Abstract
In order to identify phytohormones involved in the initiation and maintenance of galls on the hackberry tree, Celtis occidentalis (Ulmaceae), in the presence of the insect Pachypsylla celtidis (Psyllidae); endogenous levels of cytokinins (CKs) and abscisic acid (ABA) were measured in the tissues of leaves, galls, and larval insects using liquid-chromatography-tandem-mass-spectrometry. The CKs isopentenyl adenine, isopentenyl adenosine, trans-zeatin and cis-zeatin, were extremely concentrated in insect larvae compared to surrounding tissue of leaves and galls. ABA concentrations in the insects were also relatively high at about 3268 pmol•g-1 fresh mass – approximately 25 times higher than in leaves, and 17 times higher than in galls. This represents a novel case of high concentrations of ABA being found in a larval insect. These findings indicate that manipulation of CK and ABA are involved in the initiation and maintenance of hackberry galls in the presence of larval P. celtidis.
Introduction
Plants and herbivorous insects have co-evolved closely, driving what has been called an ecophysiological ‘arms race’ between herbivorous insects and the plants they attack (Ehrlich and Raven Citation1964; Berenbaum and Feeny Citation1981). While many of these contests are purely physical, involving structural aspects of plant physiology, chemical interactions between insects and their hosts are also important (Schultz Citation2002; Raman Citation2007). Schultz (Citation2002) extended the warfare analogies even further, noting the similarities between cytokinins used for regulation of growth and metabolism in both plants and animals, and describing some of their interactions as ‘phylogenetic espionage’. As herbivores, gall-forming insects are presented with a particularly interesting problem because they often live within the plant on which they are feeding for extended periods of time, and cause it to form structures that can be used for feeding as well as shelter. Cytokinins (CKs), which usually play a role in promoting rapid growth and cell division, have been linked to the formation of galls in a number of plant/herbivore systems (Elzen Citation1983). Examples include the tumour-forming pathogen Agrobacterium tumifasciens (Van Onckelen et al. Citation1984), the gall-forming wasp Trichilogaster acaciaelongifoliae (Pteromalidae) on Acacia longifalia (Dorchin et al., 2009), and the goldenrod ball-gall fly, Eurosta solidaginis (Tephridae) on Solidago spp. (Mapes and Davies Citation2001). Mapes and Davies (Citation2001) used gas chromatography-mass spectrometry to isolate high concentrations of CKs from larval Eurosta solidaginis, and postulated that the insects may have been producing and secreting the CK isopentenyladenine.
The role of abscisic acid (ABA) as an insect-to-plant chemical signal is less clear than that of many CKs. ABA is usually involved in stress-responses related to osmotic regulation (Wilkinson and Davies Citation2002), and is important for responses to impending drought or salt stress such as stomatal closure (Wilkinson and Davies Citation2002; Zhang et al. Citation2006). ABA may also play roles in regulating seed-maturation (Baud et al. Citation2008), and defence against herbivores and pathogens as part of the upstream methyl-jasmonate pathway (Thaler and Bostock Citation2004). Recently, ABA has been found to interact with ethylene to help form arbuscules, facilitating plant-micorrhizal associations (Herrera-Medina et al. Citation2007). ABA may also be used by fungi to suppress defensive responses in host plants (Schmidt et al. Citation2008), although no studies to date have reported large amounts of ABA in herbivorous insects.
In order to initiate the formation of galls that are useful for protection and feeding, insect larvae must first successfully invade the plant, avoiding or overcoming its physical and chemical defences (Tooker et al. Citation2008). Then the insects must cause the gall to be created, which often involves manipulating the plant's chemical signalling system to alter the course of cellular development and differentiation (Tooker et al. Citation2008). Several different scenarios have been suggested, by which invading insects might produce this effect:
-
Insects could manipulate the plant, causing it to form galls by using high concentrations of phytohormones. These phytohormones could be produced by the insect itself (Lewis and Walton Citation1964; Mapes and Davies Citation2001), or concentrated from the plant (Giron et al. Citation2007; Van Staden and Davey Citation1978). In this case, the phytohormones responsible for the formation of galls would be present at low concentrations in the tissues of the plant and gall, and highly concentrated in the tissues of the insect.
-
Plants could form the galls as a response to the presence of insects. This would mean that the formation of galls must either be a generalized response to invasion by pathogens, or it might be a specific response to invasion by a particular insect, meaning the plant must pick up on chemical cues to recognize that insect (Hartley Citation1999; Tooker et al. Citation2008). In this case, insects would contain low concentrations of phytohormones that could be used to manipulate the plant. In contrast, the plant and the galls would be expected to contain high concentrations of these phytohormones.
One gall complex that has not been studied in detail in terms of its chemical physiology is the formation of galls on hackberry, Celtis occidentalis (Ulmaceae), by the aphid Pachypsylla celtidis-mamma (Fletcher 1883) (Psyllidae) (hereafter referred to as P. celtidis). Despite a lack of knowledge regarding the chemical interactions involved, the natural history of the Celtis/Pachypsylla complex has been fairly well described. The adult insects over-winter on tree bark or leaf litter, and emerge in the spring to mate just before leaves emerge (Wells Citation1920). Females lay eggs on the undersides of leaves just as they begin to expand (Wells Citation1920). After 2–3 days, eggs hatch, and larvae migrate to the upper side of leaves, where they burrow in, and initiate the formation of galls (Wells Citation1920). Larvae live and feed in the galls throughout the summer, reaching a maximum length of approximately 1.5 mm, and emerge as winged adults in late September (Wells Citation1920). The galls formed by P. celtidis on hackberry are also interesting in that they show clearly defined ‘green islands’ () – a phenomenon that is often associated with high levels of cytokinin activity (Giron et al. Citation2007; Walters et al. Citation2008).
Figure 1. Hackberry galls formed by the aphid Pachypsylla celtidis on leaves collected from the ground. The galls could be described as ‘green islands’, since they clearly appear green compared to the surrounding leaf tissue.
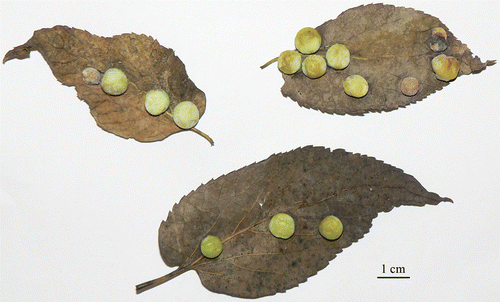
The purpose of this study was to examine the possible roles of CK and ABA in the formation of galls on hackberry, Celtis occidentalis, by the aphid P. celtidis. Recent advancements in liquid-chromatography-tandem-mass-spectrometry enabled us to differentiate between different forms of cytokinins, and compare our results to concentrations reported from the Solidago/Eurosta study system by Mapes and Davies (Citation2001).
Given the established roles of CKs in gall-formation, and the potential for them to be produced by gall-inducing insects (Mapes and Davies Citation2001; Dorchin et al. 2009), we hypothesized that CKs would be involved in the formation and maintenance of galls on hackberry by larvae of P. celtidis. We predicted that CKs would be present at high concentrations in the tissues of insects, and lower concentrations in the tissues of galls and leaves. Based on the role of endogenous ABA in pathways for defence against insect herbivores (Thaler and Bostock Citation2004), we hypothesized that ABA would be involved in the formation and maintenance of galls as a generalized anti-herbivore response by the plant. We predicted that concentrations of ABA would be highest in the tissues of galls, slightly lower in leaves, and extremely low or undetectable in insects.
Materials and methodology
Collection of tissue samples
Green leaves with and without galls were collected on 15 October 2008 from three hackberry trees in Peterborough, Ontario, Canada (44°21′03″ N, 78°18′18.75″ W). The leaves were taken directly from the trees and from the ground at the base of the trees. Approximately 70 galls were dissected into separate leaf tissue, galls, and larval insects. This was done by hand using sterilized scalpels and forceps. During dissection, galls were found to contain a waxy outer skin, fleshy soft body, and solid inner chamber, in which a single larval insect was housed. The fleshy part of the galls was easily removed from around the hard inner chamber, and larvae were removed from the chambers. The outer part of the gall (body and skin, excluding the hard inner chamber), was used as a source of tissue for the analysis. This minimized the possibility of contamination between treatments, since the insects did not come into direct contact with the fleshy part of the gall.
Leaves with no galls present were treated as controls. All tissue was stored at −20°C. The mass of leaf tissue, galls, and insects were taken after dissection. The amount of tissue used per extraction was 0.8–1.5 g of leaf tissue, 1.0–1.3 g of gall tissue, and 0.04–0.07 g of insect tissue. The full process of extraction and analysis was conducted on three replicates per tissue type.
Extraction and purification of phytohormones
Extraction and purification methods were modified from Quesnelle and Emery (Citation2007). The abbreviations of cytokinins used here are those recommended by Kamínek et al. (Citation2000). The insects were homogenized in approximately 0.5 ml cold (−20°C) modified Bieleski extraction buffer (CH3OH/H2O/HCOOH; 15:4:1, v/v/v) using steel ball-bearings in a Retsch MM 400 mixer-mill (Retsch GmbH, Germany) at 1 cycle per second for approximately 10 min. Frozen leaf and gall tissues were placed in 15 ml Falcon tubes and homogenized in cold modified Bieleski (at about 10 ml per gram fresh weight) using a Brinkmann Polytron homogenizer (Metrohm, USA).
As described in Quesnelle and Emery (2007), the following was added to each sample in order to quantify endogenous CK: 30 ng of 2 H-labelled 10 µg CK Mix, containing internal standards for [2H6]iP (isopentenyl adenine), [2H6][9R]iPR (isopentenyl adenine 9-riboside), [2H5]Z (trans-zeatin), [2H3]DZ (dihydrozeatin), [2H5][9R]ZR (zeatin riboside), [2H3][9R]DZR (dihydrozeatin riboside). As in Quesnelle and Emery (2007), estimates of recovery for cis-[9R]Z (cis-zeatin) and cis-[9RMP]ZR (cis-zeatin riboside) were based on recovery of 2 H-labelled standards of Z and ZR, respectively. 150 ng of 2 H-labelled ABA were added to each sample. After vortexing, tubes were centrifuged at 12,000 g, using an Eppendorf 5415D centrifuge (Eppendorf, Canada), and the supernatant was removed.
The remaining pellet was combined with 2.5 ml per gram fresh weight of modified Bieleski, centrifuged, and the supernatant was removed again. Combined supernatants were dried in vacuo at 40°C, then purified on an Oasis MCX column (Waters, Canada) following the procedure of Dobrev and Kamínek (Citation2002). ABA was eluted using 5 ml MeOH, and CK/free bases were eluted using 5 ml 0.35M NH4OH in 60% (v/v) MeOH. Extracted hormones were then dried using a SPD 111V-115 Speedvac (Thermo Fisher Scientific, USA).
Liquid chromatography – tandem mass spectrometry
Samples of extracted CK and ABA were analyzed on a Waters 2680 Alliance HPLC separation module (Waters, USA) linked to a Quattro-LC triple quadrupole mass spectrometer (Waters, USA), equipped with a Z-electrospray ionization source. The channels used for Multiple Reaction Monitoring (MRM) were those used by Quesnelle and Emery (2007) for CKs, and Radchuk et al. (Citation2007) for ABA. Data analysis was carried out using Masslynx version 3.5 software. Significant differences were determined using a one-way (factorial) ANOVA with Tukey's post hoc test. All statistics were carried out using STATISTICA 7.1 (Statsoft 2005).
Results
ABA and several CKs were recovered from all samples of insects, leaves, and galls, but in widely varying concentrations. Among the most prominent CKs were iP, iPR, iPRMP, trans-Z, and cis-Z (). Analysis of variance revealed that concentrations of iP, iPR, iPRMP, trans-Z, ZRMP, and cis-Z were significantly higher in larval insects than in galls or leaves. Total levels of CK were also extremely high in larval insects compared to galls and leaves ().
Figure 2. Differences in concentrations of total CK and ABA recovered from leaves and galls of Celtis occidentalis and larval Pachypsylla celtidis. Vertical bars represent standard error around the mean. Means with the same letter are not statistically different (p<0.05); lower-case letters are used to show relationships among total CK and upper-case letters are used for ABA.
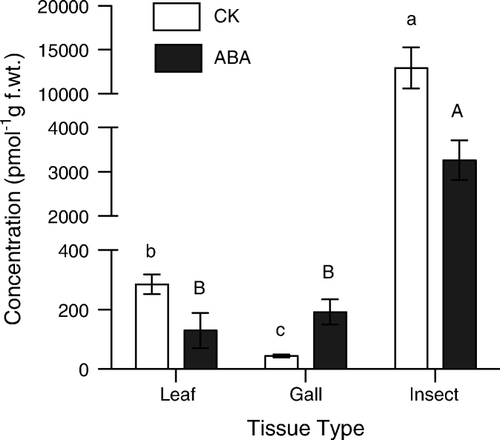
Table 1. Concentrations of cytokinins isolated from leaves and galls of Celtis occidentalis and larval Pachypsylla celtidis, expressed as pmol·g fresh weight (means±SE, n=3). Mean concentrations of phytohormones with the same letter are not statistically different among tissues types, as determined using a one-way ANOVA with Tukey's post-hoc test (p<0.05). The abbreviation n.d. denotes no detection. Concentrations of CKs found by Mapes and Davies (2001) in Eurosta solidaginis taken from developing ball-galls on Solidago spp. are provided for comparison.
Concentrations of iP, iPR, and iPRMP were lowest in galls, slightly higher in leaves, and much higher in larval insects (ANOVA; Tukey's Post Hoc, p<0.05; ). Z, ZRMP, and cisZ also had much higher concentrations in larval insects than in other tissue types, but concentrations found in leaves were not significantly different from those found in galls (ANOVA; Tukey's Post Hoc, p<0.05; ). While total CK was much lower in both leaves and galls than in the larval insects, this relationship did not hold true for all forms of CK; ZR, cisZR, DHZ, and DZHRMP all followed a similar pattern, with the highest concentrations of CK being found in leaves, lower concentrations in galls, and lowest concentrations in insects (ANOVA; Tukey's Post Hoc, p<0.05; ). CisZRMP was slightly different in that while leaves contained significantly higher concentrations than insects, the concentrations found in galls was not different from those found in either leaves or insects (ANOVA; Tukey's Post Hoc, p<0.05; ).
Levels of ABA were highest in larval insects, and significantly lower in galls and leaves () (ANOVA; Tukey's Post Hoc, p<0.05).
Only relatively low concentrations of DHZ as well as ZR and cisZR were found in galls, and none of these CKs were recovered from insects. Trace amounts of DHZRMP and both ZRMP and cisZRMP were found in leaves, galls, and insects. Of all the phytohormones examined, only endogenous DHZR was not recovered from leaves, galls, or insects.
Discussion
Larvae of P. celtidis contained extremely high concentrations of ABA, and the CKs iP, iPA, iPRMP, Z, and cisZ when compared to those found in the leaf and gall tissue. This pattern was consistent with Scenario 1, wherein we predicted that high concentrations of phytohormones would be present in the tissues of insects, and low concentrations would be present in galls and leaves. A closer examination of the relative concentration of different C CK metabolites reveals that the hormone profiles are different between plant and insect. First, there is a set of CKs that appear to be specific to the plant tissues. These are most concentrated in the leaves, present in lower concentration in the galls, and absent entirely from the larval insects. CKs that follow this pattern are ZR and cisZR (). Second, there is a group of CKs that are either synthesized or specifically concentrated in the larval insects to a point that is far in excess of that endogenously present in the tissues of the plants. These CKs were highly concentrated in larval insects, and present in far lower concentrations in the galls and leaves. The CKs that follow this pattern included iP, iPR, iPRMP, Z, and cisZ (). The presence of these particular forms of CK in high concentrations in the insect suggests the potential occurrence of a pathway for the synthesis of isopentenyl adenosine within the insect (). The ratios of CK forms suggest a pathway that involves the conversion from iPRMP to iPR and then to the free base forms iP, Z and cisZ () (Sakakibara Citation2006). It should be noted that in plants, cis-CKs are typically thought to be produced through a complex pathway involving conversion from tRNA (Armstrong Citation1994; Kasahara et al. Citation2004; Sakakibara Citation2006). However, intermediate forms of this pathway were not present in large concentrations in the aphid, suggesting instead the conversion from trans to cis free bases (). Interconversion between cis and trans isomers is thought to occur enzymatically via zeatin cis-trans isomerase, but this has not yet been shown in plants (Armstrong Citation1994; Sakakibara Citation2006).
Figure 3. Proposed pathway of CK-metabolism by the larval gall-forming insect Pachypsylla celtidis on its host plant Celtis occidentalis (simplified from Sakakibara 2006). Ratios are based on concentrations of CK in galls, leaves, and insects. Note that cis-trans isomerization (broken arrow) is thought to occur enzymatically via zeatin cis-trans isomerase, but this has not yet been shown in plants; an alternative possibility is the production of cis forms through a separate pathway involving tRNA.
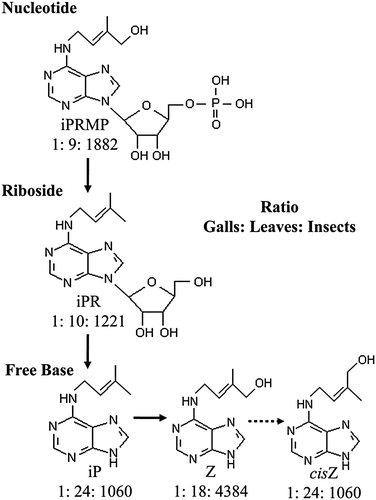
The presence of high concentration iPRMP (nucleotide) in the insect larvae suggests the production of CKs by the insect itself, rather than transport from the leaf to the insect. This is because CK nucleotides are precursor forms to the more active riboside and free base forms of cytokinin. The phenomenon of CK-production by insects has been previously suggested by several studies, which were done using different insect/herbivore complexes from the present study (Engelbrecht et al. Citation1969; Mapes and Davies Citation2001; Giron et al. Citation2007; Dorchin et al. 2009). Our concentrations were similar to those reported by Mapes and Davies (Citation2001), but we found far higher concentrations of iPR, and slightly lower concentrations of iP (). These differences may reflect variation in gall-formation strategies between different taxa of insects, or might reflect changing hormone concentrations throughout different stages of gall-development. Mapes and Davies (Citation2001) reported that the relative proportions of phytohormones were different in larvae collected early and late in gall-development, and suggested that changing hormone ratios might be important stimuli controlling the course of gall-formation. Dorchin et al. (Citation2009) later demonstrated that morphology of galls could be dependent on the dosage of CKs by measuring differences between CK profiles of male and female wasps. They also suggested that larval insects were acting as the source of iP-type CKs, and noted the potential involvement of additional phytohormones (in their case auxin).
Contrary to our predictions, ABA was present and most highly concentrated in insects, somewhat concentrated in leaves, and least concentrated in galls. We therefore concluded that ABA was not involved solely as part of an anti-herbivore response initiated by the plant. To our knowledge this is the first record of ABA in insect tissue, and thus there are no comparisons to make with other studies. Interestingly, ABA followed a similar pattern to that of the more highly concentrated CKs, which were most concentrated in insects, moderately concentrated in leaves, and least concentrated in galls. Since the galls had lower concentrations of ABA than either insects or leaves, it seems possible that both the insect and the plant may have been manipulating ABA. This concept confirms a statement by Mapes and Davies (Citation2001), who noted that galls could not be fully developed simply by injecting the most abundant CK into a partially developed gall. In one of the few studies that report the production of ABA in organisms other than plants, the fungus Cercospora beticola was discovered to be using ABA to invade its host by suppressing chemical pathways responsible for inducible defence in sugar beet, Beta vulgaris (Schmidt et al. Citation2008). A similar effect has been noticed in the suppression of salicylic-acid pathways in tomato, Solanum lycopersicum (Audenaert et al. Citation2002). This suggests a possible alternative hypothesis concerning the role of ABA in gall-formation, in which case it would be advantageous for the invading insect to manipulate ABA. Another possible explanation for the high concentrations of ABA found in the insects might be that ABA helps to retain water within the gall by playing its known roles in osmoregulation and stomatal closure (Wilkinson and Davies Citation2002). This could also be important for preventing the soft-bodied larvae from drying out, especially late in the season when leaves begin to fall, and a regular supply of water from the plant to the larvae may be cut off. Again, this may be linked to the appearance of green islands where resources are concentrated and photosynthesis is maintained by high cytokinin activity (Walters et al. Citation2008). ABA might also be used by the insect in the early stages of gall development to communicate with its host plant, possibly delivering a false message allowing it to pose as a beneficial symbiont, such as a rhizobium or micorrhiza (although such interactions are extremely complex, and also involve phytohormones other than CK and ABA) (Hartley Citation1999; Herrera-Medina et al. Citation2007).
Although CKs have been found in many animals as well as plants (Schultz 2002), relatively high concentrations of phytohormones in gall-forming insects could result from several different scenarios: first, the insect could be assimilating hormones from the plant, and hyper-concentrating them within its body (Van Staden and Davey Citation1978). This could lead to the formation and maintenance of galls caused by increased cell growth and development associated with CK activity. This scenario seems to be unlikely for several reasons. First, the insects are extremely small, and would have to filter many times their mass of plant tissue to accumulate the extremely high concentrations of cytokinins recovered. For example, a larval insect with a mass of approximately 0.00236 g would be required to consume three leaves, each with an approximate mass of 0.120 g, in order to acquire the concentration of iPR we found per insect; this would be the equivalent of the insect consuming 153 times their body mass in leaf tissue. Second, the presence of nucleotide forms of CK in the insect suggests the production of CKs in situ rather than their importation.
A second possible scenario is that the insects themselves are producing the phytohormones. The presence of high concentrations of CKs in the insect (more than presumed feasible through phloem digestion) supports this scenario (Inbar et al. Citation1995; Dorchin et al. 2009). Additionally, the insect tissue contains forms of CK that are consistent with the occurrence of an iP-type CK metabolic pathway (), thus suggesting a potential pathway for the production of CKs in the insect tissue from start to finish.
In terms of the ecological implications of this plant-herbivore system, it is difficult to assess the effects of gall-formation on hackberry. Although insecticides have been employed on many occasions in attempts to control hackberry Psyllids (Hodkinson Citation1988), the justification for this course of action is unclear. Nakamura and Ohgushi (Citation2007) found that gall-formation actually had a positive effect on shoot-growth and budding in willow (Salix spp). Unfortunately, no studies could be found discussing typical levels of endogenous ABA and CK in hackberry trees. Although follow-up work comparing the levels of phytohormones from this study to levels of endogenous phytohormones in uninfected trees would be useful, in practice it might be difficult to locate appropriate trees. During October 2008, 25 hackberry trees were inspected in Ottawa and Peterborough, Ontario, and all appeared to be heavily infested with similar galls. Both hackberry and P. celtidis are native to North America (Hodkinson Citation1988), suggesting that they have co-evolved over long periods of time, and extreme exploitation of the host by the insect is unlikely to be the result of an ecophysiological mismatch arising from a recent invasion.
Conclusion
The galls formed by P. celtidis on hackberry provide an intriguing system in which to study source-sink relations in plants, and represent a novel case in which high concentrations of the phytohormone ABA were isolated from insects. Only further study will reveal whether this is a rare occurrence in nature, or a phenomenon that is widespread among gall-forming insects. Not only do the insects in this system contain extremely high levels of CKs and unexpected concentrations of ABA, but patterns seem to indicate the existence of a CK metabolic pathway in the insect. Additional research is certainly necessary, and these patterns likely will not be explained by studying either the insect or the plant in isolation. In short, it appears that gall-formation by P. celtidis may be more analogous to ‘hacking into’ the phytohormone system of hackberry, than waging physical warfare or engaging in phylogenetic espionage. The formation of galls is likely more than just the sum of its chemical constituents and the results appear to be subtle, effective, and extremely complex.
Acknowledgments
This work was funded by the Natural Sciences and Engineering Council of Canada and Trent University. The authors would also like to thank the anonymous reviewers for the helpful recommendations that were used to improve the manuscript.
References
- Armstrong , DJ . 1994 . “ Cytokinin oxidase and the regulation of cytokinin degradation ” . In Cytokinins: Chemistry, activity, and function , Edited by: Mok , DWS and Mok , MC . 139 – 154 . Boca Raton, FL : CRC Press .
- Audenaert , K , De Meyer , GB and Hoefte , MM . 2002 . Abscisic acid determines basal susceptibility of tomato to Botrytis cinerea and suppresses salicylic acid dependent signalling mechanisms . Plant Physiol. , 128 : 491 – 501 .
- Baud , S , Dubreucq , B , Miquel , M , Rochat , C and Lepiniec , L . 2008 . Storage reserve accumulation in Arabidopsis: Metabolic and developmental control of seed filling . The Arabidopsis Book. , 63 : 1 – 24 .
- Berenbaum M , Feeny P. 1981 . Toxicity of angular furanocoumarins to swallowtail butterflies: Escalation in a coevolutionary arms race Science . 212 : 927 929 .
- Dobrev , PI and Kamínek , M . 2002 . Fast and efficient separation of cytokinins from auxin and abscisic acid and their purification using mixed-mode solid-phase extraction . J Chromatogr A. , 950 : 21 – 29 .
- Dorchin , N , Hoffmann , JH , Stirk , W , Novák , O , Strnad , M and Van Staden , J . 2009 . Sexually dimorphic gall structures correspond to differential phytohormone contents in male and female wasp larvae . Physiol Entomol. , 34 : 359 – 369 .
- Ehrlich PR Raven PH. 1964 . Butterflies and plants: A study in coevolution . Evolution . 18 : 586 608 .
- Elzen , GW . 1983 . Cytokinins and insect galls . Comp Biochem Physiol A. , 76 : 17 – 19 .
- Engelbrecht , L , Orban , U and Heese , W . 1969 . Leaf miner caterpillars and cytokinins in the green islands of autumn leaves . Nature. , 223 : 319 – 321 .
- Giron , D , Kaiser , W , Imbault , N and Casas , J . 2007 . Cytokinin-mediated leaf manipulation by a leafminer caterpillar . Biol Lett. , 3 : 340 – 343 .
- Hartley , SA . 1999 . Are gall insects large rhizobia? . Oikos. , 84 : 332 – 342 .
- Herrera-Medina , MJ , Steinkellner , S , Vierheilig , H , Ocampo Bote , JA and Garcia Garrido , JM . 2007 . Abscisic acid determines arbuscule development and functionality in the tomato arbuscular mycorrhiza . New Phytol. , 175 : 554 – 564 .
- Hodkinson , I . 1988 . The Nearctic Psylloidea (Insecta: Homoptera): An annotated check list . J Nat Hist. , 22 : 1179 – 1243 .
- Inbar , M , Eshel , A and Wool , D . 1995 . Interspecific competition among phloem-feeding insects mediated by induced host-plant sinks . Ecology. , 76 : 1506 – 1515 .
- Kamínek , M , Březinová , A , Gaudinová , A , Motyka , V , Vanková , R and Zažíá , E . 2000 . Purine cytokinins: A proposal of abbreviations . Plant Growth Reg. , 32 : 253 – 256 .
- Kasahara , H , Takei , K , Ueda , N , Hishiyama , S , Yamaya , T , Kamiya , Y , Yamaguchi , S and Sakakibara , H . 2004 . Distinct isoprenoid origins of cis- and trans-zeatin biosynthesis in Arabidopsis . J Biol Chem. , 279 : 4049 – 4054 .
- Lewis , IF and Walton , L . 1964 . Gall-formation on leaves of Celtis occidentalis L. resulting from material injected by Pachypsylla sp . Trans Am Microsc Soc. , 83 : 62 – 78 .
- Mapes , CC and Davies , PJ . 2001 . Cytokinins in the ball gall of Solidago altissima and in the gall forming larvae of Eurosta solidaginis . New Phytol. , 151 : 203 – 212 .
- Nakamura , M and Ohgushi , T . 2007 . Willow regrowth after galling increases bud production through increased shoot survival . Environ Entomol. , 36 : 618 – 622 .
- Quesnelle , P and Emery , RJN . 2007 . Cis-cytokinins that predominate in Pisum sativum during early embryogenesis will accelerate embryo growth in vitro . Can J Bot. , 85 : 91 – 103 .
- Radchuk , R , Radchuk , V , Götz , K , Weichert , H , Richter , A , Emery , RJN , Weschke , W and Weber , H . 2007 . Ectopic expression of phosphoenolpyruvate carboxylase in Vicia narbonensis seeds: Effects of improved nutrient status on seed maturation and transcriptional regulatory networks . The Plant J. , 51 : 819 – 839 .
- Raman , A . 2007 . Insect-induced plant galls of India: Unresolved questions . Curr Sci. , 92 : 748 – 757 .
- Sakakibara , H . 2006 . Cytokinins: Activity, biosynthesis, and translocation . Annu Rev Plant Biol. , 57 : 431 – 449 .
- Schmidt , K , Pflugmacher , M , Klages , S , Maeser , A , Mock , A and Stahl , D . 2008 . Accumulation of the hormone abscisic acid (ABA) at the infection site of the fungus Cercospora beticola supports the role of ABA as a repressor of plant defence in sugar beet . Mol Plant Pathol. , 9 : 661 – 673 .
- Schultz , JC . 2002 . Shared signals and the potential for phylogenetic espionage between plants and animals . Integr Comp Biol. , 42 : 454 – 462 .
- Thaler , JS and Bostock , RM . 2004 . Interactions between abscisic-acid-mediated responses and plant resistance to pathogens and insects . Ecology. , 85 : 48 – 58 .
- Tooker , JF , Rohr , JR , Abrahamson , WG and De Moraes , CM . 2008 . Gall insects can avoid and alter indirect plant defences . New Phytol. , 178 : 657 – 671 .
- Van Onckelen , H , Rudelsheim , P , Hermans , R , Horemans , S , Messens , E , Hernalsteens , JP , van Montagu , M and De Greef , J . 1984 . Kinetics of endogenous cytokinin, IAA and ABA levels in relation to the growth and morphology of tobacco crown gall tissue . Plant Cell Physiol. , 25 : 1017 – 1025 .
- Van Staden , J and Davey , JE . 1978 . Endogenous cytokinins in the laminae and galls of Erythrina latissima leaves . Botan Gaz. , 139 : 36 – 41 .
- Walters , DR , McRoberts , N and Fitt , BDL . 2008 . Are green islands red herrings? Significance of greenislands in plant interactions with pathogens and pests . Biol Rev. , 83 : 79 – 102 .
- Wells , BW . 1920 . Early stages in the development of certain Pachypsylla galls on Celtis . Am J Bot. , 7 : 275 – 285 .
- Wilkinson , S and Davies , WJ . 2002 . ABA-based chemical signalling: The co-ordination of responses to stress in plants . Plant Cell Environ. , 25 : 195 – 210 .
- Zhang , J , Jia , W , Yang , J and Ismail , AM . 2006 . Role of ABA in integrating plant responses to drought and salt stresses . Field Crops Res. , 97 : 111 – 119 .