Abstract
Serratia marcescens (TRS-1), either as aqueous suspensions or in bioformulations of sawdust, rice husk and tea waste, promoted growth in tea seedlings as evidenced by increase in height, emergence of new leaves and branches, as well as increase in leaf biomass. Survival of S. marcescens in soil after application was determined by ELISA and Dot-Blot using PAb raised against the bacterium. S. marcescens solubilized phosphate in vitro and in vivo. Following application of the bacterium, soil P content decreased, root and leaf phosphate increased, and soil phosphatase activities were enhanced. The bacterium was antagonistic to a number of fungal pathogens in vitro. It also reduced brown root rot of tea caused by Fomes lamaoensis. Significant increase in phenolics, as well as peroxidase, chitinase, β-1,3-glucanase and phenylalanine ammonia-lyase, were observed in tea plants on application of S. marcescens alone or followed by F. lamaoensis.
Introduction
India is one of the major producers of tea in the world. Tea is produced from the leaves of Camellia sinensis (L.) O. Kuntze. It is a perennial and survives for more than 100 years. Over the years, in tea plantations, insecticides, chemical fertilizers and fungicides have been used indiscriminately to check crop losses. But such indiscriminate and excessive use of chemicals and cropping practices have brought about significant undesirable changes in soil microbial activity, and have also created the problem of pesticide residue in the leaves. Since the leaves are used as beverage, the problem of pesticide residue is very important, and hence, there is an urgent need to look for environmentally friendly, natural plant protectors.
Plant growth promoting rhizobacteria (PGPRs) are a common group of bacteria that can actively colonize plant roots and increase plant growth (Kloepper and Schroth Citation1978). These PGPRs can prevent the deleterious effects of phytopathogenic organisms from the environment. The mechanisms by which PGPRs can influence plant growth may differ from species to species as well as from strain to strain. Growth promotion mechanisms may be direct, i.e. production of growth hormones, phosphate solubilization, nitrogen fixation or indirect, such as suppression of deleterious microorganisms by siderophore production or secretion of antifungal metabolites (Kloepper Citation1993). However, in field conditions, the above traits may not be sufficient to account for the observed growth promotion. The biochemical or physiological changes induced in the host that are activated by the PGPRs also lead to plant growth promotion and develop resistance capacity in the host against pathogens. Research over the past years has demonstrated that induced systemic resistance (ISR) can be a potential mechanism by which PGPR demonstrate biological disease control (Kloepper et al. Citation1996). ISR is dependent on colonization of the root system by sufficient numbers of PGPRs. Previous studies have shown that Serratia proteamaculans 1-102 promotes soybean-bradyrhizobia nodulation and growth, but the mechanism is unknown (Bai et al. Citation2002). Some biocontrol PGPB strains protect plants by activating gene encoding defense enzymes – peroxidase, chitinase, phenylalanine ammonia-lyase, β-1,3-glucanase and others, involved in synthesis of phytoalexin (M'Piga et al. Citation1997).
Phosphorus is an essential nutrient for plants and an important component in cell metabolism. Its assimilation, storage and metabolism are of major importance to plant growth and development (Duff et al. Citation1994). Some common extracellular enzymes in soil are acid and alkaline phosphatases, produced by many organisms, which remove the phosphate molecule from organic compounds such as phospholipids and nucleic acids. Once the phosphate is cleaved it becomes soluble and can be taken up by the cell. This is an important activity because phosphate is often the limiting nutrient for microbial and plant growth in soil. Acid phosphatases catalyse non-specific hydrolysis of Pi from phosphate monoesters in pH ranges from 4–6 and help in the supply of phosphate in plants (Duff et al. Citation1994; Tabaldi et al. Citation2007). Similarly alkaline phosphatases have a role in maintenance of cellular metabolism (Orhanovic and Pavela-Vrancic Citation2000).
One of the common means of application of bacterial inoculants to soil is in the form of bioformulations. The efficacy of bacterial inoculants would largely depend on the type of formulation and delivery technology (Lumsden et al. Citation1995). Experimental formulations of Bacillus spp. that have effectively reduced plant diseases have included peat and chitin (Manjula and Podile Citation2001; Sid Ahmed et al. Citation2003); ca-alginate, alginate manucol (Schmidt et al. Citation2001).
Brown root rot of tea, caused by Fomes lamaoensis (Murr.)Sacc. and Trott. is one of the major root diseases of tea in all tea growing region. Control is difficult without the use of excessive chemicals in soil. The present study was undertaken with the objective of evaluating S. marcescens-TRS1, originally isolated from tea rhizosphere, as a growth promoter and biocontrol agent and determining the mechanism of its action. S. marcescens has been used both as actively growing cells and as formulation.
Materials and methods
Test organisms
Serratia marcescens TRS-1 was isolated from the rhizosphere soil of tea bushes from Hansqua Tea Estate, West Bengal, India, and preliminarily identified on the basis of morphological, microscopic and biochemical tests. The identity of the bacterium was confirmed from the Plant Diagnostic and Identification Services, UK. The bacterium was maintained on Nutrient Agar (Himedia) slants. Fomes lamaoensis and other test fungi were obtained from the culture collection of Immuno-Phytopathology Laboratory, Department of Botany, University of North Bengal, and were maintained by regular sub-culturings in PDA.
Plant material
Eighteen-month old seedlings of five varieties of tea: TV-18, TV-23, TV-25, TV-26 and T-17, were selected for experimental purposes. The selected tea seedlings were maintained in 12-inch earthenware pots and also in the field in randomized block design. Tea seedlings were watered regularly for proper maintenance.
Inocula preparation and application
Bacterium
To prepare the inoculum, initially S. marcescens was cultured in Nutrient Broth medium (Himedia, M002-100G; ingredients: peptic digest of animal tissue, 5.00 g/l; sodium chloride, 5.00 g/l; beef extract, 1.50 g/l; yeast extract, 1.50 g/l; final pH 25°C −7.4±0.2) and was allowed to grow properly, with shaking at 37°C at 120 rpm for 48 h. At the end of the log phase, bacterial culture was centrifuged at 10,000 rpm for 15 min and the supernatant was discarded, selecting the bacterial pellet. Pellet was scraped into sterile distilled water. The aqueous suspensions were diluted as necessary to maintain the bacterial concentration at 108 cfu/ml. The aqueous suspension was then applied as a soil drench, at 100 ml/plant to the rhizosphere of tea seedlings both in field and potted conditions one month after transplantation. Application was performed at an interval of one month and three applications were carried out. The bacterial suspension was applied once more as a soil drench prior to pruning.
Fungus
Culture of F. lamaoensis was grown in sand-maize meal medium (maize meal: sand: water – 1:9:1.5 w:w:v) in autoclavable plastic bags (sterilized at 20 lb pressure for 20 min) for a period of three weeks at 28°C until the mycelia completely covered the substrate.
Preparation of bioformulation of S. marcescens
Three bioformulations were prepared using sawdust, rice husk and tea waste. For the preparation of bioformulations, 2.5 g of carboxy methyl cellulose sodium salt (Himedia) was added to 250 g of sawdust or rice husk, pH was adjusted to 7 by adding calcium carbonate and sterilized twice for 30 min. 100 ml of aqueous bacterial suspension (at a concentration of 1×1010 cfu/ml) was added to the mixture under sterile conditions. The mixture was dried under shade to reduce the moisture to less than 20%. Formulations were packed in polythene bags, sealed and stored at room temperature. In preparation of tea waste bioformulation, the procedure was similar but tea waste was initially soaked overnight in distilled water to remove the phenolic components. Survivability of S. marcescens was checked at a regular interval of one month for a period of nine months using direct plating method in nutrient agar medium. The data was expressed in log form for analysis.
Growth of tea seedlings
Growth promotion was studied in terms of increase in height, number of leaves, number of shoots and leaf dry mass in the experimental field as well as in potted plants. Plants were grown under natural conditions of light and temperature (30±2°C). Observations were recorded after 1, 2 and 6 months of application. In each plot, 10 replicates were taken for each treatment and the average of the 10 replicate plants were analyzed. Leaf number and number of branches were also determined visually. Leaves were dried and the dry biomass of all leaves above 18 inches was determined after six months of 1st bacterial application in the field.
Determination of sustainability of S. marcescens in rhizosphere
In order to determine whether the bacteria can survive in the rhizosphere following application, immunological techniques based on polyclonal antibody raised against the bacterium were used. For preparation of antigens from S. marcescens, the bacterium was first grown in nutrient broth and after 48 h the actively growing cells in log phase were harvested by centrifugation at 21,000 g, and the cells were suspended in 0.05 M sodium phosphate buffer. This suspension was then sonicated for 5 min, with 1 min treatments being separated by 1 min cooling periods (Mazarei et al. Citation1992). The sonicated cells were used as immunogens and polyclonal antibody (PAb) was raised in New Zealand white male rabbits against the prepared immunogen as described by Mazarei and Kerr (Citation1990). The serum obtained from the blood sample was purified and IgG fraction obtained following the method described by Clausen (Citation1988). IgG was used for enzyme-linked immune-sorbent assay (ELISA) and Dot-immunobinding assay (DIBA) where the antigen source was the rhizosphere soil previously inoculated with the bacterium. Prior to detection of the bacterium in soil, specificity of the antibody was checked by agar gel double diffusion test, ELISA and Dot-immunobinding assay. Soil antigens were prepared both from untreated and S. marcescens treated soil following the method of Walsh et al. (Citation1996). ELISA was performed following the method described by Chakraborty et al. (Citation1995). For ELISA, secondary antibody labeled with alkaline phosphatase was used as the enzyme source and pNPP (Sigma Chemicals, USA) as the substrate. Absorbance in ELISA was determined in a Multiscan Ex (ThermoElectro, Mumbai, India) ELISA Reader at 405 nm. For DIBA, the method of Lange et al. (Citation1989) was followed. In this case, secondary antibody labeled with alkaline phosphatase was used as the enzyme source and NBT/BCIP (Genei, India) as the substrate. Color intensity was scored visually.
Evaluation of phosphorus solubilizing activity in vitro
Primary phosphate solubilizing activity of S. marcescens was carried out by allowing the bacterium to grow in selective medium, i.e. Pikovskaya's agar (Himedia, M520; ingredients: yeast extract, 0.50 g/l; dextrose, 10.00 g/l; calcium phosphate, 5.00 g/l; ammonium sulphate, 0.50 g/l; potassium chloride, 0.20 g/l; magnesium sulphate, 0.10 g/l; manganese sulphate, 0.0001 g/l; ferrous sulphate, 0.0001 g/l; and agar, 15.00 g/l) for 7–10 days at 37°C (Pikovskaya Citation1948). The appearance of transparent halo zone around the bacterial colony indicated the phosphate solubilizing activity of the bacterium.
Extraction and quantification of soil, root and leaf phosphate
Soil sample (1 g) was air dried and suspended in 25 ml of the extracting solution (0.025N H2SO4, 0.05N HCl) to which activated charcoal (0.01 g) was also added, shaken well for 30 min on a rotary shaker and filtered through Whatman No. 2 filter paper (Mehlich Citation1984). In the case of plant leaf and root samples, oven-dried plant material was crushed with extracting solution. Quantitative estimation of phosphate was carried out following ammonium molybdate-ascorbic acid method as described by Knudsen and Beegle (Citation1988).
Assay of acid and alkaline phosphatase (EC 3.1.3.2 & EC 3.1.3.1) activity in soil
About 2 g portions of each soil sample was used for enzyme extraction and assays. The activities of enzymes were expressed according to method of Tominaga and Takeshi (Citation1974) with modifications.
For acid phosphatase assay, soil samples were extracted in 5 ml of 50 mM sodium acetate buffer (pH 5.0) using a chilled mortar and pestle which was then transferred into a tube and solution was shaken well. 1 ml of 5 mM p-nitrophenyl phosphate solution was added to the tube. All the tubes along with control were allowed to incubate at 37°C for 1 h. After incubation, 2 ml of solution was transferred into centrifuge tubes. Centrifugation was performed at 10,000 rpm for 2 min at 4°C. Finally the supernatant was transferred into clean spectrophotometer cuvettes and the reaction was terminated by addition of 4.0 ml of 100 mM NaOH. The amount of p-nitrophenol liberated was determined from the absorbance at 400 nm. Enzyme activity was expressed as µmol p-nitrophenol liberated/sec/g of soil.
The procedure for the assay of alkaline phosphatase was similar to acid phosphatase except that for enzyme extraction and incubation 100 mM sodium bicarbonate buffer (pH 10.0) was used.
Biochemical analyses
Leaves of tea seedlings grown in treated or control soil were used for all biochemical analyses. Leaves were collected for assay 72 h after inoculation.
Enzyme assays
Peroxidase (POX, EC1.11.1.7). Extraction and assay of peroxidase was carried out following the method described by Chakraborty et al. (Citation1993). For the extraction of peroxidase, the plant tissues were macerated to powder in liquid nitrogen and extracted in 5 ml of chilled 0.05(M) sodium phosphate buffer (pH 6.8) containing 2 mM β mercaptoethanol. The reaction mixture contained 1 ml of 0.2M Na-phosphate buffer (pH 5.4), 1.7 ml dH2O, 100 µl crude enzyme, 100 µl O-dianisidine (5 mg/ml methanol) and 0.1 ml of 4 mM H2O2. O-dianisidine was used as substrate and activity was assayed spectrophotometrically at 465 nm by monitoring the oxidation of O-dianisidine in presence of H2O2. Specific activity expressed as the increase in Δ A 465/g tissue/min.
Chitinase (CHT, EC 3.2.1.14). Chitinase was extracted and assayed from tea leaves following the method of Boller and Mauch (Citation1988). Leaf samples were crushed in liquid nitrogen and extracted using 5 ml of chilled 0.1(M) sodium acetate buffer (pH 5.0). Assay mixture consisted of 10 µl of 1M Na-acetate buffer (pH 4), 0.4 ml enzyme solution and 0.1 ml colloidal chitin. Incubation was done for 2 h at 37°C and centrifuged at 10,000 rpm for 3 min. 0.3 ml supernatant, 30 µl of 1 M K-PO4 buffer (pH 7.1) and 20 µl Helicase (3%) were mixed and allowed to incubate for 1 h at 37°C. 70 µl of 1 M Na-borate buffer (pH 9.8) was added to the reaction mixture. The mixture was again incubated in a boiling water bath for 3 min and rapidly cooled in ice water bath. 2 ml DMAB (2% di methyl amino benzaldehyde in 20% HCl) was finally added and incubated for 20 min at 37°C.The amount of GlcNAc released was measured spectrophotometrically at 585 nm using a standard curve and activity expressed as µg GlcNAc released/min/g fresh wt. tissue.
Phenylalanine Ammonia-Lyase (PAL, EC 4.3.1.5). Enzyme was extracted and assay was performed by the method described by Chakraborty et al. (Citation1993). Leaf samples were crushed in liquid nitrogen and extracted using 5 ml of sodium borate buffer (pH 8.8) containing 2 mM β mercaptoethanol in ice followed by centrifugation at 15,000 rpm for 20 min at 4°C. The supernatant was collected and after recording its volume, used immediately for assay or stored at −20°C. The assay mixture was prepared by mixing 500 µl crude enzyme, 300 µl of 0.3 mM borate buffer (pH 8.0), 300 µl of 2% L-phenylalanine and 1.9 ml distilled water. The mixture was allowed to incubate for 1 h at 40°C and then absorbance value was measured at 290 nm. The amount of cinnamic in the assay mixture was determined spectrophotometrically using a standard curve and the activity was expressed as the amount of cinnamic acid produced from L-phenylalanine by the enzyme from 1 g tissue/min.
β-1,3-glucanase (β-GLU, EC 3.2.1.38). β-1,3-glucanase was extracted and assayed from leaf samples following the method of Pan et al. (Citation1991). Leaf sample (1 g) was crushed in liquid nitrogen and extracted using 5 ml of chilled 0.05 M sodium acetate buffer (pH 5.0). The extract was then centrifuged at 10,000 rpm for 15 min at 4°C and the supernatant was used as crude enzyme extract. The reaction mixture consisted of 62.5 µl crude enzyme and 62.5 µl 4% laminarin which was incubated at 40°C for 10 min and 375 µl DNSA (dinitro salicylic acid) added to the mixture following incubation for 5 min on a boiling water bath. Finally the colored solution was diluted with 4.5 ml water and the amount of glucose liberated was determined spectrophotometrically using a standard curve. Activity was expressed as µg glucose released/min/g tissue.
Phenolics
Phenols were extracted and estimated from leaf samples by the method of Mahadevan and Sridhar (Citation1982). Quantification of total phenol was done using a standard of caffeic acid.
In vitro antagonistic tests
In vitro antagonism of the bacterium to five fungal pathogens was determined by paired culture on nutrient agar medium as described by Chakraborty et al. (Citation2006).
Pathogen inoculation and disease assessment
Tea plants were inoculated by adding 100 g of previously prepared inoculum of F. lamaensis to the rhizosphere of each plant. Inoculation was done three days after final application of S. marcescens. Disease assessment was performed following the method of Chakraborty et al. (Citation2006) after 15, 30 and 45 days of inoculation. Disease intensity was assessed as rot index on a scale of 0–6, depending on both underground and above ground symptoms as follows: Rot index: 0 – no symptoms; 1 – small roots turn brownish and start rotting; 2 – leaves start withering and 20–40% of roots turn brown; 3 – leaves withered and 50% of roots affected; 4 – shoot tips also start withering; 60–70% roots affected; 5 – shoots withered with defoliation of lower withered leaves, 80% roots affected; 6 – whole plants die, with upper withered leaves still remaining attached; roots fully rotted. Each experiment was replicated three times, with 10 plants/variety in each replicate.
Statistical analyses
Results were analyzed statistically by determining standard error, Student's t-test and ANOVA wherever appropriate.
Results
Plant growth promotion
Application of S. marcescens to the rhizosphere of 18-month old tea of five varieties in the field resulted in an increase in growth in terms of increase in height, leaf number, number of branches and dry mass of leaves ( and ). Determination of height in seedlings, after one and two months of application, showed an increase in all varieties. However, statistical analysis by Student's t-test revealed that after 1 and 2 months of application, the increase in height was significant in T-17 and TV-26. On the other hand, the increase in the number of leaves was highly significant after one month of application in three varieties and two months after application in four varieties. The increase in the number of leaves, number of branches and dry mass of leaves due to the application of S. marcescens was statistically significant, six months after application in most of the varieties. In potted plants, the application of S. marcescens revealed the same trend as in the field, with the increase in the number of leaves being more significant than the increase in height. In this case, a significant increase in height after one month of application was observed in TV-23 and TV-26 only. However, after two months, it was significant in four varieties, and not significant in only T-17 ().
Figure 1. Increase in height of seedlings (A) and number of leaves (B) of different tea varieties after one and two months of application of S. marcescens in the field. (C = Control; Sm = rhizosphere treated with S. marcescens; 1 mo = 1 month after application; 2 mo = 2 months after application). Different letters above bars indicate significant difference in t-test at p=0.01 between C and 1 mo or C and 2 mo of treatment of each variety.
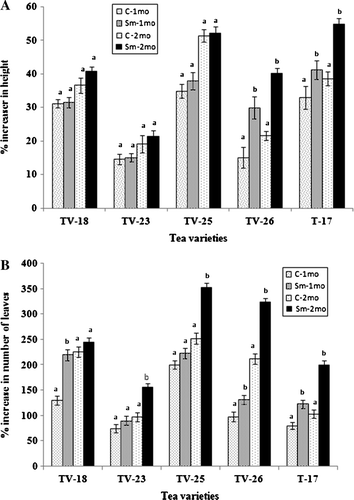
Figure 2. Effect of S. marcescens on the growth of tea varieties in the field six months after application, measured in terms of increase in number of leaves (A), number of branches (B) and dry mass of leaves (C). Different letters above bars indicate significant difference in t-test at p=0.01 between control and treated in each variety.
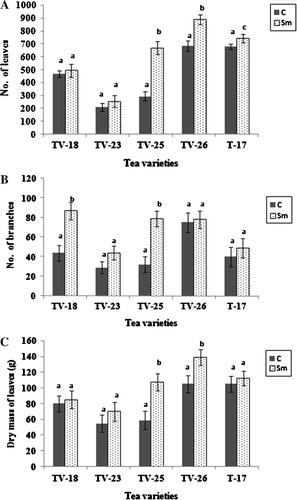
Table 1. Effect of S. marcescens applied as aqueous suspension or bioformulations on growth of potted tea plants.
Besides aqueous suspension, the effects of S. marcescens in various bioformulations were also tested on growth. Before application in the field, the survivability of S. marcescens in the various bioformulations was tested in vitro. It could survive in the range of 1×108 cfu/ml in bioformulations of sawdust, rice husk and 1×107 cfu/ml in tea waste, respectively, up to nine months or more.
The application of S. marcescens in bioformulations of sawdust, rice husk and tea waste also led to a significant increase in growth of tea seedlings (). The growth was measured in terms of increase in height and the number of leaves of seedlings one and two months after application. The observed increase was similar to that observed by application of aqueous suspension of the bacterium. Statistical analysis (ANOVA) revealed that there was no significant difference among the aqueous suspension or different bioformulations although all of them were significantly higher than control.
Survival of S. marcescens in the rhizosphere
Survival of S. marcescens in soil following application was determined immunologically using the PAb raised against the bacterium. ELISA and DIBA were performed immediately and six months after application to the soil. Tests revealed that the bacterium could survive and multiply in the rhizosphere ().
Table 2. ELISA and DIBA values of rhizosphere soil antigens reacted with PAb of S. marcescens.
Phosphate contents
Serratia marcescens could solubilize phosphate in vitro as evidenced by the appearance of halo zone around the inocula in PVK medium. Soil P content decreased following application of S. marcescens, while root and leaf phosphate contents showed an increase both in treated tea plants in potted as well as field conditions in comparison to control (, ). Maximum phosphate content was obtained in the leaf tissues.
Figure 3. Phosphate content of rhizosphere soil (A), roots (B) and leaves (C) of tea varieties grown in uninoculated or S. marcescens inoculated soil in the field. Different letters above bars indicate significant difference in phosphate contents as determined in t-test at p=0.01 between control and treated in each variety.
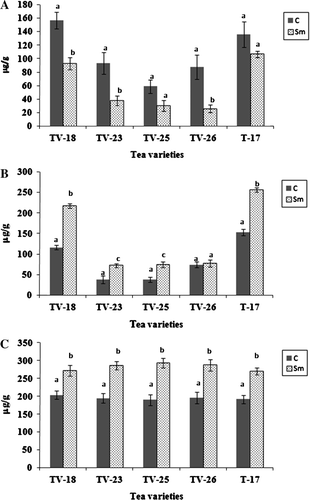
Table 3. Phosphate contents of rhizosphere soil, root and leaves of treated and control plants under field conditions.
Phosphatase activity
Both acid and alkaline phosphatase activities in rhizosphere soil of all five varieties were enhanced following application of the bacterium (). In rhizosphere soil of untreated plants, alkaline phosphatase activity was higher than acid phosphatase, but after treatment with S. marcescens, acid phosphatase showed a greater increment of activity.
Table 4. Acid and alkaline phosphatase activities from rhizosphere soil of treated and control plants in the field.
Phenolics and defense enzymes
Total phenol contents were estimated in tea leaves of the different tea varieties following the application of S. marcescens and challenge inoculation with F. lamaoensis. In all cases, young leaves were selected from tea plants of all five varieties and analyses were performed immediately after 72 h of bacterial inoculation to the rhizosphere. There was an increase in phenol contents of leaves in all three treatments (S. marcescens, F. lamaoensis and S. marcescens+F. lamaoensis), but the most significant increase was when there was challenge inoculation (). In the case of defense enzymes, POX, PAL, CHT and GLU, similar results were also obtained ( and ). Activities of POX increased about four times, while CHT was also doubled. Accumulation of phenolics was also twice in the treated plants than in control.
Figure 4. Peroxidase (A) and phenylalanine ammonia-lyase (B) activities in leaves of tea varieties grown in soil treated with S. marcescens and F. lamaoensis. Different letters above bars indicate significant difference in enzyme activities as determined in t-test at p=0.01 among the different treatments.
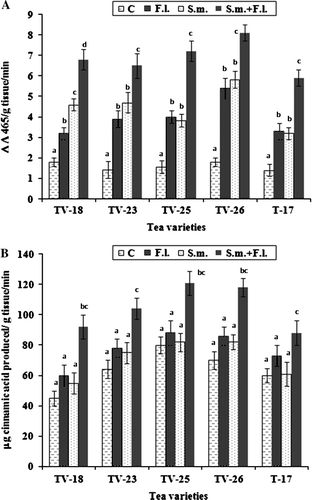
Table 5. Phenol contents in leaves of tea plants grown in treated and control soil.
Table 6. Activities of chitinase and β-1,3-glucanase in leaves of tea plants grown in treated and control soil.
In vitro antagonistic tests
Serratia marcescens was tested against four root rot pathogens of tea in both solid and liquid medium in vitro. Results revealed that S. marcscens inhibited all the test pathogens significantly (). In solid medium, inhibition ranged from 39–74%, while in liquid medium it ranged from 24–59%.
Figure 5. Pairing of S. marcescens with test fungi in solid medium and growth of test fungi in presence of S. marcescens in liquid medium. (P. h=Poria hypobrunnea; F. l=Fomes lamaoensis; S. r=Sclerotium rolfsii; U. z=Ustulina zonata; S. m=Serratia marcescens). Different letters above bars indicate significant difference in growth of each fungus alone or in combination with the bacterium as determined in t-test at p=0.01 between control and treated in each variety.
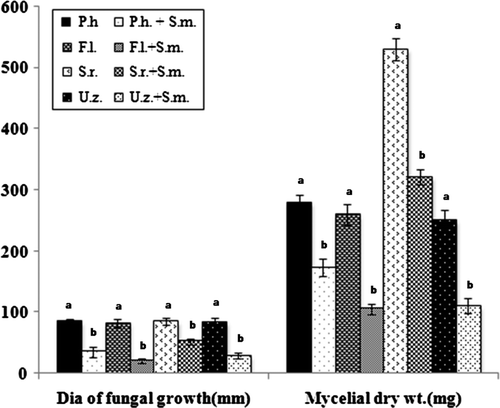
Disease control
Serratia marcescens reduced root rot disease of tea caused by F. lamaoensis in all five tested varieties when observations were made 15, 30 and 45 days after inoculation with the pathogen (). Analysis of results revealed that disease intensity was reduced by 48–65% in the different varieties 15 days after inoculation, while 45 days after inoculation, when disease severity was high, disease intensity was reduced by 33–48%.
Figure 6. Development of brown root rot disease of tea following inoculation with F. lamaoensis alone and in combination with S. marcescens. Data taken after 15, 30 and 45 days of inoculation (F. l = Fomes lamaoensis; S. m = Serratia marcescens). Different letters above bars indicate significant difference in root rot index as determined in t-test at p=0.01 between F. lamaoensis inoculated F. lamaoensis+S. marcescens inoculated in each variety.
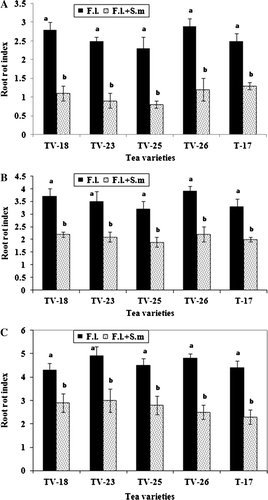
Discussion
Serratia marcescens TRS-1 was applied as aqueous suspension to the rhizosphere of 18-month old seedlings of five varieties of tea in the field and also to potted tea plants where the soil was non-sterile, with the natural rhizosphere micro flora. Application of the bacterium resulted in significant increase in growth, measured in terms of height, leaf number, number of branches and dry mass of leaves. As tea plants are cultivated for their leaves from which the beverage is made, an increase in leaf number is considered to be of importance to the tea industry. In an early study, S. marcescens NBR11213 was reported to induce plant growth promotion and biological control of foot and root rot of betelvine caused by Phytophthora nicotianeae (Lavania et al. Citation2006). Guo et al. (Citation2004) also reported that three strains of PGPRs – Serratia sp. J2, fluorescent Pseudomonas J3 and BB11 – provided disease control in tomato against tomato wilt and increased yield. Numerous studies have shown a substantial increase in plant growth and seed yield following inoculation with PGPR strains (Wani et al. Citation2007). In the present study, S. marcescens not only promoted plant growth, but also reduced intensity of brown root rot disease. Sustainability of the applied bacterium was tested immunologically by ELISA and the bacterium was found to survive well in the rhizosphere. Since the bacterium was originally isolated from tea rhizosphere, the addition of the bacterium to the rhizosphere would not have caused any ecological disbalance in the rhizosphere. In our earlier studies, S. marcescens was shown to have the ability to secrete IAA, produce siderophore, solubilize phosphate and was also antagonistic to fungal pathogens. It was also a non-HCN and chitinase-producing strain (Chakraborty et al. Citation2004). In the present study, the phosphate solubilizing ability and antagonistic activity of S. marcescens against a number of root rot pathogens of tea were confirmed. The ability of PGPRs to convert insoluble phosphorus (P) to an accessible form is an important trait for increasing plant yields. The fact that certain soil microbes are capable of dissolving relatively insoluble phosphatic compounds has opened the possibility of inducing microbial solubilization of phosphates in the soil (Asea et al. Citation1988; Nahas et al. Citation1990; Bojinova et al. Citation1997). Soil P content decreased following application of S. marcescens, while root and leaf phosphate contents showed an increase both in treated tea plants in potted as well as field conditions in comparison to control. Maximum phosphate content was obtained in the leaf tissues. Some PGPR biofertilizers also influence the availability of phosphate by secreting phosphatases for mineralization of organic phosphates (Rodriguez and Fraga Citation1999). Phosphate is often the limiting nutrient for microbial and plant growth in soil, phosphatases remove the phosphate from organic compounds and convert it in soluble form to the plants. Both acid and alkaline phosphatase activities in rhizosphere soil of all five varieties were enhanced following application of the bacterium. Results of the present study, therefore clearly revealed S. marcescens to have the ability of phosphate solubilization in the soil.
Since S. marcescens showed antagonism to F. lamoensis in vitro, it could have reduced the population of the pathogen in the soil, and hence, reduce disease severity. However, PGPRs are known to act both by direct and indirect mechanisms and biopriming with such PGPRs can cause induction of resistance in the host. In the present study, in order to determine whether the bacterium could induce systemic resistance (ISR) in tea plants, accumulation of defense-related enzymes and phenolics were studied. Results revealed that S. marcescens enhanced activities of defense-related enzymes peroxidase, chitinase, phenylalanine ammonia-lyase and β-1,3-glucanase as well as total and O-dihydroxy phenols. Since the application of S. marcescens was in the soil and increased accumulation of defense-related biochemical components were observed in the leaves, it is quite apparent that S. marcescens induces systemic response. However, since the present study involved a root disease and application of the bacterium was done in the soil itself, induction of ISR has been proposed as a mechanism on the basis of biochemical tests. While there are several reports of ISR in vegetative plants, information regarding perennials are rather less. Jeun et al. (Citation2004) reported that inoculation of plant growth promoting strain S. marcescens (90–166) and Pseudomonas fluorescens (89B61) induced systemic protection against anthracnose pathogen. Maurhofer et al. (Citation1994) reported that induction of systemic resistance by P. fluorescens was correlated with accumulation of β-1,3-glucanase. Lavania et al. (Citation2006) also reported enhanced accumulation of phenolics and defense enzymes in betelvine treated with S. marcescens and challenge inoculated with P. nicotineae. In the present study, the activities of peroxidase and chitinase were enhanced most significantly. Similarly, the accumulation of phenolics was also highly significant. Both POX and CHT are PR proteins and their increased activity would indicate activation of such PR proteins during defense. Besides, POX plays a key role in the biosynthesis of lignin which limits the extent of pathogen spread because of the antifungal activity (Bruce and West Citation1989). Similar findings of accumulation of PR proteins such as POX, CHT, GLU, and PAL after application with biocontrol agents and their involvement in inducing systemic resistance against the pathogen have been reported by several workers in different crops (Meena et al. Citation2000; Oostendorp et al. Citation2001; Bargabus et al. Citation2004; Bharati et al. Citation2004).Thus the present study indicated that higher accumulation of defense-related enzymes and phenolics occurred when resistance is induced in tea plants.
During plant growth promoting and disease management strategies, the use of plant growth promoting microbes as biocontrol agents and biofertilizers gives eco-friendly and inexpensive alternatives to the use of chemicals. However, for easy handling of such bacteria, it is necessary to pack such bacteria in inert materials which can also be packaged and stored. Initially, it is essential to determine whether the bacteria can survive in the bioformulations for a reasonable period of time and whether they can induce similar effects to those observed by live bacterial cells. Keeping this in mind, three bioformulations of S. marcescens were prepared and initially their survivability was determined. The bacterium survived in these bioformulations for more than nine months. The bioformulations were also as effective as aqueous suspensions in plant growth promotion, but the effects of all three tested bioformulations were more or less similar. The increase in growth in the bacterial bioformulations was not due to any effect of sawdust, rice husk or tea waste, as these are neutral substances with no known plant growth promoting activities. There are also several previous reports on application of PGPRs as formulations (Mathivanan et al. Citation2005; Trivedi et al. Citation2005; Hassan-El and Gowen Citation2006; Tilak and Reddy Citation2006).
In conclusion, it may be stated that S. marcescens TRS-1 has been found to be a plant growth promoter with the ability to reduce root rot disease of tea which acts both by direct and indirect mechanisms in the host. S. marcescens is also effective both as actively growing cells and as formulations.
Acknowledgements
Financial help received from Department of Biotechnology, Ministry of Science & Technology, Government of India, is gratefully acknowledged.
References
- Asea , PEA , Kucey , RMN and Stewart , JWB. 1988 . Inorganic phosphate solubilization by two Penicillium species in solution culture and soil . Soil Biol Biochem. , 20 : 459 – 464 .
- Bai , Y , Souleimanov , A and Smith , DL. 2002 . An inducible activator produced by a Serratia proteamaculans strain and its soybean growth promoting activity under green house conditions . J Exp Bot. , 53 : 1495 – 1502 .
- Bargabus , RL , Zidack , NK , Sherwood , JE and Jacobsen , BJ. 2004 . Screening for the identification of potential biological control agents that induce systemic acquired resistance in sugar beet . Biol Cont. , 30 : 342 – 350 .
- Bharati , R , Vivekananthan , R , Harish , S , Ramanathan , A and Samaiyappan , R. 2004 . Rhizobacteria-based bioformulations for the management of fruit rot infection in chillies . Crop Protect. , 23 : 835 – 843 .
- Bojinova , D , Velkova , R , Grancharov , I and Zhelev , S. 1997 . The bioconversion of Tunisian phosphorite using Aspergillus niger . Nutr Cyc Agroecosyst. , 47 : 227 – 232 .
- Boller , T and Mauch , F. 1988 . Colorimetric assay for chitinase . Meth Enzymol. , 161 : 430 – 435 .
- Bruce , RJ and West , CA. 1989 . Elicitation of lignin biosynthesis and isoperoxidase activity by pectic fragments in suspension cultures of castor bean . Plant Physiol. , 91 : 889 – 897 .
- Chakraborty , BN , Basu , P , Das , R , Saha , A and Chakraborty , U. 1995 . Detection of cross reactive antigen between Pestalotiopsis theae and tea leaves and their cellular location . Ann Appl Biol. , 207 : 11 – 21 .
- Chakraborty , U , Chakraborty , BN and Basnet , M. 2006 . Plant growth promotion and induction of resistance in Camellia sinensis by Bacillus megaterium . J Basic Microbiol. , 46 : 186 – 195 .
- Chakraborty , U , Chakraborty , BN and Kapoor , M. 1993 . Changes in the levels of peroxidase and phenyl alanine ammonia lyase in Brassica napus cultivars showing variable resistance to Leptosphaeria maculans . Folia Microbiol. , 38 : 491 – 496 .
- Chakraborty , U , Chakraborty , BN , Tongden , C , Roychowdhury , P and Basnet , M. 2004 . Assessment of tea rhizosphere microorganisms as plant growth promoters . Tea. , 25 : 38 – 47 .
- Clausen J. 1988 . Laboratory techniques in biochemistry and molecular biology 1 , part 3 . Burdon RH , Van Kinppenberg , PH , 64 65 .
- Duff , SMG , Sarath , G and Plaxton , WC. 1994 . The role of acid phosphatase in plant phosphorus metabolism . Physiol Plant. , 90 : 791 – 800 .
- Guo , JH , Qi , HY , Guo , YH , Ge , HL , Gong , LY , Zhang , LX and Sun , PH. 2004 . Biocontrol of tomato wilt by plant growth promoting rhizobacteria . Biol Cont. , 29 : 66 – 72 .
- Hassan-El , SA and Gowen , SR. 2006 . Formulation and delivery of the bacterial antagonist Bacillus subtilis for management of lentil vascular wilt caused by Fusarium oxysporum f. sp. lentis . J Phytopathol. , 154 : 148 – 155 .
- Jeun , YC , Park , KS , Kim , CH , Fowler , WD and Kloepper , JW. 2004 . Cytological observation of cucumber plants during induced resistance elicited by rhizobacteria . Biol Control. , 29 : 34 – 42 .
- Kloepper , JW. 1993 . “ Plant growth promoting rhizobacterial as biological control agents ” . In Soil microbial ecology – applications in agricultural and environmental management , Edited by: Metting , F Jr . 255 – 274 . New York : Marcel Dekker .
- Kloepper , JW and Schroth , MN. 1978 . Plant growth promoting rhizobacteria on radishes. IV. International Conference on Plant Pathogenic Bacteria. Angers . France. , 2 : 879 – 882 .
- Kloepper , JW , Zehnder , GW , Tuzun , S , Murphy , JF , Wei , G , Yao , C and Raupach , GS. 1996 . “ Toward agricultural implementation of PGPR-mediated induced systemic resistance against crop pests ” . In Advances in biological control of plant diseases , Edited by: Wenhua , T , Cook , RJ and Rovira , A . 165 – 174 . Beijing : China Agricultural University Press .
- Knudsen D , Beegle D . 1988 . Recommended phosphorous tests . In : Dahnke WC , Recommended chemical soil tests procedures for the north central region. Bull North Dakota Agric Exp Stn North Dakota USA 499 : 122 115 .
- Lange , L , Heide , M and Olson , LW. 1989 . Serological detection of Plasmodiophora brassiceae by dot immunobinding and visualization of the serological by scanning electron microscopy . Phytopathology. , 79 : 1066 – 1071 .
- Lavania , M , Chauhan , PS , Chauhan , SV , Singh , HB and Nautiyal , CS. 2006 . Induction of plant defense enzymes and phenolics by treatment with plant growth promoting rhizobacterial Serratia marcescens NBRII213 . Curr Microbiol. , 52 : 363 – 368 .
- Lumsden , RD , Lewis , JA and Fravel , DR. 1995 . “ Formulation and delivery of bio-control agents for use against soil-borne plant pathogens ” . In Biorational pest control agents formulation and delivery , Edited by: Hall , FR and Bary , JW . 166 – 182 . Washington, DC : American Chemical Society .
- M'Piga , P , Belanger , RR , Paulitz , TC and Benhamou , N. 1997 . Increased resistance to Fusarium oxysporium f.sp. radicis- lycopersici in tomato plants treated with the endophytic bacterium Pseudomonas fluorescens strain 6328 . Physiol Mol Pl Pathol. , 50 : 301 – 320 .
- Mahadevan A , Sridhar R . 1982 . Methods in physiological plant pathology , 2nd ed Sivakami Publication India .
- Manjula , K and Podile , AR. 2001 . Chitin-supplemented formulations improve biocontrol and plant growth promoting efficiency of Bacillus subtilis AF1 . Can J Microbiol. , 47 : 618 – 625 .
- Mathivanan , N , Prabhavathy , VR and Vijayanandraj , VR. 2005 . Application of talc formulations of Pseudomonas fluorescens Migula and Trichoderma viride Pers.ex S.F. Gray decrease the sheath blight disease and enhance the plant growth and yield in rice . J Phytopathol. , 153 : 697 – 701 .
- Maurhofer , M , Hase , C , Meuwly , P , Metraux , JP and Defago , G. 1994 . Induction of systemic resistance of tobacco to tobacco necrosis virus by the root colonizing Pseudomonas fluorescens CHAO: Influence of the gacA gene and of pyoverdine production . Phytopathology. , 84 : 139 – 146 .
- Mazarei , M , Hajimorad , RM and Kerr , A. 1992 . Specificity of polyclonal antibodies to different antigenic preparations of Pseudomonas syringae pv. pisi strain UQM551 and P. syringae pv. syringae strain. L . Plant Pathol. , 41 : 437 – 443 .
- Mazarei , M and Kerr , A. 1990 . Distinguishing pathovars of Pseudomonas syringae on peas: Nutritional, pathogenicity and serological tests . Plant Pathol. , 39 : 278 – 285 .
- Meena , B , Radhajeyalakshmi , R , Marimuthu , T , Vidhyasekaran , P , Sabitha , D and Velazhahan , R. 2000 . Induction of pathogenesis related proteins, phenolics and phenylalanine ammonia lyase in groundnut by Pseudomonas fluorescens . J Plant Dis Protect. , 107 : 514 – 527 .
- Mehlich , A. 1984 . Mehlich 3 soil test extractant: A modification of the Mehlich 2 extractant . Commun Soil Sci Plant Anal. , 15 : 1409 – 1416 .
- Nahas , E , Banzatto , DA and Assis , LC. 1990 . Fluorapatite solubilization by Aspergillus niger in vinasse medium . Soil Biol Biochem , 22 : 1097 – 1101 .
- Oostendorp , M , Kunz , W , Dietrich , B and Staub , T. 2001 . Induced resistance in plants by chemicals . Eur J Plant Pathol. , 107 : 19 – 28 .
- Orhanovic , S and Pavela-Vrancic , M. 2000 . Alkaline phosphatase activity in sea water: Influence of reaction conditions on the kinetic parameters of ALP . Croat Chim Acta. , 73 : 819 – 830 .
- Pan , SQ , Ye , XS and Kue , J. 1991 . A technique for detection of Chitinase, β-1,3-glucanase and protein patterns after a single separation using polyacrylamide gel electrophoresis and isoelectric focusing . Phytopathology. , 81 : 970 – 974 .
- Pikovskaya , RI. 1948 . Mobilization of phosphorous in soil connection with the vital activity of some microbial species . Microbiologiya. , 17 : 362 – 370 .
- Rodriguez , H and Fraga , R. 1999 . Phosphate solubilizing bacteria and their role in plant growth promotion . Biotechnol Adv. , 17 : 319 – 339 .
- Schmidt , CS , Lorenz , D , Wolf , GA and Jager , J. 2001 . Biological control of the grapevine dieback fungus Eutypa lata II: Influence of formulation additives and transposon mutagenesis on the antagonistic activity of Bacillus subtilis and Erwinia herbicola . J Phytopathol. , 149 : 437 – 445 .
- Sid Ahmed , A , Ezziyyani , M , Sanchez , CP and Candela , ME. 2003 . Effect of chitin on biological control activity of Bacillus spp and Trichoderma harzianum against root rot disease in peper (Capsicum annuum) plants . Eur J Plant Pathol. , 109 : 633 – 637 .
- Tabaldi , LA , Ruppenthal , R , Cargnelutti , D , Morsch , VM , Pereira , LB and Schetinger , MRC. 2007 . Effects of metal elements on acid phosphatase activity in cucumber (Cucumis sativus L.) seedlings . Environ Exp Bot. , 59 : 43 – 48 .
- Tilak , KVBR and Reddy , BS. 2006 . Bacillus cereus and B. circulans – novel inoculants for crops . Curr Sci. , 90 : 642 – 644 .
- Tominaga , N and Takeshi , M. 1974 . A sulfite dependent acid phosphatase of Thiobacillus thioxidans . J Biochem. , 76 : 419 – 428 .
- Trivedi , P , Pandey , A and Palni , LMS. 2005 . Carrier-based preparations of plant growth promoting bacterial inoculants suitable for use in cooler regions . World J Microbiol Biotech. , 21 : 941 – 945 .
- Walsh , JA , Merz , U and Harrison , JG. 1996 . Serological detection of spore balls of Spongospora subterranea and quantification in soil . Plant Pathol. , 45 : 884 – 895 .
- Wani , PA , Khan , MS and Zaidi , A . 2007 . Synergistic effects of the inoculation with nitrogen fixing and phosphate solubilizing rhizobacteria on the performance of field grown chickpea . J Plant Nutr Soil Sci. , 170 : 283 – 287 .