Abstract
Lupinus species are commonly used as annual forage and for grain production. They are considered alternative crops to soybean due to their adaptation to cool environments and dry soils. The present study is an analysis of the chemical changes coming from biomass removal in a sweet genotype of Lupinus angustifolius. Mechanical damage induced significant increases in antioxidant activity (12.4%), as well as in flavonoid and phenolic content (36.6% and 12.0%, respectively). This sweet lupin also exhibited a higher induced response (68.2%) in the alkaloid content after the damage. These same alkaloids were identified in the control samples and all of them, except lupanine, showed higher relative abundances in response to mechanical damage. Traces of α-isolupanine were detected in control samples and showed a 23.5-fold increase in response to biomass removal. Mechanical damage also produced a striking increase (8.6-fold) in relative abundance of genistin.
Introduction
Plants exhibit multiple mechanisms to defend themselves from a wide range of attackers (Hanley et al. Citation2007; Rasmann and Agrawal Citation2009). Under the selective pressure posed by herbivores, plants have evolved in several physical and biochemical ways so as to restrain their action. A common defensive strategy is the biosynthesis of chemical defenses, mainly secondary metabolites whose production is triggered by herbivores or pathogens (Gatehouse Citation2002; Kessler and Baldwin Citation2002; Rasmann and Agrawal Citation2009).
Most secondary metabolites represent adaptive traits that have gone through continuous diversification during evolution by natural selection in order to protect plants against abiotic factors (Chludil et al. Citation2008; Leicach et al. Citation2010), viruses, bacteria, fungi, and particularly herbivores (Vilariño et al. Citation2005). Inducible defenses can play different roles such as those of toxins, antifeedants, and antinutrients among them (Wink Citation2003).
Lupinus genus (Leguminosae – Papilionoideae) is a member of the tribe Genisteae (family: Fabaceae). White lupin (Lupinus albus L.), yellow lupin (Lupinus luteus L.), and narrow-leafed or blue lupin (Lupinus angustifolius L.) are employed as a protein source for both animal and human nutrition, not only for their nutritional value (high in protein, lipids, and dietary fiber), but also for their adaptability to marginal soils and climates. Although white lupin exhibits higher grain yield and protein content, narrow-leafed lupin has proved to grow faster and to be more resistant to disease (Dracup and Kirby Citation1996). The latter, L. angustifolius L. is commonly used as a dual-purpose crop: as green biomass in annual forage as well as grain yield. Some Lupinus species are currently considered emerging alternative crops to soybean, because of their easy adaptation to cool environments and dry soils. This crop is not widely cultivated in Argentine at present however; conditions seem to be favorable to introduce it in many of its regions. Several field trials were previously performed to evaluate the requirements and yields for different Lupinus genotypes (Vilariño and Ravetta Citation2008).
Most species within Leguminosae are known to produce chemical defenses against herbivores and pathogenic microorganisms, particularly phenylpropanoid derivatives (coumarins, flavonoids, and isoflavonoids) and alkaloids (Aoki et al. Citation2000; Dixon et al. Citation2002; Wink and Mohamed Citation2003). Alkaloids are responsible for the bitter taste and low palatability in Lupinus species. Plant breeders have developed sweet lupins (alkaloid concentration in seeds below 0.05%) with higher palatability but showing more vulnerability to insect attack and herbivory (Wink Citation1992; Lee et al. Citation2007).
Lupinus species are characterized by the production of quinolizidine alkaloids (QAs), which have been used for chemotaxonomic classification (Wink et al. Citation1995). Several authors have reported antiviral, antibacterial, and antifungal properties for these nitrogenated compounds (Wink Citation2003; Erdemoglu et al. Citation2007; Bermudez-Torres et al. Citation2009).
Among phenylpropanoid derivatives, isoflavonoids are known to be essential for Fabaceae survival due to their natural role in rhizosphere plant-microbial interactions. Their contribution to plant defense strategies as antifungal and anti-feeding agents seems also important (Aoki et al. 2000; Cardoza et al. Citation2005).
Dynamic aspects of isoflavonoid production can be analyzed in terms of Lupinus species defense mechanisms, as their production has been proved to be regulated by different kinds of stress caused by biotic and/or abiotic factors (Bednarek et al. Citation2003). For example, isoflavone content in Lupinus leaves and other plant organs has been shown to vary with temperature, light exposure, drought, and frequency of pathogens and/or herbivore attack (Bednarek et al. Citation2003; Von Baer et al. Citation2006).
Isoflavone content also depends on the ontogenic state of the plant. Characterization of individual isoflavones has been carried out mainly on young lupin plants grown in laboratory conditions or open-field experiments (D'Agostina et al. Citation2008).
A previous report showed changes in alkaloid profiles in two Lupinus species of leaves produced by Anticarsia gemmatalis attack, also describing their effects on subsequent herbivory (Vilariño et al. Citation2005). Increments in the alkaloid content following herbivory seem to improve plant resistance to subsequent caterpillars attack, suggesting that they play an active role in these species defensive strategies. However, changes in other potential chemical defenses were not evaluated (Vilariño et al. Citation2005).
The aim of this study was to evaluate changes in chemical defenses from L. angustifolius Gungurru genotype aerial parts in response to mechanical damage.
Materials and methods
General
Analytical solvents were purchased from Sintorgan (Chemical Center SRL, Buenos Aires, Argentina). Kieselgel 60 F254 TLC aluminum sheets for thin layer chromatography were purchased from Merck (Research AG, Buenos Aires, Argentina). Chemical standards and reagents, such as 1,1-diphenyl-2-picryl-hydrazyl (DPPH), Folin and Ciocalteu reagent, quercetin, ascorbic acid, butylated hydroxytoluene (BHT), genistin, genistein, kaempferol-3-O-glucoside, and chlorogenic acid hemihydrate were purchased from Sigma Aldrich S.A. (Buenos Aires, Argentina).
Plant material and treatments
Lupinus angustifolius Gungurru (Gu) is an Australian genotype that has a short growth cycle in Buenos Aires (85 days at a mean temperature of 14.4 °C) (Vilariño and Ravetta, Citation2008). Twenty plants per square meter were sown in a silty clay loam soil (Argiudoll, pH: 6.5) in an experimental field located in Buenos Aires, Argentina (34° 37′ S, 58° 20′ W). Seeds were inoculated with Bradyrhizobium spp. strains specific for Lupinus provided by Rizobacter Argentina S.A.
The experimental design was a split-plot with four replications. Plants were harvested during the beginning of flowering excluding those showing evidence of insects attack.
The chemical analysis was performed on aerial parts from plants that were previously subjected to mechanical damage (D) and undamaged samples were used as controls (C). Five D samples (20% shoot length removed) and five C samples were randomly harvested 10 days after cutting treatment. Biomass removed included the main apex, stems, and leaves from the upper shoot. The cutting level resembled that of biomass loss caused by large herbivores (sheep, cattle) attack.
The relative abundances of the most common phytoalexins and the antioxidant capacity were evaluated in Lupinus aerial parts samples 10 days after mechanical damage and were compared to the corresponding values obtained from control samples.
Chemical extraction
Dry plant material (10 g) was milled and submitted to continuous extraction with light petroleum ether (6 h) using a Soxhlet apparatus in order to exclude lipidic components. Remaining plant material was then extracted by the same procedure but using methanol as a solvent (6 h). The corresponding crude extract was evaporated to dryness under vacuum conditions at 40 °C, and processed further to separate alkaloids. Methanolic dry extract was dissolved in water, acidified with 5% HCl up to pH 2, and extracted with chloroform (3×). Acid aqueous solution was alkalinized up to pH 14 with NH4OH and then extracted with chloroform (3×) to obtain the alkaloid extract (AE). The resultant alkaline aqueous solution was neutralized and then extracted with EtAcO:n-BuOH (2:1) (3×) to obtain the polar extract (PE) that was analyzed further to detect phenylpropanoid compounds.
Extracts AE and PE were analyzed by chromatography on Kieselgel 60 F254 TLC aluminium sheets. Chromatograms were visualized by UV light and/or by a chromogenic reaction with both Draggendorff and Gibbs reagents to detect alkaloids and phenolic compounds, respectively. Alkaloid and phenylpropanoid profiles, as well as phenolic content (PC) and flavonoid contents (FC) were determined in all samples.
DPPH radical-scavenging assay (DPPH-RSA)
DPPH-RS assay modified method (Brand-Williams et al. Citation1995) was performed to detect antioxidant compounds known to reduce the stable DPPH free radical.
A 10−3 M DPPH radical solution was prepared by dissolving 10 mg DPPH in 25 ml methanol. 0.5 ml 10−3 M DPPH methanol solution was added to 0.500, 0.250, and 0.125 ml of the PE stock solution (10 mg ml−1), respectively. Each mixture was brought to 5.0 ml with methanol, shaken vigorously and allowed to stand at room temperature under dark conditions. Thirty minutes after the incubation, absorbance decrease was monitored at 517 nm in a spectrophotometer (Hewlett-Packard Model 8453) using 10−4 M DPPH solution as blank; DPPH-radical scavenging activity was expressed as inhibition percentage for each sample by means of the following equation:
where Abs DPPH is DPPH methanol solution absorbance and Abs SAMPLE is the sample absorbance. BHT and ascorbic acid were used as positive controls.
Antioxidant EC50 values were calculated by lineal regression from plots, where the x-axis represented the concentration of tested plant extracts and positive controls and the y-axis the average percent of scavenging capacity.
Determination of phenolic content (PC)
Polar extract PC values were determined according to Swain and Hillis (Citation1959) modified method. PE was dissolved in methanol (10 mg ml−1). A 100 µl aliquot of each extract was mixed with 50 µl 2N Folin-Ciocalteu reagent and 500 µl 1N sodium carbonate solutions, and then brought to 5.0 ml with deionized water. Each solution was allowed to stand at room temperature for one hour and its absorbance was measured at 725 nm, using a spectrophotometer (Hewlett-Packard Model 8453). PC data were expressed as chlorogenic acid µmols equivalents g−1 DM, by extrapolation from the correlation curve.
Determination of FC
Flavonoid content was determined using the aluminum chloride colorimetric method described by Chang et al. (Citation2002). One and half milliliter of 95% alcohol, 0.1 ml 10% aluminum chloride hexahydrate, 0.1 ml 1 M potassium acetate, and 2.8 ml of deionized water were added to 0.5 ml of a 0.1 g ml−1 solution of each sample in deionized water. After 40 min incubation at room temperature, absorbance was measured at 415 nm in a spectrophotometer (Hewlett-Packard Model 8453), using deionized water as blank. Extrapolations from a quercetin standard curve (0–50 µg ml−1) allowed us to obtain FC values, expressed as milligram quercetin equivalents (QE) g−1 DM.
Phenylpropanoid HPLC analysis
Each sample was dissolved in methanol (5 mg ml−1) and analysis was performed in a high-pressure liquid chromatograph (HPLC), Agilent 1100 A series equipped with a UV detector (Agilent Technologies, Inc., Wilmington, DE, USA), using an Eclipse XDB-C18 reversed phase HPLC column (5 µm, 4.6×150 mm). The mobile phases included degassed solutions of solvent A (0.1% glacial acetic acid in Milli-Q water) and solvent B (0.1% glacial acetic acid in acetonitrile). Following the injection of 5 μl of the sample, solvent B was increased from 15 to 45% over 40 min and then held at 45% for 10 min. The solvent flow rate was 1 ml min−1. The wavelength of the UV detector was set at λ 270 nm. Solvent ratios were expressed on a volume basis. The same procedure was applied to standard compounds solutions (0–100 µg ml−1).
Compounds were identified by their retention times and by co-chromatography with commercial standards (genistin, genistein, and kaempferol-3-O-glucoside).
Total alkaloid content
A weighted fraction of each AE was dissolved in CHCl3 and analyzed by gas chromatography (GC) with a GC 6890N Agilent Technologies (Agilent Technologies, Inc., Wilmington, DE, USA) using HP-5 (30 m×0.25 mm i.d.×0.25 µm) capillary column with 1:20 split ratio and helium as carrier gas; flow rate: 1 ml min−1; injector temperature: 250 °C and detector temperature: 280 °C. Temperature program: isothermal at 120 °C for 2 min, 120 °C to 300 °C at a rate of 6 °C min−1; then 10 min isothermal. Total alkaloid content was calculated on the basis of the sum of individual alkaloids peak areas in the GC chromatogram, and the value was related to weighted dry matter (DM).
Gas chromatography – mass spectrometry alkaloid analysis
Alkaloids analysis was performed on a GC 6890N Agilent coupled with a MS 5973 (Agilent Technologies, Inc., Wilmington, DE, USA) using HP-5 ms (30 m×0.25 mm i.d.×0.25 µm) capillary column and same chromatographic conditions. The interface temperature was 300 °C, and the acquisition was from m/z 40 to 560 UMA. Electron impact mass spectra were recorded at 70 eV. Alkaloids structures were tentatively identified according to their mass fragmentation through library search (Nist Mass Spectral Database Ms Search v. 1.6d 98. L) and by their Kovats indexes, which were determined by co-chromatography with a mixture of linear alkanes: pentadecane (C15), docosane (C22), tetracosane (C24), triacontane (C30), and hexatriacontane (C36). Mass spectral data were further confirmed by comparison with literature data (Wink et al. Citation1995).
Statistical analysis
Data is reported as mean values±standard deviation of four independent extractions, and activity measurements were done in four replications. Analysis of variance was performed for all data using General Linear Model. Mean values were compared by least significant difference (LSD) at 0.05 probability level (Tukey's test – InfoStat/Professional, Version 1.1, 2002).
Results
DPPH radical-scavenging activity
shows the chemical data and antioxidant capacity of L. angustifolius Gungurru samples. Gu PE showed a concentration-dependent on antiradical activity. Gu control samples exhibited a free radical scavenging capacity with EC50 mean value of 677±19 µg ml−1. Mechanical damage produced a significant increase of antioxidant activity (12.4%) with EC50 mean value of 593±34 µg ml−1 for D samples.
Table 1. Antioxidant capacity, flavonoid, phenolic, and alkaloid content.
Butylated hydroxytoluene (EC50 10.81 µg ml−1) and ascorbic acid (EC50 5.94 µg ml−1) were used as positive controls showing free radical scavenging activities 55- to 100-fold higher than all studied samples.
Phenolic content (PC)
Phenolic content values exhibit a moderate increment (12%) in response to biomass removal treatment ().
Flavonoid content (FC)
Mechanical damage produced an increase (36.6%) in Gu samples FC, with a mean value of 28.0±2.1 quercetin milligram equivalents g−1 DM ().
Total alkaloid content
Gungurru, showed a low alkaloid content 0.44±0.07 mg g−1 DM (). However it exhibited inductive increases (68.2%) in alkaloid content after mechanical damage in newly produced tissues (). Similar results were reported in previous works (Wink Citation1983; Vilariño et al. Citation2005; Chludil et al. Citation2009).
Phenylpropanoid HPLC profiles
High-pressure liquid chromatograph analysis allowed the identification of two isoflavonoids, genistin (genistein-7-O-glucoside) (1) and genistein (3), and a flavonoid, kaempferol-3-O-glucoside (2) in L. angustifolius Gungurru genotype PE ().
Figure 1. Lupinus angustifolius Gungurru genotype chemical defenses.
Note: 1, genistein-7-O-glucoside; 2, kaempferol-3-O-glucoside; 3, genistein; 4, sparteine; 5, 11,12-dehydrosparteine; 6, Tetrahydrorhombifoline; 7, angustifoline; 8, α-isolupanine; 9, lupanine; 10, 13α-hydroxylupanine; and 11, 13-tigloyloxylupanine.
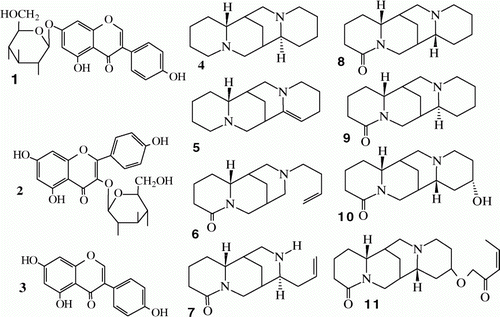
Undamaged Gu samples (C) showed kaempferol-3-O-glucoside as the major phenylpropanoid (2.39±0.66) while both isoflavonoids presented lower relative abundances, genistin (1.83±0.63), and genistein (1.29±0.16). Mechanical damage triggered a striking increment in genistin relative abundance (8.6-fold) with a lower increment in genistein (56%) and without significant changes in kaempferol-3-O-glucoside relative abundance (2.19±0.83).
Alkaloid profiles
Gas chromatography alkaloid analysis of Gu samples revealed the presence of eight alkaloids. GC-MS analysis showed that six of them exhibited tetra cyclic structures including one tigloyl-ester derivative ().
Identified alkaloids: sparteine [KI: 1786, M+: 234 (17%), fragmentary ions at m/z: 193 (37%), 176 (9%), 150 (8%), 148 (7%), 137 (100%), 136 (41%), 122 (22%), 110 (9%), 98 (55%)]; 11,12-dehydrosparteine [KI: 1836, M+: 232 (44%), fragmentary ions at m/z: 175 (38%), 163 (19%), 148 (36%), 135 (19%), 134 (100%), 97 (15%), 96 (29%)]; tetrahydrorhombifoline [KI: 2035, M+: 248 (1%), fragmentary ions at m/z: 208 (14%), 207 (100%), 112 (46%), 108 (29%), 55 (29%)]; angustifoline [KI: 2071, M+: 234 (1%), fragmentary ions at m/z: 193 (100%), 150 (56%), 112 (71%), 84 (17%), 55 (38%)]; α-isolupanine [KI: 2097, M+: 248 (25%), fragmentary ions at m/z: 150 (31%), 149 (57%), 136 (100%), 98 (22%), 94 (16%)]; lupanine [KI: 2160, M+: 248 (33%); fragmentary ions at m/z: 219 (9%), 150 (22%), 149 (54%), 136 (100%), 110 (17%), 98 (29%), 84 (38%)]; 13α-hydroxylupanine [KI: 2409, M+: 264 (18%), fragmentary ions at m/z: 246 (61%), 165 (44%), 152 (100%), 148 (38%), 134 (68%), 112 (19%), 108 (28%)]; and 13-tigloyloxylupanine [KI: 2764, M+: 346 (1%), fragmentary ions at m/z: 246 (100%), 148 (32%), 134 (92%), 112 (13%), 98 (11%)].
Most important alkaloids in Gungurru control samples (C) were 13-tigloyloxylupanine (44.9%) as the major alkaloid followed by lupanine (17.2%), 11,12-dehydrosparteine (13.6%), tetrahydrorhombifoline (12.1%), 13α-hydroxylupanine (8.5%), and sparteine (1.8%). Angustifoline and α-isolupanine relative abundances were lower than 1% ().
Table 2. Changes in alkaloid and phenylpropanoid relative abundances triggered by mechanical damage.
The same alkaloids were identified in control and mechanical damaged samples, most of them showing higher relative abundances in the latter. With the exception of sparteine, most of the alkaloids exhibited an increase in their relative abundances higher than 6%. A striking increment was detected for α-isolupanine (23.5-fold) and also for angustifoline (10.6-fold).
Significantly higher relative abundances were also found in the treated group for other alkaloids: more than threefold (379%) for 13α-hydroxylupanine, 107% for tetrahydrorhombifoline, 100% for sparteine, and 82% for 11,12-dehydrosparteine. No significant difference was observed in 13-tigloyloxylupanine relative concentration (6.4%). Lupanine was the only alkaloid showing a significantly lower concentration in damaged Gu samples compared to control ones (C).
Discussion
The two main responses of plants to herbivory are tolerance (i.e. the ability to support a certain level of damage by activating primary metabolism, also known as compensation), and evasion (i.e. biochemical changes related to increments in chemical defenses) (Kessler and Baldwin Citation2002; Nuñez-Farfán et al. Citation2007). Both mechanisms vary according to the species and type of herbivore.
Increments of herbivore-induced chemical defenses in leaves may render in a less palatable plant for future potential herbivores. This resistance may involve increased toxicity and/or decreased nutritive quality of leaves (Rasmann and Agrawal Citation2009).
Plants rarely produce a single class of defensive compounds. In fact, they usually biosynthesize several families of compounds that may be selectively induced in response to biotic stresses (Wittstock and Gershenzon Citation2002). QAs and phenolic compounds, particularly isoflavones, play an important role in the biochemistry and physiology of species within the Fabaceae family. In agreement with previous reports, the results on this paper show that alkaloid and phenylpropanoid profiles change in response to biotic stress factors (Pilewska et al. Citation2002; Bednarek et al. Citation2003; Vilariño et al. Citation2005; Chludil et al. Citation2009).
Secondary metabolite levels are known to depend on many factors including organ, developmental stage, and leaf age among others (Wink and Hartmann Citation1982; Aoki et al. Citation2000; Katagiri et al. Citation2000; Lee et al. Citation2007; D'Agostina et al. Citation2008). QAs and isoflavonoids are biosynthesized within the chloroplasts of active photosynthetic tissues and accumulated in different proportions in plant organs.
Samples of L. angustifolius Gungurru genotype showed, as expected from sweet varieties, low alkaloid content. This content significantly increased when the plant was subjected to mechanical damage. The main component in C and D samples was 13-tigloyloxylupanine. No new alkaloids were found as a result of mechanical damage. The significantly lower lupanine relative abundance in D samples (0.049±0.009) compared to the corresponding value in C samples (0.077±0.007) might be partially related to the increase of its derivatives 13α-hydroxylupanine, isolupanine and 13-tigloyloxylupanine abundances in the same samples, as it is their metabolic precursor. These results are in agreement with data reported by Wink (Citation1983). Mülbauer also reported high proportions of derived esters such as 13-tigloyloxylupanine, 13-benzoyloxylupanine, 13-trans-cinnamoyloxylupanine, and 4-acetoxylupanine for the same species (Mülbauer et al. Citation1987).
Alkaloid diversity seems to be correlated to herbivory resistance. In the case of lupin, such variability may play an important role in the defense against biotic stresses (Adler and Kittelson Citation2004). It was previously demonstrated that induction of alkaloid production triggered in L. albus by A. gemmatalis attack resulted in an effective way to avoid further damage by the same species (Vilariño et al. Citation2005). During the present bioassay, Gungurru samples exhibited an increase of 68,2% in total alkaloid content, after being subjected to a cutting level resembling that of a large herbivore attack. This suggests that QAs might still play a role in this genotype resistance at ten days after damage.
Mechanical damage also resulted in a higher biosynthesis of phenolics and isoflavonoids in the newly produced tissues. Gungurru C samples produced kaempferol-3-O-glucoside as the major phenylpropanoid derivative followed by genistin and genistein. It has been suggested that the level of induction of phenylpropanoid may depend on every particular herbivore attack. High levels of these compounds have been correlated with oxidative stress produced by herbivore damage (Adler and Kittelson Citation2004; Cardoza et al. Citation2005).
A high free radical quenching capacity was correlated in D samples with high levels of phenolics and phenylpropanoids. The antioxidant activity did not seem to be affected by the important increase found in isoflavonoids abundances. Isoflavonoids have been reported to be less active towards DPPH assay than some flavonoids and phenolic acids. Moreover, their glycosilated derivatives have been proved to be even less active than non glycosilated ones. In fact, it was recently reported that glucose linkage can reduce fifty to one-hundred times the antioxidant potential of isoflavones (Zheng et al. Citation2010).
It has been stated that isoflavonoids display negative effects on herbivores, in general. (Simmonds and Stevenson Citation2001; Karowe and Radi Citation2011). For example, genistein and rutin exert deleterious effects on different insects feeding on Leguminosae. Genistin relative abundance has also been correlated to the resistance displayed by some soybean genotypes to pathogens (Piubelli et al. Citation2005).
Nevertheless, the presence of kaempferol glycoside does not seem to deter the herbivore attack to Glycine max, suggesting that they do not represent an important defensive strategy in this particular species. Chan et al. (Citation1978) early observed that kaempferol was less toxic to Heliothis virescens, Heliothis zea, and Pectinophora gossypiella than quercetin-based compounds exhibiting a catechol group. This reduced defensive capacity might help to understand the fact that no significant differences in kaempferol glycoside relative abundance were observed when comparing Gungurru C and D samples, suggesting that it does not play a defensive role in this L. angustifolius sweet genotype.
Environmental factors, along with genetic factors, seem to play an important role in determining alkaloid and phenylpropanoid profiles in L. angustifolius sweet genotype. Results on this paper may contribute to clarify chemical changes involved in the complex interactions between herbivores and plant defenses, where phenotypic traits are usually determined by a particular combination of both environmental and genetic factors.
The detection and identification of secondary metabolites, which are modulated by biochemical changes and take place after mechanical damage, contribute to increase knowledge on the defense mechanisms of this species, thus providing potentially useful information to develop more resistant cultivars in crop breeding programs.
Acknowledgements
We thank Universidad de Buenos Aires for financial support by UBACYT G067 and 20020090200215 grants, and Dr L. Rosso (Arkansas University-USA) for English language revision.
References
- Adler , LS and Kittelson , PM . 2004 . Variation in Lupinus arboreus alkaloid profiles and relationships with multiples herbivores . Biochem Syst Ecol. , 32 : 371 – 790 .
- Aoki , T , Akashi , T and Ayabe , S-I . 2000 . Flavonoids of leguminous plants: structure, biological activity, and biosynthesis . J Plant Res. , 113 : 475 – 488 .
- Bednarek , P , Kerhoas , L , Einhorn , J , Franski , R , Wojtaszek , P , Rybus-Zajac , M and Stobiecki , M . 2003 . Profiling of flavonoid conjugates in Lupinus albus and Lupinus angustifolius responding to biotic and abiotic stimuli . J Chem Ecol. , 29 : 1127 – 1142 .
- Bermudez-Torres , K , Martinez Herrera , J , Figueroa Brito , R , Wink , M and Legal , L . 2009 . Activity of quinolizidine alkaloids from three Mexican Lupinus against the lepidopteran crop pest Spodoptera frugiperda . BioControl. , 54 : 459 – 466 .
- Brand-Williams , W , Cuvelier , ME and Berset , C . 1995 . Use of a free radical method to evaluate antioxidant activity . LWT-Food Sci Technol. , 28 : 25 – 30 .
- Cardoza , YJ , Reidy-Crofts , J and Edwards , OR . 2005 . Differential inter- and intra-specific defense induction in Lupinus by Myzus prsicae feeding . Entomol Exp Appl. , 117 : 155 – 165 .
- Chan , BG , Waiss , AC (Jr) , Binder , RG and Elliger , CA . 1978 . Inhibition of lepidopterous larval growth by cotton constituents . Entomol. Exp. Appl. , 24 : 94 – 100 .
- Chang , CC , Yang , MH , Wen , HM and Chern , JC . 2002 . Estimation of total flavonoid content in propolis by two complementary colometric methods . J Food Drug Anal. , 10 ( 3 ) : 178 – 182 .
- Chludil , HD , Corbino , GB and Leicach , SR . 2008 . Soil quality effects on Chenopodium album flavonoid content and antioxidant potential . J Agric Food Chem. , 56 : 5050 – 5056 .
- Chludil , HD , Vilariño , M , del , P , Franco , ML and Leicach , SR . 2009 . Changes in Lupinus albus and Lupinus angustifolius alkaloids profiles in response to mechanical damage . J Agric Food Chem. , 57 : 6107 – 6113 .
- D'Agostina , A , Boschin , G , Resta , D , Annicchiarico , A and Arnoldi , A. 2008 . Changes of isoflavones during the growth cycle of Lupinus albus . J. Agric Food Chem. , 56 : 4450 – 4456 .
- Dixon , RA , Achnine , L , Kota , P , Liu , C , Srinivasa Reddy , MS and Wang , L . 2002 . The phenylpropanoid pathway and plant defence: a genomics perspective . Mol Plant Pathol. , 3 ( 5 ) : 371 – 390 .
- Dracup , M and Kirby , EJM . 1996 . Pod and seed growth and development of narrow-leafed lupin in a water limited Mediterranean-type environment . Field Crop Res. , 48 : 209 – 222 .
- Erdemoglu , N , Ozkan , S and Tosun , F . 2007 . Alkaloid profile and antimicrobial activity of Lupinus angustifolius L. alkaloid extract . Phytochem Rev. , 6 : 197 – 201 .
- Gatehouse , JA . 2002 . Plant resistance towards insect herbivores: a dynamic interaction . New Phytol. , 156 : 145 – 169 .
- Hanley , ME , Lamont , BB , Fairbanks , MM and Rafferty , CM . 2007 . Plant structural traits and their role in anti-herbivore defence . Perspect Plant Ecol Evol Syst. , 8 : 157 – 178 .
- Karowe , DN and Radi , JK . 2011 . Are the phytoestrogens genistein and daidzein anti-herbivore defenses? A test using the Gypsy Moth (Lymantria dispar) . J Chem Ecol. , 37 ( 8 ) : 830 – 837 .
- Katagiri , Y , Ibrahim , RK and Tahara , S . 2000 . HPLC analysis of white lupin isoflavonoids . Biosci Biotech Bioch. , 64 ( 6 ) : 1118 – 1125 .
- Kessler , A and Baldwin , IT . 2002 . Plant responses to insect herbivory: the emerging molecular analysis . Annu Rev Plant Biol. , 53 : 299 – 328 .
- Lee , MJ , Pate , JS , Harris , DJ and Atkins , CA . 2007 . Synthesis, transport and accumulation of quinolizidine alkaloids in Lupinus albus L. and L. angustifolius L . J Exp Bot. , 58 : 935 – 946 .
- Leicach , SR , Garau , AM , Guarnaschelli , AB , Yaber Grass , MA , Sztarker , ND and Dato , A . 2010 . Changes in Eucalyptus camaldulensis essential oil composition as response to drought preconditioning . J Plant Interaction , 5 ( 3 ) : 205 – 210 .
- Mülbauer , P , Witte , L and Wink , M . 1987 . New ester alkaloids from Lupinus (genus Lupinus) . Planta Med. , 3 : 237 – 239 .
- Nuñez-Farfán , J , Fornoni , J and Valverde , PL . 2007 . The evolution of resistance and tolerance to herbivores . Annu Rev Ecol Evol S. , 38 : 541 – 566 .
- Pilewska , M , Bednarek , P , Stobiecki , M , Zieli Ska , M and Wojtaszek , P . 2002 . Cell wall-associated isoflavonoids and β-glucosidase activity in Lupinus albus plants responding to environmental stimuli . Plant Cell Environ. , 25 : 29 – 40 .
- Piubelli , GC , Hoffmann-Campo , CB , Moscardi , F , Miyakubo , SH and Neves de Oliveira , MC . 2005 . Are chemicals compounds important for soybean resistance to Anticarsia gemmatalis? . J Chem Ecol. , 31 ( 7 ) : 1509 – 1525 .
- Rasmann , S and Agrawal , AA . 2009 . Plant defense against herbivory: progress in identifying synergism, redundancy, and antagonism between resistance traits . Curr Opin Plant Biol. , 12 : 473 – 478 .
- Simmonds , MS and Stevenson , PC . 2001 . Effects of isoflavonoids from Cicer on larvae of Heliocoverpa armigera . J Chem Ecol. , 27 ( 5 ) : 965 – 977 .
- Swain , T and Hillis , WE . 1959 . The phenolic constituents of Purmus domestica. I. The quantitative analysis of phenolic constituents . J Sci Food Agric. , 10 : 63 – 68 .
- Vilariño , M , del , P , Mareggiani , G , Yaber Grass , M , Leicach , SR and Ravetta , DA . 2005 . Post-damage alkaloid concentration in sweet and bitter lupin varieties and its effect on subsequent herbivory . J Appl Entomol. , 129 ( 5 ) : 233 – 238 .
- Vilariño , M , del , P and Ravetta , DA . 2008 . Tolerance to herbivory in lupin genotypes with different alkaloid concentration: Interspecific differences between Lupinus albus L. and L. angustifolius L . Environ Exp Bot. , 63 : 130 – 136 .
- Von Baer , D , Saelzer , R , Vega , M , Ibieta , P , Molina , L , Von Baer , E , Ibanez , R and Hashagen , U . 2006 . Isoflavones in Lupinus albus and Lupinus angustifolius: quantitative determination by capillary zone electrophoresis, evolution of their concentration during plant development and effect on anthracnose causing fungus Colletotrichum lupini . J Chil Chem Soc. , 51 : 1025 – 1029 .
- Wink , M . 1983 . Wounding-induced increase of quinolizidine alcaloide accumulation in lupin leaves . Z Naturforsch. , 38c : 905 – 909 .
- Wink , M . 1992 . “ The role of quinolizidine alkaloids in plant insect interactions ” . In Insect-plant interactions , Edited by: Bernays , EA . Vol. 4 , 131 – 166 . Boca Raton (FL) : CRC-Press .
- Wink , M . 2003 . Evolution of secondary metabolites from an ecological and molecular phylogenetic perspective . Phytochemistry , 64 : 3 – 19 .
- Wink , M and Hartmann , T . 1982 . Localization of the enzymes of quinolizidine alkaloid biosynthesis in leaf chloroplasts of Lupinus polyphyllus . Plant Physiol. , 70 : 74 – 77 .
- Wink , M , Meibner , C and Witte , L . 1995 . Patterns of quinolizidine alkaloids in 56 species of the genus Lupinus . Phytochemistry , 38 : 139 – 153 .
- Wink , M and Mohamed , GIA . 2003 . Evolution of chemical defense traits in the Leguminosae: mapping of distribution patterns of secondary metabolites on a molecular phylogeny inferred from nucleotide sequences of the rbcL gene . Biochem Syst Ecol. , 31 : 897 – 917 .
- Wittstock , U and Gershenzon , J . 2002 . Constitutive plant toxins and their role in defense against herbivores and pathogens . Curr Opin Plant Biol. , 5 ( 4 ) : 300 – 307 .
- Zheng , CD , Li , G , Li , HQ , Xu , XJ , Gao , JM and Zhang , AL . 2010 . DPPH-scavenging activities and structure-activity relationships of phenolic compounds . Nat Prod Commun. , 5 ( 11 ) : 1759 – 1765 .