Abstract
Carrot (Daucus carota L.) is widely utilized all over the world due to its enriched healthy nutritional composition. However, its growth and quality is adversely affected due to saline stress. To assess salt-induced regulation in different enzymatic and nonenzymatic antioxidants in the edible part of two cultivars (T-29 and DC-4) of carrot, a greenhouse experiment was conducted. The cultivars were grown for 90 days under varying (0, 50, 100, and 150 mM NaCl) saline regimes. High accumulation of glycinebetaine (GB), malondialdehyde (MDA), and ascorbic acid (AsA) contents was found in the roots of both carrot lines under varying saline regimes. However, total soluble proteins and activities of peroxidase (POD), catalase (CAT), and superoxide dismutase (SOD) declined in the edible part of both carrot cultivars. Alpha-tocopherol (α-Toco) contents remained almost unaffected at all saline regimes except at 150 mM NaCl, where tocopherol content increased markedly. Of both carrot cultivars, cv. DC-4 accumulated relatively higher amount of GB, soluble protein and α-Toco contents than cv. T-29. The cv. T-29 had considerably higher amounts of AsA and MDA and activities of POD, SOD, and CAT than those in cv. DC-4 both under saline and nonsaline conditions. Overall, GB, AsA, and MDA contents increased while enzymatic antioxidant activities decreased in both carrot cultivars under different saline regimes which indicated that the enzymatic antioxidant metabolism was negatively influenced in the edible part of carrot due to salinity stress. So it can be suggested that the carrot is salt sensitive and its nutritional value in terms of antioxidants declines under salt stress.
Introduction
Vegetables are essentially required to safeguard health particularly by precluding diseases as they are good source of vitamins and mineral nutrients (Jamil et al. Citation2006; Shahbaz et al. Citation2008, Citation2012; Noreen et al. Citation2012). Vegetables also supply trace elements which are essentially required for the prevention of diseases. Although extensive studies have been carried out on vegetables in relation to their growth and yield (Maas & Hoffman Citation1977; Noreen et al. Citation2010a, Citation2010b, Citation2012; Saleem et al. Citation2012), very little work has been conducted to appraise the influence of salinity stress on the nutritional composition of different vegetable crops (Noreen et al. Citation2010a, Citation2010b; Saleem et al. Citation2011, Citation2012).
Vegetables generally show upregulation of antioxidant metabolism in response to salinity stress as has been already observed in a number of vegetables such as brinjal, pea, radish, turnip, cauliflower, carrot, and cucumber (Volden et al. Citation2009; Colla et al. Citation2010; Neffati et al. Citation2011). Carrot (Daucus carota) is one of the most preferred vegetables worldwide for its edible roots possessing high-quality antioxidants (enzymatic and nonenzymatic), phytonutrients, amino acids, proline, mineral composition, vitamins A, sugars, carotene, proteins, carotenoids, and dietary fiber (Kumar et al. Citation2004). However, the production of this crop is very low due to its considerable sensitivity to a variety of abiotic stresses including salinity which is prevalent in many countries (Schmidhalter & Oertli Citation1991). Despite salt-induced reduction in carrot production, a variety of physiological processes are also impaired by this stressful factor. For example, Gibberd et al. (Citation2002) reported considerable reduction in leaf gas exchange attributes including photosynthetic rate in carrot plants. Recently, while working with a variety of attributes in two carrot cultivars, Bano et al. (Citation2012) have found a significant salt-induced suppression in plant growth, chlorophyll pigments, water relation attributes, photosynthetic rate, essentially required nutrients such as K+ and Ca2 +, leaf malondialdehyde (MDA), total phenolics, total soluble proteins, and activities of catalase (CAT), superoxide dismutase (SOD), and peroxidase (POD), while they observed a considerable increase in leaf turgor potential, Na+ and Cl− contents, proline, glycinebetaine (GB), ascorbic acid (AsA), and H2O2 contents in the leaf or root tissues of carrot plants. The relatively better growth of carrot cv. T-29 was found to be associated mainly with upregulation of water relations and higher leaf Ca2 +, proline, phenolics, and activity of SOD enzyme under saline conditions.
Very few reports are accessible from the literature on the effects of saline stress on the composition, particularly of antioxidative system, in carrot root, the edible part. In view of lack of such information, it was hypothesized that the salinity stress could alter the antioxidative system in carrot roots. Thus, the present investigation was conducted to examine how far salt stress could regulate antioxidative (enzymatic and nonenzymatic) system in the edible part of carrot, i.e. root. These findings of the present study would be helpful for the farmers whose soil or irrigation water are saline as well as those people who frequently consume carrot root for nutritional purposes.
Materials and methods
A greenhouse experiment was carried out to examine salt-induced changes in antioxidant metabolism (enzymatic and nonenzymatic) in edible part (root) of a potential vegetable – carrot (Daucus carota L.). Seeds of two carrot cultivars (DC-4 and T-29) were supplied by the Ayub Agricultural Research Institute, Faisalabad, Pakistan. A two-factor factorial completely randomized design with four replications was employed to set up the experiment. During experimentation, average values of photoperiod, light intensity, relative humidity, and temperature have already been described in Bano et al. (Citation2012). After 10 days of germination, three seedlings were maintained at two-leaf stage per replicate, and the pots were separated into four sets and supplied weekly with Hoagland's nutrient solution supplemented with four varied levels of salt (NaCl; 0, 50, 100, and 150 mM). The salt concentrations were increased stepwise per day with 50 mM NaCl. The plants were harvested three months after the commencement of the saline treatment. The plant roots were separated and kept in liquid nitrogen for the estimation of several attributes.
GB determination
Fresh root tissue (500 mg) was triturated in 10 mL of toluene (0.5% v/v) solution. After filtration, 1 mL of the supernatant was blended with 1 mL H2SO4 (2N). Then 0.5 mL of this solution was kept in a test tube, and 0.2 mL (0.1 M) KI3 solution was mixed to it. The mixture was ice cooled for 1 h. Then 2.8 mL of distilled H2O and dichloroethane (6 mL) were mixed to the solution. The absorbance of the organic layer was determined at 365 nm. The GB concentration was calculated following Grieve and Grattan (Citation1983).
MDA determination
MDA content in fresh roots was estimated with a spectrophotometer following Carmak and Horst (Citation1991). The contents were measured using the coefficient of difference between optical densities (ODs) at 600 and 532 nm.
AsA determination
AsA was determined following Mukherjee and Choudhuri (Citation1983). Fresh root material (500 mg) was blended in 10 mL trichloroacetic acid (6% v/v). Then 4.0 mL of the sample extract was mixed with 2 mL dinitrophenyl hydrazine (2% v/v) and one drop of thiourea (10% w/v). The solution was heated for 15 min in a water bath and cooled down, and 5 mL of sulfuric acid (80% v/v) was mixed to the solution. The OD was recorded at 530 nm.
Activities of antioxidant enzymes
The fresh root (500 mg) was ground well in 5 mL well cooled potassium phosphate buffer (50 mM). Then the mixture was vortexed and centrifuged at 12,000 × g for 15 min. The extract was separated, and SOD (EC 1.15.1.1) activity was determined using the procedure proposed by Giannopolitis and Ries (Citation1977). Inhibition in photoreduction of nitroblue tetrazolium (NBT) was used to appraise the activity of SOD. The reaction mixture (1 mL) (400 µL distilled H2O, 250 µL phosphate buffer [pH 7.8; 50 mM], 100 µL [13 mM] L-methionine, 100 µL [0.1% v/v] triton-X-100, 50 µL [50 µM] NBT, 50 µL [1.3 µM] riboflavin and 50 µL sample extract) in cuvettes were kept under light for 15 min. The OD of the irradiated aliquot was read at 560 nm, and SOD enzyme activity per unit was based on the amount of enzyme that inhibited 50% of NBT photoreduction.
The protocol of Chance and Maehly (Citation1955) was used for the determination of POD (EC 1.11.1.7) and CAT (EC 1.11.1.6) activities. The reaction mixture contained 0.1 mL plant extract, 20 mM guaiacol, 40 mM H2O2, and phosphate buffer (pH 5.0). The changes in OD of reaction mixture were recorded at 470 nm after every 30 second. A change in OD of reaction mixture per minute was obtained equivalent to 1 unit of POD activity. The reaction solution (3.0 mL) contained H2O2, phosphate buffer, and plant extract (0.1 mL). For CAT activity, the reaction was commenced by mixing the plant extract, and the changes in OD of the reaction mixture after every 20 second were recorded at 240 nm. The activities of all the three enzymes were measured on the basis of total protein measured as described by Bradford (Citation1976).
α-Toco content determination
The α-Toco content was determined following Backer et al. (Citation1980). Fresh root (500 mg) was extracted with 10 mL of a solution containing ethanol and petroleum ether (1.6:2 v/v) after which the mixture was centrifuged at 12,000×g. To 1 mL of the supernatant, 0.2 mL of 2,2-dipyridyl (2% v/v) in ethanol was mixed. Then the mixture was mixed occasionally and placed in dark for 5 min. The end product (red colored) was mixed well in 4 mL dH2O. The OD of the supernatant was recorded at 520 nm. The α-Toco contents were measured against a known curve obtained using known concentrations of α-Toco.
Statistical analysis
Analysis of variance (ANOVA) was worked out using the individual values of each parameter using JMP v.6.0. Significance between the salt levels was observed using the least significance difference test at 0.05% level.
Results
Salt stress had a marked reducing effect on root fresh and dry weights of both carrot cultivars. Of all salt regimes, 150 mM NaCl was highly inhibitory as compared to the other salt treatments and this has already been presented by Bano et al. (Citation2012).
A significant (P ≤ 0.001) increase in GB concentration was examined in the edible part of carrot when exposed to different (50, 100, and 150 mM NaCl) salt regimes as compared to the control plants grown under nonsaline conditions. Of both carrot cultivars, cv. DC-4 accumulated relatively higher amount of GB than cv. T-29 (). Maximum GB accumulation was observed at 150 mM NaCl.
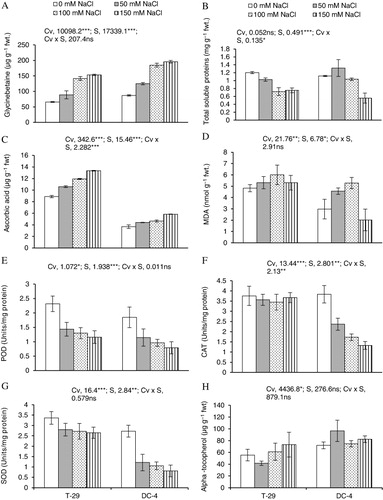
A significant inhibition in fresh root total soluble proteins of both carrot lines was examined due to root growing medium different salt regimes. Salt-induced reduction in soluble protein content was significantly higher in cv. DC-4 than in cv. T-29, particularly at 150 mM NaCl ().
AsA contents in the edible part of both carrot cultivars increased markedly (P ≤ 0.001) under saline conditions. A maximal increase in AsA was observed at 150 mM NaCl. Cultivar T-29 had considerably higher amount of AsA than cv. DC-4 both under saline and nonsaline conditions ().
A slight (P ≤ 0.05) increase in MDA contents was observed in roots of both carrot cultivars under all salt regimes. Overall, cv. T-29 had markedly higher concentration of MDA than that in cv. DC-4, particularly under saline regimes ().
In the present study, the activity of root POD, a key antioxidant enzyme, in both carrot cultivars suppressed considerably (P ≤ 0.001) under saline conditions (). The salt-induced decrease in POD activity was not consistent in both carrot cultivars under saline regimes, and cv. T-29 was higher than the other carrot cultivar in POD enzyme activity. Salt stress had a considerable reducing effect on root CAT activity in cv. T-29, whereas in cv. DC-4, it remained similar to that in plants grown under nonsaline conditions. Of both carrot cultivars, cv. T-29 had significantly higher activity of CAT than cv. DC-4 under all salt regimes (). Activity of SOD enzyme decreased in fresh root tissues of both carrot cultivars under saline stress. Cultivar T-29 had significantly higher activity of SOD than cv. DC-4 under all salt levels ().
α-Toco contents in the edible part of both carrot cultivars almost remained unchanged under saline conditions except at 150 mM NaCl where it increased markedly. Carrot cultivar DC-4 had considerably higher amount of α-Toco than cv. T-29 both under saline and nonsaline conditions ().
Discussion
In the present study, salt-induced regulation in enzymatic and nonenzymatic antioxidative defense system as well as osmoprotectants including GB in the edible part (root) of carrot was assessed. GB, as a potential osmoprotectant, plays an important role in ameliorating the adverse effects of high salt concentration by accumulating rapidly in many plants under stress conditions (Akram et al. Citation2011; Hu et al. Citation2012). In the present study, an increase in GB concentration was examined in the edible part of carrot when exposed to different salt regimes, particularly at 150 mM NaCl. These results are parallel to some earlier reports on different vegetable crops such as eggplant (Abbas et al. Citation2010; Shaheen et al. Citation2013), carrot (Bano et al. Citation2012), and cauliflower (Batool et al. Citation2013) in which enhanced accumulation of GB was reported in the upper parts (shoot) of all these plants.
AsA commonly known as vitamin C has been considered as an effective antioxidant not only in humans but also in plants which can efficiently detoxify free radicals as well as oxidants (Ashraf Citation2009; Ashraf & Akram Citation2009; Li et al. Citation2012). Generally, AsA concentration in plants ranges from 2 to 25 mmol L−1 (Davey et al. Citation2000), and a relatively high concentration has been reported in different plants under saline stress conditions (Afzal et al. Citation2005; Akram et al. Citation2012). In the present study, AsA contents in the edible part of both carrot cultivars increased markedly under saline conditions. Similarly, while working with eggplant, Saleem et al. (Citation2012) also observed a significant increase in AsA contents in the fruits of two okra cultivars under different saline regimes. Such a salt-induced rise in AsA has also been observed in eggplant plants (Shaheen et al. Citation2013).
It is now well known that the high MDA contents are an indication of oxidation-induced lipid peroxidation in plants under adverse environmental conditions (M-Kalantari & Oloumi Citation2005; Akram et al. Citation2012; Hu et al. Citation2012). In the present investigation, an increase in MDA contents was observed in the roots of both carrot cultivars under all (50, 100, and 150 mM) salt regimes. Parallel to our present study, salt-induced increase in MDA contents was also observed in eggplant plants (Shaheen et al. Citation2013). However, the reverse was true in five turnip cultivars (Noreen et al. Citation2010b).
In response to adverse environmental conditions, production of nonenzymatic as well as enzymatic antioxidants in different plants varies considerably (Noreen et al. Citation2010b; Perveen et al. Citation2011, Citation2012; Ahmad et al. Citation2013). Of the enzymatic antioxidants, CAT, SOD, and POD are considered to be the key enzymes, which play an effective role in plants’ oxidative defense mechanism (Mittler Citation2002; Akram et al. Citation2012). In the present investigation, the activity of root POD, CAT, and SOD enzymes decreased markedly in both carrot cultivars under saline conditions. Recently, Hu et al. (Citation2012) have also observed a considerable inhibition in the activities of various antioxidant enzymes, e.g. SOD, CAT, and ascorbate peroxidase (APX) in bermudagrass (Cynodon dactylon). However, the reverse has been observed in other plant species with respect to these enzymes. For example, the activities of CAT and POD increased in eggplant plants under saline conditions (Shaheen et al. Citation2013). Similarly, salt stress-induced enhanced activities of CAT, POD, and SOD were observed in sunflower (Akram et al. Citation2012) and pistachio plants (Abbaspour Citation2012).
Tocopherols, nonenzymatic lipophilic antioxidants, can scavenge a variety of reactive oxygen species and free radicals particularly under stress conditions (Hollander-Czytko et al. Citation2005). In the present study, α-Toco contents in the edible part of both carrot cultivars remained almost unchanged under saline conditions except at 150 mM NaCl, where they increased markedly. Of both carrot cultivars, cv. DC-4 had considerably higher amount of α-Toco than that in cv. T-29 both under saline and nonsaline conditions. Generally, salt stress is known to enhance tocopherols in plants (Abbasi et al. Citation2007; Sorkheh et al. Citation2012). For example, in a set of nine pea cultivars, Noreen and Ashraf (Citation2009) observed a considerable increase in γ-and Δ-tocopherols under varying saline regimes. Analogous to our results, Tounekti et al. (Citation2011) also reported no change in α-Toco contents in common sage (Salvia officinalis L.) at 100 mM NaCl.
Overall, salt stress-induced high accumulation of GB, AsA, and MDA contents in the edible part of carrot, i.e. root. In contrast, total soluble proteins and activities of SOD, POD, and CAT were reduced due to saline stress in the edible part of carrot plants. α-Toco contents remained unchanged at different saline regimes used in the study. Of both carrot cultivars, cv. DC-4 accumulated relatively higher amount of GB, soluble proteins, and α-Toco contents than did cv. T-29, whereas, cv. T-29 had considerably higher amounts of AsA and MDA as well as activities of POD, SOD, and CAT than those in cv. DC-4 both under saline and nonsaline conditions. Overall, GB, AsA, and MDA contents increased, while enzymatic antioxidant activities decreased in both carrot cultivars under different saline regimes which indicated that enzymatic antioxidant metabolism was adversely affected in the edible part of carrot due to different saline regimes. So it can be suggested that the carrot is salt sensitive and its nutritional value in terms of antioxidants declines under salt stress.
Acknowledgments
The authors gratefully acknowledge the funding from the Pakistan Academy of Sciences (PAS) (Grant No. 5-9/PAS/4778) as well as the partial funding from the King Saud University, Riyadh, Saudi Arabia, through the research grant no. KSU-VPP-101.
References
- Abbas W, Ashraf M, Akram NA. 2010. Alleviation of salt-induced adverse effects in eggplant (Solanum melongena L.) by glycinebetaine and sugarbeet extracts. Sci Hort. 125:188–195. 10.1016/j.scienta.2010.04.008
- Abbasi A, Hajirezaei M, Hofius D, Sonnewald U, Voll LM. 2007. Specific roles of α- and γ-tocopherol in abiotic stress responses of transgenic tobacco. Plant Physiol. 143:1720–1738. 10.1104/pp.106.094771
- Abbaspour H. 2012. Effect of salt stress on lipid peroxidation, antioxidative enzymes, and proline accumulation in pistachio plants. J Med Plants Res. 6:526–529. 10.5897/JMPR11.1710
- Afzal I, Basra SMA, Ahmed N, Farooq M. 2005. Optimization of hormonal priming techniques for alleviation of salinity stress in wheat (Triticum aestivum L.). Caderno de Pesquisa Serie Biol. 17:95–109.
- Ahmad P, Ashraf M, Azooz MM, Rasool S, Akram NA. 2013. Potassium starvation-induced oxidative stress and antioxidant defense responses in Brassica juncea. J Plant Interact. doi:10.1080/17429145.2012.747629
- Akram NA, Ashraf M, Al-Qurainy F. 2011. Aminolevulinic acid-induced changes in yield and seed-oil characteristics of sunflower (Helianthus annuus L.) plants under salt stress. Pak J Bot. 43:2845–2852.
- Akram NA, Ashraf M, Al-Qurainy F. 2012. Aminolevulinic acid-induced changes in some key physiological attributes and activities of antioxidant enzymes in sunflower (Helianthus annuus L.) plants under saline regimes. Sci Hort. 142:143–148. 10.1016/j.scienta.2012.05.007
- Ashraf M. 2009. Biotechnological approach of improving plant salt tolerance using antioxidants as markers. Biotechnol Adv. 27:84–93. 10.1016/j.biotechadv.2008.09.003
- Ashraf M, Akram NA. 2009. Improving salinity tolerance of plants through conventional breeding and genetic engineering: an analytical comparison. Biotechnol Adv. 27:744–752. 10.1016/j.biotechadv.2009.05.026
- Backer H, Frank O, De Angells B, Feingold S. 1980. Plasma tocopherol in man at various times after ingesting free or ocetylaned tocopherol. Nutr Rep Int. 21:531–536.
- Bano S, Ashraf M, Akram NA, Al-Qurainy F. 2012. Regulation in some vital physiological attributes and antioxidative defense system in carrot (Daucus carota L.) under saline stress. J Appl Bot Food Qual. 85:105–115.
- Batool A, Ashraf M, Akram NA, Al-Qurainy F. 2013. Salt-induced changes in the growth, key physicochemical and biochemical parameters and enzyme activities, and levels of non-enzymatic anti-oxidants in cauliflower (Brassica oleracea L.). J Hort Sci Biotechnol. 88:231–241.
- Bradford MM. 1976. A rapid and sensitive for the quantitation of microgram quantities of protein utilizing the principle of protein–dye binding. Anal Biochem. 72:248–254. 10.1016/0003-2697(76)90527-3
- Carmak I, Horst JH. 1991. Effects of aluminum on lipid peroxidation, superoxide dismutase, catalase, and peroxidase activities in root tips of soybean (Glycine max). Physiol Plant. 83:463–468. 10.1111/j.1399-3054.1991.tb00121.x
- Chance M, Maehly AC. 1955. Assay of catalases and peroxidases. Methods Enzymol. 2:764–817.
- Colla G, Rouphael Y, Leonardi C, Bie Z. 2010. Role of grafting in vegetable crops grown under saline conditions. Sci Hort. 127:147–155. 10.1016/j.scienta.2010.08.004
- Davey MW, Van Montagu M, Inzé D, Sanmartin M, Kanellis A, Smirnoff N, Benzie IJJ, Strain JJ, Favell D, Fletcher J. 2000. Plant L-ascorbic acid: chemistry, function, metabolism, bioavailability and effects of processing. J Sci Food Agric. 80:825–860.
- Giannopolitis CN, Ries SK. 1977. Superoxide dismutase. I. Occurrence in higher plants. Plant Physiol. 59:309–314. 10.1104/pp.59.2.309
- Gibberd MR, Turner NC, Storey R. 2002. Influence of saline irrigation on growth, ion accumulation and partitioning, and leaf gas exchange of carrot (Daucus carota L.). Ann Bot. 90:715–724. 10.1093/aob/mcf253
- Grieve CM, Grattan SR. 1983. Rapid assay for determination of water soluble quaternary ammonium compounds. Plant Soil. 70:303–307. 10.1007/BF02374789
- Hollander-Czytko H, Grabowski J, Sandorf I, Weckermann K, Weiler EW. 2005. Tocopherol content and activities of tyrosine aminotransferase and cystine lyase in Arabidopsis under stress conditions. J Plant Physiol. 162:767–770. 10.1016/j.jplph.2005.04.019
- Hu L, Huang Z, Liu S, Fu J. 2012. Growth response and gene expression in antioxidant-related enzymes in two bermudagrass genotypes differing in salt tolerance. J Am Soc Hort Sci. 137:134–143.
- Jamil M, Lee DB, Jung KY, Ashraf M, Lee SC, Rha ES. 2006. Effect of salt (NaCl) stress on germination and early seedling growth of four vegetables species. J Cent Eur Agric. 7:273–282.
- Kumar S, Dhingra A, Daniell H. 2004. Plastid-expressed betaine aldehyde dehydrogenase gene in carrot cultured cells, roots, and leaves confer enhanced salt tolerance. Plant Physiol. 136:2843–2854. 10.1104/pp.104.045187
- Li Q, Li Y, Li C, Yu X. 2012. Enhanced ascorbic acid accumulation through overexpression of dehydroascorbate reductase confers tolerance to methyl viologen and salt stresses in tomato. Czech J Genet Plant Breed. 48:74–86.
- Maas EV, Hoffman GJ. 1977. Crop salt tolerance: current assessment. J Irrig Drain Div. 103:115–134.
- Mittler R. 2002. Oxidative stress, antioxidants and stress tolerance. Trends Plant Sci. 7:405–410. 10.1016/S1360-1385(02)02312-9
- M-Kalantari K, Oloumi H. 2005. Study the effects of CdCl2 on lipid peroxidation and antioxidant compounds content in Brassica napus. Iran J Sci Technol Trans A. 29:201–208.
- Mukherjee SP, Choudhuri MA. 1983. Implications of water stress-induced changes in the levels of endogenous ascorbic acid and hydrogen peroxide in Vigna seedlings. Physiol Plant. 58:166–170. 10.1111/j.1399-3054.1983.tb04162.x
- Neffati M, Sriti J, Hamdaoui G, Kchouk ME, Marzouk B. 2011. Salinity impact on fruit yield, essential oil composition and antioxidant activities of Coriandrum sativum fruit extracts. Food Chem. 124:221–225. 10.1016/j.foodchem.2010.06.022
- Noreen Z, Ashraf M. 2009. Assessment of variation in antioxidative defense system in salt treated pea (Pisum sativum L.) cultivars and its putative use as salinity tolerance markers. J Plant Physiol. 166:1764–1774. 10.1016/j.jplph.2009.05.005
- Noreen Z, Ashraf M, Akram NA. 2010a. Salt-induced modulation in some key physio-biochemical processes and their use as selection criteria in potential vegetable crop pea (Pisum sativum L.). Crop Pasture Sci. 61:369–378. 10.1071/CP09255
- Noreen Z, Ashraf M, Akram NA. 2010b. Salt-induced regulation of some key antioxidant enzymes and physio-biochemical phenomena in five diverse cultivars of turnip (Brassica rapa L.). J Agron Crop Sci. 196:273–285.
- Noreen Z, Ashraf M, Akram NA. 2012. Salt-induced regulation of photosynthetic capacity and ion accumulation in some genetically diverse cultivars of radish (Raphanus sativus L.). J Appl Bot Food Qual. 85:91–96.
- Perveen S, Shahbaz M, Ashraf M. 2011. Modulation in activities of antioxidant enzymes in salt stressed and non-stressed wheat (Triticum aestivum L.) plants raised from seed treated with triacontanol. Pak J Bot. 43:2463–2468.
- Perveen S, Shahbaz M, Ashraf M. 2012. Changes in mineral composition, uptake and use efficiency of salt stressed wheat (Triticum aestivum L.) plants raised from seed treated with triacontanol. Pak J Bot. 44:27–35.
- Saleem A, Ashraf M, Akram NA. 2011. Salt (NaCl)-induced modulation in some key physio-biochemical attributes in okra (Abelmoschus esculentus L.). J Agron Crop Sci. 197:202–213. 10.1111/j.1439-037X.2010.00453.x
- Saleem A, Ashraf M, Akram NA, Al-Qurainy F. 2012. Salinity-induced changes in the composition of some key enzymatic and non-enzymatic antioxidants, osmo-protectants, chlorophyll pigments and some inorganic elements in okra (Abelmoschus esculentus L.) fruit. J Hort Sci Biotechnol. 87:271–277.
- Schmidhalter U, Oertli JJ. 1991. Germination and seedling growth of carrots under salinity and moisture stress. Plant Soil. 132: 243–251.
- Shahbaz M, Ashraf M, Al-Qurainy F, Harris PJC. 2012. Salt tolerance in selected vegetable crops. Crit Rev Plant Sci. 31:303–320. 10.1080/07352689.2012.656496
- Shahbaz M, Ashraf M, Athar HR. 2008. Does exogenous application of 24-epibrassinolide ameliorate salt induced growth inhibition in wheat (Triticum aestivum L.). Plant Growth Regul. 55:51–64. 10.1007/s10725-008-9262-y
- Shaheen S, Naseer S, Ashraf M, Akram NA. 2013. Salt stress affects water relations, photosynthesis, and oxidative defense mechanisms in Solanum melongena L. J Plant Interact. 8:85–96. 10.1080/17429145.2012.718376
- Sorkheh K, Shiran B, Rouhi V, Khodambashi M, Sofo A. 2012. Salt stress induction of some key antioxidant enzymes and metabolites in eight Iranian wild almond species. Acta Physiol Plant. 34:203–213. 10.1007/s11738-011-0819-4
- Tounekti T, Iker H, Maren M, Habib K, Sergi M. 2011. Kinetin applications alleviate salt stress and improve the antioxidant composition of leaf extracts in Salvia officinalis. Plant Physiol Biochem. 49:1165–1176. 10.1016/j.plaphy.2011.07.011
- Volden J, Bengtsson GB, Wicklund T. 2009. Glucosinolates, L-ascorbic acid, total phenols, anthocyanins, antioxidant capacities and colour in cauliflower (Brassica oleracea L. ssp. botrytis); effects of long-term freezer storage. Food Chem. 112:967–976. 10.1016/j.foodchem.2008.07.018