Abstract
The ability to analyze the gene activity occurring within a single cell has ushered in a new understanding of complex biological processes. Furthermore, this capability has established the prerequisite technologies for the analysis of cells involved in complex pathogenic and/or symbiotic interactions. Collectively, the identification of biological models permitting the analysis of individual cells and improvements in histological technology are allowing for analyses of cells positioned within tissues and involved in complex cellular interactions at unprecedented resolution. Here, a plastic embedding procedure is used for laser microdissection of plant tissues infected with a pathogen. This technique enabled the acquisition of nucleic acids from semi-thin sections that can be used for downstream biological studies of host–pathogen interaction at the single-cell resolution.
Introduction
The identity of a cell is defined by its function. In this regard, determining a cell's activity at the genomics level would aid in understanding cell identity and function. It is well known from histological studies that many cell types are involved vastly in different metabolic processes even from their direct neighbors (). Due to the nature of cell integration within tissues, isolating cells from close neighbors is difficult and tends to complicate downstream analyses. The ability to isolate individual cells from their neighboring cells would improve the ability to identify individual cell chemistries and biological processes. When aided by high-throughput reverse genetic capabilities, functional studies of the genes identified from those genomics analyses can be performed without the need for traditional time-consuming genetic screens (Gandotra et al. Citation2013). This is particularly important when the system under study is not genetically tractable.
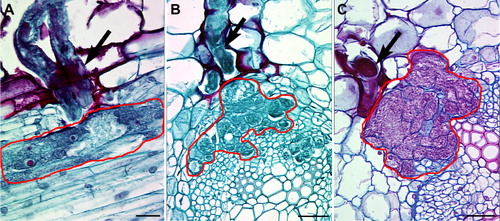
The isolation of cells for biological analyses
A number of cell isolation procedures have been developed over the years in order to determine the gene activity of specific cell types. The procedures fall into two main categories: (1) cell culturing and processing procedures that lack the requirement of embedding or infiltration in a support medium to facilitate cell isolation and collection, and (2) processing procedures that require embedding or infiltration in a support medium which facilitates cell isolation and collection. Each type is discussed briefly.
Cell culture and cell sorting processes are strategies that do not require a support medium and allow for a variety of physical separation techniques to be performed. Cell culturing methods permit cells to proliferate by mitosis under defined culture conditions and then can be collected in greater numbers. Cell culturing processes can, but do not always, include tissue maceration to separate cells. The cells then are allowed to grow in culture for defined periods of time. Physical characteristics of the cells and/or marker-assisted tools are then used to separate the cells by fluorescence-activated cell sorting (FACS). Details of FACs and related methods can be obtained from a series of reviews (Fraker et al. Citation1995; Galbraith et al. Citation1999; Herzenberg & De Rosa Citation2000; Miura et al. Citation2000; Valitutti & Dessing Citation2000; Maric & Barker Citation2004; Tung et al. Citation2007; Zhu & Murthy Citation2013). An underlying commonality of cells used in these isolation procedures is that they are relatively easy to separate based on their physical or chemical characteristics.
Some cell types, particularly those in plants, are difficult to separate because of structural barriers like a cell wall and/or their position within the tissue or organ. However, FACS has been used successfully in plants (Birnbaum et al. Citation2003; Bargmann & Birnbaum Citation2009; Bargmann et al. Citation2013). In cases where the cells are difficult to purify, cell separation can be accomplished through histology-associated physical procedures such as laser-assisted microdissection (LM; Isenberg et al. Citation1976; Emmert-Buck et al. Citation1996, Asano et al. Citation2002). Unlike the FACS technique, which is limited by the need for the presence of a specific cell marker, LM has the advantage of not requiring a specific cell marker. The LM procedure entails the embedding of tissue in one of several types of tissue support media, followed by microdissection under a microscope (Isenberg et al. Citation1976; Emmert-Buck et al. Citation1996). Some of the common support media in which the tissue is embedded or infiltrated are paraffin (Goldsworthy et al. Citation1999; Tam et al. Citation1999) or those that allow better preservation of the biological molecules (i.e. RNA) through cryopreservation (Tissue-Tek® optimal cutting temperature [OCT®] Compound, Sakura Finetek, INC., Torrance, CA, USA; Bhattacharya et al. Citation2003; Tadros et al. Citation2003; Dalmas et al. Citation2008). There are advantages to each embedding/infiltration type. For example, paraffin is easy to maintain in the lab, requires little technical experience to use, and allows tissue to be stored and used at ambient temperature. Tissue embedded in paraffin also can potentially be stored for years at ambient temperature or temperatures as low as 4°C at which point the paraffin becomes brittle and cracks. Another major advantage of paraffin is that it preserves anatomical detail well which aids in the ability to identify the cells during LM. However, for studies focused on gene expression, the paraffin-embedding procedure has drawbacks. The major drawback of paraffin is contamination with RNAses, resulting in RNA degradation (Jonsson & Lagerstedt Citation1957; Nair Citation1958; Groelz et al. Citation2013; Staff et al. Citation2013). Furthermore, some of the early steps in the paraffin embedding procedure, prior to the casting of tissue into molds, involve temperatures >50°C which has negative impacts on the tissue. Another drawback of the paraffin-embedding strategy is that the cells must be sectioned at thicknesses of 4 µm or greater (typically 10 µm) because of the physical limitations of the paraffin support medium with regard to sectioning. In contrast, OCT-based methods rely on cold-stored tissue. The OCT-infiltrated tissue must be cryosectioned, requiring relatively more technical capability which could be a problem since more expensive sectioning devices requiring more expertise are required. Notably, OCT which is composed of water-soluble glycols and resins can compromise the tissue whereby membranes are disrupted and cellular contents are released. The formation of ice crystals is also a major problem, because of how they can puncture membranes and disrupt cellular structure. Furthermore, the fine structure of plants is not preserved well when tissue is infiltrated with OCT (Emrich et al. Citation2007). This feature of OCT makes the identification of specific cell types in cryopreserved tissues virtually impossible. Therefore, these characteristics of cryopreservation may allow cells to gain false or misleading structural information in a field of sectioned tissue. Thus, the desired cells cannot be collected without further staining or use of molecular markers. This problem presented by OCT is exacerbated if there are no available stains or molecular probes to detect the cells. In many cases, the identification of uniquely expressed genes is the reason why the LM technique is used in the first place. Furthermore, like paraffin embedding, cells in OCT must also be thick-sectioned, increasing the probability that undesired cells are collected.
The collection of semi-thin sections
The LM technique has been a very powerful tool in a variety of biological fields including plant and animal research where cell collection can be problematic (reviewed in Simone et al. Citation1998, Citation2000; Emmert-Buck et al. Citation2000; Gillespie et al. Citation2001; Espina et al. Citation2004, Citation2005, Citation2009; Klink et al. Citation2005; Balestrini et al. Citation2009; Vicidomini et al. Citation2010; Gandotra et al. Citation2013). In addition, the LM technology has allowed for the study of DNA mutations, transcriptome, proteome, metabolome, and miRNAs (Meier-Ruge et al. Citation1976; Isenberg et al. Citation1976; Hedrum et al. Citation1994; Emmert-Buck et al. Citation1996; Bernsen et al. Citation1998; Schütze & Lahr Citation1998; Sgroi et al. Citation1999; Ornstein et al. Citation2000; Jessani et al. Citation2002, Citation2004; Schad et al. Citation2005; Nonn et al. Citation2010; Cancer Genome Atlas Research Network Citation2011; Nishimoto et al. Citation2012). Unfortunately in some areas of study like plant–organism interactions, isolating cells directly involved in the process is often difficult because relatively few cells are actually involved in the interaction while the remainder of the plant body actually masks the molecular events occurring locally within the infected cells.
The number of localized responses that would be aided by the increased specificity afforded by obtaining quality RNA from tissue sections is vast (Santos et al. Citation2012; Ghodke et al. Citation2013; Gupta et al. Citation2013; Ozawa et al. Citation2013). For example, plant parasitic nematodes cause as much as 100–150 billion dollars in damage annually, worldwide (Sasser & Freckman Citation1987; Chitwood Citation2003). Therefore, with this plant–pathogen interaction alone there exists an urgency to identify the precise spatial gene expression patterns that underlie susceptibility. Since many plant pathogens infect systemically or are interwoven with the plant cells, it is difficult to differentiate between cells that are directly infected versus the cells that are passively damaged. However, LM of tissue supported in paraffin has allowed for understanding the localized gene activity in the cells undergoing infection and has led directly to the identification of resistance genes (Jammes et al. Citation2005; Klink et al. Citation2005, Citation2007, Citation2009, Citation2010, Citation2011; Matsye et al. Citation2011; Citation2012; Matthews et al. Citation2013; Pant et al. Citationin press). Notably, the paraffin-embedding method, followed by LM and gene expression analyses allowed for the identification of the vesicular transport machinery being involved in the defense response of Glycine max (soybean) to Heterodera glycines (soybean cyst nematode) (Matsye et al. Citation2012). The identified α-SNAP resistance gene which is located at the rhg1 resistance locus is not a classical resistance (R) gene (Matsye et al. Citation2012). However, it could be that α-SNAP regulates other genes involved in resistance. Important contributions have also been made in plant–fungal studies (Chandran et al. Citation2009; Hacquard et al. Citation2010). Even with the ability to perform genomics analyses of collected cell populations, recent advances in single-cell genomics are showing that even cell types that appear similar or identical are undergoing vastly different genetic programs (Guo et al. Citation2010). Thus, to gain a better understanding of these cells, it is necessary to perform single-cell gene expression analyses to further increase the resolution of the analysis.
Plastic embedding and single-cell gene study
The application of single-cell genomics has permitted the study of various developmental processes (Schütze & Lahr Citation1998; Chiang & Melton Citation2003; Guo et al. Citation2010). Such a goal is complicated in plants due to the problems presented by the cell wall and the limits it places with regard to the isolation of cells. However, cell populations can be collected from complex tissues by FACs methods or LM (Asano et al. Citation2002; Birnbaum et al. Citation2003). In these analyses, hundreds if not thousands of cells are required to perform the molecular studies. As can be imagined, it is possible that each cell in those populations may actually have unique gene expression profiles based on their specific function and absolute position in the tissue or organ (Guo et al. Citation2010). This problem would represent a major challenge for genomics studies involving plant–organism interactions because of the varied position of the pathogen, the limited amount of sample, and technical problems involved in isolating individual cells. However, the number of localized responses that would be aided by the increased specificity afforded by obtaining quality RNA from semi-thin sections is extensive (Santos et al. Citation2012; Ghodke et al. Citation2013; Gupta et al. Citation2013; Ozawa et al. Citation2013).
One particular type of pathogen, plant parasitic nematodes present a unique opportunity whereby the plant cells that are intimately associated with the pathogen are well defined. This feature of their biology permits the collection and downstream analysis of individual cells at the genomic and molecular levels. For example, some plant parasitic nematodes create a nurse cell called a syncytium that is actually a conglomeration of many cells that fuse together due to cell wall degradation (). As already noted, the combination of LM and Illumina® deep sequencing of nurse cells undergoing defense has led to the identification of resistance genes (Matsye et al. Citation2011, Citation2012). In contrast, a different type of nurse cell known as a giant cell that develops from a single cell can be formed in plants (e.g. cotton) from nuclear division followed by cell expansion during infection by root knot nematodes (e.g. Meloidogyne incognita), Giant cells are well suited for single-cell genomics analyses. However, giant cells form in many different plants that interact with root knot-forming plant parasitic nematodes.
Materials and methods
The methods used in this analysis follow our previously published procedures (Klink et al. Citation2005, Citation2007, Citation2009, Citation2013). All glassware and metal utensils were made RNAse-free by baking at 180°C for 8 hr. Solutions were made RNAse-free with Nano-pure® (Barnstead, Thermo Fischer Scientific Inc., Waltham, MA) water with 0.1% diethyl pyrocarbonate (DEPC; Sigma, St. Louis, MO). The DEPC was dissolved in the aqueous solutions and stirred overnight for 12 hr before autoclaving to remove the DEPC. Tissue was fixed in 75% ethanol:25% glacial acetic acid (Klink et al. Citation2005). The specimens were dehydrated with a graded ethanol series (75%, 90%, and 3× 100%). Tissues were infiltrated and embedded with Technovit (Electron Microscopy Sciences, Hatfield, PA) with xylene as a transitional fluid according to the manufacturer's instructions (http://www.ebsciences.com/histology/methacrylate.htm) following Klink et al. (Citation2013). Tissue pieces were placed into Beem® capsules and polymerized at –8°C for 24 hr (Klink et al. Citation2013). Semi-thin sections were cut with a diamond knife at 0.8 µm with a Reichert-Jung Ultracut-E ultramicrotome (Leica Microsystems®, Germany). Several drops of DEPC-treated water were placed on PEN MembraneSlides® (Leica Microsystems®) that are set on a slide warmer set at 40°C, allowing for the plastic sections to flatten out and adhere to the slide once the DEPC-treated water evaporates. The slides were used immediately for LM once the water was completely evaporated. LM was performed on an Arcturus® Veritas® microscope (Molecular Devices, Sunnyvale, CA) at the College of Veterinary Medicine, Mississippi State University. No special treatment of the slides was required. Cells were collected on CapSure HS LCM Caps (Applied Biosystems, Foster City, CA). Cells from the LM were washed-off of the HS cap by micropipetting 20 µl of XB buffer (Applied Biosystems) onto the HS cap, and moving the solution to a microfuge tube. RNA samples were isolated with the PicoPure RNA Isolation Kit (Applied Biosystems) according to the manufacturer's instructions (Klink et al. Citation2005, Citation2007, Citation2009) with the addition of a DNAse treatment using DNA-free (Ambion), just before the second column wash (Klink et al. Citation2005, Citation2007). RNA quality and yield were tested with the Nanodrop® (Biorad, Hercules, CA) according to the manufacturer's instructions (Klink et al. Citation2013). In comparative studies, RNA was isolated from G. max roots using the UltraClean® Plant RNA Isolation Kit (Mo Bio Laboratories®, Inc.; Carlsbad, CA) and treated with DNase I to remove genomic DNA.
Results and discussion
The plastic embedding LM (pe-LM) approach, used in concert with LM and RNA isolation methodologies, has recently been demonstrated showing that high-quality RNA can be obtained from cells long understood to be difficult for their isolation (Thibaudeau & Altig Citation1988; Klink et al. Citation2013). The protocol focused on using the Technovit 9100 polymer. Technovit 9100 has been applied to the study of tadpole tooth and soft tissues and has proven to be superior in retaining antigenicity as compared to paraffin sections and other plastics (Arnold et al. Citation1998, Citation2003; Yang et al. Citation2003; Brorson & Reinholt Citation2008; Vertenten et al. Citation2008; Singhrao et al. Citation2009; Wittenburg et al. Citation2009; Steiniger et al. Citationin press). Because all tissue processing steps could be done at low temperatures (−20°C or lower), RNA quality would be expected to maximize preservation (Klink et al. Citation2013). The developed procedure has allowed for the sectioning of semi-thin sections at a thickness of 0.8 µm (Klink et al. Citation2013). This thickness is far thinner than the 10 µm typically used for paraffin sectioning and LM (Klink et al. Citation2005). Certainly obtaining ultrathin sections, while not a focus here, is possible with Technovit 9100. A major advantage of the pe-LM procedure is that the tissue can be sectioned and collected onto slides for LM without having to etch away the plastic (). This property is in contrast to tissues that are embedded in paraffin where the paraffin must be dissolved away prior to the LM procedure. In LM procedures using paraffin as a tissue support, the crystalline nature of the paraffin interferes with the visualization of the cells due to the refraction of the light as it passes through the paraffin. Furthermore, loss of biological molecules in the form of RNA happens as the paraffin is dissolved away (Jonsson & Lagerstedt Citation1958; Nair Citation1958; Groelz et al. Citation2013; Staff et al. Citation2013). As can be imagined, this situation may be particularly problematic for small RNA species like microRNAs (miRNAs) that would be easily liberated from the section. In contrast, the collection of serial, semi-thin sections from plastic-embedded tissues allows for the reconstruction of those section files onto LM slides. Furthermore, light passing through the plastic section does not experience the same interference/diffraction, allowing the cells to be easily visualized, even when the plastic is not removed from the section (Klink et al. Citation2013). Thus, the desired cells are easily observed, allowing for their collection by LM and all materials in the plastic-embedded cell are captured since no etching is done (). With the reduction of these technological limitations presented by small sample volume in transcriptional analyses, it is possible to study the interaction of plants in relation to model plant pathogens at high resolution without the loss of significant cellular information (), making analyses of single cells possible (Abad et al. Citation2008; Guo et al. Citation2010; Citri et al. Citation2011; Jang et al. Citation2011; Byers et al. Citation2012; Klink et al. Citation2013; Livak et al. Citation2013).
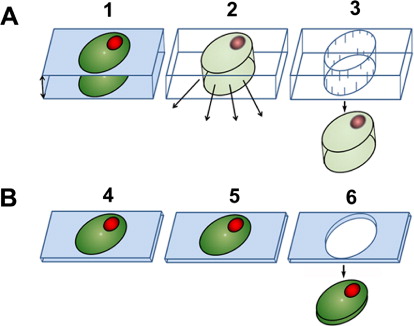
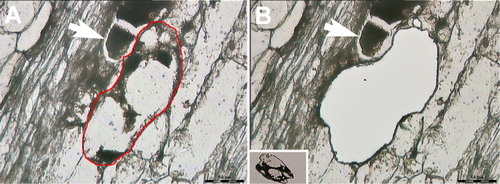
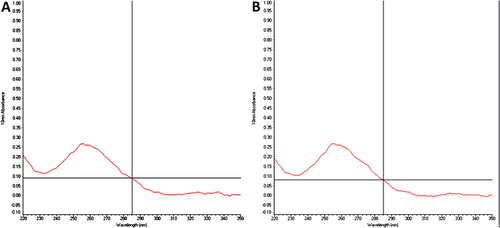
Conclusion
Genomics-level analyses of complex cellular processes at cellular resolution are difficult to perform, particularly when the cells are buried deeply in tissues. This problem is true in plants for the study of complex interactions of pathogenic or symbiotic organisms. Recent advances in tissue processing permit the collection of semi- and ultrathin sections while minimizing the loss of biologically important molecules (Klink et al. Citation2013). The combination of this strategy with recently developed genomics tools is revolutionizing the study of single cells undergoing important biological interactions, making the understanding of the intricacies of complex biological processes possible in systems once thought to be non-model and genetically intractable.
Acknowledgments
This publication was made possible by the start-up support of Mississippi State University and the Department of Biological Sciences (DBS). The work is supported jointly between the College of Arts and Sciences (DBS) and the Mississippi Agricultural and Forestry Experimental Station (MAFES). The authors are thankful to Dr Robert Nichols, Cotton Inc., and Dr Larry Heatherly, Mississippi Soybean Promotion Board, for their continued support. The authors thank George Hopper, Reuben Moore, and Wes Burger (MAFES) whose support has made the work possible. Support has also been provided by Amanda Lawrence of the Institute for Imaging and Analytical Technologies, Mississippi State University.
References
- Abad P, Gouzy J, Aury JM, Castagnone-Sereno P, Danchin EG, Deleury E, Perfus-Barbeoch L, Anthouard V, Artiguenave F, Blok VC, et al. 2008. Genome sequence of the metazoan plant-parasitic nematode Meloidogyne incognita. Nat Biotechnol. 26:909–915. 10.1038/nbt.1482
- Arnold WH, Gaengler P, Kalkutschke L. 1998. Three-dimensional reconstruction of approximal subsurface caries lesions in deciduous molars. Clin Oral Investig. 2:174–179. 10.1007/s007840050066
- Arnold WH, Konopka S, Kriwalsky MS, Gaengler P. 2003. Morphological analysis and chemical content of natural dentin carious lesion zones. Ann Anat. 185:419–424. 10.1016/S0940-9602(03)80099-7
- Asano T, Masumura T, Kusano H, Kikuchi S, Kurita A, Shimada H, Kadowaki K. 2002. Construction of a specialized cDNA library from plant cells isolated by laser capture microdissection: toward comprehensive analysis of the genes expressed in the rice phloem. Plant J. 32:401–408. 10.1046/j.1365-313X.2002.01423.x
- Balestrini R, Gómez-Ariza J, Klink VP, Bonfante P. 2009. Application of laser microdissection (LM) in plant pathogenic and symbiotic interactions. J Plant Interact. 4:81–92. 10.1080/17429140902770396
- Bargmann BOR, Birnbaum KD. 2009. Positive fluorescent selection permits precise, rapid, and indepth overexpression analysis in plant protoplasts. Plant Physiol. 149:1231–1239. 10.1104/pp.108.133975
- Bargmann BO, Vanneste S, Krouk G, Nawy T, Efroni I, Shani E, Choe G, Friml J, Bergmann DC, Estelle M, Birnbaum KD. 2013. A map of cell type-specific auxin responses. Mol Syst Biol. 9:688. 10.1038/msb.2013.40
- Bernsen MR, Dijkman HB, de Vries E, Figdor CG, Ruiter DJ, Adema GJ, van Muijen GN. 1998. Identification of multiple mRNA and DNA sequences from small tissue samples isolated by laser-assisted microdissection. Lab Invest. 78:1267–1273.
- Bhattacharya SH, Gal AA, Murray KK. 2003. Laser capture microdissection MALDI for direct analysis of archival tissue. J Proteome Res. 2:95–98. 10.1021/pr025547m
- Birnbaum K, Shasha DE, Wang JY, Jung JW, Lambert GM, Galbraith DW, Benfey PN. 2003. A gene expression map of the Arabidopsis root. Science. 302:1956–1960. 10.1126/science.1090022
- Brorson SH, Reinholt FP. 2008. The intensity of immunogold labeling of deplasticized acrylic sections compared to deplasticized epoxy sections – theoretical deductions and experimental data. Micron. 39:144–150. 10.1016/j.micron.2006.11.006
- Byers RL, Harker DB, Yourstone SM, Maughan PJ, Udall JA. 2012. Development and mapping of SNP assays in allotetraploid cotton. Theor Appl Genet. 124:1201–1214. 10.1007/s00122-011-1780-8
- Cancer Genome Atlas Research Network. 2011. Integrated genomic analyses of ovarian carcinoma. Nature. 474:609–615. 10.1038/nature10166
- Chandran D, Inada N, Hather G, Kleindt CK, Wildermuth MC. 2009. Laser microdissection of Arabidopsis cells at the powdery mildew infection site reveals site-specific processes and regulators. Proc Natl Acad Sci USA. 107:460–465. 10.1073/pnas.0912492107
- Chiang MK, Melton DA. 2003. Single-cell transcript analysis of pancreas development. Dev Cell. 4:383–393. 10.1016/S1534-5807(03)00035-2
- Chitwood DJ. 2003. Nematicides. In: Plimmer JR, editor. Encyclopedia of agrochemicals, Vol. 3. New York (NY): Wiley; p. 1104–1115.
- Citri A, Pang ZP, Südhof TC, Wernig M, Malenka RC. 2011. Comprehensive qPCR profiling of gene expression in single neuronal cells. Nat Protoc. 7:118–127. 10.1038/nprot.2011.430
- Dalmas DA, Scicchitano MS, Chen Y, Kane J, Mirabile R, Schwartz LW, Thomas HC, Boyce RW. 2008. Transcriptional profiling of laser capture microdissected rat arterial elements: fenoldopam-induced vascular toxicity as a model system. Toxicol Pathol. 36:496–519. 10.1177/0192623307311400
- Emmert-Buck MR, Bonner RF, Smith PD, Chuaqui RF, Zhuang Z, Goldstein SR, Weiss RA, Liotta LA. 1996. Laser capture microdissection. Science. 274:998–1001. 10.1126/science.274.5289.998
- Emmert-Buck MR, Gillespie JW, Paweletz CP, Ornstein DK, Basrur V, Appella E, Wang QH, Huang J, Hu N, Taylor P, Petricoin EF 3rd. 2000. An approach to proteomic analysis of human tumors. Mol Carcinog. 27:158–165. 10.1002/(SICI)1098-2744(200003)27:3%3C158::AID-MC2%3E3.0.CO;2-2
- Emrich SJ, Barbazuk WB, Li L, Schnable PS. 2007. Gene discovery and annotation using LCM-454 transcriptome sequencing. Genome Res. 17:69–73. 10.1101/gr.5145806
- Espina V, Dettloff KA, Cowherd S, Petricoin EF 3rd, Liotta LA. 2004. Use of proteomic analysis to monitor responses to biological therapies. Expert Opin Biol Ther. 4:83–93. 10.1517/14712598.4.1.83
- Espina V, Geho D, Mehta AI, Petricoin EF 3rd, Liotta LA, Rosenblatt KP. 2005. Pathology of the future: molecular profiling for targeted therapy. Cancer Invest. 23:36–46. 10.1081/CNV-46434
- Espina V, Wulfkuhle J, Liotta LA. 2009. Application of laser microdissection and reverse-phase protein microarrays to the molecular profiling of cancer signal pathway networks in the tissue microenvironment. Clin Lab Med. 29:1–13. 10.1016/j.cll.2009.03.001
- Fraker PJ, King LE, Lill-Elghanian D, Telford WG. 1995. Quantification of apoptotic events in pure and heterogeneous populations of cells using the flow cytometer. Methods Cell Biol. 46:57–76.
- Galbraith DW, Anderson MT, Herzenberg LA. 1999. Flow cytometric analysis and FACS sorting of cells based on GFP accumulation. Methods Cell Biol. 58:315–341.
- Gandotra N, Coughlan SJ, Nelson T. 2013. The Arabidopsis leaf provascular cell transcriptome is enriched in genes with roles in vein patterning. Plant J. 74:48–58. 10.1111/tpj.12100
- Ghodke AB, Chavan SG, Sonawane BV, Bharose AA. 2013. Isolation and in vitro identification of proteinase inhibitors from soybean seeds inhibiting Helicoverpa gut proteases. J Plant Interact. 8:170–178. 10.1080/17429145.2012.668952
- Gillespie JW, Ahram M, Best CJ, Swalwell JI, Krizman DB, Petricoin EF, Liotta LA, Emmert-Buck MR. 2001. The role of tissue microdissection in cancer research. Cancer J. 7:32–39.
- Goldsworthy SM, Stockton PS, Trempus CS, Foley JF, Maronpot RR. 1999. Effects of fixation on RNA extraction and amplification from laser capture microdissected tissue. Mol Carcinog. 25:86–91. 10.1002/(SICI)1098-2744(199906)25:2%3C86::AID-MC2%3E3.0.CO;2-4
- Groelz D, Sobin L, Branton P, Compton C, Wyrich R, Rainen L. 2013. Non-formalin fixative versus formalin-fixed tissue: a comparison of histology and RNA quality. Exp Mol Pathol. 94:188–119. 10.1016/j.yexmp.2012.07.002
- Gupta P, Ravi I, Sharma V. 2013. Induction of β-1,3-glucanase and chitinase activity in the defense response of Eruca sativa plants against the fungal pathogen Alternaria brassicicola. J Plant Interact. 8:155–161. 10.1080/17429145.2012.679705
- Guo G, Huss M, Tong GQ, Wang C, Sun LL, Clarke ND, Paul Robson P. 2010. Resolution of cell fate decisions revealed by single-cell gene expression analysis from zygote to blastocyst. Dev Cell. 18:675–685. 10.1016/j.devcel.2010.02.012
- Hacquard S, Delaruelle C, Legué V, Tisserant E, Kohler A, Frey P, Martin F, Duplessis S. 2010. Laser capture microdissection of uredinia formed by Melampsora larici-populina revealed a transcriptional switch between biotrophy and sporulation. Mol Plant Microbe Interact. 23:1275–1286. 10.1094/MPMI-05-10-0111
- Hedrum A, Pontén F, Ren Z, Lundeberg J, Pontén J, Uhlén M. 1994. Sequence-based analysis of the human p53 gene based on microdissection of tumor biopsy samples. Biotechniques. 17:118–119, 122–124, 126–129.
- Herzenberg LA, De Rosa SC. 2000. Monoclonal antibodies and the FACS: complementary tools for immunobiology and medicine. Immunol Today. 21:383–390. 10.1016/S0167-5699(00)01678-9
- Isenberg G, Bielser W, Meier-Ruge W, Remy E. 1976. Cell surgery by laser micro-dissection: a preparative method. J Microsc. 107:19–24. 10.1111/j.1365-2818.1976.tb02419.x
- Jammes F, Lecomte P, de Almeida-Engler J, Bitton F, Martin-Magniette ML, Renou JP, Abad P, Favery B. 2005. Genomewide expression profiling of the host response to root-knot nematode infection in Arabidopsis. Plant J. 44:447–458. 10.1111/j.1365-313X.2005.02532.x
- Jang JS, Simon VA, Feddersen RM, Rakhshan F, Schultz DA, Zschunke MA, Lingle WL, Kolbert CP, Jen J. 2011. Quantitative miRNA expression analysis using fluidigm microfluidics dynamic arrays. BMC Genomics. 12:144. 10.1186/1471-2164-12-144
- Jessani N, Humphrey M, McDonald WH, Niessen S, Masuda K, Gangadharan B, Yates JR 3rd, Mueller BM, Cravatt BF. 2004. Carcinoma and stromal enzyme activity profiles associated with breast tumor growth in vivo. Proc Natl Acad Sci USA. 101:13756–13761. 10.1073/pnas.0404727101
- Jessani N, Liu Y, Humphrey M, Cravatt BF. 2002. Enyzme activity profiles of the secreted and membrane proteome that depict cancer cell invasiveness. Proc Natl Acad Sci USA. 99:10335–10340. 10.1073/pnas.162187599
- Jonsson N, Lagerstedt S. 1957. Demonstration of ribonuclease activity in sections from carnoy fixed rat pancreas. Experientia. 13:321–323. 10.1007/BF02296821
- Jonsson N, Lagerstedt S. 1958. Losses of nucleic acid derivatives from fixed tissue during flattening of paraffin sections on water. Experientia. 14:157–159. 10.1007/BF02157138
- Klink VP, Hosseini P, Matsye P, Alkharouf N, Matthews BF. 2009. A gene expression analysis of syncytia laser microdissected from the roots of the Glycine max (soybean) genotype PI 548402 (Peking) undergoing a resistant reaction after infection by Heterodera glycines (soybean cyst nematode). Plant Mol Biol. 71:525–567. 10.1007/s11103-009-9539-1
- Klink VP, Hosseini P, Matsye PD, Alkharouf NW, Matthews BF. 2011. Differences in gene expression amplitude overlie a conserved transcriptomic program occurring between the rapid and potent localized resistant reaction at the syncytium of the Glycine max genotype Peking (PI 548402) as compared to the prolonged and potent resistant reaction of PI 88788. Plant Mol Biol. 75:141–165. 10.1007/s11103-010-9715-3
- Klink VP, MacDonald M, Alkharouf N, Matthews BF. 2005. Laser capture microdissection (LCM) and expression analyses of Glycine max (soybean) syncytium containing root regions formed by the plant pathogen Heterodera glycines (soybean cyst nematode). Plant Mol Biol. 59:969–983. 10.1007/s11103-005-2416-7
- Klink VP, Overall CC, Alkharouf N, MacDonald MH, Matthews BF. 2007. Laser capture microdissection (LCM) and comparative microarray expression analysis of syncytial cells isolated from incompatible and compatible soybean roots infected by soybean cyst nematode (Heterodera glycines). Planta. 226:1389–1409. 10.1007/s00425-007-0578-z
- Klink VP, Overall CC, Alkharouf NW, MacDonald MH, Matthews BF. 2010. Microarray detection calls as a means to compare transcripts expressed within syncytial cells isolated from incompatible and compatible soybean (Glycine max) roots infected by the soybean cyst nematode (Heterodera glycines). J Biomed Biotech. 1–30. 10.1155/2010/491217
- Klink VP, Thibaudeau G, Altig RG. 2013. A novel sample preparation method that enables nucleic acid analysis from ultrathin sections. Microsc Microanal. 19:1–7. 10.1017/S1431927613000044
- Livak KJ, Wills QF, Tipping AJ, Datta K, Mittal R, Goldson AJ, Sexton DW, Holmes CC. 2013. Methods for qPCR gene expression profiling applied to 1440 lymphoblastoid single cells. Methods. 59:71–79. 10.1016/j.ymeth.2012.10.004
- Maric D, Barker JL. 2004. Neural stem cells redefined: a FACS perspective. Mol Neurobiol. 30:49–76. 10.1385/MN:30:1:049
- Matthews BF, Beard H, MacDonald MH, Kabir S, Youssef RM, Hosseini P, Brewer E. 2013. Engineered resistance and hypersusceptibility through functional metabolic studies of 100 genes in soybean to its major pathogen, the soybean cyst nematode. Planta. 237:1337–1357. 10.1007/s00425-013-1840-1
- Matsye PD, Kumar R, Hosseini P, Jones CM, Tremblay A, Alkharouf NW, Matthews BF, Klink VP. 2011. Mapping cell fate decisions that occur during soybean defense responses. Plant Mol Biol. 77:513–528. 10.1007/s11103-011-9828-3
- Matsye PD, Lawrence GW, Youssef RM, Kim K-H, Matthews BF, Lawrence KS, Klink VP. 2012. The expression of a naturally occurring, truncated allele of an α-SNAP gene suppresses plant parasitic nematode infection. Plant Mol Biol. 80:131–155. 10.1007/s11103-012-9932-z
- Meier-Ruge W, Bielser W, Remy E, Hillenkamp F, Nitsche R, Unsold R. 1976. The laser in the Lowry technique for microdissection of freeze-dried tissue slices. Histochem J. 8:387–401. 10.1007/BF01003828
- Miura I, Takahashi N, Kobayashi Y, Saito K, Miura AB. 2000. Molecular cytogenetics of stem cells: target cells of chromosome aberrations as revealed by the application of fluorescence in situ hybridization to fluorescence-activated cell sorting. Int J Hematol. 72:310–317.
- Nair KK. 1958. The effect of some common fixatives on the enzymatic activity of ribonuclease. Experientia. 14:172–173. 10.1007/BF02158645
- Nishimoto K, Rigsby CS, Wang T, Mukai K, Gomez-Sanchez CE, Rainey WE, Seki T. 2012. Transcriptome analysis reveals differentially expressed transcripts in rat adrenal zona glomerulosa and zona fasciculata. Endocrinology. 153:1755–1763. 10.1210/en.2011-1915
- Nonn L, Vaishnav A, Gallagher L, Gann PH. 2010. mRNA and micro-RNA expression analysis in laser-capture microdissected prostate biopsies: valuable tool for risk assessment and prevention trials. Exp Mol Pathol. 88:45–51. 10.1016/j.yexmp.2009.10.005
- Ornstein DK, Gillespie JW, Paweletz CP, Duray PH, Herring J, Vocke CD, Topalian SL, Bostwick DG, Linehan WM, Petricoin EF 3rd, Emmert-Buck MR. 2000. Proteomic analysis of laser capture microdissected human prostate cancer and in vitro prostate cell lines. Electrophoresis. 21:2235–2242. 10.1002/1522-2683(20000601)21:11%3C2235::AID-ELPS2235%3E3.0.CO;2-A
- Ozawa R, Shiojiri K, Kishimoto K, Matsui K, Arimura G, Urashimo S, Nishioka T, Takabayashi J. 2013. Cytosolic LOX overexpression in Arabidopsis enhances the attractiveness of parasitic wasps in response to herbivory and incidences of parasitism. J Plant Interact. 8:207–215. 10.1080/17429145.2013.772663
- Pant SR, Matsye PD, McNeece BT, Sharma K, Krishnavajhala A, Lawrence GW, Klink VP. in press. Syntaxin 31 functions in Glycine max resistance to the plant parasitic nematode Heterodera glycines. Plant Mol Biol.
- Santos JC, Santos CIR, Cares, JE, Almeida-Cortez JS. 2012. Impact of nematode-induced galls on Miconia prasina (Sw.) DC (Melastomataceae) traits in the Atlantic forest of northeastern Brazil. J Plant Interact. 7:197–203. 10.1080/17429145.2011.652678
- Sasser JN, Freckman DW. 1987. A world perspective on nematology: the role of the society. In: Veech JA, Dickerson DW, editors. Vistas on nematology. Hyattsville: Society of Nematologists; p. 7–14.
- Schad M, Mungur R, Fiehn O, Kehr J. 2005. Metabolic profiling of laser microdissected vascular bundles of Arabidopsis thaliana. Plant Methods. 1:2. 10.1186/1746-4811-1-2
- Schütze K, Lahr G. 1998. Identification of expressed genes by laser-mediated manipulation of single cells. Nat Biotechnol. 16:737–742.
- Sgroi DC, Teng S, Robinson G, LeVangie R, Hudson JR Jr, Elkahloun AG. 1999. In vivo gene expression profile analysis of human breast cancer progression. Cancer Res. 59:5656–5661.
- Simone NL, Bonner RF, Gillespie JW, Emmert-Buck MR, Liotta LA. 1998. Laser-capture microdissection: opening the microscopic frontier to molecular analysis. Trends Genet. 14:272–276. 10.1016/S0168-9525(98)01489-9
- Simone NL, Paweletz CP, Charboneau L, Petricoin EF 3rd, Liotta LA. 2000. Laser capture microdissection: beyond functional genomics to proteomics. Mol Diagn. 5:301–307.
- Singhrao SK, Müller CT, Gilbert SJ, Duance VC, Archer CW. 2009. An immunofluorescence method for postembedded tissue in the acrylic resin Technovit 9100 New using fluorescein isothiocyanate secondary detection. Microsc Res Tech. 72:501–506. 10.1002/jemt.20705
- Staff S, Kujala P, Karhu R, Rökman A, Ilvesaro J, Kares S, Isola J. 2013. Preservation of nucleic acids and tissue morphology in paraffin-embedded clinical samples: comparison of five molecular fixatives. J Clin Pathol. 66:807–810. 10.1136/jclinpath-2012-201283
- Steiniger BS, Bubel S, Böckler W, Lampp K, Seiler A, Jablonski B, Guthe M, Stachniss V. in press. Immunostaining of pulpal nerve fibre bundle/arteriole associations in ground serial sections of whole human teeth embedded in Technovit® 9100. Cells Tissues Organs.
- Tadros Y, Ruiz-Deya G, Crawford BE, Thomas R, Abdel-Mageed AB. 2003. In vivo proteomic analysis of cytokine expression in laser capture-microdissected urothelial cells of obstructed ureteropelvic junction procured by laparoscopic dismembered pyeloplasty. J Endourol. 17:333–336. 10.1089/089277903322145530
- Tam AS, Foley JF, Devereux TR, Maronpot RR, Massey TE. 1999. High frequency and heterogeneous distribution of p53 mutations in aflatoxin B1-induced mouse lung tumors. Cancer Res. 59:3634–3640.
- Thibaudeau G, Altig R. 1988. Sequence of ontogenetic development and atrophy of the oral apparatus of six anuran tadpoles. J Morphol. 197:63–69. 10.1002/jmor.1051970106
- Tung JW, Heydari K, Tirouvanziam R, Sahaf B, Parks DR, Herzenberg LA, Herzenberg LA. 2007. Modern flow cytometry: a practical approach. Clin Lab Med. 27:453–468. 10.1016/j.cll.2007.05.001
- Valitutti S, Dessing M. 2000. Measurement of calcium mobilization responses in killer cell/target conjugates by FACS analysis. Methods Mol Biol. 121:305–311.
- Vertenten G, Vlaminck L, Ducatelle R, Lippens E, Cornelissen M, Gasthuys F. 2008. Immunohistochemical analysis of low-temperature methyl methacrylate resin-embedded goat tissues. Anat Histol Embryol. 37:452–457. 10.1111/j.1439-0264.2008.00881.x
- Vicidomini R, Tortoriello G, Furia M, Polese G. 2010. Laser microdissection applied to gene expression profiling of subset of cells from the Drosophila wing disc. J Vis Exp. 38:1895.
- Wittenburg G, Volkel C, Mai R, Lauer G. 2009. Immunohistochemical comparison of differentiation markers on paraffin and plastic embedded human bone samples. J Physiol Pharmacol. 60:43–49.
- Yang R, Davies CM, Archer CW, Richards RG. 2003. Immunohistochemistry of matrix markers in Technovit 9100 New-embedded undecalcified bone sections. Eur Cell Mater. 6:57–71.
- Zhu B, Murthy SK. 2013. Stem cell separation technologies. Curr Opin Chem Eng. 2:3–7. 10.1016/j.coche.2012.11.002