Abstract
We investigated the bioactive potential of fungal endophytes isolated from the roots of Glycine max (L.) Merr. and Cucumis sativus. Initial screening results showed that endophytes, (Chrysosporium pseudomerdarium, Aspergillus fumigates and Paecilomyces sp., Penicillium sp., Phoma glomerata, and Paecilomyces formosus) were promoting the growth attributes (shoot length, chlorophyll contents, and biomass) of mutant and wild-type rice. To know whether, these endophytes were producing bioactive chemical constituents; the endophytes were grown in potato dextrose, czapek broth, and ICI mediums that resulted in varying mycelia biomass. The advance chromatographic analysis of endophytic fungal culture filtrate showed gibberellins (GA) production namely GA1 (0.05–10.55 ngml−1), GA3 (0.48–9.0 ngml−1), GA4 (0.2–8.0 ngml−1), GA7 (1.4–6.5 ngml−1), and GA9 (0.03–1.05 ngml−1) while among endophytes, P. glomerata and P. formosus were highly potent in GAs production. Additionally, the endophyte's culture also contained indole-3-acetic acid (0.23–71.51 µgml−1). Furthermore, oxalic (0.009–0.3 mgl−1), quinic (0.019–0.22 mgl−1), malic (1.94–17.2 mgl−1), and citric (0.012–0.95 mgl−1) acids were also present in the endophytic cultures. The biochemical potential of endophytes greatly varied depending on nutrient source and pH, however, czapek broth rich in carbon revealed higher potential for bioactive chemical constituent's production. In conclusion, the current findings suggest that endophyte can play a vital role in essential crop plant growth by synthesizing a wide array of bioactive metabolites. Furthermore, an increased production of chemical constituents can be achieved by changing the in vitro growth conditions of endophytes
Introduction
Phytohormones are versatile signaling molecules affecting various physiological processes of plants development even at low concentration (Khan et al. Citation2012). Gibberellins (GAs) can stimulate cell division and elongation in plants while its exogenous application can improve plant growth under normal and stress conditions like salinity, drought, and temperature (Davičre & Achard Citation2013; Khan et al. Citation2013). Most important of all GAs are bioactive GAs which include GA1, GA3, GA4, and GA7. GAs production and regulation have been extensively elaborated in plants (Davičre & Achard Citation2013), while some fungal species have also been known to possess active GAs biosynthesis pathway. Among fungi, endophytes have been frequently overlooked for their potential to produce GAs and other plant growth-regulating compounds (Khan et al. Citation2012, Citation2013). Endophytic fungi comprise of fungal symbionts associated with plants living inside tissues without causing any disease symptoms (Mayerhofer et al. Citation2013). Endophytic fungi have been recently recognized to produce GAs and indole-3-acetic acid (IAA) under in vitro conditions.
Unlike bacteria, fungi do not have a well-established biosynthesis of IAA (Kumla et al. Citation2013); however, recently some fungi including endophytes were known to secrete it in their growing mediums (Khan et al. Citation2012; Waqas et al. Citation2012; Hoffman et al. Citation2013). IAA is a phytohormone known to play an essential role in cell elongation, division, and enlargement (Ishida et al. Citation2013; Kumla et al. Citation2013; Zhao et al. Citation2013). Both GAs and IAA are active in undertaking physiological process at cellular level inside plants (Davičre & Achard Citation2013; Ishida et al. Citation2013; Zhao et al. Citation2013). Organic acids production, on the other hand, is also an important property of microbes specially bacteria. The release of organic acid plays a vital role in microbial nutrition and physiology (Barros et al. Citation2013), while these can assist microbes in cycling of essential minerals from soil to plants, deactivating metal ions to stable chelating agents/complexes and regulating host–plant physiology (Barros et al. Citation2013; Yadava et al. Citation2013). Additionally, organic acids are pivotal in biodeterioration and weathering (Cai et al. Citation2013; Wei et al. Citation2013). Such prospective potentials of endophytes can be utilized in enhancing plant's resistance to biotic and abiotic stresses (Khan et al. Citation2012; Albermann et al. Citation2013; Cassán et al. Citation2013).
Many fungal/endophytes species for example Gibberella fujikuroi, Fusarium proliferatum, Fusarium konzum, Fusarium sacchari (Albermann et al. Citation2013) Sesamum indicum (Choi et al. Citation2005), Phaeosphaeria sp. L487 (Kawaide Citation2006), Penicillium citrinum (Khan et al. Citation2008), and Penicillium funiculosum (Khan et al. Citation2011) have been known to produce GAs. Endophytes have been mostly reported for their behavior to condone plant growth. They can influence key aspects of plant physiology and host's protection against biotic and abiotic stresses (Khan et al. Citation2013; Mayerhofer et al. Citation2013). Secretion of bioactive metabolites such as phytohormones by endophytes can extend greater benefits to the host plants even during extreme environmental conditions (Khan et al. Citation2013) while on the other hand, being eco-friendly these can be extradited for commercial application and use at industrial levels.
Production of plant hormones and biochemicals by microbes depends on the conditions like pH, temperature, incubation period, types of microbe, growth dynamics, and its internal physiology (Khan et al. Citation2012; Wei et al. Citation2013). Selection of optimal growth conditions is necessary to outline the strategies for industrial production of GAs and other bioactive metabolites on commercial level (Albermann et al. Citation2013). Assessment of such potential would satisfy dual benefits of improved crop growth and sustainable agricultural yields. However, these phenomenon's are little understood while nothing is known with regards to endophytic fungi (Urbanováa et al. Citation2011). In the present study, we aimed to evaluate the optimum media conditions required for endophyte's growth and production of phytohormones and organic acids. For this purpose, six different endophytic strains previously isolated from soybean and cucumber plants were selected and grown in three different nutrient and pH conditions.
Materials and methods
Selection of endophytic fungi, media, and mutant rice bioassay
To differentiate the growth promoting and biochemicals production ability of fungal endophytes cultured in different media, six endophytes known for GAs production namely Chrysosporium pseudomerdarium (EU823311), Aspergillus fumigates (EU823312), Paecilomyces sp. (EU823315), Penicillium sp. (JX111910), Phoma glomerata (JX111911), and Paecilomyces formosus (JQ013813) were selected (Hamayun et al. Citation2009a, Citation2009b; Waqas et al. Citation2012).
The endophytic fungal strains were grown for 10 days at 30°C at 120 rpm in (1) Czapek media (0.01 gl−1 Glucose; 0.01 gl−1 peptone; 0.0005 gl−1 KCl; 0.0005 gl−1 MgSO4; 0.000001 gml−1 FeSO4·7H2O; pH 7.3 ± 0.2), (2) potato dextrose broth (PDB; 0.02 gl−1 Dextrose; 0.0065 gl−1 potato infusion; pH 5.1 ± 0.2), and (3) Imperial chemical industry fungal culture broth (ICI) media (0.08 gl−1 glucose; 0.005 gl−1 MgSO4; 0.01 gl−1 KH2PO4; 0.01 gml−1 NH4NO3; pH 7.0 ± 0.2). Two hundred and fifty ml of each medium was added to 500 ml Erlenmeyer flask. All flasks along with media were sterilized at 1.1 kg/cm2 pressure (121°C) for 15 min. The media were inoculated with 10 mm potato dextrose agar (PDA) disk from master plates and grown for 10 days at 30°C at 120 rpm. The cultural media were filtered and fresh mycelia weights were measured with electronic balance. The remaining cultural filtrates were used for rice plant bioassay and quantification of metabolites (GAs, IAA, and organic acids).
The culture filtrate (CF) was centrifuged to separate the pure culture (50 ml) and mycelium. The GAs biosynthesis dwarf mutant Waito-C and normal phenotype Dongjin-byeo rice seeds were surface sterilized according to Khan et al. (Citation2011) and germinated (25°C in dark conditions; moisture content 74%). After germination, the rice seeds were transferred into autoclaved pots (0.008 gml−1 agar) as described by Khan et al. (Citation2012). Ten rice plants per treatment were grown in a growth chamber (day/night cycle: 14 h; 25°C C/10 h; 18°C; relative humidity 65%; light intensity1000 µEm−2 s−1 Natrium lamps) for two weeks. The freeze dried CF was diluted with 1 ml autoclaved double distilled water (DDW). Each pot received 10-µl of CF at two-leaf stage. All growth characteristics such as shoot length, fresh and dry weight, and chlorophyll content were recorded after one week of treatment.
GAs quantification in pure culture
CFs of all the six-selected strains in each media were quantified for GAs production. For this purpose, a very sensitive procedure was established from the protocols of Khan et al. (Citation2012); Waqas et al. (Citation2012); Urbanováa et al. (Citation2011) with some modifications to distinguish endogenous GAs from other compounds present in CF. Fifty ml of each CF was taken to extract, purify, and quantify GAs. The pH of the CF was adjusted to 2.5 using 6 N HCl. It was partitioned with ethyl acetate (EtOAc). Before partitioning, deuterated GAs internal standards (20 ng; [17, 17–2H2] GA1, GA3, GA4, GA8, GA12, and GA24) as a reference compound were added in the CF for quantification, reliability of our procedure, and compensation of analyte loss during purification. Tritiated Gas, i.e. [1, 2–3H2] GA9 and [1, 2–3H2] GA20 were also added (obtained from Prof. Lewis N. Mander, Australian National University, Canberra, Australia). The organic layer was vacuum dried and added with 60% methanol (MeOH) while the pH was adjusted to 8.0 ± 0.3 using 2 N NH4OH. After adjusting the pH of organic layer, it was proceeded to strong anion exchange chromatography and C18 solid phase extraction. Extraction of GAs was further processed for fractionation in reversed phase C18 high-performance liquid chromatography (HPLC). For chromatography 3.9 × 300 mm Bondapak, C18 Column (Waters corp., Milford, MA, USA) was used. Column was eluted at 1.5 ml/min having the following gradient: 0–5 min, isocratic 28% MeOH in 1% aqueous acetic acid; 5–35 min, linear gradient from 28% to 86% MeOH;35–36 min, 86%–100% MeOH; 36–40 min, and isocratic 100%MeOH. Total of 48 fractions of 1.5 ml were obtained. For further analysis these fraction vials were prepared for gas chromatography mass spectrometer with selected ion monitoring (GC/MS-SIM) (6890 N network GC system, and 5973 network mass selective detector; Agilent Technologies, Palo Alto, CA, USA). Before injection each fraction was methylated with ethereal diazomethane and then trimethylsilylated with N,O-Bis (trimethylsilyl) trifluoroacetamide (Sigma–Aldrich Co. LLC.). The silylating reagents are highly reactive and more volatile thus separated easily and make free derivatized GAs of interest, which are then introduced into a mass spectrometer. Sample of l µl for each GA was injected in 30 m × 0.25 mm i.d., 0.25 µm film thickness DB-1 capillary column (J & W Scientific Co., Folsom, CA, USA). The GC oven temperature was programed as such, for 1 min hold at 60°C, then rise at 15°C min−1 to 200°C followed by 5°C min−1 to 285°C (Table S1Footnote1). Helium carrier gas was maintained at a head pressure of 30 kPa. The GC was directly interfaced to a Mass Selective Detector with an interface and source voltage of 70 eV and a dwell time of 100 ms. First trial (full scan mode) and three major ions of the supplemented 17, 17-D2- GAs internal standards (HS Tariff No. 29321900) obtained from Prof. Lewis N. Mander, Austrlian National University, Canberra, Australia, and fungal GAs were monitored simultaneously (Table S2Footnote1). The retention time was determined using hydrocarbon standards to calculate the KRI (Kovats Retention Index) value. The retention times and mass spectrum of both internal standards and endogenous GAs were also compared to ensure compound identification. While the GAs quantification was based on the peak area ratios of non-deuterated (extracted) GAs to deuterated GAs and expressed as nano gram per milliliter (see Tables S1 and 2 for GC-MS/SIM conditions and fragmentation pattern for GAs analysis).
IAA acid determination by HPLC in vitro condition
For IAA determination in vitro condition, strains isolated from cucumber and soybean were grown in Czapek, PDB, ICI medium supplemented with Tryptophan (100 µg mlFootnote1), and without tryptophan. Endophytes were allowed to grow for one week at 28°C, in a shaker (120 rpm). HPLC chromatograms were produced by injecting 20 µl of the filtered extract into a 5 µm reverse phase column (Waters Associates µBondapak C18, 250 mm × 4 mm) in a Waters Associates liquid chromatograph. Samples were analyzed under isocratic conditions with MeOH and water (80:20 v/v) as separation solvent, at a flow rate of 1.0 ml/min. Elutes were detected by a differential ultraviolet detector at 280 nm. IAA was quantified by reference to the peak area obtained for the IAA standard.
Quantification of organic acids
The HPLC analysis for quantification of organic acids was performed with Waters Co. 600E model comprising Refractive Index Detector (RI, model 410). The regression equation was obtained by using dilution of 10–100 mg l−1 of standards purchased from Bio-Rad Pacific Ltd Hong Kong and Sigma–Aldrich St Louis, MO, USA. These internal standards were also used for the purpose to confirm the identities of organic acids and experimental recovery of each compound in cultural filtrate. The recovery was calculated by dividing the determined amount by supplemented amount and multiplying by 100%. During quantification of organic acids, the sensitivity of detection was calculated by the limit of detection and limit of quantitation as signal-to-noise ratio according to the manufacturer instructions. For ultimate sensitivity, the HPLC system was maintained with a smooth delivery system and using HPLC grade solvent to minimize the baseline noise. Furthermore, the injection volume of 20 µl is quite enough to increase the sensitivity by increasing the peak signal. Before analysis, Dowex 50 W-X8 (BDH; 20–50 US mesh) was added to each fungal culture broth and shaken for 15 min to remove salt by adsorbing cations and obtain pure acids. The cultural filtrate was then passed through a 0.22-µm syringe filter (cellulose acetate, VWR) and injected (20 µl). The isocratic conditions for HPLC analysis were: mobile phase: 0.005 M H2SO4, flow rate 0.6 ml/min, and column temperature 65°C (PL Hi-Plex H column [7.7 mm × 300 mm] specially designed for organic acids analysis). Organic acids were quantified in endophytes culture broth by direct interpolation of the peak area obtained for the quinic, maleic, tartaric, citric, succinic, and oxalic acids from the corresponding regression equation of the standards.
Statistical analysis
All experiments were independently conducted three times. The data from the effect of endophytes grown in different media on rice growth experiment were subjected to two-way analysis of variance (ANOVA). The results of bioactive secondary metabolites quantification, and endophytes growth in different culture broth were analyzed with one-way ANOVA. Onward Duncan multiple range test (DMRT using SAS version 9.3) was applied to sort out the significant difference among treatment means at P < .05 and P < .01. The values are the means ± standard deviation (SD).
Results
Endophyte growth in different nutrient mediums
Three different nutrient mediums namely PDB, ICI, and Czapek broth were used to culture the isolated endophytes. After the inoculation period of endophytes, the pH of these medium varied greatly. The Czapek broth slightly increased the pH to weak alkaline () except Penicillium sp. and P. formosus. However, in case of ICI and PDB mediums, the pH reduced two-folds resulting in higher acidity. While it was also observed that the pH varies with different nutrient source and type of endophyte. The strains Penicillium sp, P. formosus, A. fumigates, and C. pseudomerdarium produced significantly higher fungal biomass in Czapek media (). Contrarily, P. glomerata and Paecilomyces sp. produced significantly higher amount of fungal biomass in PDB which was followed by ICI.
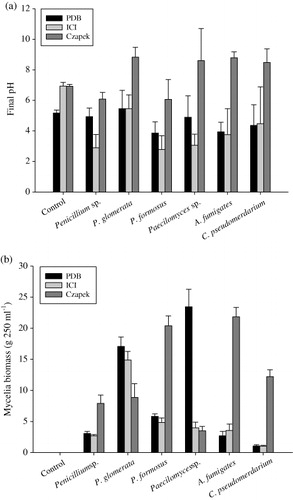
Effect of different endophytes on rice plant growth
Since, the strains were bioactive enough to produce phytohormones during their growth in different media; therefore, we also assessed their effects on the growth of Waito-C and Dongjin-byeo. Waito-C rice variety is a dy mutant with dwarf phenotypes because of suppressed C13-hydroxylation pathway for GAs biosynthesis (Khan et al. Citation2012). Dongjin-byeo, on the other hand, is a normal rice variety with active GAs biosynthesis pathway. It was hypothesized that any presence of bioactive metabolites will not only increases the shoot growth of dwarf mutant but will also improve the growth of normal rice variety. The application of different endophyte's CF belonging to different media has different growth-promoting effects. Overall, CF of all endophytes grown in Czapek media showed growth-promoting effect both on mutant rice Waito-C and normal cultivar Oryza sativa L. cv Dongjin-byeo ( and ).
Table 1. Effects of different fungal endophyte's CF treatment on the shoot length (cm), shoot fresh and dry weight (gm), and chlorophyll content (SPAD) of GAs mutant Waito-C rice.
Table 2. Effects of different fungal endophyte's CF on the shoot length (cm), shoot fresh and dry weight (gm) and chlorophyll content (SPAD) of normal phenotype rice cultivar (Dongjin-byeo).
Among endophytes, P. formosus inoculated in Czapek media has significantly increased the shoot length, chlorophyll content, and shoot biomass of Waito-C and Dongjin-byeo as compared to the control ( and ). P. glomerata inoculated in Czapek media has also significantly promoted the physiological characteristics of Waito-C and Dongjin-byeo. The Penicillium sp. only influences the shoot fresh and dry weight, and chlorophyll content when inoculated in Czapek media and no significant results were recorded when inoculated in ICI and PDB. Similarly, endophytes C. pseudomerdarium when inoculated in Czapek media has significantly increased the shoot length, chlorophyll content, shoot fresh weight of Waito-C, and shoot length in Dongjin-byeo as compared to control DDW-treated plants ( and ). A. fumigates grown in Czapek media has also promoted the shoot length, dry weight, and chlorophyll content in Waito-C and all growth parameters in Dongjin-byeo. However, Paecilomyces sp. inoculated in Czapek media has significant effects on growth attributes of Waito-C and shoot length, shoot fresh weight, and chlorophyll content of Dongjin-byeo.
Endophytic potential for GAs and IAA production in different media
Upon increased growth of mutant and normal rice line, we assessed the endophytic culture for phytohormonal analysis. The results showed the presence of physiological active and inactive GAs (GA1, GA3, GA4, GA7, and GA9) in CFs of fungal endophytes. Since the nutrient and pH conditions of each fungal growth medium were different, therefore, we observed a different GAs profile in six endophytes (). The quantification of GAs was significantly higher in Czapek media for almost all endophytes as compared to ICI and PDB (). Contrarily, the PDB and ICI did not present any significant GA's production ability. The highest level of GA's accumulation was recorded by P. formosus in Czapek media followed by P. glomerata and Penicillium sp. Five different GAs in Czapek media were found in the CF of P. formosus. Among physiologically active GAs, GA1, GA3, GA4, GA7, and GA9 were detected. P. glomerata produced 1.97 and 2.64-fold higher quantity of GA1 and GA3 in Czapek media respectively. Additionally, in Penicillium sp. GA1 was detected only in Czapek media while GA3 was 2.28 fold higher in Czapek media as compared to other growth mediums. The Paecilomyces sp. repeated the same trend and presented significant level of GAs accumulation in Czapek media as compared to PDB and ICI. The bioactive GAs detected in the CF were GA1, GA3, GA4, GA7, and GA9. However, C. pseudomerdarium had 4.61 (GA3) and 3.17 fold (GA9) higher GAs production ability as compared to other endophytes.
Table 3. Gibberellins (ngml−1) and indole acetic acid (µgml−1) production by fungal endophytes growing in different mediums.
To validate if the endophytes are capable of IAA production or not, the endophytes were grown in media with or without tryptophan and HPLC analysis was carried out to determine IAA production. All the selected strains were active in their ability to produce IAA (). Previously, some of these strains were unexplored for quantification of IAA. The fungal endophytes produced high amount of IAA in Czapek media when compared to ICI and PDB. Penicillium sp., on the other hand, produced higher IAA in PDB which was 18.68% and 409.37% more than the Czapek and ICI media respectively. The P. formosus was highest in IAA production as compared to Penicillium sp. and P. glomerata from cucumber (). The fungal endophyte C. pseudomerdarium produced significantly higher IAA followed by Paecilomyces sp. and A. fumigates. The IAA results revealed that the endophytes isolated from soybean produced significantly higher amount of IAA in Czapek media than the ICI and PDB.
Organic acid production capability in different media
The CF of endophytes was subjected for quantification of oxalic, quinic, tartaric, malic, citric, and succinic acids. The result showed that ICI medium was active to yield highest quantity and diversity of organic acids. The analysis revealed that the CF of Penicillium sp. contained oxalic, quinic, malic, citric, and succinic acids while P. glomerata and P. formosus were found to produce quinic, malic, citric, oxalic, and tartaric respectively. The organic acid analysis also showed that all three endophytic strains isolated from cucumber have the ability to produce malic acid and its quantity was significantly higher in ICI medium than Czapek and PDB (). However, the amount of malic acid varied from endophyte to endophyte as P. formosus was highest followed by Penicillium sp. and P. glomerata. Conversely, P. glomerata and P. formosus (except quinic acid) produced organic acids in ICI but not in Czapek and PDB.
Table 4. Organic acids (mgl−1) production in different microbial media by fungal endophytes isolated from cucumber and soybean plants.
Among endophytes isolated from soybean, A. fumigates was detected for higher quantity and diversity of organic acids as compared to other () namely quinic, tartaric, malic, and citric acids followed by C. pseudomerdarium which produced only oxalic and malic acid. Moreover, these organic acids were found in different quantity in different media. C. pseudomerdarium and A. fumigates produced malic acid 616% and 494% higher in ICI than the PDB respectively. Interestingly, none of the strains isolated from soybean produced malic acid in Czapek media.
Discussions
We selected three most commonly used media (Czapek, PDB, and ICI) for endophyte growth and bioactive metabolites production. Czapek and PDB are the simplest in formulation and support a broad range of fungal species growth. Moreover, ICI is the chemically defined media and may be the best source for isolation of secondary metabolites. Though all the three media were different in composition, however, the criteria for selection were based on the optimum production of phytohormones i.e. GAs, IAA, and organic acids. Among the three media, Czapek revealed the marked differences in production of most desirable phytohormones, i.e. bioactive GAs and IAA. The possible reason for the optimum production of phytohormones in Czapek media may be the low amount of glucose as our results confirm with Barboráková et al. (Citation2012). Barboráková et al. (Citation2012) found that an increased concentration of glucose (up to 3%) had a positive impact on biomass growth of fungi, but it negatively influenced the production of secondary metabolites. On the other hand, Czapek media supported a large number of different species of fungi (Khan et al. Citation2008). The presence of peptone in the medium (as in Czapek) seems to be another factor in high yield of phytohormones which contribute to its rich nutritive composition and more efficient pH buffering (Rossi & Oliveira Citation2011). The Czapek and ICI media share the same source of carbon though varies in amount and dextrose in PDB. In ICI medium, NH4NO3 is used as a nitrogen source which is different from Czapek and PDB. The main reason of ICI usually used for the fungal growth is the inorganic sources of nitrogen, NH4+or NO3−, ammonium being the main source, and most of the fungi can metabolize because enzymes involved in its assimilation are present (Rossi & Oliveira Citation2011). It was assumed that endophytes can also utilize the ammonium of nitrogen, however, in present case, the nitrogen presence effected phytohormones productions.
Albermann et al. (Citation2013) reported that GAs in fungi are synthesized by the consequent intermediates like geranylgeranyl diphosphate (GGDP), ent-copalyl diphosphate (CDP), ent-kaurene, and ent-kaurenoic acid which led the way to GA12-aldehyde production and this is identical in higher plants and G. fujikuroi (; Albermann et al. (Citation2013). Following conversion to GA12-aldehyde, the pathways in plants and the fungi differs. In fungi, GA12-aldehyde is 3 β-hydroxylated to GA14-aldehyde and then oxidized to form GA14. The subsequent conversion of GA14 to GA4 is comparable to the production of GA9 and GA20 in plants (Peters Citation2013). Desaturation of GA4 results in the formation of GA7 and then GA3 – which is the main product in G. fujikuroi. GA1 is formed as a minor product by 13-hydroxylation of GA4 and is not converted to GA3 (Albermann et al. (Citation2013). This pathway is also comparable to what we quantified in endophytes except the GA14 (see Table S2 for the GC/MS-SIM fragmentation pattern of GAs analysis; ). Previously, strains of Penicillium like Penicillium citrinum (Khan et al. Citation2008), Penicillium sp. MH7 (Hamayun et al. Citation2010), Penicillium minioluteum LHL09, and Penicillium funiculosum (Khan et al. Citation2011) have been found to produce various physiologically active and inactive GAs in their growing culture mediums. Troncoso et al. (Citation2010) showed that strains of Fusarium sacchari, F. konzum, and F. subglutinans have GAs biosynthesis gene cluster. Khan et al. (Citation2011) detected physiologically active GAs (GA1, GA3, and GA4) in the CF of P. funiculosum and P. minioluteum through GC/MS-SIM. Khan et al. (Citation2008) also reported that endophytic fungus P. citrinum and P. commune can produce GAs in various quantities. A similar report was also shown by Hamayun et al. (Citation2010) that Penicillium can produce GAs. Thus, our results do conform to the previous studies which suggest the production of phytohormones by endophytes.
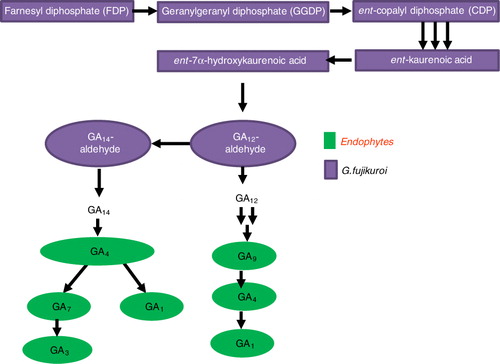
IAA is the phytohormone which is involved in plant stem and root growth regulation. The fabrication ability of IAA is widespread among soil- and plant-associated microorganisms (Kumla et al. Citation2013; Sukumar et al. Citation2013). We found this ability in all of our selected isolated endophytes strains from cucumber and soybean. The production of phytohormones like IAA by endophytes is an important property which plays a final role in soil fertility by expending the roots in search of nutrient. That is why that even in an extreme condition like in salinity and drought they offer protection by enhancing different cellular defense systems (Sukumar et al. Citation2013; Kumla et al. Citation2013). Plant usually synthesized IAA from the amino acid precursor tryptophan (Zhao et al. Citation2013). To identify that the selected isolated strains produce IAA through tryptophan dependent or independent pathway, we grow in PDB, ICI, and Czapek media with or without tryptophan. Our HPLC analysis revealed the existence of tryptophan dependent pathway in IAA production for all fungal endophytes used in this study. In current investigation, the endophytes grown in Czapek media amended with tryptophan produced highest quantity of IAA and these results are in agreement with Kumla et al. (Citation2013) and Yu et al. (Citation1970). Furthermore, the high concentration of glucose in ICI media resulted in decreasing the pH and consequently causing decrease in GAs and IAA production, same effect was also reported by Rossi & Oliveira (Citation2011) when they determine the secondary metabolites production.
Organic acids have an important role in fungal nutrition and physiology apart from their possible utilization as carbon and energy sources, which include contributions to intracellular osmotic potential, charge balance, and pH homeostasis (Li et al. Citation2012; Barros et al. Citation2013). In addition, the production of metal-complexing organic acids assists both essential metal and anionic nutrition of fungi and plants via solubilization of phosphate and sulfate, from insoluble metal containing substances, including salts and minerals (Cai et al. Citation2013; Wei et al. Citation2013). Organic acid production is wide spread in fungi with factors affecting biosynthesis including carbon and nitrogen sources and changes in pH. For example, nitrate acts as a nitrogen source and maintain the pH to enhance oxalate production by S. rolfsii. Usually HPLC is used for the analysis of organic acid in a pure cultural filtrate and we found different organic acids in cultural filtrate of all our selected strains except in Paecilomyces sp. from soybean (Carballo et al. Citation2013). Microorganisms producing organic acids have an important role in mineral solubilization; most important example is mineral phosphate mineralization. In crops even when inorganic phosphatic fertilizer is applied it immobilized rapidly and becomes unavailable to plants. The production of organic acids results in the acidification of surroundings and as a result P may be released by proton substitution for Ca2 + from mineral sources. This may be one of the supporting reasons for enhancing the growth of crop plants in stress like salinity and drought by endophytes.
A plethora of important and chemically diverse secondary metabolites are derived from endophytic fungi (Higginbotham et al. Citation2013). The growth as well as secondary metabolites production of different species of fungi varies from medium to medium (Rabbani et al. Citation2011; Rodrigues et al. Citation2011; Kautharapu et al. Citation2013). To enhance the production of these secondary metabolites, a reliable and optimum growth conditions are needed for microbes (Moges et al. Citation2012; Sathiyanarayanan et al. Citation2013). Nitrogen and carbon are the most important element for the growth and secondary metabolites production of endophytic fungi. The carbon to nitrogen ratio may contribute in controlling the pH and hence to increase the secondary metabolites production (Rossi & Oliveira Citation2011).
Conclusions
Based upon the present results, Czapek medium was revealed the best medium for the production of bioactive GAs, IAA, and maintaining higher biomass for endophytic fungi. From the course of this study, we also concluded that Czapek media with low quantity of glucose resulted in high pH and yield of bioactive GAs, IAA, and fresh mycelia weight. Contrarily, ICI with high amount of glucose yielded in low pH and higher quantity of organic acids. Czapek media needs further study to assess the gradient concentration of its compositional nutrients for diverse array of phytohormones like salicylic acid, abscisic acid, kinetin etc.
Supplemental_Material.doc
Download MS Word (43 KB)Acknowledgements
This study was supported by Korean Ministry of Environment through ‘The Eco-Innovation Project’. This study was supported by Kyungpook National University Research Fund, 2013.
Notes
1. Supplemental Content may be viewed online at http://dx.doi.org/10.1080/17429145.2013.860562
References
- Albermann S, Elter T, Teubner A, Krischke W, Hirth T, Tudzynski B. 2013. Characterization of novel mutants with an altered gibberellin spectrum in comparison to different wild-type strains of Fusarium fujikuroi. Appl Microbiol Biotechnol. 97:7779–7790. 10.1007/s00253-013-4917-7
- Barboráková Z, Labuda R, Häubl G, Tančinová, D. 2012. Effect of glucose concentration and growth conditions on the fungal biomass, pH of media and production of Fumagillin by a non-pathogenic strain Penicillium scabrosum. J Microbiol Biotechnol Food Sci. 1:466–477.
- Barros L, Pereira C, Ferreira ICFR. 2013. Optimized analysis of organic acids in edible mushrooms from Portugal by ultra fast liquid chromatography and photodiode array detection. Food Anal Methods. 6:309–316. 10.1007/s12161-012-9443-1
- Cai L, Xiao HR, Huang SM, Li H, Zhou GT. 2013. Solubilization of magnesium-bearing silicate minerals and the subsequent formation of glushinskite by Aspergillus niger. Geomicrobiol J. 30:302–312. 10.1080/01490451.2012.688924
- Carballo S, Zingarello FA, Maestre SE, Todoli JL, Prats MS. 2013. Optimisation of analytical methods for the characterization of oranges, clementines and citrus hybrids cultivated in Spain on the basis of their composition in ascorbic acid, citric acid and major sugars. Int J Food Sci Tech. doi:10.1111/ijfs.12289
- Cassán F, Vanderleyden J, Spaepen S. 2013. Physiological and agronomical aspects of phytohormone production by model plant-growth-promoting rhizobacteria (pgpr) belonging to the genus Azospirillum. J Plant Growth Regul. doi:10.1007/s00344-013-9362-4
- Choi WY, Rim SO, Lee JH, Lee JM, Lee IJ, Cho KJ, Rhee IK, Kwon JB, Kim JG. 2005. Isolation of gibberellins producing fungi from the root of several Sesamum indicum plants. J Microbiol Biotechnol. 15:22–28.
- Davičre JM, Achard P. 2013. Gibberellin signaling in plants. Development. 140:1147–1151. 10.1242/dev.087650
- Hamayun M, Khan SA, Iqbal I, Ahmad B, Lee IJ. 2010. Isolation of a gibberellin-producing fungus (Penicillium sp. MH7) and growth promotion of crown daisy (Chrysanthemum coronarium). J Microbiol Biotechnol. 20:202–207.
- Hamayun M, Khan SA, Iqbal I, Na CI, Khan AL, Hwang YH, Lee BH, Lee IJ. 2009a. Chrysosporium pseudomerdarium produces gibberellins and promotes plant growth. J Microbiol. 47:425–430. 10.1007/s12275-009-0268-6
- Hamayun M, Khan SA, Khan MA, Khan AL, Kang SM, Kim SK, Joo GJ, Lee IJ. 2009b. Gibberellin production by pure cultures of a new strain of Aspergillus fumigatus. World J Microbiol Biotechnol. 25:1785–1792. 10.1007/s11274-009-0078-3
- Higginbotham SJ, Arnold AE, Ibañez A, Spadafora C, Coley PD, Kursar TA. 2013. Bioactivity of fungal endophytes as a function of endophyte taxonomy and the taxonomy and distribution of their host plants. PLoS ONE. 8:e73192. 10.1371/journal.pone.0073192
- Hoffman MT, Gunatilaka MK, Wijeratne K, Gunatilaka L, Arnold AE. 2013. Endohyphal bacterium enhances production of indole-3-acetic acid by a foliar fungal endophyte. PLoS ONE. 8:e73132. 10.1371/journal.pone.0073132
- Ishida Y, Nakamura A, Mitani Y, Suzuki M, Soeno K, Asami T, Shimada Y. 2013. Comparison of indole derivatives as potential intermediates of auxin biosynthesis in Arabidopsis. Plant Biotech. 30:185–190. 10.5511/plantbiotechnology.13.0128c
- Kautharapu KB, Rathmacher J, Jarboe LR. 2013. Growth condition optimization for docosahexaenoic acid (DHA) production by Moritella marina MP-1. Appl Microbiol Biotechnol. 97:2859–2866. 10.1007/s00253-012-4529-7
- Kawaide H. 2006. Biochemical and molecular analysis of gibberellins biosynthesis in fungi. Biosci Biotech Biochem. 70:583–590. 10.1271/bbb.70.583
- Khan AL, Hamayun M, Kang SM, Kim YH, Jung HY, Lee JH, Lee IJ. 2012. Endophytic fungal association via gibberellins and indole acetic acid can improve plant growth under abiotic stress: an example of Paecilomyces formosus LHL10. BMC Microbiol. 12. doi:10.1186/1471-2180-12-3
- Khan AL, Hamayun M, Kim YH, Kang SM, Lee IJ. 2011. Ameliorative symbiosis of endophyte (Penicillium funiculosum sp. LHL06) under salt stress elevated plant growth of Glycine max L. Plant Physiol Biochem. 49:852–862. 10.1016/j.plaphy.2011.03.005
- Khan SA, Hamayun M, Yoon HJ, Kim HY, Suh SJ, Hwang SK, Kim JM, Lee IJ, Choo YS, Yoon UH, et al. 2008. Plant growth promotion and Penicillium citrinum. BMC Microbiol. 8:231–239. 10.1186/1471-2180-8-231
- Khan AL, Hussain J, Al-Harrasi A, Al-Rawahi A, Lee IJ. 2013. Endophytic fungi: resource for gibberellins and crop abiotic stress resistance. Crit Rev Biotechnol. 1–13. doi:10.3109/07388551.2013.800018 10.3109/07388551.2013.800018
- Kumla J, Suwannarach N, Bussaban B, Matsui K, Lumyong S. 2013. Indole-3-acetic acid production, solubilization of insoluble metal minerals and metal tolerance of some sclerodermatoid fungi collected from northern Thailand. Ann Microbiol. doi:10.1007/s13213-013-0706-x
- Li HP, Yeager CM, Brinkmeyer R, Zhang S, Ho YF, Xu C, Jones WL, Schwehr KA, Otosaka S, Roberts KA, et al. 2012. Bacterial production of organic acids enhances H2O2-dependent Iodide oxidation. Environ Sci Technol. 46:4837–4844. 10.1021/es203683v
- Mayerhofer MS, Kernaghan G, Harper KA. 2013. The effects of fungal root endophytes on plant growth: a meta-analysis. Mycorrhiza. 23:119–128. 10.1007/s00572-012-0456-9
- Moges F, Prabhakar T, Sankar G, Latha S, Ramana T. 2012. Optimization of media for production of bioactive compounds by Streptomyces parvullus SS23/2 isolated from marine algae in the Bay of Bengal, India. Ethiop J Biol Sci. 11:1–11.
- Peters RJ. 2013. Gibberellin phytohormone metabolism. In Bach TJ, Rohmer M, editors. Isoprenoid synthesis in plants and microorganisms: new concepts and experimental approaches. New York: Springer; p. 233–249.
- Rabbani N, Bajwa R, Javaid A. 2011. Influence of culturing conditions on growth and sporulation of Drechslera hawaiiensis, the foliar blight pathogen of Marsilea minuta L. Afr J Biotechnol. 10:1863–1872.
- Rodrigues C, Vandenberghe LP, de Oliveira J, Soccol CR. 2011. New perspectives of gibberellic acid production: a review. Cri Rev Biotechnol. 32:263–273. doi:10.3109/07388551.2011.615297
- Rossi MJ, Oliveira VL. 2011. Growth of the ectomycorrhizal fungus Pisolithus microcarpus in different nutritional conditions. Brazil J Microbiol. 42:624–632. 10.1590/S1517-83822011000200027
- Sathiyanarayanan G, Gandhimathi R, Sabarathnam B, Kiran SG, Selvin J. 2013. Optimization and production of pyrrolidone antimicrobial agent from marine sponge-associated Streptomyces sp. MAPS15. Bioprocess Biosyst Eng. doi:10.1007/s00449-013-1023-2
- Sukumar P, Legué V, Vayssières A, Martin F, Tuskan GA, Kalluri UC. 2013. Involvement of auxin pathways in modulating root architecture during beneficial plant–microorganism interactions. Plant Cell Environ. 36:909–919. 10.1111/pce.12036
- Troncoso C, González X, Bömke C, Tudzynski B, Gong F, Hedden P, Rojas MC. 2010. Gibberellin biosynthesis and gibberellin oxidase activities in Fusarium sacchari, Fusarium konzum and Fusarium subglutinans strains. Phytochem. 71:1322–1331. 10.1016/j.phytochem.2010.05.006
- Urbanováa T, Tarkowskáa D, Strand M, Hidden P. 2011. Gibberellins–terpenoid plant hormones: biological importance and chemical analysis collect. Czech Chem Commun. 76:1669–1686. 10.1135/cccc2011098
- Waqas M, Khan AL, Kamran M, Hamayun M, Kang SM, Kim YH, Lee IJ. 2012. Endophytic fungi produce gibberellins and indoleacetic acid and promotes host-plant growth during stress. Molecules. 17:10754–10773. 10.3390/molecules170910754
- Wei Z, Liang X, Pendlowski H, Hillier S, Suntornvongsagu K, Sihanonth P, Gadd GM. Fungal biotransformation of zinc silicate and sulfide mineral ores. Environ Microbiol. 15:2173–2186. 10.1111/1462-2920.12089
- Yadava H, Gothwala RK, Nigamb VK, Sinha-Roya S, Ghosha P. 2013. Optimization of culture conditions for phosphate solubilization by a thermo-tolerant phosphate-solubilizing bacterium Brevibacillus sp. BISR-HY65 isolated from phosphate mines. Biocat Agric Biotech. 2:217–225.
- Yu PH, Chen CC, Wu LC. 1970. The production of indoleacetic acid by Nectria pterospermi Saw. Bot Bulletin of Acad Sinica. 11:98–104.
- Zhao Z, Zhang Y, Liu X, Zhang X, Liu S, Yu X, Ren Y, Zheng X, Zhou K, Jiang L, et al. 2013. A role for a dioxygenase in auxin metabolism and reproductive development in rice. Dev Cell. 27:113–122. 10.1016/j.devcel.2013.09.005