ABSTRACT
Objectives
Obstructive sleep apnea (OSA) is reported to be a cause of secondary polycythemia. The present study (i) reviewed the literature reporting the prevalence of secondary polycythemia in patients with OSA and (ii) determined the effect of continuous positive airway pressure (CPAP) therapy on hemoglobin and hematocrit levels in patients with OSA.
Methods
We searched MEDLINE, Embase and Cochrane for studies of adult patients with OSA that reported hemoglobin and/or hematocrit levels. We performed summary estimates of (i) polycythemia prevalence and a subgroup analysis according to OSA severity, and (ii) change in hemoglobin and hematocrit levels following treatment with CPAP.
Results
Synthesis of seven studies including 3,654 patients revealed an overall polycythemia prevalence of 2% (95% CI 1–4%); 2% (95% CI 1–3%) in mild-to moderate and 6 % (95% CI 3–12%) in severe OSA. In the pooled analysis of ten single-arm trials including 434 patients, CPAP treatment reduced hemoglobin by 3.76 g/L (95% CI −4.73 to −2.80 g/L). Similarly, pooled analysis of ten single-arm trials including 356 patients without baseline polycythemia showed that CPAP treatment reduced hematocrit by 1.1% (95% CI −1.4 to −0.9%).
Conclusion
Our pooled analysis supports an increased prevalence of secondary polycythemia in OSA. This estimated prevalence is likely underestimated due to the change in the polycythemia diagnostic criteria in 2016. Future randomized controlled trials are needed to evaluate the effect of CPAP in patients with baseline polycythemia.
HIGHLIGHTS
Pooled analysis shows OSA is associated with an increased prevalence of secondary polycythemia
Prevalence of polycythemia is greater in severe OSA
CPAP treatment for OSA reduces both the hemoglobin and hematocrit
1. Introduction
Obstructive sleep apnea (OSA) is a common sleep-related breathing disorder characterized by recurrent cessation (apnea) or reduction (hypopnea) in breathing during sleep caused by transient upper airway collapse [Citation1]. OSA may lead to excessive daytime sleepiness and impaired quality of life, as well as an increased risk of incident hypertension, cardiovascular and cerebrovascular disease through a combination of apnea-related pathophysiological mechanisms that include intermittent hypoxia, excessive swings in negative intra-thoracic pressure, and cortical arousals [Citation1]. Continuous positive airway pressure (CPAP), the gold standard therapy for OSA, can attenuate the effects of these pathophysiological mechanisms by abolishing apneas and hypopneas [Citation2,Citation3].
Polycythemia is defined as an increase in hemoglobin and/or hematocrit above normal, i.e. hemoglobin > 165 g/L in men and > 160 g/L in women; or hematocrit > 49% in men and > 48% in women [Citation4]. The terms polycythemia and erythrocytosis are often used interchangeably, and are classified as primary or secondary [Citation5]. Primary polycythemia, a.k.a. polycythemia vera, is caused by a myeloproliferative neoplasm that leads to autonomous excessive production of erythrocytes with subsequent suppression of erythropoeitin (EPO) levels [Citation5]. In contrast, in secondary polycythemia, EPO levels are increased, either inappropriately, such as with renal artery stenosis or tumors with ectopic EPO production, or appropriately, in response to hypoxia, as is the case in chronic lung disease or cyanotic heart disease [Citation5]. In a study of 2 large health databases in the U.S.A., the prevalence of primary polycythemia was 44–57 per 100,000 [Citation6]. In a large Dutch cohort, the prevalence of erythrocytosis of any cause was 0.3% using 2008 World Health Organization (WHO) criteria, and 3.4% using less strict 2016 WHO criteria [Citation7].
The sleep-related intermittent hypoxia associated with OSA is also a potential pathophysiological driver of secondary polycythemia, and this is reflected in hematological guidelines, which recommend routine evaluation for the presence of sleep-related breathing disorder as a possible causative factor in patients with polycythemia [Citation8–10]. However, while there is some evidence that EPO levels and hematocrit levels are higher in OSA patients compared to controls, the true prevalence of secondary polycythemia in OSA is uncertain with reported prevalence ranging widely from 0.3% to 8% [Citation11–15]. It is also uncertain whether treatment of OSA leads to reduction in hemoglobin and/or hematocrit levels. In light of the recognized association between elevated hematocrit and increased risk of cardiovascular disease [Citation16] evaluation of the impact of treatment of OSA on the hematocrit has potential clinical relevance.
The objectives of this meta-analysis were to: (i) systematically review the literature reporting the prevalence of secondary polycythemia in patients with OSA and (ii) determine the effect of CPAP therapy on hemoglobin and hematocrit levels in patients with OSA.
2. Materials and methods
2.1. Registration
This systematic review was prospectively registered in the PROSPERO international database (registration number CRD42020178296).
2.2. Eligibility criteria
We included studies of adult patients with OSA, confirmed by the use of in-laboratory polysomnography or home sleep testing, that reported hemoglobin and/or hematocrit levels. For objective (ii), we included studies that compared hemoglobin and/or hematocrit pre- and post-CPAP treatment. We excluded studies if they included patients with comorbidities known to affect hemoglobin and/or hematocrit levels, such as hematological disorders (anemia, hemoglobinopathy, hematological malignancy), chronic kidney or liver disease, exogenous testosterone, chronic hypoventilation, resting hypoxia, sleep-related breathing disorders other than OSA, or a procedure or transfusion in the previous three months. We included clinical trials, cohort studies, case–control studies and cross-sectional studies.
2.3. Search methods
A comprehensive search strategy was developed by a librarian and information specialist (A.O–C.) for the two core concepts of OSA and hemoglobin levels. The initial search strategy was developed for Ovid Medline by using a combination of database-specific subject headings and text words. The search strategy was then customized for each database. Searches were executed on March 31, 2020 and updated on February 23, 2022 in the following databases: Ovid MEDLINE; Ovid MEDLINE Epub Ahead of Print and In-Process & Other Non-Indexed Citations; Ovid Embase; Cochrane Database of Systematic Reviews; and Cochrane Central Register of Controlled Trials (CENTRAL). See Appendix A for search strategies. Results were limited to humans, adults, and English language. Conference and book materials were excluded where possible. The reference lists of retrieved publications were also searched and considered for inclusion.
2.4. Study selection
Two independent review authors (V.M. and E.C.) screened the titles and/or abstracts retrieved by the search. V.M. and E.C. then independently assessed the screened full text references for inclusion.
2.5. Data collection process
A standardized form was used to extract the following data from the included studies: study design, population characteristics (age, sex, apnea hypopnea index (AHI), minimum oxygen saturation (MinSpO2) during sleep), duration of CPAP treatment, hemoglobin and/or hematocrit levels, and timing of blood sample collection.
2.6. Risk of bias in individual studies
For studies reporting the prevalence of polycythemia in patients with OSA, the risk of bias was assessed based on the reliability with which other causes of polycythemia were excluded within the study population. In particular, smokers’ polycythemia (caused by carbon monoxide displacement of oxygen and diagnosed if carboxyhemoglobin levels are ≥ 5%) was considered excluded if current smokers were excluded or if carboxyhemoglobin levels were verified [Citation17]. Other causes of polycythemia were considered reliably excluded if the study exclusion criteria included: chronic lung disease, cyanotic heart disease, renal artery stenosis, renal cysts or tumours with ectopic EPO production.
For clinical trials of the effect of CPAP on hemoglobin and/or hematocrit levels, the risk of bias was assessed using the Cochrane Handbook for Systematic Reviews of Interventions criteria: random sequence generation; allocation concealment; blinding of participants and personnel; blinding of outcome assessment; incomplete outcome data; selective reporting and other sources of bias (in this case, smokers included in the study population) [Citation18]. Each criterion was graded as high, low or unclear risk of bias.
2.7. Summary measures and synthesis of results
In the analysis for objective (i), the summary measure reported was the mean prevalence of polycythemia across included studies, using a generalized linear mixed model. Subgroup analysis was performed to assess variation in the prevalence of polycythemia by OSA severity groups.
In the analyses for objective (ii), the summary measures of treatment effect were the mean difference in hemoglobin and the mean difference in hematocrit, comparing values after CPAP treatment to those before. The inverse variance weighting method was used, with the between-study variance estimated by REML. Both fixed-effect and random-effects pooled estimates were computed. Heterogeneity was quantified using the I2 statistic. Separate meta-regression analyses were used to estimate the relationship between the change in hemoglobin or hematocrit and the two predictor variables mean MinSpO2 and mean baseline AHI.
Two post-hoc analyses were performed. Subgroup analysis was performed to determine whether the timing of blood sample collection (morning versus not specified) was associated with the magnitude of change in hemoglobin or hematocrit. Meta-regression was performed to estimate the relationship between the change in hemoglobin or hematocrit and duration of CPAP treatment. All analyses were carried out in R 4.1.1 using the meta package [Citation19, Citation20].
3. Results
3.1. Study selection
The literature search provided 3,854 citations, of which 171 full text articles were assessed for eligibility (). A total of seven studies were included in the objective (i) meta-analysis [Citation13, Citation14, Citation21–25]. A total of 14 studies were included in the objective (ii) meta-analysis [Citation25–38].
3.2. Objective (i): prevalence of polycythemia in patients with OSA
3.2.1. Study characteristics
The seven studies in the meta-analysis included adult patients from centers in North America, Europe and Asia. The diagnostic criteria used for polycythemia differed between studies ().
Table 1. Summary of Included Studies Measuring the Prevalence of Secondary Polycythemia in Patients with OSA.
3.2.2. Risk of bias assessment
Out of the seven included studies, only two excluded smokers and four reliably excluded causes of polycythemia other than smoking ().
Table 2. Risk of Bias in Included Studies Measuring the Prevalence of Secondary Polycythemia in Patients with OSA.
3.2.3. Synthesis of results
3,654 patients were included in the seven studies. Overall, the summary estimate of the prevalence of polycythemia was 2% (95% CI 1–4%) (). There was considerable heterogeneity (I2 = 88%).
Figure 2. Overall Prevalence of Polycythemia in Patients with OSA and Subgroup Analysis Based on OSA Severity. OSA: obstructive sleep apnea.
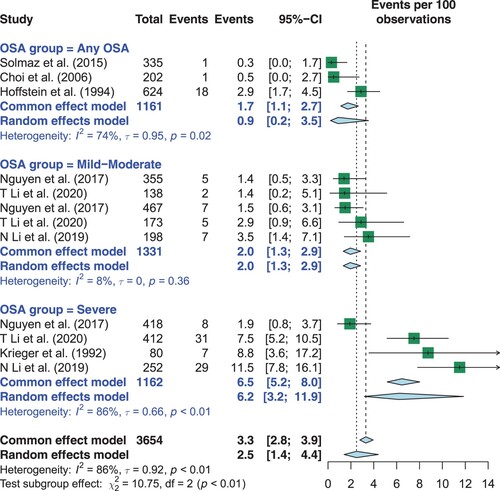
Subgroup analysis based on OSA severity (any, mild-moderate or severe) showed that the prevalence of polycythemia was greater in patients with severe OSA (p for interaction <0.01). Specifically, in patients with any OSA severity, the summary estimate of polycythemia prevalence was 0.9% (95% CI 0.2–3.5%). In patients with mild-moderate OSA, the summary estimate of polycythemia prevalence was 2% (95% CI 1.3–2.9%). In patients with severe OSA, the summary estimate of polycythemia prevalence was 6.2 % (95% CI 3.2–12.0%).
3.3. Objective (ii): the effect of CPAP on hemoglobin and/or hematocrit levels in patients with OSA
3.3.1. Study characteristics
All 14 studies were single-arm trials. We included 13 studies of patients without baseline polycythemia in the meta-analysis. The study by Zhang et al. included patients with baseline polycythemia and was not included in the meta-analysis based on clinical rationale that this patient population was physiologically different from that without baseline polycythemia [Citation38].
The meta-analysis included 509 participants, most of whom were male with a mean age above 40 years (). With the exception of the study by Lakadamyali et al., the included studies had patient populations with a predominantly severe degree of OSA. The duration of CPAP treatment ranged from 1 night to 1 year.
Table 3. Summary of Included Studies Evaluating the Effect of CPAP on Hemoglobin and/or Hematocrit Levels.
The study by Zhang et al. included 41 patients with OSA (22 moderate and 19 severe) and the duration of CPAP treatment was 1 month [Citation38].
3.3.2. Risk of bias assessment
Given that all 14 studies included were single-arm trials, they scored as ‘high risk’ for the criteria of random sequence generation; allocation concealment; blinding of participants and personnel; and blinding of outcome assessment. Regarding completeness of outcome data, studies scored as ‘high risk’ excluded patients who were non-compliant to CPAP, thereby failing the intention-to-treat analysis criterion. All 14 studies scored as ‘unclear risk’ for selective reporting as none had a protocol published prior to the undertaking of the study. Four of the 14 studies excluded active smokers and therefore scored as ‘low risk’ for this criterion ().
Table 4. Risk of Bias in Included Studies Evaluating the Effect of CPAP on Hemoglobin and/or Hematocrit Levels.
3.3.3. Synthesis of results
Ten studies included in the meta-analysis reported the effect of CPAP on hemoglobin levels, including 434 patients (). In the pooled analysis, CPAP treatment reduced hemoglobin by −3.76 g/L (95% CI −4.73 to −2.80 g/L) (A). There was no significant heterogeneity (I2 = 31%).
Ten studies included in the meta-analysis reported the effect of CPAP on hematocrit level, including 356 patients (). In the pooled analysis, CPAP treatment reduced hematocrit by −1.1% (95% CI −1.4 to −0.9%) (B). There was moderate heterogeneity (I2 = 61%). The Zhang et al. study, which included patients with baseline polycythemia, showed a much greater reduction in hematocrit: from a baseline of 59.1 ± 4.2% to 44.1 ± 4.9% when 6 am values were compared; and from a baseline of 50.4 ± 5.1% to 43.8 ± 5.7% when 4 pm values were compared [Citation38].
3.2.4. Additional analyses
Subgroup analysis based on timing of blood sample collection (morning vs. not specified) showed that the effect of CPAP was greater in studies in which blood samples were known to be collected in the morning for both hemoglobin (p = 0.02) and hematocrit levels (p<0.01) (). Specifically, the summary estimate of studies in which blood samples were collected in the morning showed that CPAP treatment reduced hemoglobin by −5.11 g/L (95% CI −6.55 to −3.67 g/L), and reduced hematocrit by −2.0 % (95% CI −2.0 to −1.0 %). In contrast, in the pooled analysis of studies in which blood sample collection timing was not specified, CPAP treatment reduced hemoglobin by −2.65 g/L (95% CI −3.96 to −1.34 g/L) and reduced hematocrit by −1.0 % (95% CI −1.0–0.0 %).
Figure 4. Subgroup Analysis of Effect of CPAP on Hemoglobin and/or Hematocrit Levels Based on Timing of Blood Sample Collection.
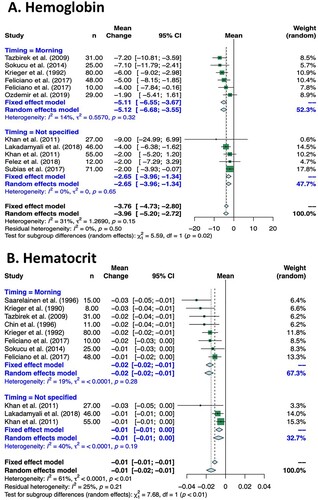
Meta-regression of change in hemoglobin or hematocrit vs. mean MinSpO2 and mean baseline AHI showed no correlation (A, B and A, B).
Figure 5. Meta-regression of Effect of CPAP on Hemoglobin Based on Minimum Nocturnal SpO2, Baseline AHI and CPAP Treatment Duration. Hb: hemoglobin; MinSpO2: minimum nocturnal oxygen saturation; AHI: apnea hypopnea index; CPAP: continuous positive airway pressure.
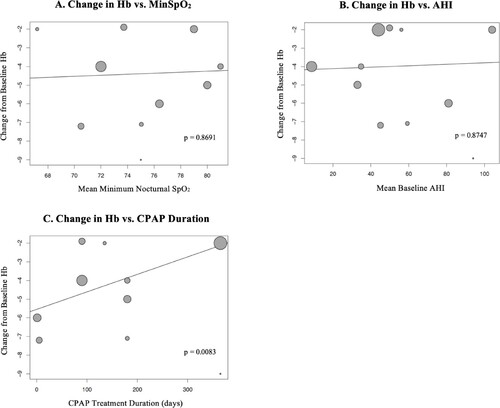
Figure 6. Meta-regression of Effect of CPAP on Hematocrit Based on Minimum Nocturnal SpO2, Baseline AHI and CPAP Treatment Duration. Hct: hematocrit; MinSpO2: minimum nocturnal oxygen saturation; AHI: apnea hypopnea index; CPAP: continuous positive airway pressure.
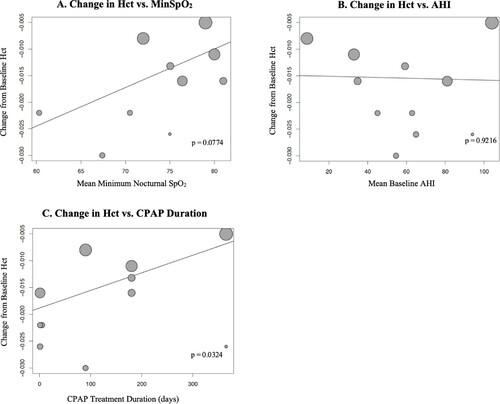
Meta-regression of change in hemoglobin or hematocrit vs. CPAP treatment duration showed that longer treatment duration was associated with less reduction in hemoglobin (0.94 less reduction per 100 days; p = 0.008) or hematocrit (0.33% less reduction per 100 days; p = 0.032) (C and C).
4. Discussion
This meta-analysis showed an overall prevalence of polycythemia in patients with OSA of 2%, and, in those with severe OSA, a higher prevalence of 6%. Secondly, this meta-analysis demonstrated average reductions in hemoglobin and hematocrit levels by CPAP therapy in OSA patients of 4 g/L and 1%, respectively.
A major strength of this meta-analysis, when evaluating the prevalence of polycythemia, was the inclusion of 3,654 patients from centers across three continents, thereby increasing the precision and the generalizability of the estimate. However, there were two important limitations to this meta-analysis. Firstly, the included studies used different diagnostic criteria for polycythemia. The current diagnostic criteria are based on the 2016 World Health Organization (WHO) Classification of Tumours of Haematopoietic and Lymphoid Tissues: hemoglobin > 165 g/L in men and > 160 g/L in women; or hematocrit > 49% in men and > 48% in women [Citation4]. These criteria are based on receiver operating characteristic curve analyses primarily developed to increase the sensitivity and specificity of the diagnosis of polycythemia vera; however, they are also used as the diagnostic cut-offs for other causes of polycythemia [Citation39]. Prior WHO criteria from 2008 had higher hemoglobin cut-off values (> 185 g/L in males and > 165 g/L in females) and did not include hematocrit criteria [Citation39]. Six of the seven included studies used criteria concordant with the 2008 guidelines. Only the study by N. Li et al. used criteria in keeping with the current guidelines and consequently reported the highest polycythemia prevalence: 4% in mild-to-moderate OSA and 12% in severe OSA [Citation14]. Thus, our pooled analysis of the available evidence likely underestimated the prevalence of polycythemia in patients with OSA. A second limitation of this meta-analysis was that only two of the seven studies reliably excluded smokers’ polycythemia and only four of the seven studies reliably excluded causes of polycythemia other than smoking, which may have led to an overestimation of the prevalence of polycythemia in patients with OSA.
This meta-analysis showed modest reductions of on average a 4g/L and 1% in hemoglobin and hematocrit levels, respectively, with CPAP treatment. In view of the known association between elevated hematocrit levels and increased total venous thromboembolism, cardiovascular disease and all-cause mortality [Citation16], these modest reductions may translate into improvements into patient-relevant clinical outcomes. It is important to note that the included OSA patients did not have baseline polycythemia. There is only one published study looking at the effect of CPAP in patients with baseline polycythemia. This study by Zhang et al. was not included in this meta-analysis based on clinical rationale that this patient population was physiologically different from that without baseline polycythemia. In fact, this study showed a much greater reduction in hematocrit after 1 month of CPAP treatment: from a baseline of 59.1 ± 4.2 % to 44.1 ± 4.9% [Citation38]. Therefore, it is possible that the effect of CPAP treatment is greater and occurs via a different physiological mechanism in OSA patients with higher baseline hemoglobin and hematocrit levels. Another potential explanation for the larger reduction in hematocrit in the Zhang et al. study would be regression to the mean.
In all included studies, the diagnosis of OSA was made based on level 1 polysomnography. However, as indicated in the footnotes of and , four studies did not use the recommended American Academy of Sleep Medicine (AASM) scoring criteria for hypopnea, and one of the four did not use the recommended AASM criteria for apnea [Citation40]. This is a limitation of this meta-analysis as it increases the clinical heterogeneity of the included studies.
The possible etiologies of polycythemia in untreated OSA are increased EPO levels (secondary polycythemia), hemoconcentration (relative polycythemia) or a combination of both mechanisms [Citation11, Citation41]. This meta-analysis, while not designed to determine the etiology of polycythemia in OSA, supports some previously postulated theories. Firstly, we did not demonstrate any correlation between the reductions in hemoglobin and hematocrit levels and baseline AHI or minimum oxygen saturation during sleep. In keeping with this observation is the point estimate (−4 g/L change in hemoglobin and −1% change in hematocrit) of the effect of CPAP on hemoglobin and hematocrit levels in the study Lakadamyali et al. having included a majority of patients with mild OSA, which was similar to the point estimate of this meta-analysis including a majority of patients with severe OSA [Citation27]. This is of interest as it suggests that the pathophysiological effect of OSA on hemoglobin and hematocrit levels may not be attributable to intermittent hypoxia.
Secondly, the finding of a greater reduction in hemoglobin and hematocrit levels with CPAP treatment when morning values were compared is of particular interest. There is previous evidence supporting this finding. In a study by Xilong et al., the diurnal variation in hematocrit levels was compared in patients with OSA vs. controls by collecting blood samples at 6 am and 4 pm [Citation42]. In patients with OSA, morning hematocrit values were statistically significantly higher by on average 7 % compared to afternoon values; whereas, there was no significant difference in the control group. It is postulated that these diurnal changes are related to the effect of untreated OSA on plasma volume, namely a reduction in plasma volume which occurs during sleep secondary to the release of atrial natriuretic peptide (ANP) [Citation43, Citation44]. The downstream effects of ANP include: a) increased renal sodium excretion thereby causing fluid to leave the plasma volume via urine, and b) increased vascular permeability thereby causing a fluid shift from the plasma volume into the extracellular space. Thus, it has been postulated that hemoglobin and hematocrit levels are higher in the morning in patients with untreated OSA due to hemoconcentration occurring during sleep; and, comparing morning pre-CPAP treatment to post-CPAP treatment values results in a higher magnitude of change observed.
Thirdly, this meta-analysis showed that one night of CPAP treatment resulted in the same if not a greater reduction in hemoglobin and hematocrit levels compared to longer treatment durations. This supports the hypothesis that the mechanism for the reduction in hemoglobin and hematocrit observed in these studies is the reversal of hemoconcentration during sleep, which can occur with as little as one night of treatment. Based on the results of this meta-analysis, the reduction in hemoglobin and hematocrit levels observed may not be caused by a reduction in EPO levels, as the lifespan of a red blood cell is approximately 115 days [Citation45]. This conclusion may not apply to patients with OSA and baseline polycythemia, for whom EPO may be playing a more significant role.
Our meta-analysis aimed to combine data across studies to estimate the effect of CPAP treatment on hemoglobin and hematocrit levels with more precision. The main limitation of this meta-analysis is the risk of bias related to non-randomized and uncontrolled study design: all 13 studies included were single-arm trials. Furthermore, our literature search was limited by publication status and availability within databases used.
In conclusion, our meta-analysis estimates the prevalence of polycythemia in patients with OSA as 2%. Future population studies applying the current diagnostic criteria and reliably excluding other causes of polycythemia are needed. However, the fact that our estimated prevalence is higher than the prevalence of erythrocytosis of any cause of 0.3% measured in a large Dutch cohort supports an association between OSA and polycythemia [Citation7]. Furthermore, our pooled analysis shows that CPAP treatment reduces hemoglobin and hematocrit levels in patient with OSA by on average 4g/L and 1%, respectively, and there is some evidence that the magnitude of this reduction may be greater in patients with baseline polycythemia. This evidence supports current guideline recommendations for routine evaluation for the presence of sleep-related breathing disorder as a possible causative factor in patients with polycythemia; however, based on this meta-analysis, the expected treatment benefit is modest. Future randomized controlled trials are needed to support this finding and determine if treatment has a meaningful cardiovascular benefit for patients.
Geolocation information
Unrelated disclosures
Dr. Ryan has received consultant fees from Jazz Pharmaceutical, unrelated to this work. Dr. Ryan has received research grant funding from the Canadian Institute of Health Research (CIHR) unrelated to this work. Dr Lyons has received research grant funding from the Ontario Lung Association. Dr. Tomlinson has received research grant funding from the CIHR unrelated to this work. Dr. Kuo has received research support from NHLBI/NIH: 1R33HL147845, Thalassemia Foundation Canada, Peter Munk Cardiac Centre, University of Toronto, Cincinnati Children's Hospital Medical Center, Canadian Hematology Society, and Pfizer. He has performed consultancy for Agios, Alexion, Apellis, Aruvant, Bluebirdbio, Celgene/BMS, Forma, Novartis, and Pfizer. He is Chair of Data Safety Monitoring Board for Bioverativ/Sanofi (ZFN gene editing trial for sickle cell disease NCT03653247). He is involved in research collaboration for Phoenicia Biosciences.
C.R. is the guarantor of the article, taking responsibility for the integrity of the work as a whole, from inception to published article. C.R., VM, OL conceptualized and designed the study. ACC, VM, EC participated in selection of studies, screening and eligibility. GT performed data analysis. C.R., V.M, O.L, K.K wrote the manuscript. All authors contributed to data analysis, drafting or revising the article, have agreed on the journal to which the article will be submitted, gave final approval of the version to be published, and agree to be accountable for all aspects of the work.
Supplemental Material
Download MS Word (89.1 KB)Disclosure statement
The authors report there are no competing interests to declare.
Notes
1 From: Page MJ, McKenzie JE, Bossuyt PM, Boutron I, Hoffmann TC, Mulrow CD, et al. The PRISMA 2020 statement: an updated guideline for reporting systematic reviews. BMJ 2021;372:n71. doi: 10.1136/bmj.n71
References
- Jordan AS, McSharry DG, Malhotra A. Adult obstructive sleep apnoea. Lancet. 2014;383(9918):736–747.
- Sullivan CE, Issa FG, Berthon-Jones M, et al. Reversal of obstructive sleep apnoea by continuous positive airway pressure applied through the nares. Lancet. 1981;1(8225):862–865.
- Jenkinson C, Davies RJ, Mullins R, et al. Comparison of therapeutic and subtherapeutic nasal continuous positive airway pressure for obstructive sleep apnoea: a randomised prospective parallel trial. Lancet. 1999;353(9170):2100–2105.
- Arber DA, Orazi A, Hasserjian R, et al. The 2016 revision to the world health organization classification of myeloid neoplasms and acute leukemia. Blood. 2016;127(20):2391–2405.
- Provan D, Weatherall D. Red cells II: acquired anaemias and polycythaemia. Lancet. 2000;355(9211):1260–1268.
- Mehta J, Wang H, Iqbal SU, et al. Epidemiology of myeloproliferative neoplasms in the United States. Leuk Lymphoma. 2014;55(3):595–600.
- Wouters H, Mulder R, van Zeventer IA, et al. Erythrocytosis in the general population: clinical characteristics and association with clonal hematopoiesis. Blood Adv. 2020;4(24):6353–6363.
- McMullin MF, Bareford D, Campbell P, et al. Guidelines for the diagnosis, investigation and management of polycythaemia/erythrocytosis. Br J Haematol. 2005;130(2):174–195.
- McMullin MF. Investigation and management of erythrocytosis. Curr Hematol Malig Rep. 2016;11(5):342–347.
- Mithoowani S, Laureano M, Crowther MA, et al. Investigation and management of erythrocytosis. CMAJ. 2020;192(32):E913–E9E8.
- Zhang XB, Zeng YM, Zeng HQ, et al. Erythropoietin levels in patients with sleep apnea: a meta-analysis. Eur Arch Oto-Rhino-Laryngol. 2017;274(6):2505–2512.
- Wu M, Zhou L, Zhu D, et al. Hematological indices as simple, inexpensive and practical severity markers of obstructive sleep apnea syndrome: a meta-analysis. J Thorac Dis. 2018;10(12):6509–6521.
- Solmaz S, Duksal F, Ganidagli S. Is obstructive sleep apnoea syndrome really one of the causes of secondary polycythaemia? Hematology. 2015;20(2):108–111.
- Li N, Li HP, Wang P, et al. Nocturnal mean oxygen saturation Is associated with secondary polycythemia in young adults with obstructive sleep apnea, especially in Men. Nature & Science of Sleep. 2019;11:377–386.
- Rha MS, Jeong Y, Kim J, et al. Is obstructive sleep apnea associated with erythrocytosis? A systematic review and meta-analysis. Laryngoscope Investigative Otolaryngology. 2022.
- Danesh J, Collins R, Peto R, et al. Haematocrit, viscosity, erythrocyte sedimentation rate: meta-analyses of prospective studies of coronary heart disease. Eur Heart J. 2000;21(7):515–520.
- Smith JR, Landaw SA. Smokers' polycythemia. N Engl J Med. 1978;298(1):6–10.
- Cochrane Handbook for Systematic Reviews of Interventions: Cochrane. (2019). Available from: www.training.cochrane.org/handbook.
- Balduzzi S, Rucker G, Schwarzer G. How to perform a meta-analysis with R: a practical tutorial. Evid Based Ment Health. 2019;22(4):153–160.
- R: A language and environment for statistical computing.: R Foundation for Statistical Computing. (2021). [Available from: https://www.R-project.org/.
- Li T, Covassin N, Tan L, et al. Sex-Specific associations between erythrocyte measures and obstructive sleep apnea. J Clin Sleep Med. 2020;24(24.
- Nguyen CD, Holty JC. Does untreated obstructive sleep apnea cause secondary erythrocytosis? Respir Med. 2017;130:27–34.
- Choi JB, Loredo JS, Norman D, et al. Does obstructive sleep apnea increase hematocrit? Sleep Breath. 2006;10(3):155–160.
- Hoffstein V, Herridge M, Mateika S, et al. Hematocrit levels in sleep apnea. Chest. 1994;106(3):787–791.
- Krieger J, Sforza E, Delanoe C, et al. Decrease in haematocrit with continuous positive airway pressure treatment in obstructive sleep apnoea patients. Eur Respir J. 1992;5(2):228–233.
- Ozdemir C, Sokucu S, Aydin S, et al. Response of blood parameters to CPAP treatment in patients with obstructive sleep apnea. Noropsikiyatri Arsivi. 2019;56(3):182–185.
- Lakadamyali H, Kivanc T, Yilmaz Avci A. Correlation of changes in the red blood cell distribution width with the response to continuous positive airway pressure in patients with obstructive sleep apnea. Sleep Biol Rhythms. 2019;17(1):141–148.
- Felez M, Grau N, Ruiz A, et al. Increased urinary erythropoietin excretion in severe sleep apnea-hipoapnea syndrome: The effect of CPAP. Arch Bronconeumol. 2018;54(5):255–259.
- Leon Subias E, Gomara de la Cal S, Marin Trigo JM. Red cell distribution width in obstructive sleep apnea. Arch Bronconeumol. 2017;53(3):114–119.
- Feliciano A, Vaz F, Torres VM, et al. Evening and morning peroxiredoxin-2 redox/oligomeric state changes in obstructive sleep apnea red blood cells: correlation with polysomnographic and metabolic parameters. Biochimica et Biophysica Acta - Molecular Basis of Disease. 2017;1863(2):621–629.
- Feliciano A, Linhas R, Marcoa R, et al. Hematological evaluation in males with obstructive sleep apnea before and after positive airway pressure. Rev Port Pneumol. 2017;23(2):71–78.
- Sokucu SN, Ozdemir C, Dalar L, et al. Complete blood count alterations after six months of continuous positive airway pressure treatment in patients with severe obstructive sleep apnea. J Clin Sleep Med. 2014;10(8):873–878.
- Khan AM, Ashizawa S, Hlebowicz V, et al. Anemia of aging and obstructive sleep apnea. Sleep Breath. 2011;15(1):29–34.
- Tazbirek M, Slowinska L, Skoczynski S, et al. Short-term continuous positive airway pressure therapy reverses the pathological influence of obstructive sleep apnea on blood rheology parameters. Clinical Hemorheology & Microcirculation. 2009;41(4):241–249.
- Saarelainen S, Hasan J, Siitonen S, et al. Effect of nasal CPAP treatment on plasma volume, aldosterone and 24-h blood pressure in obstructive sleep apnoea. J Sleep Res. 1996;5(3):181–185.
- Chin K, Ohi M, Kita H, et al. Effects of NCPAP therapy on fibrinogen levels in obstructive sleep apnea syndrome. American Journal of Respiratory & Critical Care Medicine. 1996;153(6 Pt 1):1972–1976.
- Krieger J, Sforza E, Barthelmebs M, et al. Overnight decrease in hematocrit after nasal CPAP treatment in patients with OSA. Chest. 1990;97(3):729–730.
- Zhang X, Yin K, Wang H, et al. Effect of continuous positive airway pressure treatment on elderly Chinese patients with obstructive sleep apnea in the prethrombotic state. Chin Med J. 2003;116(9):1426–1428.
- Misawa K, Yasuda H, Araki M, et al. The 2016 WHO diagnostic criteria for polycythemia vera renders an accurate diagnosis to a broader range of patients including masked polycythemia vera: comparison with the 2008 WHO diagnostic criteria. Am J Hematol. 2017;92(7):E128–EE30.
- Berry RBQS, Abreu AR, et al. for the American Academy of Sleep Medicine. The AASM manual for the scoring of sleep and associated events. rules, terminology and technical specifications. version 2.6 ed. Darien (IL): American Academy of Sleep Medicine; 2020.
- Eisensehr I, Noachtar S. Haematological aspects of obstructive sleep apnoea. Sleep Med Rev. 2001;5(3):207–221.
- Xilong Z, Kaisheng Y, Enzhi J, et al. Investigation on risk factor of vascular thrombotic diseases in patients with obstructive sleep apnea hypopnea syndrome. Vasc Dis Prev. 2006;3(1):15–17.
- Krieger J. Regulation of plasma volume during obstructive sleep apnoea. J Sleep Res. 1995;4(S1):107–111.
- Baruzzi A, Riva R, Cirignotta F, et al. Atrial natriuretic peptide and catecholamines in obstructive sleep apnea syndrome. Sleep. 1991;14(1):83–86.
- Franco RS. Measurement of red cell lifespan and aging. Transfus Med Hemother. 2012;39(5):302–307.