ABSTRACT
Objective: Imatinib mesylate (IM), a tyrosine kinase inhibitor, exhibits clinically prominent effects against chronic myeloid leukemia (CML); however, a few patients have shown resistance to IM treatment, resulting in disease progression. Smad4 is a tumor inhibitor that transduces TGF-β signaling and modulates genomic stability. Previous studies have indicated that decreased Smad4 expression played a bidirectional role in chemosensitivity in many types of cancers. Therefore, this study aims to evaluate the association between IM sensitivity and decreased Smad4 expression in human CML K562 cells.
Methods: Bone marrow (BM) samples were acquired from the patients prior to treatment. qRT-PCR, Western Blotting (WB), colony formation assay (CFA), and apoptosis assay were used to detect relevant indices.
Results: Smad4 expression was downregulated in the bone marrow and plasma of patients with multidrug-resistant CML as well as IM-resistant K562 (K562R) cells compared with samples collected from CML patients and K562 cells. Smad4 overexpression inhibited IM-treated K562R cell proliferation and augmented apoptosis, whereas Smad4 silencing promoted viability and inhibited apoptosis in IM-treated K562 cells. In addition, Smad4 expression was inversely correlated with laminin subunit gamma 1 (LAMC1) expression. The upregulation or downregulation of LAMC1 expression partially abolished the effect of Smad4 overexpression or silencing on the IM resistance of CML cells.
Conclusion: The downregulation of Smad4 expression might induce drug resistance in CML cells and displayed a possible mechanism through which Smad4 modulates CML cell survival and apoptosis upon IM treatment.
Introduction
Chronic myeloid leukemia (CML) is a kind of clonal myeloproliferative disease [Citation1], in which hematopoietic stem/progenitor cells generally lose differentiation ability, and also their proliferation and apoptosis are out of control [Citation2]. BCR-ABL1, an oncoprotein, is a constitutively active tyrosine kinase that is produced through the reciprocal translocation of the Philadelphia chromosome [Citation3]. It can transform pluripotent hematopoietic stem cells and induce CML [Citation4]. Discovering BCR-ABL1 (oncoprotein)-targeted tyrosine kinase inhibitors (TKIs) has completely revolutionized CML treatment [Citation5]. However, it was hypothesized that leukemia stem cells are not sensitive to TKIs, which cannot stop malignant cell growth, finally resulting in blast transformation, which can be lethal [Citation6]. CML patients fail to respond to treatment after blastic transformation because this transformation results in enhanced resistance to chemotherapeutic drugs [Citation7]. Thus, improving the sensitivity of CML cells may provide new treatment methods.
Although the use of imatinib mesylate (IM) has improved the long-term survival of most CML patients, several studies have shown that a few patients exhibit resistance to IM therapy, resulting in disease progression [Citation8, Citation9]. The mechanism underlying the development of this resistance comprises a complicated network involving many molecular and cellular signaling pathways.
Smad4 is reported as a tumor suppressor that exerted its function via transducing TGF-β and BMP signaling [Citation10]. Decreased Smad4 expression is caused by a combination of mutations, copy number loss, and transcriptional downregulation, and it has been observed in many malignancies, including non-small-cell lung cancer (NSCLC) [Citation11–13]. Decreased intensity of Smad4 immunostaining has been found to be associated with decreased survival of patients with lung and pancreatic cancers [Citation13,Citation14]. The role of Smad4 in suppressing tumors has been demonstrated in animal models in which Smad4 deprivation resulted in the formation of new tumors [Citation15] and facilitated the development of oncogene-induced lesions [Citation16] and metastases [Citation17]. However, the effect of Smad4 on tumor chemosensitivity is controversial. In human lymphoma cells, Smad4 silencing improves their chemosensitivity to adriamycin by suppressing the activation of the TGF-β signaling pathway [Citation18], while in colorectal cancer, Smad4 deprivation elicits resistance to 5-fluorouracil through the Akt pathway [Citation19].
The results of this study indicated that the downregulation of Smad4 expression in CML cells was possibly associated with the progression of the disease and caused IM resistance by regulating laminin subunit gamma 1 (LAMC1) expression. Downregulation of Smad4 expression was observed in samples collected from patients with multidrug-resistant (MDR) CML and IM-resistant CML K562 (K562R) cells. Therefore, Smad4 might be a biomarker candidate for the treatment of CML patients with the combined use of a LAMC1 inhibitor and IM-based chemotherapy.
Material and methods
Patients’ samples
This study has been approved by the Ethics Committee of Jingzhou Central Hospital. In total, 31 patients recently diagnosed with chronic phase CML at the Jingzhou Central Hospital were enrolled in the present study after signing informed consent forms. Bone marrow (BM) tissues were acquired from the patients prior to treatment. CML was strictly diagnosed based on the results of morphological tests, multiparameter flow cytometry (FC), and cytogenetics. P-gp expression was detected using FC to confirm the resistant phenotype. Patients who did not have complete clinical records and displayed disease progression were excluded. The study included two groups: non-MDR CML (5 men and 4 women; median age, 53 years) and CML/MDR (15 men and 6 women; median age, 51 years). The study was approved by the ethics committee of Jingzhou Central Hospital. Mononuclear cells were separated from BM specimens and incubated in plastic dishes at 37°C for 1 day to remove adherent cells. The viability of non-adherent cells was evaluated using trypan blue exclusion test. Newly isolated cells were maintained in modified Dulbecco's medium containing 10% FBS, 50 ng/mL stem cell factor, 10 ng/mL IL-3, 10 ng/mL IL-6, 10 mM β-mercaptoethanol, and 2 mM L-glutamine. ST3GAL4 levels were measured using qRT-PCR.
Cell cultivation and K562R cell line establishment
CML K562 cells were obtained from KeyGEN (Nanjing, China) and cultivated in RPMI 1640 medium supplemented with 10% heat-inactivated FBS and 1% penicillin–streptomycin in a humidified atmosphere containing 5% CO2 at 37°C. Stable K562R cells were obtained through stepwise treatment of parental K562 cells with increasing drug concentrations (from 0.1 to 1 μM) for 6 months. IM (1 μM) was added to the complete medium of K562R cell clones to maintain selection pressure. Moreover, K562R cells exhibited resistance to other chemotherapeutic agents. K562R cells (>90% viability, Supplementary Figure S1) were rinsed via washing and low speed centrifugation to remove IM before subsequent experiments. K562R clonal sublines retained their drug resistance phenotype even after long-term storage in liquid N2.
Transfection
shRNA-control (5′-GAC GCT AAT GCT AGC-3′), shRNA-Smad4 (5′-ATT GAA AGT TTG GTA AAG AAG CTG AAG GA-3′), and shRNA-LAMC1 (5′-TCT AGA AAT CGG GAA CAA AGG GTT TT-3′) were synthesized by Shanghai GenePharma Co., Ltd. Plasmid production and purification were performed by Shanghai GenePharma Co., Ltd. Full length frame of Smad4 (NM_005359.6) and LAMC1 (NM_002293.4) were cloned into the pcDNA3.1 and pCMV, respectively. Cells transfection was carried out using Lipofectamine® 2000 (Invitrogen; Thermo Fisher Scientific, Inc., Waltham, MA, USA) according to manufacturer's protocol. The cells with different transfection were harvested at 36 h post-transfection, or used for further investigations. The transfection efficiency was additionally identified via qRT-PCR.
qRT-PCR
Total RNA was isolated from samples through TriPure Isolation Reagent (Roche Diagnostics, Basel, Switzerland) as the relevant guidance and was reversely transcribed to first-strand cDNA through cDNA Synthesis Kit (Thermo Fisher Scientific, Waltham, MA, USA). Real-time PCR was conducted with GAPDH as the internal reference, and the 2−ΔΔCt method was used to determine the relative expression of mRNA.
Western Blotting (WB)
Total protein was isolated with NP-40 lysis buffer (Beyotime) and its concentration was measured through Enhanced BCA Assay Kit (Beyotime). Samples (40 mg) were treated with SDS-PAGE. Subsequently, the isolated protein was added to PVDF membranes (Millipore, Bedford, MA, USA), which were then blocked with 5% non-fat milk and incubated with primary antibodies overnight at 4°C, followed by incubation with goat anti-rabbit or anti-mouse HRP-labeled secondary antibodies for 45 min at 37°C. Next, the protein blots were developed using an ECL Detection System (Seven-sea pharmtech Co., Ltd, Shanghai, China) and protein expression was standardized to that of GAPDH by densitometric analysis.
Colony formation assay (CFA)
Cells in RPMI 1640 mixed equally with 1% low-melting-point agar were seeded onto 24-well plates and cultivated in a humidified atmosphere of 5% CO2 at 37°C for 7 days. The surviving colonies were counted following fixation with methanol/acetone (1:1) and were finally stained with 0.5% methylene blue (MedChemExpress). The colonies obtained were counted using a Nikon TE200 microscope (10× objective).
Apoptosis assay
Apoptosis in K562/K562R cells was determined through Annexin V-FITC/PI Kit (BD Biosciences, Franklin Lakes, NJ, USA) according to the manufacturer's protocol. In brief, cells (5.0 × 105) were rinsed with ice cold PBS, suspended in binding buffer (195 μL), and stained with Annexin V-FITC (5 μL) for 10 min at RT. Cells were rinsed with binding buffer to remove the free Annexin V antibody. The percentage of apoptotic K562/K562R cells was measured using a FACSCalibur cytometer (BD Biosciences) and investigated using CellQuest software (BD Biosciences).
Statistical analysis
Data are expressed as the mean ± SEM, unless otherwise noted, and were statistically analyzed using SPSS (version 13.0, SPSS Inc.). We conducted t-test and ANOVA to analyze the significance of differences between two groups and among multiple groups, respectively. Statistical significance was set at P < 0.05.
Results
Smad4 expression is downregulated in MDR CML patient samples and K562R cells
Several previous studies have indicated that Smad4 plays a critical role in tumor progression [Citation20]. To investigate the role of Smad4 in CML occurrence, we determined its levels in the plasma and BM specimens of CML patients and healthy volunteers. We found that Smad4 expression in the plasma and BM specimens of CML patients was lower than that in specimens from healthy volunteers ((A,B)). Next, we analyzed Smad4 levels in the plasma and BM of patients with non-MDR CML and patients with chemoresistant CML (CML/MDR). The results indicated that the levels of Smad4 in the plasma and BM samples of patients with CML/MDR were lower than those in CML samples ((C,D)). We then determined Smad4 expression in K562 and K562R cells. The data demonstrated that Smad4 levels were lower in K562R cells than in K562 cells ((E,F)). Collectively, our findings indicate that Smad4 expression is downregulated in samples from patients with chemoresistant CML and drug-resistant cell lines, suggesting a potential role for Smad4 in CML chemoresistance.
Figure 1. Smad4 expression in the plasma and BM of patients with CML and K562 cells. (A) Smad4 mRNA levels in the plasma of patients with CML (n = 30) and healthy volunteers (n = 9) were determined by qRT-PCR. (B) Smad4 mRNA levels in the BM of patients with CML (n = 30) and healthy volunteers (n = 9) were determined by qRT-PCR. (C) Smad4 expression was investigated by qRT-PCR in the plasma from patients with CML/MDR (n = 21) or CML (n = 9). (D) Smad4 expression was investigated by qRT-PCR in the BM from patients with CML/MDR (n = 21) or CML (n = 9). (E, F) Smad4 expression in K562 and K562R cells were determined by qRT-PCR and WB. *p < .05, **p < .01, compared with indicated group.
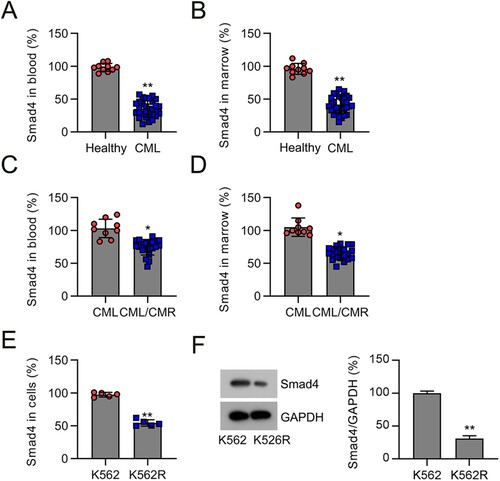
Knockdown and overexpression of Smad4 affects the survival and apoptosis of IM-treated K562 and K562R cells
To investigate the association between Smad4 levels and drug resistance and leukemia cell survival, cell lines with low or high Smad4 expression were established. K562 and K562R cells were transfected with Smad4 shRNA or pcDNA3.1-Smad4 to knockdown or overexpress Smad4, respectively. Effective silencing or overexpression of Smad4 was validated by qRT-PCR and WB ((A,B)).
Figure 2. Expression of Smad4 in K562 and K562R cells transfected with shRNA-control, shRNA-Smad4, pcDNA3.1-control, or pcDNA3.1-Smad4 for 24–36 h. n = 5. (A, B) Smad4 expression in K562 and K562R cells was determined by qRT-PCR and WB. WB pictures shown in the Figures are representative from multiple performed immunoblots. n = 3. ***p < .001, compared with indicated group.
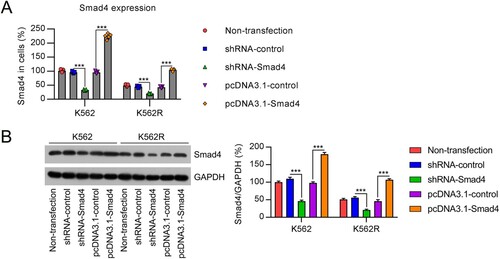
The changes in cell growth following Smad4 knockdown or overexpression were evaluated by CFA. Treatment of K562 cells with IM significantly reduced the number of cell colonies compared with untreated cells. No such effect was observed in K562R cells. Silencing of Smad4 in K562 cells partially restored the cell colony number after IM treatment, while Smad4 overexpression did not show any notable effect. In K562R cells, knockdown of Smad4 further augmented the number of cell colonies after IM treatment, but overexpression of Smad4 caused a drastic reduction in their number ((A)).
Figure 3. Influence of Smad4 on cell survival and apoptosis in IM-treated K562 and K562R cells. K562 and K562R cells were transfected with shRNA-control, shRNA-Smad4, pcDNA3.1-control, and pcDNA3.1-Smad4 for 24 h and then exposed to 0.5 μM IM for 24 h. (A) Cell growth rate was assessed by CFA. n = 5. (B) Cell apoptosis rate was determined by FC with Annexin V-FITC/PI staining. n = 3. (C) Expression of Bax and Bcl-2 proteins were examined by WB. WB pictures shown in the Figures are representative from multiple performed immunoblots. n = 3. *p < .05, **p < .01, ***p < .001, compared with indicated group.
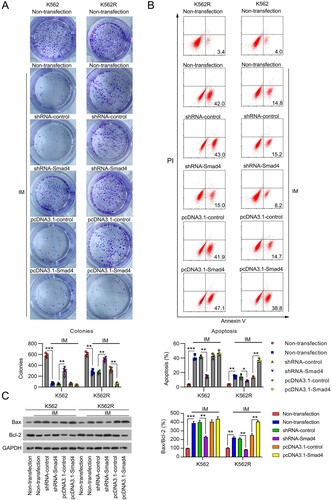
Next, we examined the percentage of apoptotic K562 and K562R cells subjected to Smad4 silencing or overexpressing Smad4 using flow cytometry. At first, the administration of IM led to a significant increase in the percentage of apoptotic K562 cells. In contrast, the percentage of apoptotic K562R cells was reduced. Apoptosis in both K562 and K562R cells was found to be significantly reduced after Smad4 knockdown, whereas it was further augmented by Smad4 overexpression in K562R cells only ((B)). WB analysis showed that IM administration increased the expression of Bax and decreased that of Bcl-2 in both cell lines. These changes were alleviated by Smad4 silencing in K562 and K562R cells but were further promoted by Smad4 overexpression in K562R cells ((C)). Overall, these results indicate that downregulation of Smad4 expression may induce chemoresistance in K562 cells by affecting the apoptotic pathway.
LAMC1 levels are inversely correlated with Smad4 levels in CML samples and cell lines
LAMC1 has been demonstrated to be a downstream effector of Smad4 in mediating TGF-β signaling [Citation21], and its oncogenic effect has been documented in many previous studies [Citation22–26]. However, whether and how it crosstalk with Smad4 affects the chemosensitivity of CML remains unclear. First, to address the correlation between LAMC1 and Smad4 expression, we examined the mRNA levels of LAMC1 in the plasma and BM samples of patients with CML and CML/MDR. The results demonstrated that LAMC1 levels in CML/MDR samples were higher than those in CML samples ((A,B)). qRT-PCR and WB analyses showed that LAMC1 expression was also upregulated in K562R cells at both the mRNA and protein levels, compared with K562 cells ((C,D)). To evaluate the correlation between LAMC1 and Smad4 expression, we examined the expression of LAMC1 in K562 and K562R cells using the above-mentioned transfection conditions. First, we confirmed that LAMC1 expression was higher in K562R cells than in K562 cells under all transfection conditions. Knockdown of Smad4 resulted in upregulation of LAMC1 expression in both K562 and K562R cells; in contrast, LAMC1 expression was reduced in Smad4-overexpressing cells ((E,F)). Collectively, these results demonstrate an inverse correlation between Smad4 and LAMC1 expression in CML cell lines.
Figure 4. Correlation of LAMC1 and Smad4 expression in samples from patients with CML and cells. (A) LAMC1 expression was examined by qRT-PCR in the plasma of patients with CML/MDR (n = 21) or CML (n = 9). (B) LAMC1 expression was examined by qRT-PCR in the BM from patients with CML/MDR (n = 21) or CML (n = 9). (C, D) LAMC1 expression in K562 and K562R cells was determined by qRT-PCR and WB. n = 5. (E, F) K562 and K562R cells were transfected with shRNA-control, shRNA-Smad4, pcDNA3.1-control, and pcDNA3.1-Smad4 for 36 h. LAMC1 expression was determined by qRT-PCR (n = 5) and WB (n = 3). WB pictures shown in the Figures are representative from multiple performed immunoblots. *p < .05, **p < .01, compared with indicated group.
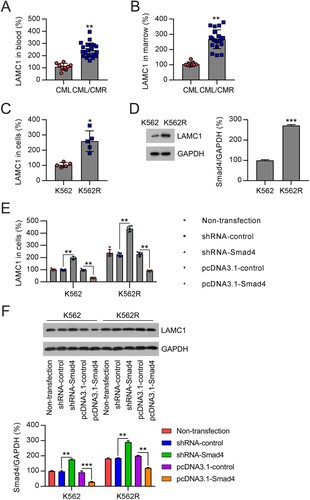
Effect of Smad4 on the chemosensitivity of K562 cells is LAMC1-dependent
To assess whether LAMC1 is involved in Smad4 loss-induced chemoresistance of K562 cells, we silenced LAMC1 in Smad4-knockdown K562 and K562R cells and overexpressed LAMC1 in Smad4-overexpressing cells. qRT-PCR and WB analyses revealed that LAMC1 expression was downregulated in cells transfected with shRNA-LAMC1, and a significant increase in LAMC1 expression was observed in cells transfected with pCMV-LAMC1 ((A–D), Supplementary Figure 2A).
Figure 5. Compensation of LAMC1 in CML cells with altered expression of Smad4. (A, B) K562 and K562R cells were co-transfected with shRNA-Smad4 and shRNA-control or shRNA-LAMC1 for 36 h. LAMC1 expression in K562 and K562R cells was determined by qRT-PCR and WB. n = 5. (C, D) K562 and K562R cells were co-transfected with pcDNA3.1-Smad4 and pcDNA3.1-control or pcDNA3.1-LAMC1 for 36 h. LAMC1 expression was determined by qRT-PCR and WB. WB pictures shown in the Figures are representative from multiple performed immunoblots. n = 3. **p < .01, ***p < .001, compared with indicated group.
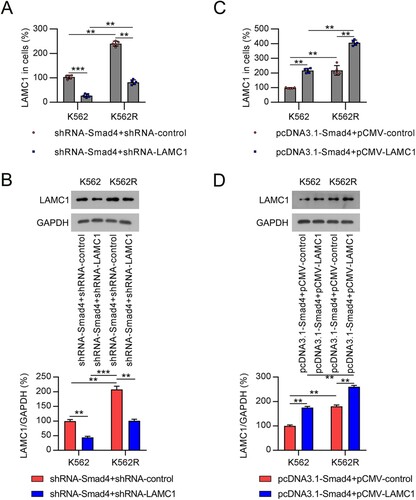
Next, CFA was used to detect the role of LAMC1 silencing in the effect of Smad4 on the growth of IM-treated cells. It was found that knockdown of LAMC1 led to a decreased number of colonies in both K562 and K562R cells with Smad4 knockdown ((A), Supplementary Figure 2B). Consistently, LAMC1 silencing further increased the percentage of apoptotic IM-treated K562 and K562R cells, which was alleviated by Smad4 knockdown ((B)). Bax expression in both cell lines was elevated, whereas that of Bcl-2 was reduced upon LAMC1 knockdown ((C)).
Figure 6. Involvement of LAMC1 in the Smad4-modulated cell survival and apoptosis in IM-treated CML cells. (A) K562 and K562R cells were co-transfected with shRNA-Smad4 and shRNA-control or shRNA-LAMC1 for 24 h, and then exposed to 0.5 μM IM for 1 d. Cell growth rate was assessed by CFA. n = 5. (B) Cell apoptosis rate was determined by FC with Annexin V-FITC/PI staining. n = 3. (C) Expression of Bax and Bcl-2 proteins was examined by WB. n = 3. (D) K562 and K562R cells were co-transfected with pcDNA3.1-Smad4 and pcDNA3.1-control or pcDNA3.1-LAMC1 for 24 h, and then exposed to 0.5 μM IM for 1 d. Cell growth rate was assessed by CFA. n = 5. (E) Cell apoptosis rate was determined by FC with Annexin V-FITC/PI staining. n = 3. (F) Expression of Bax and Bcl-2 proteins was examined by WB. WB pictures shown in the Figures are representative from multiple performed immunoblots. n = 3. *p < .05, **p < .01, compared with indicated group.
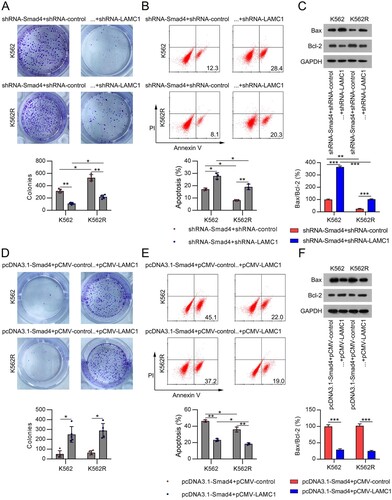
We also observed that LAMC1 overexpression promoted colony formation in both cell lines with Smad4 overexpression and IM treatment, as determined by CFA ((D), Supplementary Figure 2B). FC and WB analyses showed that the percentage of apoptotic cells and Bax levels in Smad4-overexpressing and IM-treated K562 and K562R cells were reduced following LAMC1 expression, while the levels of Bcl-2 were restored ((E,F)). Taken together, these results suggest that the downstream effector of Smad4, LAMC1, is involved in the Smad4-regulated chemosensitivity of CML cells.
Discussion
This study showed that Smad4 knockdown can lead to upregulation of the expression of LAMC1, thereby enhancing the chemoresistance of CML cells to IM. Chemotherapy is the most common treatment for cancer [Citation27]. The role of IM in suppressing neoplastic transformation in chronic myeloid leukemia and facilitating survival have been confirmed [Citation28], in addition to its effects on CML [Citation29]. A previous study demonstrated the role of differential expression and genetic variants of TGFβ-Smad pathway in CML. Decreased TGFβR2 and SMAD4 levels observed in the present study may be responsible for reduced tumor suppressive effects of this pathway in CML [Citation30], which is in agreement with our findings, regarding the expressing profiling and role of Smad4 in CML. Additionally, we presented critical evidence confirming that decreased Smad4 expression could inhibit the apoptosis of IM-treated CML cells through negative modulation of LAMC1 expression. These results indicate that Smad4 may play a role in IM treatment in CML.
As mentioned above, Smad4 overexpression, deficiency, or mutation is associated with the growth, metastasis, and resistance to radiotherapy or chemotherapy of multiple tumors [Citation20, Citation31–33]. However, whether Smad4 is a tumor suppressor or an oncogene remains controversial. Our study showed that Smad4 expression was decreased in the plasma and BM of patients with CML. Notably, in K562R cells and samples from patients with MDR CML, Smad4 expression was significantly reduced. Silencing Smad4 in K562 cells reduced chemoresistance to IM, while elevated Smad4 levels in K562R cells promoted apoptosis, suggesting that Smad4 may be a tumor suppressor in CML.
LAMC1 encodes the laminin γ1 chain and has been shown to have oncogenic effects on several malignancies. An impartial random survival forest analysis of 247 subjects with hepatocellular carcinoma suggested that increased LAMC1 levels were positively correlated with terminal tumor staging and short overall and non-tumor survival [Citation34]. In a multiple myeloma study, LAMC1 gene in a prednisone-resistant myeloma cell subline was dramatically overexpressed, while it was decreased in melphalan-resistant variant [Citation35]. In prostate cancer, miR-22 directly targets LAMC1 [Citation36]. In glioblastoma, miR-124a directly modulates LAMC1 expression [Citation37]. In hepatocellular carcinoma, both SP1 and Wnt can transactivate the LAMC1 promoter [Citation38,Citation39]. These studies indicate that LAMC1 expression is usually regulated by another important regulator in many tumors. Here, we observed that LAMC1 expression was inversely regulated or correlated with Smad4 expression. As we have shown that Smad4 serves as a tumor suppressor in drug-resistant CML cells, LAMC1 might act as an oncogene participating in Smad4-regulated CML cell survival and apoptosis. To this end, we obtained the following lines of evidence: (1) LAMC1 knockdown resulted in reduced cell growth rate and increased apoptosis in Smad4-silenced and IM-treated K562 and K562R cells. (2) Overexpression of LAMC1 restored cell survival and alleviated Smad4-induced apoptosis. These results also suggest that the effect of Smad4 on the chemoresistance of CML cells is LAMC1-dependent.
In summary, this study provides insights into the mechanism of action of Smad4 in CML cells, in which Smad4 silencing might reduce the chemosensitivity of CML cells to IM by promoting LAMC1 expression. Moreover, these findings displayed a therapeutic potential and tumor suppressive role for Smad4 in CML. Nonetheless, follow-up animal research and clinical trials are needed for validating our results.
Supplemental Material
Download TIFF Image (1.2 MB)Supplemental Material
Download TIFF Image (67.4 KB)Disclosure statement
No potential conflict of interest was reported by the author(s).
Additional information
Funding
References
- Hamad, A., et al., Emerging therapeutic strategies for targeting chronic myeloid leukemia stem cells. 2013.
- Stapnes C, Gjertsen B, Reikvam H, et al. Targeted therapy in acute myeloid leukaemia: current status and future directions. Expert Opinion on Investigational Drugs. 2009;18(4):433–455.
- Ito TJIJOH. Stem cell maintenance and disease progression in chronic myeloid leukemia. Int J Hematol. 2013;98(6):641–647.
- Cross N, Daley G, Green A, et al. BCR-ABL1-positive CML and BCR-ABL1-negative chronic myeloproliferative disorders: some common and contrasting features. Leukemia. 2008;22(11):1975–1989.
- Perrotti D, Jamieson C, Goldman J, et al. Chronic myeloid leukemia: mechanisms of blastic transformation. J Clin Invest. 2010;120(7):2254–2264.
- El-Shami K, Smith BJL. Immunotherapy for myeloid leukemias: current status and future directions. Leukemia. 2008;22(9):1658–1664.
- Cea M, Cagnetta A, Nencioni A, et al. New insights into biology of chronic myeloid leukemia: implications in therapy. Curr Cancer Drug Targets. 2013;13(7):711–723.
- Hochhaus A, O'Brien A, Guilhot S, et al. Six-year follow-up of patients receiving imatinib for the first-line treatment of chronic myeloid leukemia. Leukemia. 2009;23(6):1054–1061.
- Pophali PA, Patnaik MMJCJ. The role of new tyrosine kinase inhibitors in chronic myeloid leukemia. Cancer J. 2016;22(1):40.
- Massagué J. TGFβ signalling in context. Nat Rev Mol Cell Biol. 2012;13(10):616–630.
- Malkoski SP, Wang X-J. Two sides of the story? Smad4 loss in pancreatic cancer versus head-and-neck cancer. FEBS Lett. 2012;586(14):1984–1992.
- Haeger SM, Thompson JJ, Kalra S, et al. Smad4 loss promotes lung cancer formation but increases sensitivity to DNA topoisomerase inhibitors. Oncogene. 2016;35(5):577–586.
- Liu J, Cho S-N, Akkanti B, et al. Erbb2 pathway activation upon Smad4 loss promotes lung tumor growth and metastasis. Cell Rep. 2015;10(9):1599–1613.
- Tascilar M, et al. The SMAD4 protein and prognosis of pancreatic ductal adenocarcinoma. Clin Cancer Res. 2001;7(12):4115–4121.
- Bornstein S, et al. Smad4 loss in mice causes spontaneous head and neck cancer with increased genomic instability and inflammation. J Clin Invest. 2009;119(11):3408–3419.
- Izeradjene K, Combs C, Best M, et al. Krasg12d and Smad4/Dpc4 haploinsufficiency cooperate to induce mucinous cystic neoplasms and invasive adenocarcinoma of the pancreas. Cancer Cell. 2007;11(3):229–243.
- Ding Z, Wu C-J, Chu GC, et al. SMAD4-dependent barrier constrains prostate cancer growth and metastatic progression. Nature. 2011;470(7333):269–273.
- Li Q, Liu X, Zhao C. Smad4 gene silencing enhances the chemosensitivity of human lymphoma cells to Adriamycin via inhibition of the activation of transforming growth factor β signaling pathway. J Cell Biochem. 2019;120(9):15098–15105.
- Zhang B, Zhang B, Chen X, et al. Loss of Smad4 in colorectal cancer induces resistance to 5-fluorouracil through activating Akt pathway. Br J Cancer. 2014;110(4):946–957.
- Zhao M, Mishra L, Deng CX. The role of TGF-β/SMAD4 signaling in cancer. Int J Biol Sci. 2018;14(2):111–123.
- Fang L, et al. LAMC1, upregulated by TGFβ in tumor cells, contributed to the formation of inflammatory cancer-associated fibroblasts via NF-kB/CXCL1/STAT3 in esophageal squamous cell carcinoma. 2021.
- Nishikawa R, et al. Tumor-suppressive microRNA-29s inhibit cancer cell migration and invasion via targeting LAMC1 in prostate cancer. Int J Oncol. 2014;45(1):401–410.
- Zhang Y, Xi S, Chen J, et al. Overexpression of LAMC1 predicts poor prognosis and enhances tumor cell invasion and migration in hepatocellular carcinoma. J Cancer. 2017;8(15):2992.
- Ye G, Qin Y, Wang S, et al. Lamc1 promotes the Warburg effect in hepatocellular carcinoma cells by regulating PKM2 expression through AKT pathway. Cancer Biol Ther. 2019;20(5):711–719.
- Kunitomi H, Kobayashi Y, Wu R-C, et al. LAMC1 is a prognostic factor and a potential therapeutic target in endometrial cancer. J Gynecol Oncol. 2020;31(2):e11.
- Liu J, Liu D, Yang Z, et al. High LAMC1 expression in glioma is associated with poor prognosis. Onco Targets Ther. 2019;12:4253.
- Brachet-Botineau M, Deynoux M, Vallet N, et al. A novel inhibitor of STAT5 signaling overcomes chemotherapy resistance in myeloid leukemia cells. Cancers (Basel). 2019;11(12):2043.
- Chalandon Y, Thomas X, Hayette S, et al. Randomized study of reduced-intensity chemotherapy combined with imatinib in adults with Ph-positive acute lymphoblastic leukemia. Blood. 2015;125(24):3711–3719.
- Kantarjian H, Cortes J, Jabbour E, et al. Chronic myeloid leukemia. Mol Hematol. 2019: 71–86.
- Shokeen Y, et al. Association between altered expression and genetic variations of transforming growth factor β-Smad pathway with chronic myeloid leukemia. Int J Hematol Oncol Stem Cell Res. 2018;12(1):14–22.
- Cheng Y, Li Z, Xie J, et al. MiRNA-224-5p inhibits autophagy in breast cancer cells via targeting Smad4. Biochem Biophys Res Commun. 2018;506(4):793–798.
- Wang F, Xia X, Yang C, et al. SMAD4 gene mutation renders pancreatic cancer resistance to radiotherapy through promotion of autophagy. Clin Cancer Res. 2018;24(13):3176–3185.
- Xia X, Wu W, Huang C, et al. SMAD4 and its role in pancreatic cancer. Tumour Biol. 2015;36(1):111–119.
- Désert R, Mebarki S, Desille M, et al. “Fibrous nests” in human hepatocellular carcinoma express a Wnt-induced gene signature associated with poor clinical outcome. Int J Biochem Cell Biol. 2016;81:195–207.
- Mutlu P, Ural AU, Gündüz U. Differential gene expression analysis related to extracellular matrix components in drug-resistant RPMI-8226 cell line. Biomed Pharmacother. 2012;66(3):228–231.
- Pasqualini L, Bu H, Puhr M, et al. miR-22 and miR-29a are members of the androgen receptor cistrome modulating LAMC1 and Mcl-1 in prostate cancer. Mol Endocrinol. 2015;29(7):1037–1054.
- Fowler A, Thomson D, Giles K, et al. miR-124a is frequently down-regulated in glioblastoma and is involved in migration and invasion. Eur J Cancer. 2011;47(6):953–963.
- Lietard J, et al. Sp1-mediated transactivation of LamC1 promoter and coordinated expression of laminin-gamma1 and Sp1 in human hepatocellular carcinomas. Am J Pathol. 1997;151(6):1663.
- Nagendran M, et al. Canonical Wnt signalling regulates epithelial patterning by modulating levels of laminins in zebrafish appendages. Development. 2015;142(2):320–330.