Abstract
The transformation of freshwater wetlands to pastures is a common practice in Mexico. This rapid loss of wetlands contrasts with the scarce information that exists about these ecosystems. To identify the environmental factors that control vegetation structure of a freshwater wetland invaded by the African grass Echinochloa pyramidalis, we characterized the vegetation (species composition, cover and aerial biomass), soil (moisture, redox potential, bulk density and topography) and water (water depth level, electric conductivity and pH) in two seasons of the year (dry and rainy). In addition, we analyzed the soil and water of three vegetation areas in the wetland, one dominated by E. pyramidalis, another by Sagittaria lancifolia and a third by Typha domingensis. The parameters associated with the hydrology of the wetland (water level, soil moisture, redox potential and bulk density) explained the plant species distribution. The invasive grass dominated in the relatively drier areas in the wetland while native species such as S. lancifolia, T. domingensis and Pontederia sagitatta dominated wetter sites. Introduction of E. pyramidalis has caused negative changes in the wetland, in particular a decrease of the diversity of plant species. In addition, we believe that the invader grass, as a C4 species, has more efficient use of water than the native plants, as well as a larger biomass, characteristics that can change the hydrological pattern of this wetland.
Introduction
The freshwater wetlands have ecological, economic, social and cultural importance, recognized globally (Weller Citation1994, Mitsch & Gosselink Citation2000). In Mexico, particularly in the coastal plain of the Gulf of Mexico, the wetland areas have historical importance because they supported a high agricultural and fishing productivity in pre-hispanic times (Siemens et al. Citation1988, Siemens Citation1998). However, in the last decades these coastal ecosystems have been disappearing or deteriorating due, among other things, to the increase of extensive cattle grazing on the wetlands (Melgarejo Vivanco Citation1980, Del Ángel Pérez Citation1993).
The loss of freshwater wetlands in Mexico contrasts with the scarce information that exists about these ecosystems. The pioneer studies of the 1970s (i.e. Lot Citation1971, Vázquez-Yanes Citation1971, Orozco & Lot Citation1976) had the main objective of analyzing the aquatic flora and the structural characteristics of the vegetation of these ecosystems. Nowadays, valuable qualitative data about aquatic vegetation exists (Diego Pérez et al. Citation1993, Lot et al. Citation1993, Rojas & Novelo Citation1995, Ocaña & Lot Citation1996) and there is already an inventory of hydrophytes for the country (Lot et al. Citation1999). None of these studies, however, includes quantitative data of the vegetation nor do they relate the distribution of the vegetation to important physiochemical parameters (i.e. water level, pH, electric conductivity, Eh). In wetlands, the floristic composition and distribution are associated with the physiochemical characteristics of soil and water. Particular hydrological conditions of each wetland are responsible for this physiochemical environment (Mitsch & Gosselink Citation2000).
In this study, we have analyzed the vegetation of a native coastal freshwater marsh, partially invaded by the African grass Echinochloa pyramidalis (Lam.) Hitchc. & A. Chase introduced by cattle ranchers for pasture. The general objective was to identify the environmental factors that have influenced the structure of vegetation: species composition, abundance, biomass and distribution. In addition, we have characterized the physiochemical environment in soil and water of three clearly distinguished areas in the wetland: an area dominated by E. pyramidalis, one dominated by cattail (Typha domingensis Pers.) and one dominated by arrowhead (Sagittaria lancifolia L. subsp. media (Michelin) Bogin).
Materials and methods
Study site
The wetland studied is a coastal freshwater marsh of approximately 3 ha located in the La Mancha Coastal Research Center (CICOLMA, Instituto de Ecología A. C.), in the central region of the state of Veracruz on the Gulf of Mexico, at 19°36’ N and 96°22'40” W (a,b). Novelo (Citation1978) described the floristic composition of this wetland as a pure association of Typha domingensis Pers., a species sometimes mixed with other native species such as Pontederia sagitatta C. Presl, Sagittaria lancifolia L. subsp. media (Michelin) Bogin, Hydrocotyle umbellata L., Hydrocotyle bonariensis Comm. ex Lam., Bacopa monnieri (L.) Wettst., Cyperus articulatus L., Crinum erubescens Aiton and Limnocharis flava (L.) Buchenau. After Novelo's work, the floristic composition in the wetland changed, mainly because of the introduction of the African grass Echinochloa pyramidalis (Lam.) Hitchc. & A. Chase, as well as the increased use of land as pasture. This change in land use coincided with a decrease in the extent of the native species communities. Although cattle grazing stopped at this site some 10 years ago, E. pyramidalis still dominates most of the wetland today. Three different plant communities can be distinguished in the area.
Sampling design and data collection
We divided the study area into four large (42×90 m) rectangular plots and one small (42×60 m) rectangular plot (c). This last plot was smaller because of topographic changes. The plots were adjoining and oriented east-west. We assigned a Roman numeral (I–V) to each plot. Within each plot we traced a random transect; in the large plots transects were 90 m long, while in the small plot the transect was 60 m long. Each transect was divided into three equal segments. We placed two square-meter quadrats randomly on the middle section of the segment and one square-meter quadrat on each extreme segment, making a total of 20 quadrats, marked with Arabic numerals (1–4, c). We considered 20 quadrats a sufficient number of samples because of the homogeneous conditions and the extent of the invaded area. The size of the sampling quadrats was determined by the herbaceous nature of plants (Barbour et al. Citation1999). A previous pilot study showed that the middle segment on the plots contains the native wetland. It is the richest community, thus we decided to increase the number of quadrats in this area. The pilot study also showed that the inclusion of more quadrats out of the native area only increased numbers but not new information because E. pyramidalis dominate this area.
In March (dry season), we marked and sampled the quadrats. In August (rainy season), we placed and sampled one square-meter quadrat adjacent to each quadrat marked in the dry season. For each sample, we collected biotic and abiotic data. The biotic data were species composition, percent cover by species (Kent & Coker Citation1992) and dry aerial biomass of each species by quadrat. To get the dry aerial biomass we cut the vegetation from each quadrat to soil level; then we separated this vegetation by species and oven-dried it for 120 h at 65°C.
The abiotic data measured were water level, soil moisture, electric conductivity, pH, soil redox potential (Eh), bulk density and topography. During the dry season, we measured the water level using an in situ water sampler as described in McKee et al. (Citation1988). The sampler was introduced slowly in the soil while it was aspired with a plastic syringe until the water table was reached. For the rainy season, we measured the water level with a ruler. We obtained the relative soil moisture by taking 15 cm depth soil samples in aluminum boxes. For each sample, we recorded the wet weight and dry weight after oven-drying for 48 h at 80°C. Relative soil moisture results by subtracting dry weight from wet weight, divided by dry weight and multiplied by 100. Electric conductivity and pH were measured from interstitial water samples at 15 cm below soil level, almost within the middle of the root zone. Eh was measured at 15 cm soil depth using three platinum electrodes, one calomel reference electrode (Corning 476340) and a digital pH/ORP Barnant meter. Platinum electrodes were calibrated in the laboratory with quinhydrone (Sigma Q-1001) in pH 4.0 buffer solutions (Bohn Citation1971). To calculate Eh,+244 mV was added to each mV reading. We used an average of the three redox values for data analysis. Bulk density (oven-dry weight by volume) was determined after drying soil samples of 198 cm3 at 105°C for 24 hours (Hausenbuiller Citation1972). We measured the topography with reference to the sea using a level hose (García Márquez Citation1984).
In addition, to analyze in more detail the physiochemical characteristics of interstitial water and soil, during the rainy season we sampled the three clearly distinguished vegetation areas in the wetland (Echinochloa area, Sagittaria area and Typha area). In each area, we selected randomly 10 sampling points where we measured Eh at the soil surface (1 cm) and at 15 cm depth. In addition, we collected samples of interstitial water and soil, both at 15 cm depth. The interstitial water was filtered through a 0.45 micron syringe filter and two aliquots were used to measure electric conductivity, pH, selected macronutrients (Ca, K, Mg, Na, P, S) and micronutrients (Al, B, Fe, Mn, Zn). Electric conductivity and pH were measured with the proper meters for small samples. The aliquot for macronutrients and micronutrients was acidified to less than pH 2 with nitric acid and then we determined the nutrient concentrations by inductively coupled argon plasma emission spectrometry (ICP) (Williams et al. Citation1986).
The collected soil samples were processed to get relative soil moisture and bulk density, extractable NH4-N, P, metals (Cu, Fe, Mn, Zn) and cations (Ca, K, Mg, Na). Soil extractions followed standard techniques (Byrnside & Sturgis Citation1958, Bremner & Keeney Citation1966, Baker & Amacher Citation1982). After the extraction of NH4-N, we filtered the samples through a 0.45 micron syringe filter and measured the concentrations by the Colorimetric, Automated Phenate Method (EPA Citation1979). The remaining elemental concentrations were measured by ICP (Williams et al. Citation1986).
Data analysis
The dry aerial biomass was compared for dry and rainy seasons for each species using paired t-tests (Zar Citation1984) carried out with SAS/STAT for Windows, version 8.0 (SAS Institute Citation1999).
For each season two data matrices were composed. The Biotic matrix (20 quadrats×25 species in dry season and 21 species in rainy season) included presence/absence data. The Abiotic matrix (20 quadrats×7 environmental factors) was based on the quantitative data of the factors considered. We transformed the data in the Biotic matrix by Beals smoothing (Beals Citation1984); this is a powerful transformation effective on heterogeneous data such as community data (McCune Citation1994). The similarity between quadrats in each season was measured using Czekanowski (Bray-Curtis) index (Faith et al. Citation1987), the robustness of which is proper for the aims of this study. The main variation trends in the structure of the wetland vegetation in each season were detected by nonmetric multidimensional scaling ordination (NMDS) using PC-ORD for Windows (McCune & Mefford Citation1999). We used NMDS because this does not assume an underlying nature of species distributions along compositional gradients. NMDS is the most robust form of ordination for detection of ecological patterns (Faith et al. Citation1987, Minchin Citation1987). For each ordination, we evaluated the relationships between environmental variables and the ordination patterns using joint plots with the PC-ORD method (McCune & Grace Citation2002). This procedure directs and correlates the variables with the ordination scores.
The environmental data (interstitial water and soil variables), which were measured to identify differences among the three vegetation areas in the wetland, were first collectively analyzed using Multivariate Analysis of Variance (MANOVA). This method considers the correlation among multiple variables, which separate one-way analysis of variance (ANOVA's) cannot do; therefore, it provides more powerful testing if there is correlation among the variables (Zar Citation1984). After we detected a significant multivariate community effect by the Wilk's λ test, individual univariate tests were performed on all abiotic data. The univariate model was a randomized ANOVA. We used the Fisher's protected LSD test to identify significant differences among communities (Zar Citation1984). The MANOVA was carried out using SAS/STAT for Windows, version 8.0 (SAS Institute Citation1999).
Results
Percent cover and aerial biomass
Twenty-five species were present in the dry season, while 21 species were present in the rainy season (). In the dry season only seven species had coverage of 50 percent or higher in at least one quadrat, while in the rainy season there were five species with coverage higher than 50 percent. The species’ that had coverage of 50 percent or higher in both seasons were the African grass E. pyramidalis, S. lancifolia, T. domingensis, the giant fern A. aureum and the vine, I. tiliacea (). For aerial biomass, some species showed an increase of biomass in the dry season; others showed a decrease (). The only species with significant differences between seasons were E. pyramidalis and S. lancifolia. Echinochloa pyramidalis produced more biomass in the rainy season than in the dry season, while S. lancifolia produced more biomass in the dry season than in the rainy season (). Data in both tables show a clear dominance of a few species as well as little vegetation change throughout the year.
Table I Species present in the freshwater marsh in La Mancha, Veracruz, Mexico. Twenty quadrats were sampled in each season, dry and rainy. For each species, the number of quadrats where it was present is noted. The number of quadrats where the species coverage was 50 percent or higher appears in parenthesis.
Table II Dry aerial biomass for the main species evaluated in dry and rainy seasons in the wetland. Means of 20 data points are shown±1 standard error. Significant differences in means are indicated with different letters (paired t-test, *P<0.05).
Ordination
NMDS ordination diagrams, with associated vectors, are presented in (dry season) and (rainy season). The abiotic factors with major correlations with the ordination solution were soil moisture (r=0.60 in dry season and 0.54 in rainy season), water table depth (r=0.58 in dry season and 0.34 in rainy season) and bulk density (r= − 0.50 in dry season and −0.55 in rainy season). The species found on the upper-right side of and show clear preference for the moister quadrats. Some of these species are characteristic of freshwater wetlands, such as P. sagitatta, S. lancifolia, Hymenocallis litoralis, Hydrocotyle umbellata, T. domingensis and A. aureum. In contrast, from the centre to the lower-left side in both diagrams, were those species that appeared in the drier quadrats, such as Dalbergia brownei, Calopogonium caeruleum, Vigna vexillata and Hyptis capitata. Echinochloa pyramidalis was on the lower-left side of the graph, but near the centre.
Figure 2. NMDS ordination of quadrats and wetland species in the dry season (stress=7.1% for two dimensions; final instability = 4×10−5 with 41 iterations). Ordination based on percent cover of species data. Abiotic data are represented by vectors. The angle and length of vectors indicates the direction and strength of the relationships between abiotic factors and ordination scores.
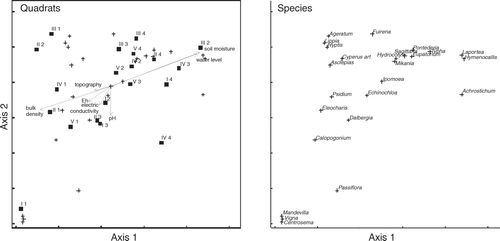
Figure 3. NMDS ordination of quadrats and wetland species in the rainy season (stress=5.7% for two dimensions; final instability = 7×10−5 with 44 iterations). Ordination based on percent cover of species data. Abiotic data are represented by vectors. The angle and length of vectors indicates the direction and strength of the relationships between abiotic factors and ordination scores.
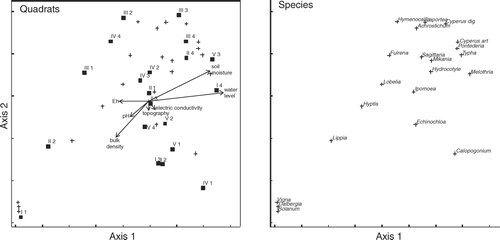
Environmental variation in the three communities of the wetland
The MANOVA of the 27 environmental variables showed a significant community effect (Wilk's λ = 1.5×10−6, F=30.1, d. f.=2, 54, P<0.05); therefore the environmental data as a whole differed significantly among communities. As a result, an ANOVA was conducted on each of the individual variables and the results are discussed below.
Eh, bulk density, soil moisture, electric conductivity and pH
Surface and 15 cm-depth Eh, bulk density and soil moisture varied significantly between the Echinochloa area and the Sagittaria and Typha areas (). Both Eh measures and bulk density were higher in the Echinochloa than in the Sagittaria and Typha areas. The opposite pattern was observed with soil moisture, which was higher in the areas with Sagittaria and Typha than in the Echinochloa area. Electric conductivity was higher in zones with Echinochloa and Typha. pH was not significantly different among communities ().
Table III Physiochemical characteristics of soil and water in the three vegetation areas in the wetland of La Mancha, Veracruz. Means of 10 replicates are shown±1 standard error. Significant differences in means are indicated with different letters (one-way ANOVA, * P<0.05; ** P<0.01; *** P<0.0001; n.s. non-significant). Samples were taken during the rainy season.
Nutrients
The nutrients that varied significantly among the three communities were interstitial Al, B, Mg, Mn, Na, P and extractable Fe, Mn, Zn, Mg (). Nutrients that only varied among the Echinochloa area and the Sagittaria and Typha areas were interstitial Al, B, Mg, Mn and extractable Mg. Interstitial Al, B, Mg and extractable Mg had higher values in the Echinochloa than in the Sagittaria and Typha areas; while interstitial Mn was higher in Sagittaria and Typha communities than in the Echinochloa area. Interstitial Na and extractable Fe and Mn were higher in the Typha zone than in the Sagittaria and Echinochloa zone. Extractable Zn was higher in the Sagittaria area than in areas with Echinochloa and Typha. Interstitial Ca was significantly higher in Typha communities than in the Sagittaria area; this element has intermediate values in the Echinochloa zone. Interstitial P was significantly higher in the Typha area than in the Echinochloa area; intermediate values for this element were found in the Sagittaria zone. Finally, the elements without differences among communities were interstitial Fe, K, S, Zn and extractable Cu, NH4, P, Ca, K, Na ().
Discussion
The invasions of tropical ecosystems by African grasses in the Americas are the result of increased cattle grazing in these regions (Parsons Citation1972). Invaded ecosystems tend to lose biodiversity and differ markedly from adjacent non-invaded areas in structure and function (Williams & Baruch Citation2000). In this study, we found the invasion by the African grass E. pyramidalis caused negative changes in the wetland. The most obvious change was the decrease in the cover of native plants and the generation of a dense, almost pure stand dominated by the invader. Despite the success of this grass in invading the wetland, there are still areas where this grass has not been able to settle. Native plants still dominate those areas. There are two types: one dominated by T. domingensis forming a monospecific stand; and a more diverse type dominated by S. lancifolia. The latter forms a single patch with the presence of other representative wetland species such as P. sagitatta, A. aureum, T. domingensis, H. litoralis, H. umbellata and the vines I. tiliacea and M. micrantha.
The likeness found in both ordinations of the vegetation quadrats suggests that the spatial variation in the structure of the vegetation changes little from season to season during the year. The major temporal changes in the structure of the vegetation are because of phenological changes of the species E. pyramidalis and S. lancifolia. The flowering and increased production of biomass in E. pyramidalis occurs in the rainy season; while increased biomass production in S. lancifolia takes place in the dry season. These differences in biomass production can be a result of the differences in photosynthetic pathways between both species; E. pyramidalis is a C4 species while S. lancifolia is a C3 species. The coexistence of species with different photosynthetic pathways is possible because they have a temporal separation in peak photosynthetic production. In general, the biomass production of C3 species is higher in the less illuminated and colder seasons, while the opposite occurs in C4 plants (Ramakrishnan & Vitousek Citation1989). In the study site March is less illuminated (mean daily hours light = 12.64), cooler (mean daily temperature = 22.6°C) and drier (mean daily precipitation = 0.84 mm) than August (13.17 hours, 26.3°C and 9.84 mm respectively). Despite this temporal variation, we expect little water stress in the wetland because the water level in extremely dry years is not deeper than 35 cm under the soil level (López Rosas et al. Citation2006).
The ordinations highlighted the presence of transitional zones in the wetland. The presence of integrated zones or ecotones where the principal vegetation type is in transition has long been recognized in ecology (Kent et al. Citation1997). In this study, the presence of transitional zones with a mixed floristic composition (and position in the ordination joint plots) reflects the transition between drier areas and areas that are more humid. This is a result of the presence of E. pyramidalis, already dominating in drier areas, but also advancing towards the native species wetland. The environmental factors that explained the spatial variation in the distribution of species in the wetland were the soil moisture, the water level and the bulk density. The vegetation quadrats dominated by E. pyramidalis had low moisture values, low flooding conditions and high bulk density values. On the other hand, the quadrats dominated by the native species of the wetland had high moisture, high water level and low bulk density. This spatial variation in environmental factors was higher during the dry season than in the rainy season. It suggests that native species are in areas with a more extended flood period than the areas invaded by E. pyramidalis. These results agree with the work of Howard-Williams and Walker (Citation1974) in Lake Chilwa, Malawi, Africa. They found the transition between the T. domingensis stands and the community dominated by grasses (including E. pyramidalis) was because of changes in hydrology; the water level was deeper in the T. domingensis marsh than in the floodplain grassland.
The noted pattern in the ordinations is coherent with the results of the MANOVA. The areas dominated by the native wetland species (Sagittaria and Typha areas) presented higher soil moisture than the area dominated by the invader grass; these differences in moisture are associated with the bulk density and the Eh. The areas invaded by E. pyramidalis had greater values of bulk density; therefore, those areas have more compact soils with greater percolation rates (Bradley & Morris Citation1990) than the soils of the areas dominated by native plants.
Soil Eh reflects the time that soil remains flooded or saturated and to characterize the intensity of reduction or oxidation in those soils (Patrick et al. Citation1996). The comparison of Eh values among the three vegetation areas supports the results of the ordination: the areas invaded by E. pyramidalis have shorter periods of flooding than the areas dominated by the native plants. This shorter flood period allows the soil in the Echinochloa area to be significantly more oxidized than the soil in the Sagittaria and Typha areas. During the rainy season, the 15 cm-depth soil in the Echinochloa area is moderately reduced; meanwhile, at the same depth, the soil in the Sagittaria and Typha areas is reduced (Patrick et al. Citation1996).
The topographical variation in the wetland does not explain the spatial variation of the hydrology. The correlation of those factors in both, dry and rainy ordinations were low ( and ). It is related to the variable water table in this wetland (Yetter Citation2004). The variation noted in the hydrology could be the result of the invasive process by E. pyramidalis. The grass invasions, particularly the invasions by African C4 grasses can change the hydrological conditions of the invaded ecosystems because they are species with more effective uptake of water than those of the invaded species (D'Antonio & Vitousek Citation1992). This is because the C4 grasses have higher transpiration at leaf level (Williams & Black Citation1994) and massive production of shallow or deep roots (Daubenmire Citation1972, Fisher et al. Citation1994). Echinochloa pyramidalis is important in the central floodplains, in the south and east of Africa, where it originated and where it grows in dense, pure stands (Vesey-FitzGerald Citation1963, Howard-Williams & Walker Citation1974, Denny Citation1993). It is a very productive, perennial, rhizomatous and stoloniferous species with tolerance to moderate drought and to a wide range in duration and depth of flooding (Howard-Williams & Walker Citation1974, Skerman & Riveros Citation1990), as well as to intensive grazing (John et al. Citation1993). Those characteristics were neither present nor dominant in the indigenous wetland species. Biological invasions have a greater impact on the system when the functional types of the invaded plants are different from the functional type of the invasive plant (Huston Citation1994). In the studied wetland, E. pyramidalis could have caused negative changes in the hydrology because the high biomass production of this species is related to an increase of water output by evapotranspiration (Mitsch & Gosselink Citation2000, Williams & Baruch Citation2000). This possible change in the hydrology could have caused the displacement of the native plants toward deeper areas in the wetland.
We find it necessary to conduct future studies that evaluate with greater emphasis the microtopographic differences among the Echinochloa area and the Sagittaria and Typha areas. The transition zones must be measured with special attention to differences because of the effect of sediment build-up, organic matter build-up (vertical accretion) or both. It is also necessary to conduct modeling studies to simulate the changes in the hydrology of the wetland considering the transpiration rates, the build-up of surface and under-ground biomass and the organic matter decomposition rates in the three vegetation areas.
We thank the valuable comments and suggestions provided by Dr J. A. López-Portillo, Dr F. Flores Verdugo, Dr A. Yáñez-Arancibia and Dr C. Siebe regarding this manuscript. We thank J. F. Rodríguez Ruíz, S. Garza Garza, D. Infante Mata and A. P. García Rodríguez for their support in fieldwork and S. Garza Garza and D. Infante Mata for their help in the laboratory. We thank Justine Gillham for linguistic corrections. We also thank the “La Mancha” Coastal Research Center (CICOLMA, Instituto de Ecología, A.C.) for the logistical support provided. This study was funded by a doctoral grant to H. López Rosas from Consejo Nacional de Ciencia y Tecnología (CONACYT 144658) and by funding from Instituto de Ecología, A.C. (Departamento de Ecología Vegetal 902-16), Secretaría de Medio Ambiente y Recursos Naturales (SEMARNAT-2002-C01-0190) and by NAWCA Program.
References
- Baker DE , Amacher MC . 1982 . Nickel, copper, zinc, and cadmium . In: Page AL , Miller RH , Keeney DR , editors . Methods of soil analysis, Part 2. Chemical and microbiological properties. Agronomy Monograph no. 9 . Madison (WI) : American Society of Agronomy . p 323 – 336 .
- Barbour , M , Burk , JH , Pitts , WD , Gulliam , FS and Schwartz , MW . 1999 . Terrestrial plant ecology , Menlo Park (CA) : Benjamin/Cummings .
- Beals , EW . 1984 . Bray-Curtis ordination: an effective strategy for analysis of multivariate ecological data . Adv Ecol Res , 14 : 1 – 55 .
- Bohn , HL . 1971 . Redox potential . Soil Sci , 112 : 39 – 45 .
- Bradley , PM and Morris , JT . 1990 . Physical characteristics of salt marsh sediments – ecological implications . Mar Ecol Prog Ser , 61 : 245 – 252 .
- Bremner , JM and Keeney , DR . 1966 . Determination and isotope-ratio analysis of different forms of nitrogen in soils. 3. Exchangeable ammonium, nitrate, and nitrite by extraction-distillation methods . Soil Sci Soc Am Proc , 30 : 577 – 582 .
- Byrnside , DS and Sturgis , MB . 1958 . Soil phosphorous and its fractions as related to response of sugar cane to fertilizer phosphorous , Baton Rouge (LA) : Louisiana State University and Agricultural and Mechanical College, Agriculture Experiment Station .
- D'Antonio , CM and Vitousek , PM . 1992 . Biological invasions by exotic grasses, the grass/fire cycle, and global change . Ann Rev Ecol Syst , 23 : 63 – 87 .
- Daubenmire , R . 1972 . Ecology of Hyparrhenia rufa (Nees) in derived savanna in north-western Costa Rica . J Appl Ecol , 13 : 11 – 23 .
- Del Ángel Pérez , AL . 1993 . “ La ganadería bovina entre ejidatarios: ¿sistema de producción o mecanismo de sobrevivencia? ” . In Desarrollo y medio ambiente en Veracruz , Edited by: Barrera , N and Rodríguez , H . 289 – 297 . México, DF : Fundación Friedrich Ebert .
- Denny , P . 1993 . “ Wetlands of Africa: introduction ” . In Wetlands of the world I: inventory, ecology and management , Edited by: Whigham , DF , Dykyjová , D and Hejný , S . 1 – 28 . Dordrecht, , the Netherlands : Kluwer Academic Publishers .
- Diego Pérez , N , Fonseca , RM , Pérez Lozada , L and Lorea Hernández , F . 1993 . Vegetación de las lagunas costeras y zonas inundables del Estado de Guerrero, México . Brenesia , 39–40 : 7 – 28 .
- EPA . 1979 . Methods for chemical analysis of water and wastes . EPA-600/4-79-020 . Cincinnati (OH) : U.S. Environmental Protection Agency .
- Faith , DP , Minchin , PR and Belbin , L . 1987 . Compositional dissimilarity as a robust measure of ecological distance . Vegetatio , 69 : 57 – 68 .
- Fisher , MJ , Rao , IM , Ayarza , MA , Lascano , CE , Sainz , JI , Thomas , RJ and Vera , RR . 1994 . Carbon storage by introduced deep-rooted grasses in the South American Savannas . Nature , 371 : 236 – 238 .
- García Márquez , F . 1984 . Topografía aplicada , México, DF : Editorial Concepto .
- Hausenbuiller , RL . 1972 . Soil science principles and practices , Dubuque (IA) : Wm. C. Brown Publishers .
- Howard-Williams , C and Walker , BH . 1974 . The vegetation of a tropical African lake: classification and ordination of the vegetation of Lake Chilwa (Malawi) . J Ecol , 62 : 831 – 854 .
- Huston , MA . 1994 . Biological diversity: the coexistence of species on changing landscapes , Cambridge : Cambridge University Press .
- John , EM , Lévêque , C and Newton , LE . 1993 . “ Western Africa ” . In Wetlands of the world I: inventory, ecology and management , Edited by: Whigham , DF , Dykyjová , D and Hejný , S . 47 – 78 . Dordrecht, , the Netherlands : Kluwer Academic Publishers .
- Kent , M and Coker , P . 1992 . Vegetation description and analysis. A practical approach , London : CRC Press .
- Kent , M , Gill , WJ , Weaver , RE and Armitage , RP . 1997 . Landscape and plant community boundaries in biogeography . Prog Phys Geog , 21 : 315 – 353 .
- López Rosas , H , Moreno-Casasola , P and Mendelssohn , IA . 2006 . Effects of experimental disturbances on a tropical freshwater marsh invaded by the African grass Echinochloa pyramidalis . Wetlands , 26 : in press
- Lot , A . 1971 . Estudios sobre fanerógamas marinas en las cercanías de Veracruz, Veracruz . An Inst Biol UNAM, ser Bot , 42 ( 1 ) : 2 – 48 .
- Lot , A , Novelo , A and Ramírez , P . 1993 . “ Diversity of Mexican aquatic vascular plant flora ” . In Biological diversity of Mexico: Origins and distribution , Edited by: Ramamoorthy , TP , Bye , R , Lot , A and Fa , J . 577 – 591 . New York : Oxford University Press .
- Lot A , Novelo A , Olvera M , Ramírez P. 1999 . Catálogo de angiospermas acuáticas de México: hidrófitas estrictas emergentes, sumergidas y flotantes . Serie Cuadernos del Instituto de Biología No. 33 . México, DF : Instituto de Biología, UNAM .
- McCune , B . 1994 . Improving community analysis with the Beals smoothing function . Ecoscience , 1 : 82 – 86 .
- McCune , B and Grace , JB . 2002 . Analysis of ecological communities , Gleneden Beach (OR) : MjM Software Design .
- McCune B , Mefford MJ. 1999 . PC-ORD for Windows. Multivariate analysis of ecological data . Version 4.01 . Gleneden Beach (OR) : MjM Software Design .
- McKee , KL , Mendelssohn , IA and Hester , MW . 1988 . A reexamination of pore water sulfide concentrations and redox potentials near the aerial roots of Rhizophora mangle and Avicennia germinans . Am J Bot , 75 : 1352 – 1359 .
- Melgarejo Vivanco , JL . 1980 . Historia de la ganadería en Veracruz , Xalapa, Mexico : Ediciones del Gobierno de Veracruz .
- Minchin , PR . 1987 . An evaluation of the relative robustness of techniques for ecological ordination . Vegetatio , 69 : 89 – 108 .
- Mitsch , WJ and Gosselink , JG . 2000 . Wetlands , 3rd ed. , New York : John Wiley & Sons .
- Novelo , RA . 1978 . La vegetación de la estación biológica El Morro de la Mancha, Veracruz . Biotica , 3 : 9 – 23 .
- Ocaña , D and Lot , A . 1996 . Estudio de la vegetación acuática vascular del sistema fluvio-lagunar-deltaico del Río Palizada, en Campeche, México . An Inst Biol UNAM, ser Bot , 67 ( 2 ) : 303 – 327 .
- Orozco , SA and Lot , A . 1976 . La vegetación de las zonas inundables del sureste de Veracruz . Biotica , 1 : 1 – 44 .
- Parsons , JJ . 1972 . Spread of African pasture grasses to the American tropics . J Range Manage , 25 : 12 – 17 .
- Patrick WH , Gambrell RP , Faulkner SP . 1996 . Redox measurements of soils . In: Sparks DL , editor . ‘Methods of soil analysis, Part 3. Chemical methods . SSSA Book Series no. 5’ . Madison (WI) : American Society of Agronomy . p 1255 – 1273 .
- Ramakrishnan , PS and Vitousek , PM . 1989 . “ Ecosystem-level processes and the consequences of biological invasions ” . In Biological invasions: a global perspective , Edited by: Drake , JA , Mooney , HA , di Castri , F , Groves , RH , Kruger , FJ , Rejmánek , M and Williamson , M . 281 – 300 . New York : John Wiley & Sons .
- Rojas , J and Novelo , A . 1995 . Flora y vegetación acuáticas del Lago de Cuitzeo, Michoacán, México . Acta Bot Mex , 31 : 1 – 17 .
- SAS Institute . 1999 . SAS/STAT® User's Guide , Version 8 . Cary (NC) : SAS Institute Inc.
- Siemens AH. 1998 . A favored place . San Juan river wetlands, Central Veracruz, A. D. 500 to the present . Austin (TX) : University of Texas Press .
- Siemens , AH , Hebda , RH , Navarrete Hernández , M , Piperno , DR , Stein , JK and Zolá Báez , MG . 1988 . Evidence for a cultivar and a chronology from patterned wetlands in Central Veracruz, Mexico . Science , 242 : 105 – 107 .
- Skerman , PJ and Riveros , F . 1990 . Tropical Grasses , Rome : FAO .
- Vázquez-Yanes , C . 1971 . La vegetación de la laguna de Mandinga, Veracruz . An Inst Biol UNAM, ser Bot , 42 ( 1 ) : 49 – 94 .
- Vesey-FitzGerald , DF . 1963 . Central African grasslands . J Ecol , 51 : 243 – 274 .
- Yetter JC. 2004 . Hydrology and geochemistry of freshwater wetlands on the Gulf coast of Veracruz, Mexico [dissertation] . Waterloo, Ontario : University of Waterloo . 168 p . Available from: University of Waterloo Library; CA2ON UW420 2004Y47 .
- Weller , MW . 1994 . Freshwater marshes , 3rd ed , Minneapolis (MN) : University of Minnesota Press .
- Williams , DG and Black , RA . 1994 . Response to drought for a native and introduced Hawaiian grass . Oecologia , 97 : 512 – 519 .
- Williams , DG and Baruch , Z . 2000 . African grass invasion in the Americas: ecosystem consequences and the role of ecophysiology . Biol Inv , 2 : 123 – 140 .
- Williams , TR , Van Doren , JB , Smith , BR , McElvany , SW and Zink , H . 1986 . ICP analysis of biological samples . Am Lab , 18 : 52 – 57 .
- Zar , JH . 1984 . Biostatistical analysis , Englewood Cliffs (NJ) : Prentice-Hall .