Abstract
In order to assess the role of the antioxidant defense system against salt treatment, the activities of some antioxidative enzymes and levels of some nonenzymatic antioxidants were estimated in Azolla caroliniana subjected to NaCl treatment (50 mM) for 10 days in absence or presence of nitrate. In A. caroliniana, salt treatment in absence of nitrate preferentially enhanced electrolyte leakage, lipid peroxidation, and H2O2 content. Also, the specific activitiy of guaiacol peroxidase (POX), glutathione reductase (GR), catalase (CAT), ascorbate peroxidase (APX), and superoxide dismutase (SOD) increased. In addition, reduced glutathione level increased and consequently, glutathione/oxidized glutathione (GSH/GSSG) ratio increased. Accumulation of Na+ increased significantly by salinity stress which resulted in a significant decrease in K+ accumulation, accordingly, K+/Na+ ratio decreased. Replacement of potassium chloride by potassium nitrate in nutrient solution under salt stress (50 mM NaCl) exhibited a reduction in electrolyte leakage, lipid peroxidation, and H2O2 contents. Conversely, the specific activity of APX, POX, GR, CAT, and SOD increased. The content of total ascorbate decreased, in contrast, reduced and GSSG increased and the ratio of GSH/GSSG increased 2.3-fold compared to the control value. Sodium ion accumulation was minimized in the presence of nitrate, potassium ion accumulation increased and as a result, K+/Na+ ratio increased when compared with the corresponding salinized plants. The differential changes in the specific activity of antioxidant enzymes due to NaCl treatment and nitrate may be useful as markers for recognizing salt tolerance in A. caroliniana.
Introduction
Abiotic stresses like salinity and drought limit plant growth and crop productivity (Owens Citation2001). They affect plants via different mechanisms, causing osmotic stress and ionic imbalance, in addition to inducing oxidative stress (Hasegawa et al. Citation2000). In higher plants, it is known that the generation of reactive oxygen species (ROS) can be enhanced by salinity and causes oxidative destruction of the cell components through oxidative damage of membrane lipids, nucleic acid, and proteins (Wang et al. Citation1998; Rios-Gonzalez et al. Citation2002). Overproduction of ROS caused by salinity usually leads to lipid peroxidation and induces K+ leakage from the cell by activating K+ efflux channels (Demidchik et al. Citation2003; Cuin and Shabala Citation2007). Malondialdehyde (MDA), a product of lipid peroxidation, showed greater accumulation in plants under stress condition (Chinta et al. Citation2001; Diego et al. Citation2003).
The ability of adaptation to osmotic stress is found in all living systems, from bacteria to higher plants with minor modifications. Plants avoid the deleterious effect of NaCl by various strategies (Parida and Das Citation2005). To counteract the toxicity of ROS, the defense systems in scavenging of cellular ROS have been developed in plants to cope with oxidative stress via the non-enzymatic and enzymatic systems (Noctor and Foyer Citation1998; Asada Citation1999). Small antioxidant molecules such as water-soluble ascorbate, glutathione (GSH), water insoluble α-tocopherol, and carotenoids are the main nonenzymatic agents for ROS scavenging (Noctor and Foyer Citation1998; Munné-Bosch and Alegre Citation2002). Several enzymes are involved in the detoxification of ROS; superoxide dimutase (SOD) can convert to H2O2 (Bowler et al. Citation1992). A variety of peroxidases such as ascorbate peroxidase (APX) and Guaiacol peroxidase (POX) catalyze the breakdown of H2O2. APX, often presenting in high levels in chloroplast, plays a key role in the ascorbate–glutathione cycle (Tijen and Ìsmail Citation2005). POX, which is broadly distributed in the cells and various plant tissues, can catalyze the oxidation of various substrates in the cell at the expense of hydrogen peroxide (Thomas et al. Citation2005). Several reports have shown that there is a close association of antioxidant capacity and salinity tolerance in higher plants (Amor et al. Citation2005; Kim et al. Citation2005).
Azolla, is an aquatic fern native of Asia and Africa. There is a symbiotic relationship between Azolla and the nitrogen fixer, cyanobacteria alga Anabaena azollae which invades certain cavities on the dorsal lobes of the leaves with true roots on the ventral surface. It is used as a biofertilizer to crops because of its ability to fix dinitrogen at high rates and low cost. In Azolla, nitrogen nutrition is assured by either elemental nitrogen or mineral nitrogen like nitrate and ammonia. Nitrate is generally the most prevalent form of mineral nitrogen in normal field conditions. It was suggested that combined-N sources restrict the entry of Na+ into the cells of Azolla significantly probably by its own enhanced uptake and consequent reduction of Na+ entry into the cells as reported in the higher plant Zostera maraina (Garcia-Sanchez et al. Citation2000; Singh et al. Citation2008).
Interest in salt tolerance of Azolla arises for its possible contribution in nitrogen economy. Recently, many workers studied the biology, its uses and the factors including the salt (NaCl) affecting the growth and other metabolic activities of Azolla spp. (Rai and Rai Citation1999; Rai et al. Citation2001; Singh et al. Citation2008). However, no reports are available that concern the changes in the isoforms of the major antioxidative enzymes in absence or presence of nitrate source in Azolla during salt stress and the process of adaptation. The present study focused on the effect of salt stress on growth, lipid membrane peroxidation, accumulation of hydrogen peroxide, electrolyte leakage, some nonenzymatic antioxidant contents, and specific activity of major antioxidative enzymes in absence or presence of nitrate in Azolla–Anabaena association which will be helpful in formulating the strategies to understand the salt tolerance in Azolla caroliniana, in developing a suitable salt-tolerant Azolla biofertilizer under saline soils and also in minimizing the salt toxicity.
Materials and methods
Plant material and growth condition
A. caroliniana Wild (known as water velvet) was provided by Prof. C. Van Hove, Catholic University of Louvain, Belgium. The plants were acclimated in the greenhouse of the Faculty of Science, Alexandria, in 2500 cm3 polyethylene vessels which were filled with a nitrogen free, modified [KNO3 and Ca(NO3)2 were replaced by KCl and CaCl2, respectively] Hoagland solution (2/5 concentration, pH 5.1). About 5 g (fresh mass) of Azolla from the stock material were inoculated in each vessel. After 15 days an inoculum was taken from each vessel to make a new subculture, and so on.
Epiphytic microorganisms were removed from the plants by thorough washing with distilled water. The cultures were grown in a growth chamber under 16-h photoperiod at an irradiance of 1200 µmol m−2 s−1 (cool white fluorescent tubes) and (light/dark) temperature of 28–30/20–25 °C (stock culture). Before being used the plants were surface sterilized with 0.2% Clorax (El-Aggan Citation1982), then thoroughly washed with water.
Salt treatment and growth estimation
Five grams of Azolla plants were transferred to 250 ml vessels containing 2/5 modified Hoagland solution and different concentrations of sodium chloride (0, 50, 100, and 150 mM) for 5, 10, and 15 days. Replacement of potassium chloride of modified Hoagland solution by potassium nitrate was taken place in another treatment.
The number of generations and doubling time (DT) were determined from the fresh mass and duration of experiment employing the expression given by Peters et al. (Citation1979): n (final mass) = n°× 2G, where G = number of generations, n° = initial mass of Azolla plants (mass of inoculum). Doubling time = duration of experiment per one generation. Relative growth rate (RGR) was calculated by using the formula of Subudhi and Watanabe (Citation1981): RGR [kg kg−1 d−1] = 0.693 DT−1.
Estimation of Na + and K+ content
After washing the plants and then blotted on filter papers, known amounts of the Azolla plants were weighted (100 mg fresh mass). Minerals were determined by the method as described by Masood et al. (Citation2006). Fresh Azolla plants were digested with HNO3:HClO4 mixture (1:1, v/v) in a boiling water bath for 30 min, and Na+ and K+ were determined using an atomic absorption spectrophotometer (Perkin-Elmer, 2380).
Estimation of electrolyte leakage
The electrolyte leakage was determined as described by Dionisio-Sese and Tobita (Citation1998). Fresh Azolla plants (200 mg) were cut into pieces of 5 mm length and placed in test tubes containing 10 ml distilled deionized water. The tubes were incubated in a water bath at 32 °C for 2 h and the initial electrical conductivity of the medium (EC1) was measured. The samples were autoclaved at 121 °C for 20 min to release all electrolytes; cooled to 25 °C and the final electrical conductivity (EC2) was measured. The electrolyte leakage% (EL%) was calculated by using the formula EL%=(EC1/EC2)×100.
Estimation of lipid peroxidation
Lipid peroxidation was measured as the amount of MDA produced by the thiobarbituric acid (TBA) reaction, as described by Dhindsa et al. (Citation1981). Fresh leaves (0.5 g) of control and NaCl treated-plants were homogenized in 2 ml of 20% (w/v) trichloroacetic acid (TCA). The homogenate was centrifuged at 3500×g for 20 min. 1.5 ml of 20% TCA containing 0.5% (w/v) TBA and 100 µL 4% butylated hydroxytoluene in ethanol were added to 0.5 ml of the aliquot of the supernatant. The mixture was heated at 95 °C for 30 min and was then quickly cooled in ice. The extracts were centrifuged at 10,000×g for 15 min and the absorbance was measured at 532 nm. The value of non-specific absorbance at 600 nm was subtracted. The concentration of MDA was calculated using an extinction coefficient of l55 mM−1 cm−1.
Estimation of hydrogen peroxide (H2O2)
Sample preparation and H2O2 estimation was done as described by Mukherjee and Choudhuri (Citation1983). Leaves (0.5 g) were homogenized in a mortar and pestle at 4 °C with 3 ml of potasssium phosphate buffer (50 mM, pH 7.8). The homogenate was centrifuged at 6000×g for 25 min. The supernatant was used in the assay for H2O2. The reaction mixture contained 3 ml of the extracted solution, 1 ml of 0.1% titanium sulfate in 20% H2SO4 (v/v). The mixture was then centrifuged at 6000×g for 15 min. The intensity of yellow color of the supernatent was measured at 410 nm. H2O2 level was calculated using the extinction coefficient of 0.28 µmol−1 cm−1.
Estimation of ascorbate and glutathione levels
The ascorbic acid (AA) concentration was measured by using the 2,6-dichlorophenol-indophenol (DCPIP) photometric method of Guri (Citation1983). Fresh leaves (1 g) were quickly detached and homogenized in l0 ml of ice-cold 0.0005 M EDTA solution containing 3% TCA for 1−2 min. The homogenate was quickly filtered through Whatman No. 4 filter paper and brought up to 20 ml with EDTA–TCA extracting solution. The reaction mixture contained 1 ml of distilled water, 2 ml of DCPIP reagent, and 2 ml of filtered leaf extract. The absorbance was measured quickly at 600 nm. The concentration of AA was determined from a standard curve that was prepared previously using various concentrations of AA. DCPIP reagent was prepared by dissolving 13 mg of DCPIP and 3 g of reagent grade anhydrous sodium acetate in 1 liter of distilled water.
Reduced glutathione and oxidized glutathione (GSSG) levels were determined according to the method of Griffith (Citation1980). Leaf tissues from control and NaCl treated plants were ground using a pestle and mortar in liquid N2 with 1 ml of 5% sulphosalicylic acid and centrifuged at 10,000×g for 5 min. A 300 µl aliquot of the supernatant was removed and neutralized to pH 7.0 by adding l8 µl of 7.5 mM triethanolamine. A 150 µl sample was used for the determination of total glutathione (GSH±GSSG). Another 150 µl sample was pretreated with 3 µl 2-vinyl pyridine for 60 min at 20 °C to mask GSH and to allow the determination of GSSG alone. Fifty micro liters from both types of samples were mixed with 700 µl of 0.3 mM NADPH, 100 µl of 10 mM 5,5′-dithiobis-(2-nitrobenzoic acid) (DTNB), and 150 µl of 125 mM NaPO4–6.3 mM EDTA buffer, pH 6.5. Ten micro liters of glutathione reductase (GR) (50 U/ml) was added and the change in absorbance at 412 nm was monitored. A standard curve prepared using GSH and GSSG was used in the calculation of the amounts of total GSH, GSSG, and reduced GSH (total GSH–GSSG).
Preparation of enzyme extract and assay of enzyme activity
Leaf tissues (0.5 g) were ground to a fine powder in liquid N2 and then homogenized in 2 ml of 50 mM potassium phosphate buffer (pH 7.0), 1 mM EDTA, 1 mM D–isoascorbic acid, 2% (w/v) polyvinylpyrrolidone (PVP), and 0.05% (w/v) Triton X-100 using a chilled pestle and mortar following the method of Gossett et al. (Citation1994). The homogenate was centrifuged at 10,000×g for 10 min at 4 °C and the supernatants were collected and used for the assays of catalase (CAT), APX, POX, and GR. Protein concentrations in the enzyme extract were determined by the method of Bradford (Citation1976) using defatted BSA (Sigma, fraction V) as a standard.
Catalase EC1.11.1.6 activity was determined spectrophotometricalty by measuring the rate of H2O2 disappearance at 240 nm, taking Δ£ at 240 nm as 43.6 M−1 cm−1 (Patterson et al. Citation1984). The reaction mixture contained 50 mM potassium phosphate (pH 7.0) and 10.5 mM H2O2 (Miyagawa et al. Citation2000). The reaction was run at 25 °C for 2 min after adding the enzyme extract and the initial linear rate of decrease in absorbance at 240 nm was used to calculate the activity.
Ascorbate peroxidase EC1.11.1.11 was assayed as described by Nakano and Asada (Citation1981). The reaction mixture contained 50 mM potassium phosphate (pH 7.0), 0.2 mM EDTA, 0.5 mM AA, and 0.25 mM H2O2. The reaction was started at 25°C by the addition of H2O2 after adding the enzyme extract. The decrease in absorbance at 290 nm for 1 min was recorded and the amount of ascorbate oxidized was calculated from the extinction coefficient 2.8 mM−1 cm−1.
Guaiacol peroxidase EC1.11.1.7 activity was measured spectrophotometrically at 25 °C by following the method of Tatiana et al. (Citation1999). The reaction mixture (2 ml) consisted of 50 mM potassium phosphate (pH 7.0), 2 mM H2O2, and 2.7 mM guaiacol. The reaction was started by the addition of enzyme extract. The formation of tetraguaiacol was measured at 470 nm (£ = 26.6 mM−1 cm −1).
Glutathione reductase EC1.6.4.2 activity was determined at 25 °C by measuring the rate of NADPH oxidation as the decrease in absorbance at 340 nm (£ = 6.2 mM−1 cm−1) according to the method of Halliwell and Foyer (Citation1978). The reaction mixture (1 ml) consisted of 100 mM Tris-HCl (pH 7.8), 21 mM EDTA, 0.005 mM NADPH, 0.5 mM GSSG, and the enzyme. NADPH was added to start the reaction. For CAT, APX, POX, and GR, one unit of enzyme was defined as the amount of enzyme necessary to decompose 1 µmol of substrate per min at 25 °C.
For assay of SOD EC1.15.1.1, fresh leaves (1 g) were homogenized in 8 ml potassium phosphate buffer (50 mM, pH 7.8) containing 0.1 mM Na2EDTA and 1% insoluble PVP with a chilled pestle and mortar. The homogenate was centrifuged at 20,000×g for 20 min. The supernatant was collected and used for the assay of SOD following the method of Beyer and Fridovich (Citation1987). The reaction mixture was prepared by mixing 27 ml of 50 mM potassium phosphate (pH 7.8), 1.5 ml of L-methionine (300 mg/l0 ml), 1 ml of nitroblue tetrazolium salt (14.4 mg /10 ml), and 0.75 ml of Triton X-100. Aliquots (1 ml) of this mixture were delivered into small glass tubes, followed by 20 µL of enzyme extract and 10 µL of riboflavin (4.4 mg/100 ml). The cocktail was mixed and then illuminated for 7 min in an aluminum foil-lined box, containing two 20 W fluorescent tubes. A control tube in which the sample was replaced by 20 µL of buffer was run in parallel and the A560 was measured in all tubes. The test tubes containing the reaction mixtures were exposed to light-immersing the glass tubes in a cylindrical glass container three-fourth filled with clean water and maintained at 25°C and placed in between two 20 W fluorescent tubes. The increase in absorbance due to formazan formation was read at 560 nm. Under the described conditions, the increase in absorbance without the enzyme extract was taken as 100% and the enzyme activity was calculated by determining the percentage inhibition per min. Fifty percent of inhibition was taken as equivalent to one unit of SOD activity.
Statistical analysis
Statistical analysis of the results was carried out according to Duncan's multiple range tests. Data were subjected to a two-way ANOVA and the LSD at p>0.05 was determined following the method of Sokal and Rohlf (Citation1995).
Results
Plant growth
When Azolla plants were treated with different concentrations of NaCl (0, 50, 100, and 150 mM) for 5, 10, and 15 days in nitrogen-free medium or the medium supplemented with 4 mM KNO3, the biomass, DT, and RGR were changed markedly (). In combined nitrogen free medium, plants incubated at 100 mM NaCl survived for 5 days. However, in the medium supplemented with NO3, plants incubated at 100 mM NaCl can survive for 10 days. Under all treatments, there were severe decrease in biomass and RGR and this decrease resulted in an increase in DT over the control. Also, the decrease in biomass and RGR was increased by increasing the concentration of NaCl and the duration of experiment except at 50 mM NaCl in presence of nitrate; there was an increase in RGR by increasing duration of experiment where the values were 0.05, 0.07, and 0.12 after 5, 10, and 15 days, respectively ().
Table 1. Growth characterized by biomass, doubling time (DT) and relative growth rate (RGR) of Azolla plants treated by different concentrations of NaCl (0, 50, 100, and 150 mM) for 5, 10, and 15 days in absence and presence of nitrate. Each value is the mean±S.E. of five replicates. Different letters indicate significant differences (p<0.01) between treatments as evaluated by Duncan's multiple comparison test.
Sodium and potassium ion contents
Data in revealed that there was a significant increase in the endogenous Na+ concentration by salt stress. Conversely, K+ concentration decreased significantly by salt stress. Accordingly, K+/Na+ ratio decreased significantly. Replacement of potassium chloride by potassium nitrate induced a significant reduction in Na+ accumulation, for example, Azolla plants treated with 50 mM NaCl for 15 days showed a reduction in Na+ accumulation equal to half value as compared with the corresponding salinized plants only. Conversely, potassium content increased as compared with plants incubated in absence of N. Accordingly, K+/Na+ ratio increased by NaCl treatment in presence of as compared with the corresponding salinized plants.
Table 2. Changes in concentrations of sodium and potassium ions and K+/Na+ ratio in Azolla plants treated by different concentrations of NaCl (0, 50, 100, and 150 mM) for 5, 10, and 15 days in absence and presence of nitrate. Each value is the mean±S.E. of five replicates. Different letters indicate significant differences (p<0.01) between treatments as evaluated by Duncan's multiple comparison test.
Hydrogen peroxide, lipid peroxidation, and percentage of electrolyte leakage
A change in H2O2 content is a good indicator of the status of ROS scavenging capacity of plants under oxidative stress. Azolla plants treated with 50 mM NaCl for 10 days showed a significant enhancement in the H2O2 content in absence or presence of nitrate and the increase was 3- and 1.7-fold, respectively, in comparison with control (A).
Figure 1. Changes in H2O2 content (A), lipid peroxidation (B) and percentage of electrolyte leakage (C) in Azolla plants exposed to 50 mM NaCl for 10 days in absence of nitrate (−N) or in its presence (+N). Each value is the mean±S.E. of five replicates. Different letters indicate significant differences (P<0.05) between treatments as evaluated by Duncan's multiple comparison test.
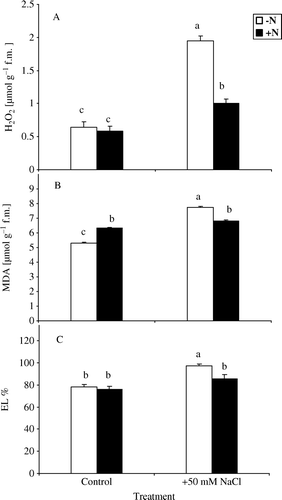
Changes in lipid peroxidation and percentage of electrolyte leakage serve as indicators of extent of oxidative damage under stress (Nagesh Babu and Devaraj Citation2008; Xiao-shan and Jian-guo Citation2009). The concentration of MDA, a product of lipid peroxidation, increased significantly in response to treatment of Azolla plant with 50 mM NaCl for 10 days in absence of nitrate. However, it did not increase significantly in presence of nitrate as a nitrogen source. The percentage of increase in absence and presence of nitrate was 32% and 7%, respectively, in comparison with controls (B).
The solute leakage of Azolla in absence and presence of nitrate was assessed to study the extent of membrane damage due to NaCl. Figure (1C) revealed that the percentage of solute leakage in response to treatment of Azolla with 50 mM NaCl was higher in absence of nitrate as compared to its presence and the values were 97.0 and 85.6%, respectively.
Status of antioxidant pool under salinity stress
Reduced GSH and AA are common antioxidants used by plants to reduce ROS levels in vivo. revealed that Azolla plants incubated in N-free medium did not exhibit significant change in AA concentration by salt stress. However, reduced GSH level increased significantly by salt stress but the GSSG level did not change significantly by NaCl treatment, it altered from 2.64 to 2.94 nmol g−1 f.m. consequently, reduced to oxidized glutathione (GSH/GSSG) ratio increased by salt stress ().
Figure 2. Changes in ascorbic acid content in Azolla plants exposed to 50 mM NaCl for 10 days in absence of nitrate (−N) or in its presence (+N). Each value is the mean±S.E. of five replicates. Different letters indicate significant differences (P<0.05) between treatments as evaluated by Duncan's multiple comparison test.
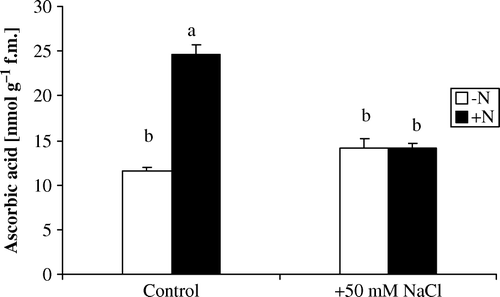
Figure 3. Changes in reduced glutathione (A), oxidized glutathione contents (B) and GSH/GSSG ratio (C) in Azolla plants exposed to 50 mM NaCl for 10 days in absence of nitrate (−N) or in its presence (+N). Each value is the mean±S.E. of five replicates. Different letters indicate significant differences (P<0.05) between treatments as evaluated by Duncan's multiple comparison test.
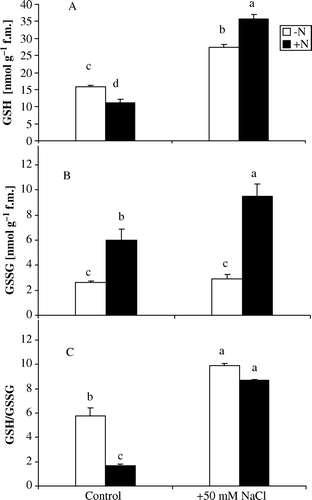
Addition of nitrate resulted in reduction in the concentration of AA from 24.66 to 14.33 nmol g−1 f.m. in salt treated-Azolla plants (). In contrast, reduced and GSSG increased by salt stress. The GSSG content was approximately 1.5-fold the control value. Reduced to GSSG ratio increased by about 2.3-fold the corresponding control value ().
Salinity-induced modulation in the activities of ROS scavenging enzymes
Since the H2O2 content varied significantly between Azolla plant treated with 50 mM NaCl for 10 days in absence or presence of nitrate and the controls, we determined the specific activity of H2O2 scavenging enzymes, CAT, POX, and APX. Interestingly, specific activity of all studied antioxidant enzymes increased significantly under salt stress in absence and presence of nitrate. However, specific activity of APX and SOD increased significantly under salt stress in presence of nitrate in comparison with its absence ().
Figure 4. Changes in GR, CAT, APX, POX and SOD [Unit mg−1 protein] specific activity in Azolla plants exposed to 50 mM NaCl for 10 days in absence of nitrate (−N) or in its presence (+N). Each value is the mean±S.E. of five replicates. Different letters indicate significant differences (P<0.05) between treatments as evaluated by Duncan's multiple comparison test.
![Figure 4. Changes in GR, CAT, APX, POX and SOD [Unit mg−1 protein] specific activity in Azolla plants exposed to 50 mM NaCl for 10 days in absence of nitrate (−N) or in its presence (+N). Each value is the mean±S.E. of five replicates. Different letters indicate significant differences (P<0.05) between treatments as evaluated by Duncan's multiple comparison test.](/cms/asset/59e0c1e4-1bd8-4c32-a37b-da5efc0a843e/tjpi_a_628452_o_f0004g.gif)
The increase in SOD activity in absence and presence of nitrate was 2- and 2.5-fold of untreated plants, respectively, (). However, it was 1.5- and 2.3-fold in APX.
Discussion
Salinity is a major environmental factor that limits plant growth and crop productivity (Parida and Das Citation2005). In the present investigation, addition of NaCl (0, 50, and 100 mM) to combined N for 5, 10, and 15 days resulted in severe reduction in growth of A. caroliniana in terms of biomass and RGR. It was suggested that salinity reduced chlorophyll content, chl a/b ratio, dry mass and whole chain electron transport activity which appears to be a characteristic of an indirect effect of salt on nitrogenase activity where this has consequently resulted in the reduced energy supply from the host for nitrogenase activity (Meeks et al. Citation1983; Rai and Rai Citation2003). Also, it was reported that the inhibition of nitrogenase activity is due to disturbed electron transport, loss in ability of heterocysts to protect nitrogenase from oxygen due to disturbed plasmamembrane permeability, and inadequate supply of reductants and ATP (Tel-Or and Melhamed-Harel Citation1981; Rai et al. Citation2001).
In the medium supplemented with nitrate, the rate of growth was better than in the N-free medium in both treated and untreated Azolla plants. Many authors reported that Azolla plants grew significantly better in presence of nitrate in comparison to those entirely dependent upon symbiotically fixed nitrogen (Rai and Rai Citation2003; Mostafa and Hassan Citation2006). In our study, addition of different concentrations of NaCl (0, 50, 100, and 150 mM) for 5, 10, and 15 days in presence of 4 mM nitrate exhibited a reduction in biomass and RGR, and this reduction resulted in an increase in DT over the control. Also, the decrease in biomass and RGR was further declined by NaCl concentration (), and duration of experiment except at 50 mM NaCl in presence of nitrate, there was an increase in RGR by increasing duration of experiment. Under the condition, i.e. in the presence of nitrate, the contribution of cyanobacterial fixed nitrogen is then negligible (Reddy et al. Citation1989; Rai and Abraham Citation1995). In contrary, Azolla-Anabaena relationship is tolerant to repression by combined nitrogen (Peters Citation1978; Mostafa and Hassan Citation2006). It has been reported reduction in the NaCl-induced growth inhibition of cyanobacteria by nitrate and the reason given is that and Na+ share a common transport system (Reddy et al. Citation1989; Rai and Tiwari Citation1999). Thus, nitrate protects the growth of Azolla plants by its own enhanced uptake and consequent reduction of Na+ entry into the cells (Rai and Rai Citation2003). Recently, it was suggested that such protection is only due to the availability of combined-N to the association, otherwise in this symbiotic association, nitrogen only available through the biological nitrogen-fixation (energy requiring process) by its cyanobiont partner (Singh et al. Citation2008). So, energy is spared due to availability of N-source, which may be utilized by Azolla for other physiological and biochemical events to counteract the adverse effect of salt stress.
Salinity stress caused a significant increase in Na+ content in Azolla plants and a considerable decrease in K+ concentration, resulting in a significant decline in the K+/Na+ ratio (). It was reported that one of the key features of plant salt tolerance is the ability of plant cells to maintain an optimal K+/Na+ ratio (Tester and Davenport Citation2003). Under salt stress, the K+/Na+ ratio shows a tendency to decrease and this occurs as a result of either excessive Na+ accumulation in plant tissue or enhanced K+ leakage from the cell. Potassium leakage normally happens as a result of NaCl induced membrane depolarization under saline conditions (Shabala et al. Citation2003). It is possible that in presence of high salt concentrations, the amount of naturally occurring K+ may suppress plant growth (Chen et al. Citation2005). Our results indicate that Azolla plants lack a mechanism to maintain homeostasis in this terms as there were high Na+ content and low K+.
Replacement of KCl by KNO3 in nutrient solution of target fern under salt stress has improved the possibility of K+ absorbance, and therefore relieves the adverse saline effects (). Potassium plays an important role in balancing membrane potential and turgor activating enzymes, regulating osmotic pressure, stoma movement, and tropisms (Cherel Citation2004). Consequently, a suitable K+/Na+ is important for the adjustment of cell osmoregulation, turgor maintenance, stomatal function, activation of enzymes, protein synthesis, oxidants metabolism, and photosynthesis (Shabala et al. Citation2003). This leads us to point out that the upregulation of the salt tolerance of Azolla by nitrate could be considerably achieved through avoiding Na+ absorption and enhancing K+ absorption and accumulation which cosequently improved K+/Na+ ratio. Thus, K+/Na+ ratio is a suitable criterion for the salt tolerance of Azolla.
Salt stress induces the production of ROS including superoxide radicals, hydrogen peroxide, and hydroxyl radicals. Under the prevailing experimental conditions, there was a significant accumulation of H2O2 in Azolla plants in absence or presence of nitrate in response to salt stress; the increase was 3- and 1.7-fold in comparison with controls, respectively. This was accompanied by increase in MDA content that is the decomposition product of polyunsaturated fatty acids of membranes and consequently, an increase in the percentage of electrolyte leakage (B, C). The rate of lipid peroxidation level in terms of MDA can therefore be used as an indication to evaluate the tolerance of plants to oxidative stress as well as the sensitivity of plants to salt stress (Jain et al. Citation2001; Masood et al. Citation2006). It is also known that the formation ROS enhances peroxidation at the cellular level and that the rate of such enhancement relates to plant species and the severity of stress (Navari-Izzo et al. Citation1996). In the present study, addition of nitrate resulted in a significant reduction in H2O2 content, MDA and the percentage of electrolyte leakage (C). It was recorded that suitable concentration of nitrate in the nutrient solution with 100 mM NaCl showed lowering in the intensity of lipid peroxidation and EL caused by salinity stress in two winter wheat cultivars (Zheng et al. Citation2008).
In this investigation, plants incubated in N-free medium under salt stress exhibited insignificant changes of ascorbate () and GSSG contents, however, reduced GSH content increased significantly by salt stress (A, B). Consequently, GSH/GSSG ratio increased significantly by salt stress (C). The increase in GSH content in A. caroliniana might be due to high activity of GR. Reduced GSH plays an important role in the antioxidant defense system of Azolla, since it not only participates in the regeneration of ascorbate via dehydroascorbate reductase, but it can also react with singlet oxygen and ·OH radical and protect protein thiol groups (Asada Citation1994).
Presence of nitrate under salt stress resulted in a reduction in the AA content () and increased level of reduced GSH and GSSG in Azolla plants (A, B). Also, reduced to GSSG ratio increased by about 2.3-fold the corresponding control value (C). The decreased level of ascorbate in Azolla plants may be due to its participation in reducing H2O2 to H2O which catalyzed by high level of APX activity. Ascorbate is the principal electron donor and it is oxidized to dehydroascorbate in the reaction of APX with H2O2 and reduced back to ascorbate in the presence of GR (Zhang and Kirkham Citation1996). Hence, the levels of ascorbate and GSH play an important role in oxidative defense. This decrease in ascorbate level might be correlated with the increased activity of APX and GR or due to its participation in the ascorbate–glutathione oxidative pathway. It was recorded that there was a decrease in ascorbate level in Bruguiera parviflora is likely to be due to its participation in reducing H2O2 to H2O (Parida et al. Citation2004).
The GR specific activity in A. caroliniana increased 2.5-fold under salinity stress relative to the control. GR induction by salinity was also observed (Chaparzadeh et al. Citation2004; Parida et al. Citation2004). In contrast, it was recorded that there was no significant alteration in GR activity of rice leaf (Lee et al. Citation2001). Increased GR in presence of nitrate may also maintain a high ratio of GSH/GSSG which is required for the regulation of several CO2 fixing enzymes (Foyer and Halliwell Citation1976). We could be suggested that nitrate elevated GR activity could increase the ratio of NADP+/NADPH, thereby ensuring the availability of NADP+ to accept electrons resulting into less flow of electron to O2 for generation of ROS (Reddy et al. Citation2004).
Plants have two major enzymatic ways to detoxify H2O2 produced by photorespiration and SOD activity. They can do so via CAT and/or via ascorbate peroxidise. In our study, CAT specific activity increased significantly in absence and presence of nitrate () which is possibly due to enhancement of H2O2 levels (Lee et al. Citation2001). Increases in the activities of CAT have been reported in soybean (Comba et al. Citation1998), tobacco (Bueno et al. Citation1998), and mulberry (Chinta et al. Citation2001). Conversely, a decrease in CAT activity was also reported in mangrove (Parida et al. Citation2004) and rice roots (Tijen and Ìsmail Citation2005) exposed to NaCl stress.
It was reported that salinity treatment to rice roots generated high levels of intercellular H2O2 which induced cytosolic APX (Asada Citation1992; Lee et al. Citation2001). In Azolla APX specific activity was increased significantly in absence and presence of nitrate (). This increase in APX specific activity could be due to activation of preexisting APX or the synthesis of new APX (Lee et al. Citation2001).
Guaiacol peroxidase specific activity has been used as an indicator for stresses like high temperature and salinity (Lee et al. Citation2001; Dash and Mohanty Citation2002). In A. caroliniana a high level of POX activity was induced in all treatments that agrees with the earlier reports of a salt induced increase in peroxidase activity in foxtail millet (Sreenivasulu et al. Citation2000) and rice (Lee et al. Citation2001).
A number of works have shown that high salinity retards CO2 fixation in mangroves and enhances reduction of molecular oxygen to generate highly toxic superoxide radicals (Cheeseman et al. Citation1997; Sobrado Citation1999; Takemura et al. Citation2000). The exposure of A. caroliniana to 50 mM NaCl induced an increase in the SOD specific activity equal to 2- and 2.5-fold of untreated plants in absence and presence of nitrate, respectively, (). This increase in SOD activity is in agreement with the results reported in Bruguiera gymnorrhiza (Takemura et al. Citation2000). However, our result is in contrast with those of Santos et al. (Citation2001) who reported that there was a reduced levels of SOD in sunflower plant under salt stress. Also, it was recorded that SOD activity was lower in ammonia fed maize and sunflower plants under salinity stress (Rios-Gonzalez et al. Citation2002). Recently, it was also recorded that activities of some antioxidant enzymes in winter wheat cultivars were enhanced in response to KNO3 and NaCl treatment (Zheng et al. Citation2008).
Reactive oxygen species have been hypothesized to serve as subcellular messenger in gene regulatory and signal transduction pathways. It has been demonstrated association of ROS in the regulation of differential gene expression of SOD in rice (Kaminaka et al. Citation1997, Citation1999). Based on our data and previous studies, it is clear that the increase in SOD activity of salt-treated Azolla plants in presence of nitrate was indirectly correlated with the increase in the transcript level.
In conclusion, addition of nitrate in the nutrient solution with NaCl could alleviate symptoms of the individual salt stress by improving growth of Azolla plants by its own enhanced uptake and consequent reduction in Na+ entry into the cells which resulted in an increase in K+ accumulation by improving its uptake or reducing its leakage (increase in K+/Na+ ratio). Consequently, its presence exhibited a significant reduction in EL, H2O2, and MDA contents. Also, it resulted in a reduction in the AA content, increase in reduced GSH and GSSG levels, and enhancement in the specific activity of antioxidant enzymes. But, it is unknown whether the enhancement in the activities of these enzymes was due to an up-regulation of the genes controlling their synthesis or an increase in the activity of constitutive enzyme pools. The results from this study provide base-line information and a system necessary to conduct further studies related to genetic basis of nitrate and salinity tolerance in A. caroliniana.
Acknowledgements
We are very grateful to Prof. Dr Weam El-Aggan, professor of Plant Physiology, Faculty of Science, University of Alexandria for her kind constructive advice.
References
- Amor , NB , Hamed , KB , Debez , A , Grignon , C and Abdelly , C . 2005 . Physiological and antioxidant responses of the perennial halophyte Crithmum maritimum to salinity . Plant Sci. , 168 : 889 – 899 .
- Asada , K . 1992 . Ascorbate peroxidase-a hydrogen peroxide scavenging enzyme in plants . Physiol Plant. , 85 : 235 – 241 .
- Asada , K . 1994 . “ Production and action of active oxygen species in photosynthetic tissues ” . In Causes of photooxidative stress and amelioration of defense systems in plants , Edited by: Foyer , CH and Mullineaux , PM . 77 – 100 . Boca Raton, Ann Arbor, London, Tokyo : CRC Press .
- Asada , K . 1999 . The water–water cycle in chloroplasts: scavenging of active oxygen and dissipation of excess photons . Ann Rev Plant Physiol Plant Mol Biol. , 50 : 601 – 639 .
- Beyer , WF and Fridovich , I . 1987 . Assaying for superoxide dismutase activity: some large consequences of minor changes in conditions . Anal Biochem. , 161 : 559 – 566 .
- Bowler , C , Montagu , MV and Inzé , D . 1992 . Superoxide dismutase and stress tolerance . Plant Mol Biol. , 43 : 83 – 116 .
- Bradford , MM . 1976 . A rapid and sensitive method for the quantification of microgram quantities of proteins utilizing the principle of protein-dye binding . Anal Biochem. , 72 : 248 – 254 .
- Bueno , P , Piqueras , A , Kurepa , J , Savoure , A , Verbruggen , N , Montagu , VM and Inze , D . 1998 . Expression of antioxidant enzymes in responses to abscisic acid and high osmoticum in tobacco BY-2 cell cultures . Plant Sci. , 138 : 27 – 34 .
- Chaparzadeh , ND , Amico , ML , Havari-Nejad , RA , Izzo , R and Navari-Izzo , F . 2004 . Antioxidative responses of Calendula officinalis under salinity conditions . Plant Physiol Biochem. , 42 : 695 – 701 .
- Cheeseman , JM , Herendeen , LB , Cheeseman , AT and Clough , BF . 1997 . Photosynthesis and photoprotection in mangroves under field conditions . Plant Cell Environ. , 20 : 579 – 588 .
- Chen , Z , Newman , I , Zhou , M , Mendham , N and Zhang , GS . 2005 . Screening plants for salt tolerance by measuring K+ flux: a case study for barley . Plant Cell Environ. , 28 : 1230 – 1246 .
- Cherel , L . 2004 . Regulation of K+ channel activities in plants: from physiological to molecular aspects . J Exp Bot. , 55 : 337 – 351 .
- Chinta , S , Lakshmi , A and Giridarakumar , S . 2001 . Change in the antioxidant enzyme efficacy in two high yielding genotypes of mulberry (Morus alba L.) under NaCl salinity . Plant Sci. , 161 : 613 – 619 .
- Comba , ME , Benavides , MP and Tomaro , ML . 1998 . Effect of salt stress on antioxidant defense system in soybean root nodules . Aust J Plant Physiol. , 25 : 665 – 671 .
- Cuin , TA and Shabala , S . 2007 . Compatible solutes reduce ROS induced potassium efflux in Arabidopsis roots . Plant Cell Environ. , 30 : 875 – 885 .
- Dash , S and Mohanty , N . 2002 . Response of seedling to heat-stress in cultivars of wheat: growth temperature-dependent differential modulation of photosystem 1 and 2 activity, and foliar antioxidant defense capacity . J Plant Physiol. , 159 : 49 – 59 .
- Demidchik , V , Shabala , SN and Coutts , KB . 2003 . Free oxygen radicals regulate plasma membrane Ca2 +- and K+-permeable channels in plant root cells . J Cell Sci. , 116 : 81 – 88 .
- Dhindsa , RS , Plumb-Dhindsa , P and Thorpe , TA . 1981 . Leaf senescence: correlated with increased levels of membrane permeability and lipid peroxidation, and decreased levels of superoxide dismutase and catalase . J Exp Bot. , 32 : 93 – 101 .
- Diego , AM , Marco , AO , Carlos , AM and José , C . 2003 . Photosynthesis and activity of superoxide dismutase peroxidase and glutathione reductase in cotton under salt stress . Environ Exp Bot. , 49 : 69 – 76 .
- Dionisio-Sese , ML and Tobita , S . 1998 . Antioxidant responses of rice seedlings to salinity stress . Plant Sci. , 135 : 1 – 9 .
- El-Aggan WH . 1982 . A comparative study of the growth and nitrogenase activity of five Azolla Species as affected by various environmental factors . [Ph.D. Thesis] . Louvain : Université Catholique De Louvain.
- Foyer , C and Halliwell , B . 1976 . The presence of glutathione and glutathione reductase in chloroplasts: a proposed role in ascorbic acid metabolism . Planta , 133 : 21 – 25 .
- Garcia-Sanchez , M , Jaime , MP , Ramos , A , Sanders , D and Fernandes , JA . 2000 . Sodium-dependent nitrate transport at the plasma membrane of leaf cells of the marine higher plant Zostera maraina L . Plant Physiol. , 122 : 879 – 885 .
- Gossett , DR , Millhollon , EP and Lucas , MC . 1994 . Antioxidant response to NaCl stress in salt tolerant and salt sensitive cultivars of cotton . Crop Sci. , 34 : 706 – 714 .
- Griffith , OW . 1980 . Determination of glutathione and glutathione disulphide using glutathione reductase and 2-vinyl pyridone . Anal Biochem. , 106 : 207 – 212 .
- Guri , A . 1983 . Variation in glutathione and ascorbic acid content among selected cultivars of Phaseolus vulgaris prior to and after exposure to ozone . Can J Plant Sci. , 63 : 732 – 737 .
- Halliwell , B and Foyer , CH . 1978 . Properties and physical function of a glutathione reductase purified from spinach leaves by affinity chromatography . Planta , 139 : 9 – 17 .
- Hasegawa , PM , Bressan , RA , Zhu , JK and Bohnert , HJ . 2000 . Plant cellular and molecular responses to high salinity . Annu Rev Plant Physiol Plant Mol Biol. , 51 : 463 – 499 .
- Jain M , Mathur , Koul S , Sarin NB . 2001 . Ameliorative effects of proline on salt stress-induced lipid peroxidation in cell lines of ground nut (Arachis hypogea L.) . Plant Cell Reports 20 : 463 – 468 .
- Kaminaka , H , Morita , S , Yokoi , H , Masumura , L and Tanaka , K . 1997 . Molecular cloning and characterization of a cDNA for plastidic copper/zinc-superoxide dismutase in rice (Oryza sativa L.) . Plant Cell Physiol. , 38 : 65 – 69 .
- Kaminaka , H , Morita , S , Tokumoto , M , Yokoyama , H , Masumura , T and Tanaka , K . 1999 . Molecular cloning and characterization of a cDNA for an iron-superoxide dismutase in rice (Oryza sativa L.) . Biosci Biotechnol Biochem. , 63 : 302 – 308 .
- Kim , SY , Lim , JH , Park , MR , Kim , YJ , Park , TI , Se , YW , Choi , KG and Yun , SJ . 2005 . Enhanced antioxidant enzymes are associated with reduced hydrogen peroxide in barley roots under saline stress . J Biochem Mol Biol. , 38 : 218 – 224 .
- Lee , DH , Kim , YS and Lee , CB . 2001 . The inductive responses of the antioxidant enzymes by salt stress in the rice (Oryza sativa L.) . J Plant Physiol. , 158 : 737 – 745 .
- Masood , A , Ahmad Shah , N , Zeeshan , M and Abraham , G . 2006 . Differential response of antioxidant enzymes to salinity stress in two varieties of Azolla (Azolla pinnata and Azolla filiculoides) . Environ Exp Bot. , 58 : 216 – 222 .
- Meeks , JC , Wycoff , KL , Chapman , JS and Enderlin , CS . 1983 . Regulation of expression of nitrate and dinitrogen assimilation by Anabaena species . Appl Environ Microbiol. , 45 : 1351 – 1359 .
- Miyagawa Y , Tamori M , Shigeoka . 2000 . Evaluation of the defense system in chloroplasts to photooxidative stress caused by paraquat using transgenic tobacco plants expressing catalase from Escherichia coli . Plant Cell Physiol . 41 : 311 – 320 .
- Mostafa , EM and Hassan , AMA . 2006 . Effect of chilling on growth and nitrogen assimilation in Azolla caroliniana . Biol Plant. , 50 ( 4 ) : 641 – 646 .
- Mukherjee , SP and Choudhuri , MA . 1983 . Implication of water stress-induced changes in levels of endogenous ascorbic acid and hydrogen peroxide in Vigna seedlings . Physiol Plant. , 58 : 166 – 170 .
- Munné-Bosch , S and Alegre , L . 2002 . The function of tocopherols and tocotrienols in plants . Crit Rev Plant Sci. , 21 : 31 – 57 .
- Nagesh Babu , R and Devaraj , VR . 2008 . High temperature and salt stress response in French bean (Phaseolus vulgaris) . Aust J Crop Sci. , 2 ( 2 ) : 40 – 48 .
- Nakano , Y and Asada , K . 1981 . Hydrogen peroxide is scavenged by ascorbate-specific peroxidase in spinach chloroplasts . Plant Cell Physiol. , 22 : 867 – 880 .
- Navari-Izzo , F , Quatacci , MF and Sgherri , CLM . 1996 . Superoxide generation in relation to dehydratation and rehydration . Biochem Society Transactions. , 24 : 447 – 450 .
- Noctor , G and Foyer , CH . 1998 . Ascorbate and glutathione: keeping active oxygen under control . Ann Rev Plant Physiol Plant Mol Biol. , 49 : 249 – 279 .
- Owens , S . 2001 . Salt of the earth. Genetic engineering may help to reclaim agricultural land and lost due to salinization . EMBO Rep. , 2 : 877 – 879 .
- Parida , AK and Das , AB . 2005 . Salt tolerance and salinity effects on plants: a review . Ecotoxicol Environ Saf. , 60 : 324 – 349 .
- Parida , AK , Das , AB and Mohanty , P . 2004 . Defense potentials to NaCl in a mangrove, Bruguiera parviflora: differential changes of isoforms of some antioxidative enzymes . J Plant Physiol. , 161 : 531 – 542 .
- Patterson , BD , Payne , LA , Chen , Y and Graham , D . 1984 . An inhibitor of catalase induced by cold chilling-sensitive plants . Plant Physiol. , 76 : 1014 – 1018 .
- Peters , GA . 1978 . Blue green algae and algal associations . Biosci. , 28 : 580 – 585 .
- Peters GA , Mayne BC , Ray TB , Toia RE. 1979 . Physiology and biochemistry of the Azolla-Anabaena symbiosis . In : Nitrogen and rice . Los Baños : International Research Institute ; p. 325 – 344 .
- Rai , AK and Abraham , G . 1995 . Relationship of combined nitrogen sources to salt tolerance in fresh water cyanobacterium Anabaena doliolum . J Appl Bacteriol. , 78 : 501 – 506 .
- Rai , AK and Rai , V . 2003 . Effect of NaCl on growth, nitrate uptake and reduction and nitrogenase activity of Azolla pinnata/Anabaena azollae . Plant Sci. , 164 : 61 – 69 .
- Rai , AK and Tiwari , SP . 1999 . NO3-nutrition and salt tolerance in the cyanobacterium Anabaena sp. PCC 7120 and mutant strains . J Appl Microbiol. , 86 : 991 – 995 .
- Rai , V and Rai , AK . 1999 . Growth behaviour of Azolla pinnata at various salinity levels and induction of high salt tolerance . Plant Soil. , 206 : 79 – 84 .
- Rai , V , Tiwari , SP and Rai , AK . 2001 . Effect of NaCl on nitrogen fixation of unadapted and NaCl-adapted Azolla pinnata–Anabaena azollae . Aquat Bot. , 71 : 109 – 117 .
- Reddy , AR , Chaitanya , KV and Vivekanandan , M . 2004 . Drought-induced response of photosynthesis and antioxidant metabolism in higher plants . J Plant Physiol. , 161 : 1189 – 1202 .
- Reddy , BR , Apte , SK and Thomas , J . 1989 . Enhancement of cyanobacterial salt tolerance by combined nitrogen . Plant Physiol. , 89 : 204 – 210 .
- Rios-Gonzalez , K , Erdei , L and Lips , SH . 2002 . The activity of antioxidant enzymes in maize and sunflower seedlings as affected by salinity and different nitrogen sources . Plant Sci. , 162 : 923 – 930 .
- Santos , CLV , Campos , A , Azevedo , H and Caldeira , G . 2001 . In situ and in vitro senescence induced by KCl stress: nutritional imbalance, lipid peroxidation and antioxidant metabolism . J Exp Bot. , 52 : 351 – 360 .
- Shabala , SN , Shabal , L and Van Volkenburgh , E . 2003 . Effect of calcium on root development and root ion fluxes in salinised barley seedlings . Funct Plant Biol. , 30 : 507 – 514 .
- Singh , SS , Singh , SK and Mishra , AK . 2008 . Na+ regulation by combined nitrogen in Azolla pinnata–Anabaena azollae symbiotic association during salt toxicity . Ecotoxicol Environ Saf. , 69 : 32 – 38 .
- Sobrado , MA . 1999 . Leaf photosynthesis of the mangrove Avicennia germinans as affected by NaCl . Photosynthetica. , 36 : 547 – 555 .
- Sokal , RR and Rohlf , FJ . 1995 . Biometry: the principles and practice of statistics in biological research , 3rd ed , 321 – 356 . New York : W.H. Freeman .
- Sreenivasulu , N , Grimm , B , Wobus , U and Weschke , W . 2000 . Differential response of antioxidant compounds to salinity stress in salt-tolerant and salt-sensitive seedlings of fox-tail millet (Setaria italica) . Physiol Plant. , 109 : 435 – 442 .
- Subudhi , BR and Watanabe , I . 1981 . Differential phosphorus requirements of Azolla species and strains in phosphorus limited continuous culture . Soil Sci Plant Nutr. , 27 : 237 – 247 .
- Takemura , T , Hanagata , N , Sugihara , K , Baba , S , Karube , I and Dubinsky , Z . 2000 . Physiological and biochemical responses to salt stress in the mangrove, Bruguiera gymnorrhiza . Aquat Bot. , 68 : 15 – 28 .
- Tatiana , Z , Yamashita , K and Matsumoto , H . 1999 . Iron deficiency induced changes in ascorbate content and enzyme activities related to ascorbate metabolism in cucumber roots . Plant Cell Physiol. , 40 : 273 – 280 .
- Tel-Or E , Melhamed-Harel H. 1981 . Adaptation to salt of photosynthetic apparatus in cyanobacteria . In : Akoyunoglou G . Photosynthesis. Philadelphia , PA : Bablan International Science Services . p. 455 – 462 .
- Tester , M and Davenport , R . 2003 . Na+ tolerance and Na+ transport in higher plants . Ann Bot. , 91 : 503 – 527 .
- Thomas , DS , Traianos , AY and Athanasios , SE . 2005 . Expression of peroxidases during seeding growth in Ebenus cretica L. as affected by light and temperature treatments . Plant Grow Reg. , 46 : 143 – 151 .
- Tijen , D and Ìsmail , T . 2005 . Comparative lipid peroxidation, antioxidant defense systems and proline content in roots of two rice cultivars differing in salt tolerance . Environ Exp Bot. , 53 : 247 – 257 .
- Wang , LJ , Liu , YJ , Ma , K , Wang , JZ and Liu , XN . 1998 . Effect of NaCl treatment on free radical metabolism of fig (Ficus cartica L.) . calli Adv Horti. , 2 : 235 – 241 .
- Xiao-shan , W and Jian-guo , H . 2009 . Changes of proline content, activity, and active isoforms of antioxidative enzymes in two alfalfa cultivars under salt stress . Agricultural Sci in China. , 8 ( 4 ) : 431 – 440 .
- Zhang , J and Kirkham , MB . 1996 . Enzymatic responses of the ascorbate glutathione cycle to drought in sorghum and sunflower plants . Plant Sci. , 113 : 139 – 147 .
- Zheng , Y , Jia , A , Ning , T , Jialin , XUJ , Li , Z and Jiang , G . 2008 . Potassium nitrate application alleviates sodium chloride stress in winter wheat cultivars differing in salt tolerance . J Plant Physiol. , 165 : 1455 – 465 .