Abstract
In order to investigate the effect of potassium (K) nutrition on secondary metabolism, Catharanthus roseus was treated by different concentrations and forms of K nutrition (KNO3 and K2SO4). The results showed that the concentration of applied K obviously affected plant growth and biomass accumulation, while K form had little influence. The concentration of alkaloids, including vinblastine in the leaves and catharanthine in the leaves or roots, had a significant increase, especially upon 80 and 100 mM K nutrition. The measurement of internal ion content using energy dispersive spectroscopy displayed that the increase of K element in leaves was tightly correlated with elevated alkaloid concentration. The transcript levels of G10h, Str, and Dat genes, encoding key enzymes in the alkaloid biosynthesis pathway, were significantly activated by applying P and tightly related to alkaloid accumulation. In conclusion, our results provided the physiological and molecular evidence that potassium could effectively regulate alkaloid metabolism in C. roseus.
1. Introduction
Catharanthus roseus (C. roseus), as an important medicinal plant, native to Madagascar, belonging to Apocynaceae family, produces more than 120 kinds of terpenoid indole alkaloids (TIAs) which are valued due to their wide spectrum of pharmaceutical effects (Pandey & Archana Citation2011). Among these indole alkaloids which have anticarcinogenic effects, vinblastine (vincaleukoblastine, VLB) present in leaves is widely used in the treatment of various types of leukemia, but occurs in very low concentrations of plant material (Tyler Citation1988). Nevertheless, it is still considered as the most interesting chemotherapeutic compound for the treatment of cancer and its current accessibility for clinical use (Pezzuto Citation1997).
Many external factors can enhance or retard the growth and development of C. roseus, such as temperature, moisture, radiation, nutrients, and gases (Jaleel et al Citation2008). Previous evidences suggested that mineral nutrition, according to the requirements of plants, was one of the most important factors of field technique (Łata Citation2007). One of the most important mineral nutrient, potassium (K) element that the plant needed, was very low (Akram Citation2012). But researches had shown that K was essential for many physiological processes, such as photosynthesis, translocation of photosynthates into sink organs, maintenance of turgescence, enzymatic activation, or decreasing excess ingestion of ions like Na and Fe in saline and flooded soils (Ashraf et al. Citation2011). It also had been reported that additional phosphorus and K had become essential now for getting profitable yields of cereals (Bakhsh et al. Citation1986).
The most significant effect of K fertilization for increasing VLB content was recorded when K was not applied in the chloride form. As opposite to this, Smith et al. (Citation1987) observed that with KCl being added into the suspension cultures, intra-cell accumulations of both catharanthine (CAT) and other indole alkaloids of C. roseus were getting higher (Smith et al. Citation1987). Biosynthesis of TIAs metabolites was according to the primary metabolic pathways () that were strictly regulated by expression of key enzyme genes in C. roseus (Rischer et al. Citation2006). A number of studies were reported about TIAs synthesis and the key enzymes in the pathway in C. roseus (Verpoorte et al. Citation2002; Hectors et al. Citation2007). In this study, these key enzymes in TIAs biosynthetic pathway involved strictosidine synthase (Str), deacetylvindoline 4-O-acetyltransferase (Dat), and Geraniol 10-hydroxylase (G10h). The transcript level of these enzymes genes had been discussed and researches showed that over-expression of these genes affected the biosynthesis of TIAs (Liu et al. Citation2007).
The aim of this research is to investigate the response to different forms and concentrations of K in C. roseus, particularly, in the variation of alkaloid contents, yield, mineral content, and gene expression. Furthermore, we chose some smaller seedlings and carried out a series of experiments to test and verify.
2. Materials and methods
2.1. Plant material and experimental treatments
The experiment was conducted in growth chambers (S10H, CONVIRON, Canada) set at 12/12 hours light/night regime at an irradiance of 450 µmol m−2 s−1 and 28°C during the day and 26°C at night and 65% relative humidity. C. roseus seeds were planted in pots using perlite as culture substrate (particle size 0.6–1.5 mm diameter), and kept moistened, being covered with a transparent sheet until the seeds had germinated. After germination, seedlings were irrigated with 1/2 strength Hoagland solution (pH 6.5, adjusted by addition of HCl/KOH). When four pairs of leaves had appeared (about one month), all the seedlings were randomized to the treatments.
For K treatment, seedlings were cultivated in the Hoagland nutrient solution containing K. The final concentrations of K were containing (10, 80, 100 mM) KNO3 or (5, 40, 50 mM) K2SO4. Every group was poured once every 2–3 days, and grasped the principles of a few times but a small amount. Each treatment was replicated three times.
Individual plants were harvested for morphological and physiological determinations 10 and 30 days after the treatments were applied. For the biomass measurements, three plants from each treatment were harvested after washing the sand from the roots, and drying off the whole plant at 80°C for 48 hours. Morphological measurements, height, root length, fresh weight, and dry weight were also measured.
2.2. Determination of alkaloid content
Dry leaf powder (0.3 g) was dissolved in 10 mL absolute methanol (analytical grade) for extraction of VLB, vindoline, and CAT. Low-frequency ultrasonication (250 W, 40 kHz) was used to extract the alkaloids for 20 min. The methanol extract was centrifuged at 8000 rmp for 10 min, concentrated to 1 mL and analyzed by high performance liquid chromatograph (HPLC) (Jasco, VG, England) equipped with a Waters Octadecylsilyl (ODS) C18 reversed-phase column (250 × 4.6 mm, 5 µm) and a photodiode-array detector at 220 nm. Sample injection volume was 10 µL at a flow-rate of 1.5 mL min−1.
2.3. Energy spectrum
The harvested seedlings were used to determine ion content. The leaf samples were first rinsed for about 10 s in approximately 500 mL of double-deionized water. Then, these samples were dried at 80°C for 48 hours. Dry leaves were ground and passed through a 5-mm mesh sieve. And the ion concentrations of carbon (C), nitrogen (N), phosphorus (P), sulfur (S), and K were determined with scanning electron microscopy and energy dispersive spectroscopy (SEM/EDS).
2.4. Ribonucleic acid (RNA) extraction, quantification, and real-time quantitative PCR
Total RNA was extracted from 50 to 100 mg samples (both leaves and roots) by TRIZOL reagent, and quantified by a NanoDrop ND-1000 spectrophotometer (NanoDrop Technologies) with absorbance at 260 nm and an ethidium bromide (EB) stained test agarose gel electrophoresis used to verify the quality. RNAs (2 µg) removed genomic deoxyribonucleic acid (DNA) were reverse-transcribed using random primers and the reaction mixture containing 1 µL 5× buffer, 2 µL 10 mM nucleotide triphosphate (NTP), 1 µL oligo (dT)18 primer, 1 µL RNase inhibitor, and 1 µL moloney murine leukemia virus (MMLV) was incubated for 1 hour at 42°C, and then incubated at 70°C for 5 min. At last, cDNA was cooled in ice water for 2 min. A 20-µL PCR amplification mixture contained 2 µL 10× buffer, 1.2 µL MgCl2, 2 µL dNTP, 0.5 µL synergy brands (SYBR), 0.1 µL Taq, 0.5 µL of each 10 µmol. L−1PF and PR primers, 0.5 µL cDNA (the equivalent of 25 ng total RNA) as template and diethylpyrocarbonate (DEPC)-treated water. The primers used in this work were as follows:
Str: F 5′-AAAATTCCCGATACTCCG-3′ and
R 5′-ACCAATGGGCACTTCCTT-3′
G10h: F 5′-TGTTTGTAGCAGGGACGGACAC-3′
and
R 5′-TGTTTGTAGCAGGGACGGACAC-3′
Dat: F 5′-AAACCCTCTTCTCCAACCCCTC-3′
and
R 5′-CTTCCACGAACTCAATTCCATC-3′
PCR was performed at 94°C for 5 min, then at 94°C for 30 s, 60°C for 30 s and 72°C for 30 s, 80°C reading plate for 1 s for 35 cycles, and remained at 72°C for 2 min. Reactions were repeated three times for each sample to ensure the reproducibility of the results. Actin gene was used as an internal control.
After PCR reaction, a melting curve was obtained by opticon version 3 and parameters were set as reading plate for 1 s every increasing 0.5°C in the range from 60 to 95°C. The comparative cycle threshold (CT) (ΔΔCT) method was used to analyze the relative transcript levels of the three genes for different experiment groups.
2.5. Statistical analysis
Results were subjected to analysis of variance (ANOVA) to determine the significant differences between treatments. When ANOVA was performed, Duncan's honestly significant difference post hoc tests were conducted to determine the differences between the individual treatments (SPSS 17.0, SPSS Inc., USA). SPSS was also used to calculate the Pearson's correlation coefficients.
3. Results
3.1. Effects of applied K nutrition on growth
As was shown, a series of physiological indicators were measured (). Plants height (p < 0.01), root length (p < 0.05), and biomass (p < 0.001) were all significantly reduced with the K dose increasing compared with the control group (CK). In addition, root length and biomass of C. roseus, respectively, rose from 9.88 ± 1.09, 2.74 ± 0.27 (control plants) to 13.25 ± 0.83, 10.68 ± 1.42 (KNO3 plants) and 4.22 ± 0.22, 3.09 ± 0.43 (K2SO4 plants) at 10 mM K but decreased at 80 and 100 mM K. It suggested that, in a certain extent, low concentration of K nutrition promoted the root and plant growth, but high concentration had some inhibitions for development.
Table 1. Biomass accumulation and morphological changes in C. roseus.
There were no significant differences in the biomass and other physiological indicators (p > 0.05) in C. roseus seedlings applied with two forms of K. But the K dose had observably effected on some morphological indicators. Besides, the biomass was strongly influenced by K type and K dose (T × D). Root/shoot ratio in the experimental group was higher than CK. In other words, K affected the development of C. roseus.
3.2. Effects of applied K nutrition on alkaloids
The accumulation of three alkaloids including vindoline, CAT, and VLB was analyzed (). The results suggested that contents of three alkaloids were enhanced in varying degrees. After a 10-day treatment by 100 mM K nutrient, there was a small increase of vindoline content in the leaves (). But, overall, the increase was not significant. Under the treatment by two forms of 10 mM K nutrition, VLB concentration was almost the same as the CK (0.16137 mg g−1) in the leaves (). Then, with K dose increasing, VLB content had a substantial enhancement, and especially at the 80 and 100 mM K, the contents, respectively, reached 0.592016 mg g−1 and 0.718028 mg g−1, which was 3–5 times more than CK. Corresponding, in the seedlings treated by K2SO4, CAT content in the leaves also had a certain increase compared to growth in KNO3 ().The data showed that CAT content in roots was also significantly improved, especially at 100 mM KNO3 (). In general, the shifty tendency was the same as VLB.
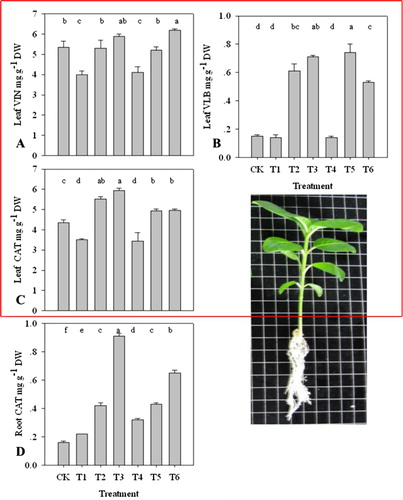
Correlation coefficient between the form and dose of K and alkaloids were analyzed (). There was a significant correlation (p < 0.001) between K form and CAT content in leaf or root, K dose and three alkaloids, and K type and K dose (T × D) and VLB in leaf or CAT in root. On the contrary, the correlations between K form and vindoline or VLB in leaf, and T × D and vindoline or CAT in the leaf were negative (p > 0.05).
Table 2. Correlation coefficient among alkaloid accumulations in leaves or roots of C. roseus, K type and K dose.
3.3. Energy spectrum
Elements contents in leaves surface were quantified in C. roseus ( and ). With the increase of K dose, K element was also increased in the leaf surface, and at 100 mM K nutrient, the concentration of K element reached to the maximum (12.59 ± 0.90, 11.20 ± 0.19). Concentrations of C, N, P, and S element were reduced with the increase of KNO3 ( and ), but enhanced with K2SO4 ( and ). C/N ratio rose at first, and then decreased under treatment of KNO3, while it was decreased all the time under K2SO4 (). It suggested that only K element content was always promoted by different forms of K nutrition.
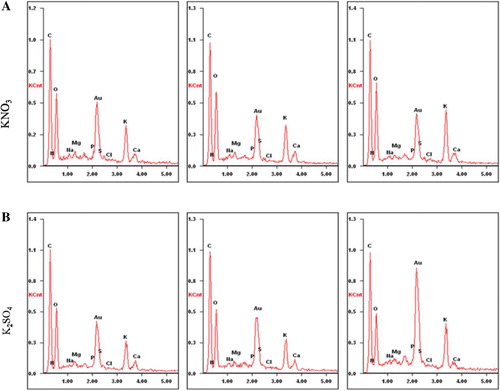
Table 3. The accumulation of nutrient elements in leaf surface of seedlings exposed to KNO3 or K2SO4.
Correlation between the C content and leaf CAT was negative, but it was significant between C/N ratio and leaf vindoline (). In addition, vindoline content in the leaves has more positive correlation with sulfur element. Obviously, none of the C, S, or C/N ratio had a regular affect on all the alkaloids.
Table 4. Correlations of alkaloid accumulation with leaf various element.
Total alkaloids significantly increased (p < 0.05, p < 0.01) with the K dose increasing. Relationship between K element content and alkaloids was positively correlated. Thus, K element's involvement in the synthesis of alkaloids was credible.
3.4. Relative quantitative polymerase chain reaction (PCR)
The transcript levels of genes in leaves, containing G10h, Str, and Dat, were measured under different treatments of K solution (). The data showed that a significant difference between treated group and CK in genes transcript level was observed. With the stress of K deepening, the transcript levels of G10h, Str, and Dat genes were all significantly promoted. Str gene transcript had an obvious augment at 40 mM KNO3 and 20 mM K2SO4, which were separately 10.447 and 15.542 times higher than CK. And the transcription level of Dat gene rose to the greatest degree at 80 mM KNO3. Thus, it was clear that K nutrition activated the high transcript level of TIAs pathway genes (G10h, Str, and Dat).
4. Verification experiments
Some C. roseus seedlings (15 days after germination) were used to verify the result that K nutrition promoted in the alkaloids accumulation. The effects of applied K nutrition (only KNO3) on alkaloid levels were shown in .
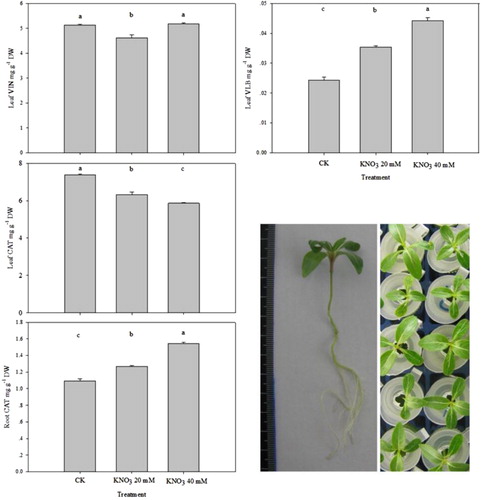
For K-fed plants, VLB contents significantly improved with K dose, compared to the CK. However, the variation of vindoline and CAT was not the same as the performance results of 30-day seedlings. It was not significant that K nutrition promoted vindoline accumulations and even inhibited CAT synthesis in the leaf. It possibly led from the synthesis of VLB causing excessive consumption for precursors. But overall, there was no doubt that K nutrition could improve the content of VLB in C. roseus.
5. Discussion and conclusion
Adequate K nutrition has important effects on the composition of harvested products and the quality of diet, especially under some stress (Ashraf et al. Citation2013). However, K (+) content in soils is usually limited, and so the crop yields are restricted. Currently, the evidences for the diverse functions of K from the molecular level to field performance had been increased. The role of K in stress signaling and stress mitigating, particularly in drought and salinity, is becoming an important topic (Cakmak Citation2005; Amtmann et al. Citation2008; Wang & Wu Citation2010).
In the study, additional K was helpful for plant growth and enhanced alkaloid accumulation, which possibly led from the fact that K nutrition promoted the growth of root. Nearly the same results were obtained by Fernández et al. (Citation2009) in soybean. On the other hand, adequate K improved well-balanced nutrition, which might activate the CAT synthesis in root and VLB content.
Alkaloid biosynthesis is regulated primarily at the expression level of gene (De Luca & Laflamme Citation2001). Str, Dat, and G10h have been identified in a selected number of genes, which control metabolites synthesis involved in the biosynthesis pathway of TIAs (Rischer et al. Citation2006). Fungal elicitors and methyl jasmonate (MeJa) had been reported to induce Str gene expression (Pasquali et al. Citation1992; Menke et al. Citation1999). The terminal step of vindoline biosynthesis is catalyzed by Dat. Dat gene is predominantly expressed in young leaves of mature plants and can be activated by light treatment (St-Pierre et al. Citation1998). Wang et al. (Citation2010) pointed out that the content of CAT was increased via overexpressing G10h genes in C. roseus hairy roots.
In the experiment, transcript levels of G10h, Str, and Dat genes were up-regulated especially at 80 mM KNO3 and 40 mM K2SO4. This change of gene expression was similar as alkaloid content.
Thus, it also proved that K enhanced accumulation of VLB by promoting root growth, gene expression, and the increasement of CAT content. The experiment of 15-day seedlings from the other side confirmed that K promoted the synthesis of VLB. Therefore, we could provide a rapid method for the increase of alkaloids which were estimated by analyzing the transcript level of genes in TIAs biosynthetic pathway.
Acknowledgments
This study was financially supported by the Fundamental Research Funds for the Central Universities (DL12CA02) and the Natural Science Foundation of Heilongjiang province (C201117).
References
- Akram M. 2012. Effect of potassium nutrition on solute accumulation, ion composition and yield of maize hybrids grown under saline conditions. J Plant Nutr. 36: 143–163.
- Amtmann A, Troufflard S, Armengaud P. 2008. The effect of potassium nutrition on pest and disease resistance in plants. Physiol Plant. 133: 682–691.
- Ashraf M, Afzal M, Ahmad R, Maqsood MA, Shahzad SM, Tahir MA, Akhtar N, Aziz A. 2011. Growth response of the salt-sensitive and the salt-tolerant sugarcane genotypes to potassium nutrition under salt stress. Arch Agron Soil Sci. 58: 385–398.
- Ashraf MY, Rafique N, Ashraf M, Azhar N, Marchand M. 2013. Effect of supplemental potassium (K + ) on growth, physiological and biochemical attributes of wheat grown under saline conditions. J Plant Nutr. 36: 443–458.
- Bakhsh A, Khattak J, Bhatti A. 1986. Comparative effect of potassium chloride and potassium sulfate on the yield and protein content of wheat in three different rotations. Plant Soil. 96: 273–277.
- Cakmak I. 2005. The role of potassium in alleviating detrimental effects of abiotic stresses in plants. J Plant Nutr Soil Sci. 168: 521–530.
- De Luca V, Laflamme P. 2001. The expanding universe of alkaloid biosynthesis. Curr Opin Plant Biol. 4: 225–233.
- Fernández F, Brouder S, Volenec J, Beyrouty C, Hoyum R. 2009. Root and shoot growth, seed composition, and yield components of no-till rainfed soybean under variable potassium. Plant Soil. 322: 125–138.
- Hectors K, Prinsen E, De Coen W, Jansen MA, Guisez Y. 2007. Arabidopsis thaliana plants acclimated to low dose rates of ultraviolet B radiation show specific changes in morphology and gene expression in the absence of stress symptoms. New Phytol. 175: 255–270.
- Jaleel CA, Gopi R, Manivannan P, Panneerselvam R. 2008. Soil salinity alters the morphology in Catharanthus roseus and its effects on endogenous mineral constituents. EurAsia J BioSic. 2: 18–25.
- Łata B. 2007. Cultivation, mineral nutrition and seed production of Catharanthus roseus (L.) G. Don in the temperate climate zone. Phytochem Rev. 6: 403–411.
- Liu D-H, Jin H-B, Chen Y-H, Cui L-J, Ren W-W, Gong Y-F, Tang K-X. 2007. Terpenoid indole alkaloids biosynthesis and metabolic engineering in Catharanthus roseus. J Integr Plant Biol. 49: 961–974.
- Menke FL, Parchmann S, Mueller MJ, Kijne JW, Memelink J. 1999. Involvement of the octadecanoid pathway and protein phosphorylation in fungal elicitor-induced expression of terpenoid indole alkaloid biosynthetic genes in Catharanthus roseus. Plant Physiol. 119: 1289–1296.
- Pandey N, Archana. 2011. Physiological and biochemical changes in Catharanthus roseus L. in response to boron nutrition. J Plant Nutr. 34: 1797–1810.
- Pasquali G, Goddijn OM, Waal A, Verpoorte R, Schilperoort R, Hoge JH, Memelink J. 1992. Coordinated regulation of two indole alkaloid biosynthetic genes from Catharanthus roseus by auxin and elicitors. Plant Mol Biol. 18: 1121–1131.
- Pezzuto JM. 1997. Plant-derived anticancer agents. Biochem Pharmacol. 53: 121–133.
- Rischer H, Oresic M, Seppanen-Laakso T, Katajamaa M, Lammertyn F, Ardiles-Diaz W, Van Montagu MC, Inze D, Oksman-Caldentey KM, Goossens A. 2006. Gene-to-metabolite networks for terpenoid indole alkaloid biosynthesis in Catharanthus roseus cells. Proc Natl Acad Sci USA. 103: 5614–5619.
- Smith JI, Smart NJ, Kurz WGW, Misawa M. 1987. The use of organic and inorganic compounds to increase the accumulation of indole alkaloids in Catharanthus roseus (L.) G. Don cell suspension cultures. J Exp Bot. 38: 1501–1506.
- St-Pierre B, Laflamme P, Alarco AM, De Luca V. 1998. The terminal O-acetyltransferase involved in vindoline biosynthesis defines a new class of proteins responsible for coenzyme A-dependent acyl transfer. Plant J. 14: 703–713.
- Tyler VE. 1988. Medicinal plant research: 1953–1987. Planta Med. 54: 95–100.
- Verpoorte R, Contin A, Memelink J. 2002. Biotechnology for the production of plant secondary metabolites. Phytochem Rev. 1: 13–25.
- Wang CT, Liu H, Gao XS, Zhang HX. 2010. Overexpression of G10H and ORCA3 in the hairy roots of Catharanthus roseus improves catharanthine production. Plant Cell Rep. 29: 887–894.
- Wang Y, Wu WH. 2010. Plant sensing and signaling in response to K + -deficiency. Mol Plant. 3: 280–287.