-7900.0001943
- Mn DOT. (2009, December). Bridge scour evaluation procedure for Minnesota bridge. Minnesota Department of Transportation. Retrieved from https://www.dot.state.mn.us/bridge/pdf/hydraulics/ScourGuidelines_12-09.pdf
- Nasr, A., Kjellström, E., Björnsson, I., Honfi, D., Ivanov, O. L., & Johansson, J. (2020). Bridges in a changing climate: A study of the potential impacts of climate change on bridges and their possible adaptations. Structure and Infrastructure Engineering, 16(4), 738–749. doi:10.1080/15732479.2019.1670215
- NTC. (2018). Ministry of Infrastructure Decree, DM 17 gennaio 2018: Aggiornamento delle Norme tecniche per le costruzioni, Suppl. or. n.30 alla G.U. n.29 del 4/2/2008 (in Italian)
- Panici, D., Kripakaran, P., Djordjević, S., & Dentith, K. (2020). A practical method to assess risks from large wood debris accumulations at bridge piers. The Science of the Total Environment, 728, 138575. doi:10.1016/j.scitotenv.2020.138575
- Pregnolato, M. (2019). Bridge safety is not for granted – A novel approach for bridge management. Engineering Structures, 196, 109193. doi:10.1016/j.engstruct.2019.05.035
- Pregnolato, M., Vardanega, P. J., Limongelli, M. P., Giordano, P. F., & Prendergast, L. J. (2020). Risk-based scour management: A survey. In H. Yokota & D. M. Frangopol (Eds.), Bridge Maintenance, Safety, Management, Life-Cycle Sustainability and Innovations: Proceedings of the 10th International Conference on Bridge Maintenance, Safety and Management (IABMAS 2020) (pp. 693–701). Sapporo, Japan, April 11–15, 2021. The Netherlands: CRC Press/Balkema Taylor & Francis Group. doi:10.1201/9780429279119-91
- Prendergast, L. J., & Gavin, K. (2014). A review of bridge scour monitoring techniques. Journal of Rock Mechanics and Geotechnical Engineering, 6(2), 138–159. doi:10.1016/j.rmge.2014.01.007
- Santarsiero, G., Masi, A., Picciano, V., & Digrisolo, A. (2021). The Italian guidelines on risk classification and management of bridges: Applications and remarks on large scale risk assessments. Infrastructures, 6(8), 111. doi:10.3390/infrastructures6080111
- Sasidharan, M., Parlikad, A. K., & Schooling, J. (2021). Risk-informed asset management to tackle scouring on bridges across transport networks. Structure and Infrastructure Engineering, 1–17. doi:10.1080/15732479.2021.1899249
- Selvakumaran, S., Plank, S., Geiß, C., Rossi, C., & Middleton, C. (2018). Remote monitoring to predict bridge scour failure using Interferometric Synthetic Aperture Radar (InSAR) stacking techniques. International Journal of Applied Earth Observation and Geoinformation, 73, 463–470. doi:10.1016/j.jag.2018.07.004
- Takano, H., & Pooley, M. (2021). New UK guidance on hydraulic actions on highway structures and bridges. Proceedings of the Institution of Civil Engineers - Bridge Engineering, 174(3), 231–238. doi:10.1680/jbren.20.00024
- TDT. (1993). Texas secondary evaluation and analysis for scour (TSEAS). Austin, TX: Texas Bridge Scour Program, Texas Department of Transportation (TDT), Division of Bridges and Structures.
- UNI TR 11634. (2016). Linee Guida per il monitoraggio strutturale. Retrieved from https://bit.ly/3o5pUUg (in Italian)
- Vardanega, P. J., Gavriel, G., & Pregnolato, M. (2021). Assessing the suitability of bridge-scour-monitoring devices. Proceedings of the Institution of Civil Engineers – Forensic Engineering, 174(4), 105–117. doi:10.1680/jfoen.20.00022
- Whitbread, J. E., Benn, J. R., & Hailes, J. M. (2000). Cost-effective management of scour-prone bridges. Proceedings of the Institution of Civil Engineers – Transport, 141(2), 79–86. doi:10.1680/tran.2000.141.2.79