Abstract
Leaf carbonic anhydrase (CA) activity, net photosynthetic rate (PN), stomatal conductance (gS) and plant dry mass (DM) exhibited a dose-dependent response to cadmium (Cd) in wheat (Triticum aestivum L.) grown under low and sufficient zinc (Zn) levels at 30 d after seedling emergence in sand culture. Cadmium at 10 µM concentration enhanced CA, PN and DM at low Zn level but these remain unaltered at sufficient Zn. Stomatal conductance decreased with increasing CdCl2 concentration at both the Zn levels. Cd higher than 10 µM inhibited the characteristics irrespective of Zn level. The activity of superoxide dismutase (SOD) was not saturated the with highest Cd concentration (50µM) at both the Zn levels. However, the activities of ascorbate peroxidase (APX) and glutathione reductase (GR) increased up to 25 µM Cd and decreased at 50 µM Cd at both the Zn levels. It is suggested that CA activity was induced at low Cd (10 µM) under low Zn level increasing the photosynthesis. The synergies among the activities of antioxidative enzymes helped to maintain CA and thus photosynthesis and plant DM at low Cd concentration under low Zn level.
Introduction
Cadmium (Cd) is one of the toxic heavy metals present in the environment (Wagner Citation1993). Although Cd occurs naturally in low concentration in agricultural soils, its level has been steadily increasing due to the application of sewage sludge, city refuse and Cd-containing phosphatic fertilizers (Williams and David Citation1973). Cadmium is easily taken up by roots and translocated to different plant parts (Baker et al. Citation1994). There is general agreement that high Cd concentration retards metabolic activities and causes plant death (Sanita di Toppi and Gabbrielli Citation1999). High Cd inhibits chlorophyll synthesis, activity of PS I and PS II (Bharadwaj and Marcarenhas Citation1989; Sheoran et al. Citation1990; Somashekariah et al. Citation1992; Siedlecka and Baszynsky Citation1993) and photosynthetic process (Sandalio et al. Citation2001; Seregin and Ivanov Citation2001; Arduini et al. Citation2004; Wojcik and Tukiendorf Citation2005; Khan et al. Citation2006; Mobin and Khan Citation2007). Contrarily, low Cd concentration has been reported to stimulate growth (Gussarsson Citation1994; Arduini et al. Citation2004). However, the physiological basis for Cd stimulated growth at low concentration has not been completely understood. It was therefore, considered to highlight physiological basis for diverse effects of Cd depending on its concentration on plants. Carbonic anhydrase (CA; EC, 4.2.1.1) is a zinc metalloprotein catalyzing reversible inter-conversion of HCO3 – and CO2 (Xin Bin et al. Citation2001; Khan et al. Citation2004); represents 1–2% of total soluble protein in leaf (Okabe et al. Citation1984) and is related to photosynthesis in higher plants (Khan Citation1994; Henry Citation1996). Any visual effect on growth could be seen only if CA and, therefore, photosynthesis are affected by Cd. The study of CA in relation to Cd is also important as Cd is associated with zinc (Zn) as contaminant in the processed Zn ores (Adriano Citation2001), of Zn mines and smelters (Sterckman et al. Citation2000). As an essential trace element, Zn has many biological functions in plants. Interaction studies on Cd and Zn suggests antagonistic effect of Zn on Cd uptake by plants (Cakmak et al. Citation2000; Zhu et al. Citation2003; Zhao et al. Citation2005).
Cd-induced generation of reactive oxygen species (ROS) triggers activation of plant antioxidative enzyme system in protection mechanism. Among antioxidative enzymes, superoxide dismutase (SOD; EC, 1.15.1.1) constitutes the primary step of cellular defense. It dismutates superoxide radicals (O2 .−) to H2O2 and O2. Further, the accumulation of H2O2 is restricted through the action of ascorbate peroxidase (APX; EC, 1.1.11.1) by converting it to H2O. Finally glutathione reductase (GR; EC, 1.6.4.2) catalyzes the NADPH-dependent reduction of oxidized GSSG to the reduced GSH (Noctor et al. Citation2002). It is evident from the literature that Cd causes change in the activities of antioxidant enzymes in plants (Luo et al. Citation1998; Schickler and Caspi Citation1999; Hegedus et al. Citation2001; Schutzendubel et al. Citation2001; Olmos et al. Citation2003; Gratao et al. Citation2006; Khan et al. Citation2007; Mobin and Khan Citation2007).
The reported research was conducted to evaluate the effect of Cd concentrations on leaf CA of plants receiving low and sufficient Zn and associated changes in photosynthetic characteristics and activities of antioxidative enzymes in wheat.
Materials and methods
Experimental system
Wheat (Triticum aestivum L. cv. HD 2204) plants were raised from seeds in clay pots filled with acid-washed sand (Hewitt Citation1966). The pots were kept in a green house under natural day/night conditions (PAR > 990 µmol m−2s−1, temperature 22±3°C, relative humidity 60–68%). Three plants per pot were maintained, and two sets of pots One set received 200 ml of full-strength Hoagland nutrient solution with 0.5 µM Zn (low Zn) and another set was supplied with the similar amount of Hoagland nutrient solution with 2 µM Zn (sufficient Zn). To each set, Cd was given as 0, 10, 25 and 50 µM Cd. The sources of Zn and Cd were ZnSO4 and CdCl2, respectively. In addition to the nutrient solution, 250 ml of de-ionized water was added to each pot every day in the evening. The treatments were arranged in a completely randomized design. Each treatment was replicated five times.
At 30 days after seedling emergence, fully expanded uppermost leaves were used for the measurement of photosynthetic characteristics with the infrared gas analyzer. For comparisons of Cd effect on CA, photosynthesis and antioxidative enzymes under low and sufficient Zn, same leaf material was used for the study of these characteristics.
Cd and Zn content
The leaf samples were dried for 48 h at 80°C, weighed and ground to fine powder, and digested with concentrated HNO3-HClO4 (3:1, v:v). Cadmium and Zn concentration was determined by atomic absorption spectrophotometer (GBG, 932 plus, Australia).
CA activity
Activity of CA in leaf was estimated according to the method described by Makino et al. (Citation1992). Sampled leaves were homogenized in 10 ml buffer containing 50 mM HEPES-NaOH (pH 7.5), 10 mM DTT, 0.5 mM EDTA and 10% (v:v) glycerol. Triton X-100 was added to the homogenate to a final concentration of 0.1% (v:v). The homogenate was centrifuged (15,000 g for 10 min), and the supernatant was used for the determination of CA. Activity of CA was determined in Wilbur–Anderson unit following time-dependent reduction in pH from 8.25–7.45 at 0–3°C. The Unit [U] of enzyme activity was calculated according to the formula U = 10 (T − T0)/T0, where T and T0 represent the time required to change the pH from 8.25–7.45, with and without the extract of crude enzyme, respectively. The enzyme activity was represented as U per mg protein.
Photosynthesis and dry mass
Net photosynthetic rate (PN) and stomatal conductance (gs) were measured on fully expanded uppermost leaves using Li6200 portable photosynthesis system (LiCOR, Nebraska, USA) at light saturating intensity between 10:00 and 11:00 h (relative humidity, 65±3%; atmospheric CO2, 360±5 µmol mol−1 and temperature 22±1°C). For the measurement of dry mass, plants were dried in an oven until constant weight.
Extraction and assay of antioxidant enzymes
The overall procedures were carried out at 4°C unless otherwise mentioned. Leaf tissue was ground in chilled mortar using different specific buffers and pH values for each enzyme. Homogenate was squeezed through four layers of cheesecloth and centrifuged at 15,000 g in cooling centrifuge at 4°C for 15 min. Supernatant obtained was used for enzyme determinations.
Superoxide dismutase
The activity of superoxide dismutase was assayed by monitoring the inhibition of photochemical reduction of nitroblue tetrazolium (NBT), according to Giannopolitis and Ries (Citation1977). The extraction buffer consisted of 50 mM phosphate buffer (pH 7.8) containing 0.1% (w:v) bovine serum albumin (BSA), 0.1% (w:v) ascorbate and 0.05% (w:v) β-mercaptoethanol. The photoreduction of NBT (production of blue formazan) was measured at A560 and an inhibition curve was made against different volumes of the extract. One unit of SOD was defined as the amount of enzyme required to cause 50% inhibition of the reduction of NBT at A560.
Ascorbate peroxidase
The activity of ascorbate peroxidase was assayed by following the reduction in a reaction mixture at A290 (extinction coefficient 2.8 mM−1 cm−1) according to Nakano and Asada (Citation1981). For the determination of APX activity, leaf samples were extracted in buffer containing 50 mM potassium phosphate buffer (pH 7.0), 1 mM EDTA and 1% polyvinyl pyrrolidone (PVP) (w:v) with the addition of 1 mM ascorbic acid. The reaction was started by the addition of the enzyme extract. For non-enzymatic oxidation of ascorbate, correction was made by H2O2.
Glutathione reductase
The activity of glutathione reductase was determined following the method of Sgherri et al. (Citation1994) by measuring decrease in the absorbance at A340 (extinction coefficient 6.2 mM−1 cm−1). The leaf samples for estimation of GR were extracted in 100 mM potassium phosphate buffer (pH 7.0) containing 1 mM Na2-EDTA and 4% polyclar AT (w:v). The reaction was started with the addition of NADPH. This method does not require correction for GSSH-independent NADH oxidation.
Estimation of protein
The protein content in the samples was determined using bovine serum albumin (BSA, Sigma) as standard (Bradford Citation1976).
Statistical analysis
Data were analyzed statistically using analysis of variance (ANOVA) using SPSS (version 10.0; SPSS Inc., Chicago, IL, USA). The results are presented as treatment mean±SE. The treatment means were compared using Duncan's multiple range test. Different letters indicate significant difference at p≤0.05.
Results and discussion
Cadmium application increased Cd concentration in leaves. Cadmium accumulation at 0 and 10 µM Cd treatments was equal in low and sufficient Zn levels. At 25 and 50 µM Cd treatment, the Cd content in low Zn was higher than sufficient Zn (a). At all concentrations of Cd, the content of Zn in leaves was equal under low Zn level Zinc was accumulated equally at all concentrations of Cd under low and sufficient Zn levels (b). At low Zn level, application of 10 µM Cd resulted in lesser Cd accumulation than 25 and 50 µM Cd possibly due to the fact that Zn and Cd compete each other for the same plasma membrane transporter. Cakmak et al. (Citation2000) have also shown that Zn interferes with phloem-mediated Cd transport in durum wheat, by competing with Cd for binding sites of a common transporter protein on the plasma membrane of sieve tube cells. The studies of Oliver et al. (Citation1997) and Hart et al. (Citation2002) on wheat have also shown that Zn application decreased Cd uptake and accumulation.
Figure 1. Cadmium (a) and Zn content (b) in the leaf of wheat (Triticum aestivum) grown with 0, 10, 25 and 50 µM Cd under low (0.5 µM Zn) and sufficient zinc (2 µM Zn) at 30 days of seedling emergence. Treatment mean±standard error (SE) (n=3). Data followed by the same letter are not significantly different at p≤0.05 level as determined by Duncan's multiple range test.
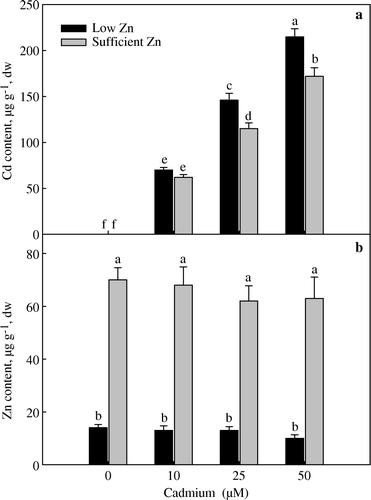
The activity of CA, net photosynthetic rate were increased at low Cd (10 µM Cd) and inhibited at higher Cd under low Zn (a, 2b). Increasing the Cd concentration decreased the CA activity. The higher activity of CA at 10 µM Cd under low Zn facilitated the production of CO2 from HCO3 − and maintained a constant supply of CO2 for Rubisco. This increased the rate of photosynthesis resulting in increased dry mass. The relationship between the activity of CA and photosynthesis has been demonstrated in higher plants (Khan Citation1994, Xin Bin et al. Citation2001). At sufficient Zn, CA activity at 10 µM Cd did not differ with control, but further increase in the Cd concentrations in the nutrient solution decreased CA activity. This effect of Cd at sufficient Zn was reflected in photosynthesis and dry mass. Stomatal conductance was significantly reduced under low and sufficient Zn at all Cd treatments (c). The variation in stomatal conductance was not in synchrony with the changes in photosynthesis, emphasizing the importance of CA in maintaining photosynthesis at different Cd concentrations. Carbonic anhydrase activity increased by 32.9%, PN by 28.8% and plant dry mass by 15.8% with 10 µM Cd in comparison to their respective controls under low Zn (a, 2b, 2d). Siedlecka and Krupa (Citation1999) also showed that low Cd concentration increased the effectiveness of CA leading to the activation of Rubisco. The possibility of Cd-dependent enzyme has been proposed by Lane and Morel (Citation2000) where Cd replaced Zn under Zn-limiting conditions. The other non-stomatal changes following Cd treatment have been found. Cadmium-induced damages of light harvesting complex (Krupa Citation1988), and photosystems I and II (Siedlecka and Baszynsky Citation1993, Siedlecka and Krupa Citation1996) have been reported earlier. Siedlecka et al. (Citation1997) reported that Cd decreased photosynthesis by inhibiting reaction steps of Calvin cycle but Di Cagno et al. (Citation2001) observed that reduction in photosynthesis was due to reduction in CO2 assimilation rate and Rubisco activity without the involvement of stomatal conductance.
Figure 2. Activity of carbonic anhydrase (a), net photosynthetic rate (b), stomatal conductance (c) and plant dry mass (d) of wheat (Triticum aestivum) grown with 0, 10, 25 and 50 µM Cd under low (0.5 µM Zn) and sufficient zinc (2 µM Zn) at 30 days of seedling emergence. Treatment mean±SE; (n=3). Data followed by the same letter are not significantly different at p≤0.05 level as determined by Duncan's multiple range test.
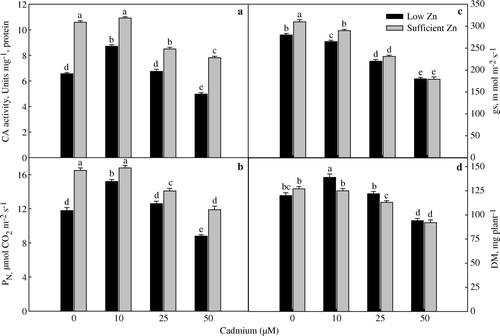
The activity of SOD increased with the increasing Cd concentration and was not saturated with the highest Cd concentration; whereas, the activities of APX and GR increased up to 25 µM Cd and decreased at 50 µM Cd (a–c). The activity of SOD was higher in control of sufficient Zn plant than low Zn but increased with the increase in Cd treatments. However, the increase of SOD activity was higher under sufficient Zn. In contrast, the increases in the activities APX and GR were higher in low Zn conditions than sufficient Zn. The enzymes APX and GR are physiologically and ecologically important in developing resistance to stress (Smirnoff Citation1995). An increase of 39.0% in APX and 42.1% in GR activity with 10 µM Cd under low Zn enabled the plants to protect chloroplast thylakoid against Cd stress (b, 3c). Thus, maintaining higher photosynthesis of 10 µM Cd-treated plants.
Figure 3. Activities of superoxide dismutase (a), ascorbate peroxidase (b) and glutathione reductase (c) of wheat (Triticum aestivum) grown with 0, 10, 25 and 50 µM Cd under low (0.5 µµM Zn) and sufficient zinc (2 µM Zn) at 30 days of seedling emergence. Treatment mean±SE; (n=3). Data followed by the same letter are not significantly different at p≤0.05 level as determined by Duncan's multiple range test.
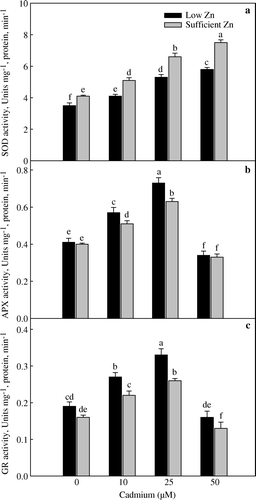
The increase in SOD activity with the increase in Cd concentration was in response to enhanced level of superoxide radicals converted to H2O2. The detoxification of H2O2 takes place via ascorbate-glutathione cycle, whereas H2O2 sends a systemic signal for the induction of APX (Karpinski et al. Citation1999), which was associated with the increase in GR activity. GR catalyzes the last rate-limiting step of ascorbate-glutathione cycle. This cycle maintains high ratio of GSH/GSSG which is required for the activation of several CO2 fixing enzymes (Noctor and Foyer Citation1998). The antioxidant enzymes response has been found variable depending upon plant species. Yadav and Yadav (Citation1995) and Chaoui et al. (Citation1997) observed increase in peroxidase activity in Triticum aestivum and Phaseolus vulgaris; whereas Shaw (Citation1995) and Gallego et al. (Citation1996) found decreased activity in superoxide dismutase in Phaseolus aureus and Helianthus annus.
It is concluded that the effect of Cd on CA was dose-dependent that stimulated the CA activity at low concentration and inhibited at high. A concentration of 10 µM Cd increased carbonic anhydrase activity, photosynthesis and dry mass at low Zn. Cadmium-induced changes in CA was concurrent with the rate of photosynthesis. The higher activities of antioxidative enzymes, APX and GR at 10 µM Cd protected the chloroplast from oxidative damage efficiently at low Zn. Higher Cd concentration inhibited CA and photosynthesis. Although the activities of antioxidative enzymes increased at higher Cd concentration but could not protect the CA and photosynthesis of sufficient Zn fed plants causing inhibitory effects.
Acknowledgements
Authors thank Prof. MNV Prasad for suggestions on the earlier version of the manuscript.
References
- Adriano , DC . 2001 . Trace elements in terrestrial environments: biogeochemistry, bioavailability, and risk of metals , 2nd ed , New York : Springer-Verlag, Inc .
- Arduini , I , Masoni , A , Mariotti , M and Ercoli , L . 2004 . Low cadmium application increase miscanthus growth and cadmium translocation . Environ Exp Bot , 52 : 89 – 100 .
- Baker , AJM , Reeves , RD and Hajar , ASM . 1994 . Heavy metal accumulation and tolerance in British populations of the metallophyte Thlaspi caerulescence J&C Presl (Brassicaceae) . New Phytol , 127 : 61 – 68 .
- Bharadwaj , R and Marcarenhas , C . 1989 . Cadmium induced inhibition of photosynthesis in vitro during development of chloroplast in Triticum aestivum L . Plant Physiol Biochem , 16 : 40 – 48 .
- Bradford , MM . 1976 . A rapid and sensitive method for the quantification of microgram quantities of protein utilizing the principle of protein dye binding . Anal Biochem , 72 : 248 – 254 .
- Cakmak , I , Welch , RM , Erenoglu , B , Romheld , V , Norvell , WA and Kochain , LV . 2000 . Influence of varied zinc supply on retranslocation of cadmium (109Cd) and rubidium (86Rb) applied on mature leaf of durum wheat seedlings . Plant Soil , 219 : 279 – 284 .
- Chaoui , A , Mazhoudi , S , Ghorbal , MH and El Ferjani , E . 1997 . Cadmium and zinc induction of lipid peroxidation and effects on antioxidant enzyme activities in bean (Phaseolus vulgaris L.) . Plant Sci , 127 : 139 – 147 .
- Di Cagno , R , Guidi , L , De Gara , L and Soldatini , GF . 2001 . Combined cadmium and ozone treatments affect photosynthesis and ascorbate-dependent defences in sunflower . New Phytol , 151 : 627 – 636 .
- Gallego , SM , Benavides , MP and Tomaro , ML . 1996 . Effect of heavy metal ion excess on sunflower leaves: evidence for involvement of oxidative stress . Plant Sci , 121 : 151 – 159 .
- Giannopolitis , CN and Ries , SK . 1977 . Superoxide dismutase occurance in higher plants . Plant Physiol , 59 : 304 – 314 .
- Gratao PL Gomes-Junior RA Delite FS Lea PJ Azevedo RA. 2006 . Antioxidant stress responses of plants to cadmium . In : Khan NA , Samiullah Cadmium toxicity and tolerance in plants . New Delhi : Narosa Publishing House . pp 1 – 36 .
- Gussarsson , M . 1994 . Cadmium-induced alterations in nutrient composition and growth of Betula pendula seedlings: the significance of fine roots as a primary target for cadmium toxicity . J Plant Nutr , 17 : 2151 – 2163 .
- Hart , JJ , Welch , RM , Norvell , WA and Kochain , LV . 2002 . Transport interactions between cadmium and zinc in roots of bread and durum wheat seedlings . Physiol Plant , 116 : 73 – 78 .
- Hegedus , A , Erdei , S and Horvath , G . 2001 . Comparative studies of H2O2 detoxifying enzymes in green and greening barley seedlings under cadmium stress . Plant Sci , 160 : 1085 – 1093 .
- Henry , RP . 1996 . Multiple roles of carbonic anhydrase in cellular transport and metabolism . Annu Rev Physiol , 58 : 523 – 538 .
- Hewitt EJ. 1966 . Sand and water culture methods used in the study of plant nutrition . Commonwealth Agricultural Bureau , UK . pp 56 – 195 .
- Karpinski , S , Reynolds , H , Karpinska , B , Wingsle , G , Creissen , G and Mullineaux , P . 1999 . Systemic signaling and acclimation in response to excess excitation energy in Arabidopsis . Science , 284 : 654 – 657 .
- Khan , NA . 1994 . Variation in carbonic anhydrase activity and its relationship with dry mass of mustard . Photosynthetica , 30 : 317 – 320 .
- Khan NA Javid S Samiullah . 2004 . Physiological role of carbonic anhydrase in CO2 fixation and carbon partitioning . Physiol Mol Biol Plants 10 : 153 – 166 .
- Khan , NA , Ahmad , I , Singh , S and Nazar , R . 2006 . Variation in growth, photosynthesis and yield of five wheat cultivars exposed to cadmium stress . World J Agric Sci , 2 : 223 – 226 .
- Khan NA , Samiullah , Singh S , Nazar R. 2007 . Activities of antioxidative enzymes, sulphur assimilation, photosynthetic activity and growth of wheat (Triticum aestivum) cultivars differing in yield potential under cadmium stress . J Agron Crop Sci (in press). DOI: 10.1111/j.1439-037X.2007.00272 .
- Krupa , Z . 1988 . Cadmium-induced changes in the composition and structure of the light-harvesting complex II in radish cotyledons . Physiol Plant , 73 : 518 – 524 .
- Lane , TW and Morel , FMM . 2000 . A biological function for cadmium in marine diatoms . Proc Natl Acad Sci USA , 97 : 4627 – 4631 .
- Luo , LX , Sun , TH and Jin , YH . 1998 . Effects of cadmium on lipid peroxidation in wheat leaves . Chinese Environ Sci , 18 : 813 – 820 .
- Makino , A , Sakashita , H , Hideina , J , Mae , T , Ojima , K and Osmond , B . 1992 . Distinctive responses of ribulose-1, 5-bi-phosphate carboxylase and carbonic anhydrase in wheat leaves to nitrogen nutrition and their possible relationship to CO2-teransfer resistance . Plant Physiol , 100 : 1737 – 1743 .
- Mobin , M and Khan , NA . 2007 . Photosynthetic activity, pigment composition and antioxidative response of two mustard (Brassica juncea) cultivars differing in photosynthetic capacity subjected to cadmium stress . J Plant Physiol , 164 : 601 – 610 .
- Nakano , Y and Asada , K . 1981 . Hydrogen peroxide is scavenged by ascorbate-specific peroxidase in spinach chloroplast . Plant Cell Physiol , 22 : 860 – 867 .
- Noctor , G and Foyer , CH . 1998 . Ascorbate and Glutathione: Keeping active oxygen under control . Annu Rev Plant Physiol Plant Mol Biol , 49 : 249 – 279 .
- Noctor , G , Gomez , L , Vanacker , H and Foyer , CH . 2002 . Interactions between biosynthesis, compartmentation and transport in the control of glutathione homeostasis and signalling . J Exp Bot , 53 : 1283 – 1304 .
- Okabe , K , Yang , S , Tsuzuki , M and Miyachi , S . 1984 . Carbonic anhydrase: Its content in spinach leaves and its taxonomic diversity studied with anti-spinach leaf carbonic anhydrase antibody . Plant Sci Lett , 33 : 145 – 153 .
- Oliver , DP , Wilhelm , NS , McFarlane , JD , Tiller , KG and Cozens , GD . 1997 . Effect of soil and foliar application of Zn on cadmium concentration in wheat grain . Aust J Exp Agricult , 37 : 677 – 681 .
- Olmos , E , Martinez-Solano , JR , Piqueras , A and Hellin , E . 2003 . Early steps in the oxidative burst induced by cadmium in cultured tobacco cells (BY-2 line) . J Exp Bot , 54 : 291 – 301 .
- Sandalio , LM , Dalurzo , HC , Gomez , M , Romero-Puertas , MC and Del Rio , LA . 2001 . Cadmium-induced changes in the growth and oxidative metabolism of pea plants . J Exp Bot , 52 : 2115 – 2126 .
- Sanita di Toppi , L and Gabbrielli , R . 1999 . Response to cadmium in higher plants . Environ Exp Bot , 41 : 105 – 130 .
- Schickler , H and Caspi , H . 1999 . Response of antioxidant enzymes to nickel and cadmium stress in hyperaccumulator plants of the genus Alyssum . Physiol Plant , 105 : 39 – 44 .
- Schutzendubel , A , Schwanz , P , Teichmann , T , Gross , K , Langenfeld-Heyser , R , Godbold , DL and Polle , A . 2001 . Cadmium-induced changes in antioxidant systems, hydrogen peroxide content, and differentiation in Scots pine roots . Plant Physiol , 127 : 887 – 898 .
- Seregin , IV and Ivanov , VB . 2001 . Physiological aspects of cadmium and lead toxic effects on higher plants . Russ J Plant Physiol , 48 : 606 – 630 .
- Sgherri , CLM , Loggini , B , Pulinga , S and Navari-Izzo , F . 1994 . Antioxidant defense system in Sporobolus stapfianus: Changes in response to dessication and rehydration . Phytochemistry , 33 : 561 – 565 .
- Shaw , BP . 1995 . Effects of mercury and cadmium on the activities of antioxidative enzymes in the seedlings of Phaseolus aureus . Biol Plant , 37 : 587 – 596 .
- Sheoran , IS , Singal , HR and Singh , R . 1990 . Effect of cadmium and nickel on photosynthesis and enzymes of the photosynthetic carbon reduction cycle in pigeon pea (Cajanus cajan L.) . Photosynth Res , 23 : 345 – 351 .
- Siedlecka , A and Baszynsky , T . 1993 . Inhibition of electron flow around photosystem I in chloroplasts of cadmium-treated maize plants is due to cadmium-induced iron deficiency . Physiol Plant , 87 : 199 – 202 .
- Siedlecka , A and Krupa , Z . 1999 . Cd/Fe interaction in plants – its consequences for the photosynthetic apparatus . Photosynthetica , 36 : 321 – 331 .
- Siedlecka , A and Krupa , Z . 1996 . Interaction between cadmium and iron and its effects on photosynthetic capacity of primary leaves of Phaseolus vulgaris . Plant Physiol Biochem , 34 : 833 – 841 .
- Siedlecka , A , Krupa , Z , Samuelsson , G , Oquist , G and Gardetrom , P . 1997 . Primary carbon metabolism in Phaseolus vulgaris plant under Cd/Fe interaction . Plant Physiol Biochem , 35 : 951 – 957 .
- Smirnoff , N . 1995 . “ Antioxidant systems and plant response to the environment ” . In Environment and plant metabolism: Flexibility and acclimation , Edited by: Smirnoff , N . 217 – 243 . Oxford : BIOS Scientific Press .
- Somashekariah , BV , Padmaja , K and Prasad , ARK . 1992 . Phytotoxicity of cadmium ions on germinating seedlings of mung bean (Phaseolus vulgaris): involvement of lipid peroxides in chlorophyll degradation . Physiol Plant , 85 : 85 – 89 .
- Sterckman , T , Douay , F , Proix , N and Fourrier , H . 2000 . Vertical distribution of Cd, Pb, and Zn in soils near smelters in the north of France . Environ Pollut , 107 : 377 – 389 .
- Yadav , VK and Yadav , N . 1995 . Influence of cadmium on germination, seedling growth and biochemical traits of wheat . Plant Physiol Biochem , 22 : 74 – 77 .
- Wagner , GJ . 1993 . Accumulation of cadmium in crop plants and its consequences to human health . Adv Agron , 51 : 173 – 212 .
- Williams , CH and David , DJ . 1973 . The effect of superphosphate on the cadmium content of soils and plants . Aust J Soil Res , 11 : 43 – 56 .
- Wojcik , M and Tukiendorf , A . 2005 . Cadmium uptake, localization and detoxification in Zea mays . Biol Plant , 49 : 237 – 245 .
- Xin Bin , D , Rongxian , Z , Wei , L , Xiaming , X and Schuqing , C . 2001 . Effects of carbonic anhydrase in wheat leaf on photosynthetic function under low CO2 concentration . Sci Agric Sinica , 34 : 97 – 100 .
- Zhao , Z-Q , Zhu , Y-G , Kneer , R and Smith , SE . 2005 . Effect of zinc on cadmium toxicity-induced oxidative stress in winter wheat seedlings . J Plant Nutr , 28 : 1947 – 1959 .
- Zhu , YG , Zhao , ZQ , Li , HY , Smith , SE and Smith , FA . 2003 . Effect of zinc-cadmium interactions on the uptake of zinc and cadmium by winter wheat (Triticum aestivum) grown in pot culture . Bull Environ Contam Toxicol , 71 : 1289 – 1296 .