Abstract
The present study was carried out to investigate the effect of plant growth promoting thermotolerant Pseudomonas putida strain AKMP7 on the growth of wheat plants to heat stress. The results indicated the superior performance by P. putida strain AKMP7 in improving survival and growth of wheat plants under heat stress. The bacterium significantly increased the root and shoot length, dry biomass, tiller, spike let and grain formation of wheat over uninoculated plants. Inoculation reduced membrane injury and the activity of several antioxidant enzymes such as SOD, APX and CAT under heat stress. Inoculation improved the levels of cellular metabolites like proline, chlorophyll, sugars, starch, amino acids, and proteins compared to uninoculated plants. Scanning electron microscopy studies confirmed the colonization of the organism on the root surface. This result suggests the possible role of microorganisms in mitigating adverse effects of climate changes on crop growth and may lead to development of microbe based climate- ready technology.
Introduction
Gaseous emissions due to human activities are substantially adding to the existing concentrations of greenhouse gases, particularly CO2, methane, chlorofluorocarbons and nitrous oxides. Future increase in greenhouse gases could raise the Earth's surface temperature anywhere between 1.5 and 11°C by 2100 (Stainforth et al. Citation2005) that would severely reduce crop production. Rising temperatures may lead to altered geographical distribution and growing seasons of agricultural crops by allowing the threshold temperature for the start of the season and crop maturity to be reached earlier (Porter Citation2005).
Heat stress is a common constraint during anthesis and grain filling stages in many cereal crops of temperate regions. Wheat (Triticum spp.) is the major Rabi crop in India and is sensitive to various biotic and abiotic stresses such as weather and inter-seasonal climatic variability (in terms of changes in temperature, rainfall, radiation). An increase in mean seasonal temperature of 2–4°C reduces the yield of wheat (Wheeler et al. Citation1996; Batts et al. Citation1997) grown in well-watered conditions. The optimum post anthesis temperature for maximum kernel weight in wheat is about 15°C (Chowdhury and Wardlaw Citation1978), and each 1°C rise in temperature above the optimum can cause 3–5% reduction in single grain weight under both controlled environments (Wardlaw et al. Citation1989) and field conditions (Wiegand and Cuellar Citation1981). Due to the unprecedented heat wave in northern India during the year 2004, 4.4 million tons of loss in productivity was reported in wheat crop (Samra and Sing Citation2004). Heat stress also affects protein denaturation and aggregation, fluidity of membrane lipids, inactivation of enzymes in chloroplast and mitochondria, inhibition of protein synthesis and loss of membrane integrity (Howarth Citation2005). These injuries eventually lead to starvation, inhibition of growth, reduced ion flux, production of toxic compounds and reactive oxygen species (ROS) (Schöffl et al. Citation1999; Howarth Citation2005). Breeding for suitable varieties, improved crop management and changes in planting dates, etc., to some extent would help in overcoming the effects of climate change (Vanaja et al. Citation2007). However, evolving low- cost methods which can be easily adopted by small farmers is a major challenge.
Plant growth promoting rhizobacteria (PGPR) are one class of beneficial bacteria inhabiting the soil ecosystem (Kloepper et al. Citation1989). PGPR are found in association with roots of many different plants. The effects of PGPR on plant growth can be mediated by direct or indirect mechanisms (Glick Citation1995). The direct effects have been most commonly attributed to the production of plant hormones such as auxins, gibberellins and cytokinins, biological nitrogen fixation and solubilization of inorganic phosphorous, etc. Indirect mechanisms include suppression of phyto-pathogens by the production of siderophores, HCN, ammonia, antibiotics, volatile metabolites, etc., by inducing systemic resistance and/or by competing with the pathogen for nutrients or for colonization space (Glick Citation1995). A particular bacterium may affect plant growth and development using any one or more of these mechanisms (Montesinos Citation2003). During the last couple of decades the use of PGPR for sustainable agriculture has increased tremendously in various parts of the world to reduce the need for chemical fertilizers. Recent reports suggest that PGPR also enhance the tolerance of plants to abiotic stresses such as drought (Timmusk and Wagner Citation1999; Sandhya et al. Citation2009), chilling injury (Ait Barka et al. 2006), salinity (Han and Lee Citation2005), metal toxicity (Dell’ Amico et al. 2008) and elevated temperature stress (Ali et al. Citation2009). The aim of the present study was to investigate the effect of inoculation with a thermotolerant plant growth promoting Pseudomonas putida strain AKMP7 on the growth of wheat plants to heat stress.
Materials and Methods
Bacterial strain
Thermotolerant Pseudomonas putida strain AKMP7 used in this study was isolated from the sorghum rhizosphere soil sample collected from Akola region Maharastra, India. Strain AKMP7 possessed plant growth promoting traits such as IAA (61.9 µg mg−1 protein under ambient (28°C) and 32 µg mg−1 protein under high temperature (50°C), GA (0.43 µg mg−1 protein, ambient and 0.18 µg mg−1 protein, under high temperature), P-solubilization (59 µg ml−1, ambient and 38.7 µg ml−1 under high temperature), siderophore, HCN and ammonia positive both under ambient and under high temperature. Molecular characterization of the strain was done and the 16S rDNA sequence was submitted to GenBank under the accession no. GU396282.
Plant growth and inoculation in pots
The study of the effect of bacterial strain AKMP7 on alleviation of heat stress in wheat (var. LOK-1) was carried out under sterile soil conditions with a temperature of 37°C to 40°C during day and 27°C to 30°C at night. Seedlings received 16/8 h light/dark cycle (350 µmol m−2 s−1 light intensity). The bacterial inoculum was produced by transferring one loop full of strain AKMP7 to 100 ml of King's B liquid medium in a 250 ml Erlenmeyer flask incubated at 28°C on a rotary shaker for 24 h. Bacteria were collected by centrifugation (5000 rpm for 5 min) and washed twice with sterile normal saline. The pellet was resuspended in saline and used for estimation of bacterial concentration by UV-spectrophotometry (600 nm). The bacterial concentration was adjusted to109 CFU/ml with sterile normal saline. The concentration was confirmed by plate counting and used for talc-based formulation containing 1% carboxy methylcellulose as adhesive.
Seeds of wheat were surface sterilized with 0.1% (w/v) HgCl2 and 70% (v/v) ethanol and coated with talc based formulation of P. putida strain AKMP7, and the bacterial population on coated seeds was determined by serial dilution plate count (108 CFU/seed) method using King's B solid medium. The seeds (six seeds per pot) were sown in plastic pots (30 cm diameter and 31.5 cm deep) with a capacity to hold 15 kg of soil. Soil was collected from homogeneous horizon (0–20 cm) of Gunegal Research Farm (GRF), CRIDA in south India falling under semi-arid region in rain-fed production system. The soil was air-dried and sieved (< 2mm) before being analyzed for the physicochemical properties. The soil contained 74% sand, 5 silt, and 24 clay with 1.40 Mg m−3 bulk density, 36.9% total porosity and 37.9% water holding capacity; it had pH 7.0 and electrical conductivity of 0.103 ms. Organic C, total N and total P content of soil were 0.82 g kg−1, 0.16 g kg−1, and 0.07 g kg−1, respectively. For preparation of sterile soil, field soil was autoclaved twice for 20 min at 120°C with a 24 h interval. The treatments included seed bacterization with and without P. putida strain AKMP7. Two weeks after initial emergence, plants were thinned to one per pot. The pots were watered (sterile water) at 09.30 h daily by weighing the individual pots and supplying each pot a given amount of water that had been pre-calculated based on the water content of dried soil and its water holding capacity. The daily watering brought the water content of the pots to that of the field capacity, allowing the plants to grow under conditions free from water stress. Pots (inoculated and uninoculated) were replicated six times in randomized block method.
Plant biochemical parameters
In order to study the mechanism of protection of wheat plants exposed to heat stress by P. putida strain AKMP7, 95-days-old seedlings were harvested and the contents of total sugars, amino acids, chlorophyll, proline, starch and protein content of the seedlings were determined. The contents of sugars and amino acids were determined by incubating 1 g of leaf sample with methanol: chloroform: water (60:25:15 v/v) mixture at 60°C for 2 h. The samples were centrifuged 10,000 rpm for 15 min at 4°C, and the content of total sugars of the supernatant was estimated by phenol sulfuric acid method (Dubois et al. Citation1956). The content of total amino acids was determined by heating 1 ml of the supernatant with 1 ml of 0.1 M acetate buffer and 1 ml of ninhydrin (5% w/v) at 95°C for 5 min. The samples were cooled and absorbance was read at 570 nm (Chen et al. Citation2007). Free proline content was determined by the method of Bates et al. (Citation1973). The leaf samples homogenized in 3% (w/v) sulphosalicylic acid were centrifuged at 10,000 rpm for 15 min at 4°C, and the supernatant was heated at 100°C after the addition of acidic ninhydrin. The samples were extracted with toluene and the chromophore containing toluene was aspirated and cooled to room temperature, and absorbance was read at 520 nm. The determination of total chlorophyll was done by immersing leaf samples in dimethyl sulphoxide (DMSO) and incubating them at 70°C for 4 h. The absorbance of the solution was read at 645, 663 and 480 nm (Barnes et al. Citation1992). The membrane injury index (MII) of leaf tissues was determined by recording electrolyte leakage in double distilled water at 50°C and 121°C (Ibrahim and Quick Citation2001). Leaf samples (0.1 g) were cut into discs of uniform size and submerged in 10 ml of deionized water in test tubes and heated at 50°C for 30 min. The tubes were incubated overnight at room temperature, and the conductance was measured using a conductivity meter. The tubes were then autoclaved for 10 min at 121°C, and the conductance was measured again. Starch analysis was determined by homogenizing leaf samples in 0.1 M phosphate buffer (pH 7.5) at 4°C and centrifuged 10,000 rpm for 15 min at 4°C. The pellet was suspended in DMSO: 8M HCl (4:1 v/v) and agitated at 60°C for 30 min. After centrifugation at 10,000 rpm for 15 min at 4°C the supernatant was treated with 100 µl of acidified iodine, incubated at room temp for 15 min and the absorbance was read at 600 nm (Ait Barka et al. 2006). The protein content was determined by grinding 0.1 g of leaf issue in phosphate buffer (pH 7.0) and estimated by Bradford method (Bradford Citation1976).
Antioxidant enzyme activities
Antioxidant enzymes were estimated after 95 days of germination. Enzyme extracts for superoxide dismutase (SOD, EC 1.15.1.1) and catalase (CAT, EC 1.11.1.6) were prepared by grinding wheat leaves (1g fresh mass) in a pre-chilled mortar and pestle first with liquid nitrogen and then with 10 ml of extraction buffer consisting of 100 mM potassium phosphate buffer, pH 7.5 containing 0.5 mM EDTA. For the estimation of ascorbate peroxidase (APX, EC 1.11.1.11), extraction buffer was further supplemented with 1 mM ascorbic acid and pH was adjusted to 7.5. Extracts were then centrifuged at 15,000 rpm for 20 min at 4°C, and the supernatants were analyzed. Enzyme assays were conducted immediately following extraction.
SOD activity was determined according to method described earlier (Dhindsa et al. Citation1981). The reaction mixture contained 13 mM methionine, 25 mM nitro-blue tetrazolium chloride (NBT), 0.1 mM EDTA, 50 mM phosphate buffer (pH 7.8), 50 mM sodium carbonate and 0.1 ml enzyme. The activity was determined by adding 2 mM riboflavin and placing the tubes under two 15 W fluorescent lamps for 15 min. A complete reaction mixture without enzyme, which gave the maximal color, served as control. Reaction was stopped by switching off the light and putting the tubes into dark. A non-irradiated complete reaction mixture served as a blank. The absorbance was recorded at 560 nm, and one unit of enzyme activity was taken as that amount of enzyme, which reduced the absorbance reading to 50% in comparison with tubes lacking enzyme.
APX was estimated by observing the decrease in absorbance due to ascorbic acid at 290 nm (Nakano and Asada Citation1981). The assay mixture contained 50 mM potassium phosphate buffer (pH 7.0), 0.5 mM ascorbic acid, 0.1 mM EDTA, 0.1 mM H2O2, and 0.1 ml enzyme. The reaction was started with the addition of 0.1 mM hydrogen peroxide. Decrease in absorbance for a period of 30 seconds was measured at 290 nm in a UV–visible spectrophotometer. Activity is expressed by calculating the decrease in ascorbic acid content by comparing with a standard curve drawn with known concentrations of ascorbic acid.
CAT activity was determined according to method described by Teranishi et al. (Citation1974). The reaction mixture consisted of 6 mM hydrogen peroxidase, 0.1 M phosphate buffer pH 7.0, and reaction started by adding 50 ml of enzyme extract. Reaction was terminated after 5 min by adding 4 ml of titanium reagent (prepared by digestion 1 g titanium dioxide with 10 g potassium sulphate and 150 ml of concentrated H2SO4). Aliquot was centrifuged at 15,000 rpm for 10 min at 4°C, and absorbance of the supernatant was recorded at 415 nm. Reaction mixture without enzyme served as blank. Catalase activity was assayed by monitoring the disappearance of H2O2 at 415 nm. One unit of catalase decomposed 1 µmol of H2O2 per milligram of protein at pH 7.0 and 25°C in 1 min.
Crop yield
Crop was harvested after 110 days, and shoots and roots were separated and dried overnight at 105°C before determining the root and shoot dry weight. Yield content was determined by root and shoot length, tillers and spikes count, spike length, grains per spike and 100 seed weight.
Root colonization studies
Bacterial colonization of root from bacterized and non-bacterized plants under heat stress was determined by plating root samples on to King's B medium amended with rifampicin, (50 µg ml−1) and also by scanning electron microscope (SEM). The root samples were fixed in 2.5% gluteraldehyde in 0.05 M phosphate buffer (pH 7.2) for 24 h at 4°C and post fixed with 2% aqueous osmium tetroxide in the same buffer for 2h. The post fixation samples were dehydrated in series with graded alcohol and dried to critical point, which was checked by drying with Electron Microscopy Science CPD unit. The dried samples were mounted over the stubs with double-sided conductivity tape, and a thin layer of gold metal was applied over the sample using an automated sputter coater (JEOL JFC-1600) for about 3 min (Bozzola and Russell Citation1999). Finally samples were analyzed by scanning electron microscopy (Model: JOEL-JSM 5600, JAPAN) at various magnifications at RUSKA Lab, SVUU, Hyderabad, India.
Statistical analyses
All data were analyzed statistically by analysis of variance. Plant studies were tested in an experiment using a randomized complete block design with six replications. Mean comparison among treatments was done by Tukey's multiple comparisons.
Results
Growth promotion of wheat by bacterial inoculation
Bacterial inoculation affected the early plant growth and biochemical parameters of wheat (var. LOK-1) grown under heat stress. The inoculation of wheat with thermotolerant P. putida strain AKMP7 significantly increased the shoot (1.12 fold increase, 52.93 cm) and root length (1.19 fold increase, 27.7 cm) over uninoculated (shoot, 47.12 cm and root, 23.13 cm) plants; similarly significant increase in dry biomass of inoculated (16.8 g/plant) plants over uninoculated control (10.5 g/plant) was recorded (1.6 fold) (a, b). Rhizosphere colonization by the inoculated strain was studied by plating root samples on King's B medium containing antibiotic (50 µg ml–1 of rifampicin). After two days of incubation, no colonies appeared on King's B solid medium plate with the root samples of uninoculated plants. However, vigorous growth was observed around the roots of inoculated plants. Scanning electron microscopy of root samples of inoculated plants revealed the presence of dense bacterial population, and the bacterial cells attached perpendicularly and horizontally to the root surface of plants ().
Figure 1. Shoot, root length, and dry biomass of wheat plants inoculated with P. putida strain AKMP7 grown under heat stress (UIN = uninoculated; INC = inoculated). (a) root and shoot length (cm); (b) dry mass (g/plant).Values are means of six readings with ± SE. Values with different letters are significantly different at p <0.05.
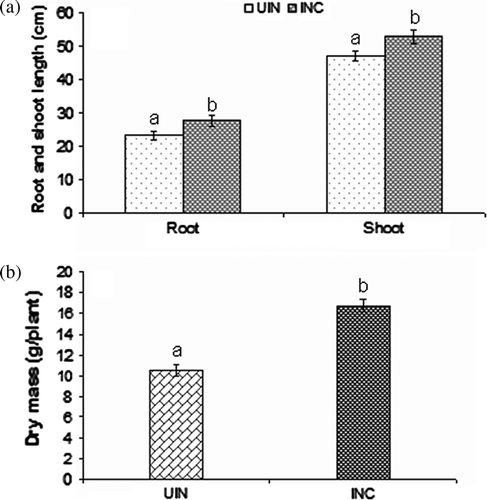
Biochemical parameters
Inoculation with P. putida strain AKMP7 significantly enhanced biochemical parameters of wheat plants compared to uninoculated plants. Inoculation significantly enhanced chlorophyll, total sugars, proline, starch, amino acids and protein content under heat stress (). As an indication of plant health, the leaf chlorophyll content of inoculated and uninoculated plants grown under heat stress was determined. In the presence of heat stress a significant decrease in chlorophyll content of uninoculated plants was observed, whereas inoculation with P. putida strain AKMP7 counteracted the adverse effect of heat stress on leaf chlorophyll (1.46 fold increase, 29.35 mg g-1 FW) compared to uninoculated plants (20.02 mg g−1 FW). The enhanced photosynthetic activity of the inoculated plants corresponded with significantly higher starch content (1.51 fold, 26.47 mg g−1 FW) over uninoculated plants (17.47 mg g-1 FW). A significant decrease in the protein (80.79 mg g−1 DW) and sugar (39.59 mg g-1 DW) content of uninoculated plants was observed under heat stress, whereas the protein and sugar (1.16 fold increase, 94.26 mg g-1 DW and 1.58 fold increase, 62.93 mg g-1 DW) content of inoculated plants significantly increased under heat stress respectively (). Inoculation also enhanced leaf amino acid (1.68 fold, 38.75 µmol g−1 DW) and proline (1.23 fold, 22.08 µmol g−1 FW) content compared to the values of the uninoculated plants (amino acids, 23.04 µmol g−1 DW and proline, 17.82 µmol g−1 FW) under heat stress. Membrane injury index as determined by electrolyte leakage showed that bacterial inoculation significantly improved the heat tolerance of the wheat plants and reduced the membrane injury over uninoculated plants ().
Table 1 Effect of inoculation with P. putida strain AKMP7 on biochemical parameters of wheat plants under heat stress.
Antioxidant enzyme activity
Inoculation with P. putida strain AKMP7 counteracted the adverse effect of heat on leaf antioxidant enzymes by lowering the ROS generation compared to uninoculated plants. Under heat stress, the percent decrease in enzyme activity of strain AKMP7 seedlings is higher (38%, 8.16 Units min-1 mg-1 protein SOD; 15.82%, 8.62 µmol Ascorbate oxidized min-1 mg-1 protein APX and 23.68%, 6.96 µmol H2O2 min−1 mg−1 protein CAT) than uninoculated plants respectively (13.35 Units min−1 mg−1 protein SOD, 10.24 µmol Ascorbate oxidized min−1 mg−1 protein APX and 9.12 µmol H2O2 min−1 mg−1 protein CAT) (a, b, c).
Figure 3. Effect of inoculation with a thermotolerant P. putida strain AKMP7 on superoxide dismutase (a) ascorbate peroxidase, (b) and catalase, and (c) of wheat grown under heat stress (UIN = uninoculated; INC = inoculated). Values are means of six readings with ± SE. Values with different letters are significantly different at p <0.05.
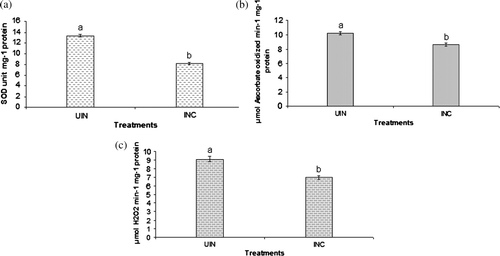
Crop yield
Inoculation with P. putida strain AKMP7 significantly increased the yield- attributing characters such as tiller and spikelet formation, spike length, grain formation per spike and 100 seed weight compared to uninoculated plants under heat stress. P. putida strain AKMP7 treated plants recorded higher number of tiller and spikelet formation per plant (8.1±0.07 each), increased spike length (6.92±0.061), enhanced grain formation (28.3±1.52) per spike compared to uninoculated plants. Heat stress decreased the 100 seed weight (2.89±0.62) of uninoculated plants whereas; P. putida strain AKMP7 inoculation enhanced 100 seed weight (5.67±0.96) ().
Table 2 Effect of inoculation with P. putida strain AKMP7 on yield of wheat under heat stress.
Discussion
Pseudomonas spp. and other rhizobacteria are well known for their ability to colonize the root tissues of wide crop plants and promote the plant growth by the production of phytohormones, antagonistic substances and enzymes (Hong et al. Citation1991). It has been suggested that bacterial inoculation alleviated abiotic stresses like drought, chilling, salinity, metal toxicity and elevated temperature (Timmusk and Wagner Citation1999; Redman et al. Citation2002; Han and Lee Citation2005; Ait Barka et al. Citation2006; Marquez et al. Citation2007; Dell’ Amico et al. Citation2008; Ali et al. Citation2009; Sandhya et al. Citation2009). Indeed, this concept is supported by our results where bacterial inoculation mitigated the adverse effect of heat stress in wheat plants. Despite the ability of plants to adapt partially to temperature stress in temperate climates, plant growth and overall productivity generally decline under stress (Haldiman Citation1998). The extent of a plant's ability to withstand such stress is determined by metabolic alterations (Seki et al. Citation2003). In the present study, we demonstrated that plant growth promoting thermotolerant P. putida strain AKMP7 colonizing wheat roots can significantly influence the plant's resistance to heat stress by influencing its metabolism. Seed inoculation with strain AKMP7 had a pronounced effect on growth, development and response to heat stress.
According to Höflich et al. (Citation1994) and Höflich and Kühn (Citation1996), Pseudomonas spp., Rhizobium spp. and Agrobacterium spp. isolated from sites at a semi-continental climate promoted the growth of young plants and increased the yields of graminae and legumes under the respective conditions. Javed and Arshad (Citation1997) also isolated bacteria, which stimulated the plant growth of wheat and rice in warm climates. We have isolated the plant growth promoting thermotolerant P. putida strain AKMP7 from the rhizosphere of sorghum plants grown under semi arid conditions, characterized by high temperature values. The strain AKMP7 could grow and survive at 50°C, which was the maximum survival temperature for the strain and possessed plant growth promoting traits (IAA, GA, P-solubilization, siderophore, HCN and ammonia production).
In the present study, bacterial inoculation possessing plant growth promoting traits significantly increased root, shoot length and dry biomass of wheat (var. LOK-1) under heat stress. Plant growth is enhanced due to the production of plant growth regulators at the root interface by inoculated plant growth-promoting bacteria which in turn stimulates root development, resulting in better absorption of water and nutrients from soil (Kloepper et al. Citation1991; Zimmer et al. Citation1995; Höflich and Kühn Citation1996). Dell’ Amico et al. (Citation2008) showed that inoculation with cadmium-resistant strains Pseudomonas tolaasi and Pseudomonas fluorescens enabled Brassica napus to grow under cadmium stress because of the production of indole acetic acid (IAA), siderophores and ACC (1-aminocyclopropane-1-carboxylate) deaminase, which protected the plants against cadmium stress. The used thermotolerant Pseudomonas putida strain AKMP7 showed plant growth-promoting traits like production of phytohormones, HCN, ammonia, siderophore and P-solubilization, which are responsible for heat tolerance in wheat plants. It was also observed that total chlorophyll content increased due to inoculation with strain AKMP7. Glick et al. (Citation1997) also found that P. putida GR12-2 increased the chlorophyll content in the shoots of canola plant. The increase in chlorophyll content may also be the result of increased photosynthetic leaf area of plants due to inoculation compared to uninoculated plants where the leaf area was reduced due to heat stress (Marcelis and van Hooijdonk Citation1999).
Our results show that the differences in heat tolerance between the uninoculated and inoculated plants were reflected by differences in their abilities to significantly accumulate carbohydrates, amino acids and proteins under heat stress. This is in agreement with our earlier report of the accumulation of cellular metabolites in sorghum seedlings subjected to elevated temperature (Ali et al. Citation2009). Lower electrolyte leakage in inoculated plants suggested protection of membrane integrity by bacterium, probably due to alterations of plant lipid metabolism. Proline is a dominant organic molecule that accumulates in many organisms upon exposure to environmental stress (Delauney and Verma Citation1993) and plays multiple roles in plants’ adaptation to stress (Nanjo et al. Citation1999; Sung et al. Citation2003). In this study, P. putida strain AKMP7 significantly increased proline accumulation in wheat plants under heat stress compared to uninoculated plants. The accumulation of proline in leaves under heat stress is an adaptation mechanism as they bind to membranes, regulating membrane permeability in cells and influencing water movement among tissue (Ashraf and Foolad Citation2007). Bano and Fatima (Citation2009) observed that the salt tolerance in Zea mays inoculated with Rhizobium and Pseudomonas was mediated by the decrease in electrolyte leakage, increase in proline production and maintenance of water content of leaves with selective uptake of K+ ions. In the present study inoculation with P. putida strain AKMP7 in wheat plants lowered the levels of ROS. The OH- radicals formed by the combination of O2 - and H2O2 is highly toxic, which can damage chlorophyll, protein, DNA, lipids and other important macromolecules, thus affecting plant metabolism and limiting growth and yield (Sairam and Tyagi Citation2004). The expression of antioxidants such as SOD converts O2 - to H2O2, which is further removed by APX or CAT. Inoculation with thermotolerant P. putida strain AKMP7 lowered the levels of ROS, making the wheat plant survive under heat stress whereas, in uninoculated plants the levels of generated ROS are high under heat stress, resulting in severe damage to cellular components which leads to cell death. Inoculation with P. putida strain AKMP7 counteracted the adverse effect of heat stress on wheat plants and enhanced yield components such as tillers and spikes count, spike length, grains per spike and seed weight compared to uninoculated plants. As successful plant growth promoting inoculants, bacteria must be able to rapidly colonize the root system during the growth of plant (Defreitas and Germida Citation1992). Successful colonization of plant roots by the introduced strain suggested a close bacterial-plant association that may be beneficial to plants, probably because inoculation triggers some stress responsive mechanisms that enable the plants to tolerate high temperatures (Timmusk and Wagner Citation1999).
Conclusion
Inoculation with thermotolerant PGP P. putida strain AKMP7 could be an effective approach for alleviating heat stress and consequently improving the growth of wheat plant in the presence of heat stress. This finding suggests the possible role of microorganisms in mitigating adverse effects of climate changes on crop growth and may lead to development of microbial products to mitigate such effects. However, further studies are required under field conditions and the mechanism of protection has to be elucidated
Acknowledgements
The authors are grateful to the Indian Council of Agricultural Research (ICAR), New Delhi, for providing financial assistance in the form of network project on Application of Microorganisms in Agriculture and Allied Sectors (AMAAS).
References
- Ait Barka , E , Nowak , J and Clement , C. 2006 . Enhancement of chilling resistance of inoculated grapevine plantlets with a plant growth promoting rhizobacterium; Burkholderia phytofirmans strain Ps Jn . Appl Environ Microbiol. , 72 : 7246 – 7252 .
- Ali SKZ , Sandhya V , Minakshi Grover , Kishore N , Venkateswar Rao L , Venkateswarlu B. 2009 . Pseudomonas sp. strain AKM-P6 enhances tolerance of sorghum seedlings to elevated temperatures . Biol Fertil Soils . 46 : 45 – 55 .
- Ashraf , M and Foolad , MR. 2007 . Roles of glycine betaine and proline in improving plant abiotic stress resistance . Environ Exp Bot. , 59 : 206 – 216 .
- Bano , A and Fatima , M. 2009 . Salt tolerance in Zea mays (L) following inoculation with Rhizobium and Pseudomonas . Biol Fertil Soils. , 45 : 405 – 413 .
- Barnes , JD , Balaguer , L , Maurigue , E , Elvira , S and Davison , AW. 1992 . A reappraisal of the use of DMSO for the extraction and determination of chlorophyll ‘a’ and ‘b’ in lichens and higher plants . Environ Exp Bot. , 32 : 87 – 99 .
- Bates , LS , Waldren , RD and Teare , ID. 1973 . Rapid determination of free proline for water stress studies . Plant Soil. , 39 : 205 – 207 .
- Batts , GR , Morison , JIL , Ellis , RH , Hadley , P and Wheeler , TR. 1997 . Effects of CO2 and temperature on growth and yield of crops of winter wheat over four seasons . Eur J Agron. , 7 : 43 – 52 .
- Bozzola , JJ and Russell , LD. 1999 . Electron microscopy principles and techniques for biologists , 2nd ed , Sudbury, MA : Jones and Bartlett .
- Bradford , M. 1976 . A rapid and sensitive method for the quantitation of microgram quantities of protein utilizing the principle of protein-dye binding . Anal Biochem. , 72 : 248 – 258 .
- Chen , Z , Cuin , TA , Zhou , M , Twomey , A , Naidu , BP and Shabala , S. 2007 . Compatible solute accumulation and stress-mitigating effects in barley genotypes contrasting in their salt tolerance . J Exp Botany. , 58 : 4245 – 4255 .
- Chowdhury , SI and Wardlaw , IF. 1978 . The effect of temperature on kernel development in cereals . Aust J Agric Res. , 29 : 205 – 223 .
- Defreitas , JR and Germida , JJ. 1992 . Growth promotion of winter wheat fluorescent Pseudomonas under field conditions . Soil Biol Biochem. , 24 : 1137 – 1146 .
- Delauney , AJ and Verma , DPS. 1993 . Proline biosynthesis and osmoregulation in plants . Plant J. , 4 : 215 – 305 .
- Dell’ Amico , E , Cavalca , L and Andreoni , V. 2008 . Improvement of Brassica napus growth under cadmium stress by cadmium-resistance rhizobacteria . Soil Biol Biochem. , 40 : 74 – 84 .
- Dhindsa , RS , Plumb-Dhindsa , P and Throne , TA. 1981 . Leaf senescence: correlated with increased levels of membrane permeability and lipid peroxidation and decreased levels of superoxide dismutase and catalase . J Expt Bot. , 32 : 93 – 101 .
- Dubois , M , Gilles , KA , Hamilton , JK , Rebers , PA and Smith , F. 1956 . Colorimetric method for determination of sugars and related substances . Anal Chem. , 28 : 30 – 35 .
- Glick , BR. 1995 . The enhancement of plant growth by free living bacteria . Can J Microbiol. , 41 : 109 – 114 .
- Glick , BR , Liu , C , Ghosh , S and Dumbroff , EB. 1997 . Early development of canola seedlings in the presence of the plant growth promoting rhizobacterium Pseudomonas putida GR12-2 . Soil Biol Biochem. , 29 : 1233 – 1239 .
- Haldiman , P. 1998 . Low growth temperature induced changes to pigment composition and photosynthesis in Zea mays genotypes differing in chilling sensitivity . Plant Cell Environ. , 21 : 200 – 208 .
- Han , HS and Lee , KD. 2005 . Plant growth promoting rhizobacteria effect on antioxidant status, photosynthesis, mineral uptake and growth of lettuce under soil salinity . Res J Agric Biol Sci. , 1 : 210 – 215 .
- Höflich , G and Kühn , G. 1996 . Förderung das Wachstums und der Nährstoffaufnahme bei kurziferen Ö l- und Zwischenfruhten durch inokulierte Rhizospherenmikroorganismen . Zeischrift fü r Pflanzenernährung und Bodenkunde. , 159 : 575 – 578 .
- Höflich , G , Wiehe , W and Kühn , G. 1994 . Plant growth stimulation with symbiotic and associative rhizosphere microorganisms . Experientia. , 50 : 897 – 905 .
- Hong , Y , Glick , BR and Pasternak , JJ. 1991 . Plant-microbial interaction under gnotobiotic condition: a scanning microscope study . Curr Microbiol. , 23 : 111 – 114 .
- Howarth CJ. 2005 . Genetic improvements of tolerance to high temperature . In: M Ashraf , PJC Harris . Abiotic stresses: plant resistance through breeding and molecular approaches . New York : Howarth Press Inc ., p. 277 – 300 .
- Ibrahim , AMH and Quick , JS. 2001 . Genetic control of high temperature tolerance in wheat as measured by membrane thermal stability . Crop Sci. , 41 : 1405 – 1407 .
- Javed , M and Arshad , M. 1997 . Growth promotion of two wheat cultivars by plant growth promoting rhizobacteria . Pak J Bot. , 29 : 243 – 248 .
- Kloepper , JW , Lifshitz , R and Zablotowicz , RM. 1989 . Free-living bacterial inocula for enhancing crop productivity . Trends biotechnol. , 7 : 39 – 43 .
- Kloepper JW , Zablowicz RM , Tipping B , Lifshitz R. 1991 . Plant growth mediated by bacterial rhizosphere colonizers . In: DL Keister , B Gregan . The rhizosphere and plant growth, 14 BARC Symposium . Dordrecht, , The Netherlands : Kluwer Academic Publishers , p. 315 – 326 .
- Marcelis , LFM and Hooijdonk , HV. 1999 . Effect of salinity on growth, water use and nutrient use in radish (Raphanus sativus L.) . Plant and Soil. , 215 : 57 – 64 .
- Marquez , LM , Redman , RS , Rodrigues , RJ and Roosinck , J. 2007 . A virus in a fungus in a plant: three way symbiosis required for thermal tolerance . Science. , 315 : 513 – 515 .
- Montesinos , E. 2003 . Plant associated microorganisms: a view from the scope of microbiology . Int Microbiol. , 6 : 221 – 223 .
- Nakano , Y and Asada , K. 1981 . Hydrogen peroxide is scavenged by ascorbate specific peroxides in spinach chloroplast . Plant Cell Physiol. , 22 : 867 – 880 .
- Nanjo , T , Kobayashi , TM , Yoshida , Y , Kakubari , Y , Yamaguchi-Shinozaki , K and Shinozaki , K. 1999 . Antisense suppression of proline degradation improves tolerance to freezing and salinity in Arabidopsis thaliana . FEBS Lett. , 461 : 205 – 210 .
- Porter JR. 2005 . Rising temperatures are likely to reduce crop yields . Nature . 174 .
- Redman RS , Sheehan KB , Stout RG , Rodriguez RJ , Henson JM. 2002 . Thermotolerance generated by plant/fungal symbiosis . Science . 298 : 1581 .
- Sairam , RK and Tyagi , A. 2004 . Physiology and molecular biology of salinity stress tolerance in plants . Curr Sci. , 86 : 407 – 421 .
- Samra , JS and Sing , G. 2004 . Heat wave of March 2004: impact on agriculture natural resource management division , New Delhi : Indian council of Agricultural Research .
- Sandhya , V , Ali , SKZ , Minakshi , G , Gopal , R and Venkateswarlu , B. 2009 . Alleviation of drought stress effects in sunflower seedlings by the exopolysaccharides producing Pseudomonas putida strain GAP-P45 . Biol Fertil Soils. , 46 : 17 – 26 .
- Schöffl F , Prandl R , Reindl A. 1999 . Molecular responses to heat stress . In: K Shinozaki , K Yamaguchi-Shinozaki . Molecular responses to cold, drought, heat and salt stress in higher plants . Austin, TX : RG Landes , p. 81 – 98 .
- Seki , M , Kamei , A , Yamaguchi-Shinozaki , K and Shinozaki , K. 2003 . Molecular responses to drought, salinity and forst: common and different paths for plant protection . Curr Opin Biotechnol. , 14 : 194 – 199 .
- Stainforth , DA , Aina , T , Christensen , C , Collins , M , Faull , N , Frame , DJ , Kettleborough , JA , Knight Martin , SA , Murphy , JM , Piani , C , Sexton , D , Smith , LA , Spicer , RA , Thorpe , AJ and Allen , MR. 2005 . Uncertainty in predictions of the climate response to rising levels of greenhouse gases . Nature. , 433 : 403 – 406 .
- Sung , DY , Kaplan , F , Lee , KJ and Guy , CL. 2003 . Acquired tolerance to temperature extremes Trends . Plant Sci. , 8 : 179 – 187 .
- Teranishi , Y , Tanaka , A , Osumi , M and Fukui , S. 1974 . Catalase activity of hydrocarbon utilizing candida yeast . Agric Biol Chem. , 38 : 1213 – 1216 .
- Timmusk , S and Wagner , EGH. 1999 . The plant growth-promoting rhizobacterium Paenibacillus polymyxa induces changes in Arabidopsis thaliana gene expression: a possible connection between biotic and abiotic stress responses . Mol Plant-Microbe Inter. , 12 : 951 – 959 .
- Vanaja M , Ramakrishna YS , Rao GGSN , Rao KV , Subba Rao VM. 2007 . Climate change and dry land agriculture . Dryland Ecosystems: Indian Perspective . Jodhpur, , India : Central Arid zone Research Institute (CAZRI) and Arid Forest Research Institute (AFRI) .
- Wardlaw , IF , Dawson , IA , Munibi , P and Fewster , R. 1989 . The tolerance of wheat to high temperatures during reproductive growth. I. Survey procedures and general response patterns . Aust J Agric Res. , 40 : 1 – 13 .
- Wheeler , TR , Batts , GR , Ellis , RH , Hadley , P and Morison , JIL. 1996 . Growth and yield of winter wheat (Triticum aestivum) crops in response to CO2 and temperature. J Agri Sci . Cambridge , 127 : 37 – 48 .
- Wiegand , CL and Cuellar , JA. 1981 . Duration of grain filling and kernel weight of wheat as affected by temperature . Crop Sci. , 21 : 95 – 101 .
- Zimmer W , Kloos K , Hundeshagen B , Niederau E , Bothe H. 1995 . Auxin biosynthesis and denitrification in plant growth promoting bacteria . In: I Fendrik , M Del Gallo J Vanderleyden , M de Zamaroczy . Azospirillum VI and related microorganisms, genetics-physiology-ecology . NATO ASI series, series G: ecological sciences, vol G37 . Springer , Berlin Heidelberg, New York , p. 121 – 128 .