Abstract
The aim of this study is to investigate the impacts of exogenous salicylic acid (SA) pretreatments on hydrogen peroxide (H2O2) accumulation, protein oxidation, and H2O2-scavenging enzymes in leaves of Cd-treated flax seedlings. Cd-enhanced H2O2 levels were related to increased activities of guaiacol peroxidase (POX, EC 1.11.1.7) and ascorbate peroxidase (APX, EC 1.11.1.11), and were independent of changes in catalase (CAT, EC 1.11.1.6) and superoxide dismutase (SOD, EC 1.15.1.1) activities. In control flax seedlings, exogenous SA pretreatments inhibited the activity of CAT, resulted in an enhanced production of H2O2 suggesting that SA requires H2O2 to initiate an oxidative stress. However, although leaves of Cd-free flax seedlings pretreated with SA accumulated in vivo H2O2 by 1.2-fold compared with leaves of Cd-only exposed ones; the damage to growth and proteins after the exposure to Cd was significantly less, indicating that SA can regulate the Cd-induced oxidative stress. Moreover, the Cd-treated seedlings primed with SA exhibited a higher level of total antioxidant capacities and increased activities of H2O2-detoxifying enzymes.
1. Introduction
Flax (Linum usitatissimum L.), one of the most important fiber crops in the world, is generally exposed to cadmium (Cd) pollution, notably affecting its growth performance. Cd is not a nutrient element for animals and plants with no biological function, but known to cause a range of negative effects on plants (Hayat et al. Citation2012; Xu et al. Citation2013; Zhang et al. Citation2013). At the leaf level, Cd stress causes the chlorosis, inhibits photosynthesis, and damages the structure and function of photosystem II (Zhang et al. Citation2013). Cd also changes hormonal status, disturbs mineral nutrition and water balance, and affects membrane structure and permeability (Xu et al. Citation2013). At the cellular level, Cd stress can cause the dislocation of nutrients resulting in deficiency effects in plant cell. Within the cell, Cd stress may generate oxidative stress (Belkadhi et al. Citation2013) and triggers indirectly the production of reactive oxygen species (ROS) including the hydrogen peroxide (H2O2) (Mittler et al. Citation2004). At the same time, plants develop many defensive mechanisms in response to Cd stress for their survival. A first barrier against Cd stress is retention of Cd in the cell wall because they can somewhat restrict the Cd into cell (Douchiche et al. Citation2010). Since ROS can damage nucleic acids, proteins, lipids and amino acids, they must be scavengered by ROS detoxification mechanisms (Mittler et al. Citation2004; Djebali et al. Citation2008). These include the antioxidants (e.g. glutathione and ascorbate) and enzymes, such as catalase (CAT, EC 1.11.1.6), guaiacol peroxidase (POX, EC 1.11.1.7), ascorbate peroxidase (APX, EC 1.11.1.11), and superoxide dismutase (SOD, EC 1.15.1.1). Thus, Cd tolerance is also associated with the activation of antioxidant defense system (Rodriguez-Serrano et al. Citation2009; Gallego et al. Citation2012).
To better characterize the enhanced response of plants to Cd-mediated oxidative stress, Gallego et al. (Citation2012) established that exogenous salicylic acid (SA) application notably reduces the oxidative damage of Cd in plant cells. Recent studies demonstrated that it also participates in the signaling of abiotic stresses such as Cd stress (Hayat et al. Citation2012; Noriega et al. Citation2012). Exogenous SA was also found to enhance the efficiency of antioxidant defense system in plants and their tolerance to oxidative stress (Shi et al. Citation2009). Under Cd stress, several studies showed that SA-induced priming enhanced numerous antioxidant enzyme activities, thereby lowering the amounts of ROS inside cells (Shi et al. Citation2009; Zhang & Chen Citation2011).
Among ROS species, H2O2 is also an important signaling molecule which is produced by the chloroplast and involved in the response of the plant to different types of environmental stressors (Pérez-Ruiz et al. Citation2006). But when it accumulates at a high level, it becomes toxic because it can be converted to highly reactive hydroxyl radicals. Although increasing evidence indicates that SA may cause oxidative stress to plants through the accumulation of H2O2 (Chao et al. Citation2010), the preliminary treatment of plants with low concentrations of SA might have stress-induced adaptation effect, causing enhanced tolerance toward most kinds of abiotic stresses due primarily to enhanced antioxidative capacity (Janda et al. Citation2003).
In the present study, the relative relationships between SA pretreatments, H2O2, and H2O2-metabolizing enzymes have been studied in leaves of Cd-treated flax plantlets.
2. Materials and methods
2.1. Plant material and growth conditions
Flax seeds (cv. Viking), were soaked for 8 h in SA solutions (250 and 1000 µM) or water (0 µM SA) as previously shown in Belkhadi et al. (Citation2010). After that they were germinated for four days at 25°C in the dark, uniform plantlets were transferred to a continuously aerated nutrient solution (pH 5.5) containing 1 mM MgSO4, 2.5 mM Ca (NO3)2, 1 mM KH2PO4, 2 mM KNO3, 2 mM NH4Cl, 50 mM EDTA–Fe–K, 30 mM H3BO3, 10 mM MnSO4, 1 mM ZnSO4, 1 mM CuSO4, and 30 mM (NH4)6Mo7O24. The nutrient solution was buffered with HCl/KOH and changed twice per week. After growing for 2 days, plantlets were subjected during 10 days to 50 or 100 µM CdCl2. Plantlets were grown in a growth chamber at a day/night cycle of 16 h/8 h, at 23°C/18°C, respectively, a relative humidity close to 75% and a light intensity of 200 µmol photons m−2 s−1.
2.2. Plant growth parameters
The shoot-to-root ratio is one measure to assess the overall growth of control and Cd-treated plants. Healthy shoot system is a key to healthy plant. Any changes from control shoot to root ratio would be an indication of a change in the overall health of plants. The shoot-to-root ratio can be calculated for each treatment as follows: Fresh weight for shoots/Fresh weight for roots = shoot/root ratio.
2.3. Chlorophyll and shoot water contents
The soil plant analysis development (SPAD) chlorophyll content in the leaf attached to their parent plant was measured by using Minolta chlorophyll meter (SPAD-502; Konica Minolta Sensing Inc, Japan) whereas the water content (WC) of stems and leaves was estimated accordingly: Fresh weight (FW) was recorded using an analytical balance, after which optical properties were measured and samples were dried at 60°C in an oven, until constant weight (dry weight, DW) was reached. WC was calculated as follows: FW – DW/FW (g g−1 FW).
2.4. H2O2 and carbonyl group contents
Root samples were homogenized in 0.2 N perchloric acid (pH 7.5). The flax H2O2 content in roots was determined as described by O'Kane et al. (Citation1996). Protein-bound carbonyl content was determined by using the dinitrophenylhydrazine (DNPH) method, according to Reznick and Packer (Citation1994).
2.5. Antioxidant enzyme assays
For enzyme assays, frozen leaf samples were ground to a fine powder with liquid nitrogen and extracted with ice-cold 50 mM phosphate buffer (pH 7.0). The extracts were centrifuged at 4°C for 30 min at 20,000 × g and the resulting supernatants; henceforth referred to as crude extracts, was collected and used for protein content assay and enzyme activities. Protein content was determined according to Bradford (Citation1976) with bovine serum albumin as standard.
CAT activity was determined by following the consumption of H2O2 (extinction coefficient 39.4 mM−1 cm−1) at 240 nm for 30 s (Aebi Citation1984). POX activity was measured according to (Fielding & Hall Citation1978). APX activity was determined following the procedure described by (Nakano & Asada Citation1981). Total SOD activity was assayed by monitoring the inhibition of the photochemical reduction of nitroblue tetrazolium (NBT) according to the method of Beauchamp and Fridovich (Citation1971).
2.6. 2,2′-diphenyl-1-picrylhydrazyl (DPPH) and ferric-reducing antioxidant power (FRAP) assays
For antioxidant potential analysis, extracts were prepared following Ferreres et al. (Citation2007). Antioxidant potential of the samples was determined spectrophotometrically by the FRAP assay developed by Benzie and Strain (Citation1996) and by monitoring the disappearance of radical DPPH according to the method of Brand-Williams et al. (Citation1995).
2.7. Statistical analysis
All presented data are the mean values of three independent experiments with five replicates. Statistical calculations were performed with SPSS-17 statistical software. Mean difference comparison among different treatments was done by analysis of variance and Tukey's (HSD) test at a 0.05 probability level.
3. Results
3.1. Growth, hydration, and SPAD values
Ten days after the addition of Cd to the nutrient medium, flax plantlets exhibited reduced shoot growth as indicated by decrease in shoot/root ratio (). Moreover, in comparison with the control, the SPAD chlorophyll values of the leaves showed approximately 24% drop in 100 µM Cd- treated plants (). However, no alteration of stem and leaf water contents was detected ().
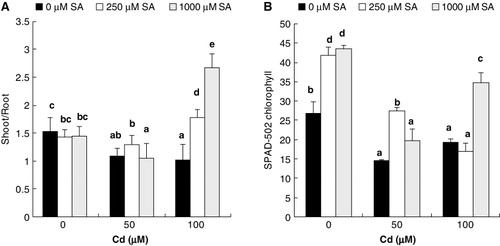
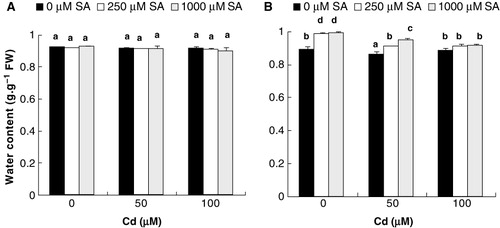
In contrast, presoaking with SA for 8 h led to an increase in the shoot/root ratio in dose-dependent manner (). In the presence of Cd, 8-h SA pretreatment (250 or 1000 µM) significantly increased the chlorophyll contents and also neutralized the harmful effect of the metal, completely at its lower and higher levels (). As a result, already after treatments with Cd, the whole shoot system showed healthy appearance and was taller and more voluminous (result not shown). However, no further amelioration in shoot hydration has been noticed in 8-h SA pretreated plantlets ().
3.2. Hydrogen peroxide, total soluble protein contents, and protein carbonylation
In leaves, Cd treatments showed a significant increase of H2O2 content compared with control (). It increased by about 100% over control at 100 µM Cd. However, upon the 8-h SA pretreatment (250 µM), there was a slight significant decrease in the H2O2 content in flax leaves compared to those with Cd only ().
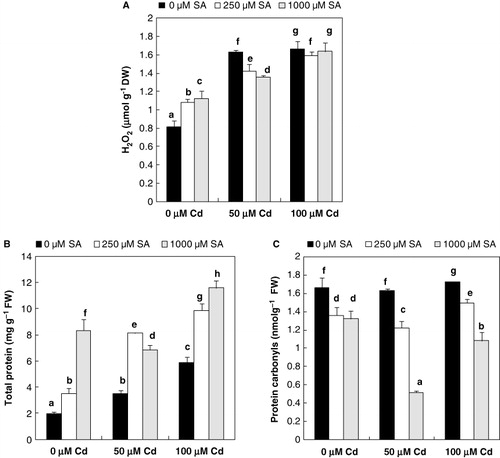
The Cd-associated damages to proteins were investigated by comparing total protein and carbonyl groups contents (). The accumulation of total soluble protein content was increased in comparison with control plantlets. In addition, 8-h SA pretreated flax plantlets exhibited a significant induction of leaf total protein content. Thus, at the highest metal concentration, the major result was at 1000 µM SA; a nearly 103% increase was noticed compared to unpretreated plantlets (). In parallel, relative to the control, Cd-treated flax plantlets with 100 µM exhibited a slightly higher rate of protein oxidation (). However, it was observed that 8-h SA pretreatments (250 or 1000 µM) exhibited a significant reduction of carbonyl groups content with or without Cd treatments (). At 100 µM Cd, these main reductions were by about 30% and 50%, respectively, compared to unpretreated plantlets ().
3.3. Catalase, guaiacol peroxidase, ascorbate peroxidase, and superoxide dismutase activities
The effect of Cd on the activities of antioxidative enzymes () was significant in most Cd-contaminated leaves. CAT activity was significantly decreased in Cd-treated flax plantlets, and the effect was similar with the application of 8-h SA pretreatment (250 or 1000 µM; ). At 50 and 100 µM Cd, leaf POX and APX showed a significantly higher activities level than control, particularly at the highest concentration of the contaminant. Significant enhanced response in both enzymes activities was occurred by 8-h SA pretreatment followed by Cd exposure (). In contrast, SOD activity was declined by Cd stress (). However, SA (250 or 1000 µM) fully suppressed the Cd response of leaf SOD activity ().
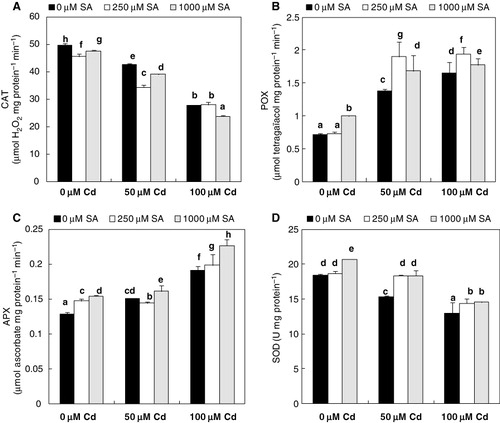
3.4. FRAP and DPPH-radical scavenging capacities
Under stress conditions, the antioxidant capacities, evaluated by monitoring FRAP and DPPH-radical scavenging activity, may not be sufficient to reduce the injurious effect of oxidative damages to flax leaves (). However, upon both 8-h SA pretreatments increases in the total antioxidant capacities were noticed in contaminated flax leaves, with respect to the unpretreated plantlets (). These SA-induced increases were in a dose-dependent manner. At 100 µM Cd, the maximum values have been registered at 1000 µM SA for both assays ().
4. Discussion
Cd is considered as one of the most toxic factors affecting plant growth performance. Thus, the responses of growth, under Cd stress, become the most important phenomena of plant physiology. In this work, we investigate the potential relationship between SA and H2O2 levels and growth performance and carbonyl groups. We also suggest that SA is able of generating H2O2 and imposed oxidative damage to growth and proteins.
Flax roots grown for 10 days with 50 and 100 µM CdCl2 adversely affected the shoot morphological aspects and shoot/root ratio is decreased (). These observations were accompanied by normally hydrated tissues (). Both processes could be a consequence of an important adaptive response of Cd-treated plantlets due to the fast inhibition of shoot growth (which limits plant biomass production) while root growth is better preserved (). It was reported that under Cd stress, growth processes may be regulated by exogenous plant hormone concentrations (Hassan et al. Citation2008). While low concentrations of salicylic acid (Belkadhi et al. Citation2013), cytokinin (Prasad Citation1995), gibberellin (Ghorbanli et al. Citation2000), auxin (Hu et al. Citation2013), and brassinosteroid (Hayat et al. Citation2007) accelerate growth of Cd-treated plants, abscisic acid (Hsu & Kao Citation2004), and jasmonic acid retard it (Maksymiec Citation2007). Moreover, in this experiment, the reductions in shoot/root ratio were alleviated by applying SA to Cd-treated flax plantlets (). This may be linked to the SA-increased levels of chlorophylls under Cd stress ().
Chlorophylls represent the central part of the entire metabolism of the green plant system. Therefore, any significant change in their level is likely the reason of large marked effects manifested primarily on the growth. In fact, it is well known that increase of chlorophyll occurs during growth. The results showed that Cd decreased the SPAD chlorophyll values () which agree with previous reports by Ishimaru et al. (Citation2012) for rice and Hasan et al. (Citation2013) for tomato. SA-increased chlorophyll concentration led to active photosynthetic activity causing growth. Furthermore, Li et al. (Citation1992) established that SA inhibited the activity of ACC synthase enzyme, preventing the formation of ethylene and chlorophyll alteration. Besides, under Cd stress, an increase in cytokinin content in 8-h SA-pretreated plantlets might also be responsible for their growth response. In fact, cytokinins have been shown to influence the activity of expansins (proteins involved in the control of cell expansion; Downes & Crowell Citation1998). Consequently stimulation of leaf expansion of flax plantlets pretreated with SA might be due to a SA-induced cytokinin synthesis. This assumption is supported by the fact that in this study the inhibitory effect of Cd on leaf growth was lower in plants which were pretreated with SA. Accordingly, Gadallah and El-Enany (Citation1999) reported a decline in the growth inhibitory effect of Cd in plants treated with exogenous cytokinin.
Since Cd-treatment is known to produce oxidative stress, increased oxidation of proteins might be due to this effect (). This is in agreement with previous studies by Djebali et al. (Citation2008). Since SA is a signaling molecule, it is probable that it reprogrammed the gene expression (Foyer & Noctor Citation2005), leading to de novo protein synthesis (Zhang et al. Citation1998) and a membrane repair mechanism (Belkadhi et al. Citation2013). The results of in vivo measurement of the effect of SA on the levels of protein and carbonyl groups () suggest a more direct effect of this hormone on antioxidative enzyme activities (Rodriguez-Serrano et al. Citation2009). In accordance with this, we found that 8-h SA pretreatment (250 or 1000 µM) enhanced the total antioxidant capacities in contaminated leaves (). The ability of SA to influence the activity of different antioxidant enzymes is known from the literature. Besides, the increased FRAP and DPPH-radical scavenging activity by injured leaves is also not excluded as contributing to the increase observed in the total protein contents of Cd-treated flax plantlets.
Other process implicated in the prevention of Cd-induced protein damage involves the regulation of the synthesis of antioxidant enzymes. In this study, Cd affected leaf SOD and CAT activities in flax plantlets, with their impact being higher at 100 µM Cd () but, tended to increase APX and POX activities, especially at this concentration () which is similar to the findings of Mishra et al. (Citation2006) in Bacopa monnieri. However, our results showed that the 8-h SA pretreatment caused a significant increase in POX, APX, and SOD in Cd-treated plantlets (). Similarly, SA is believed to inhibit CAT by the chelation of heme Fe and by causing conformational changes (Ruffer et al. Citation1995). The enhanced activities of these antioxidative enzymes under Cd stress, seems to be the result of de novo synthesis and/or activation of the enzymes, mediated through the transcription-specific genes (Foyer & Noctor Citation2005). Similarly, different plant species grown under Cd stress and pretreated with SA possessed higher antioxidative enzymatic activity, for instance rice (Panda & Patra Citation2007) and maize (Krantev et al. Citation2008).
It was proposed that high levels of endogenous SA can bind and inhibit H2O2-removing enzymes, such as CAT and APX (Durner & Klessig Citation1996). In addition, APX could post-transcriptionally suppressed by SA (Yuan & Lin Citation2004). In this study, under stress conditions, the observed stimulation of APX, POX, and SOD in leaves pretreated with low concentrations of SA (250 or 1000 µM) was coupled with a decrease in intracellular H2O2 (). Thus, the stimulation of these three major H2O2-degrading enzymes could cause further inactivation by dose-dependent manner of H2O2 accumulation. This suggests that SA-mediated activation of H2O2-degrading enzymes may further reduce H2O2 levels. It was found that increased H2O2 levels also act upstream of exogenous SA to induce endogenous SA (Leon et al. Citation1995) which leads to SA and H2O2 to compose a positive response to stress conditions. Thus, SA-pretreated plants may produce less ROS and at the same time enhance their own capacity to scavenge H2O2, which result in the less accumulation of ROS under Cd stress (Zhang & Chen Citation2011). Moreover, SA-induced redox regulation was found to be accompanied by accumulations of phytohormones like ethylene, nitric oxide, and jasmonate (Dat et al. Citation2003).
5. Conclusion
It is concluded that SA priming-induced improvements in Cd-treated flax plantlets growth were associated with chlorophyll synthesis, protein repair mechanisms and greater antioxidant capacities. In injured leaf cells, SA and H2O2 may probably use a common signaling mechanism to improve flax tolerance to Cd-induced oxidative stress. Our results suggest that these strategies can be of great help in the production of flax seed capable of growing vigorously under polluted conditions.
Acknowledgments
This research was supported by a grant from the Tunisian Ministry of Higher Education, Scientific Research and Technology, and the Doctoral School of the Faculty of Sciences of Tunis.
References
- Aebi H. 1984. Catalase in vitro. Methods Enzymol. 105:121–6.
- Beauchamp C, Fridovich I. 1971. Superoxide dismutase, improved assays and an assay applicable to acrylamide gels. Anal Biochem. 44:276–287. 10.1016/0003-2697(71)90370-8
- Belkadhi A, De Haro A, Soengas P, Obregon S, Cartea ME, Djebali W, Chaïbi W. 2013. Salicylic acid improves root antioxidant defense system and total antioxidant capacities of flax subjected to cadmium. OMICS. 17:398–406. 10.1089/omi.2013.0030
- Belkhadi A, Hediji H, Abbes Z, Nouairi I, Barhoumi Z, Zarrouk M, Chaïbi W, Djebali W. 2010. Effects of exogenous salicylic acid pretreatment on cadmium toxicity and leaf lipid content in Linum usitatissimum L. Ecotox Environ Safe. 73:1004–1011. 10.1016/j.ecoenv.2010.03.009
- Benzie IFF, Strain JJ. 1996. The ferric reducing ability of plasma (FRAP) as a measure of “antioxidant power”: the FRAP assay. Anal Biochem. 239:70–76. 10.1006/abio.1996.0292
- Bradford MM. 1976. A rapid and sensitive method for the quantitation of microgram quantities of protein utilizing the principle of protein-dye binding. Anal Biochem. 72:248–54. 10.1016/0003-2697(76)90527-3
- Brand-Williams W, Cuveleir ME, Berset C. 1995. Use of a free radical method to evaluate antioxidant activity. LWT-Food Sci Technol. 28:25–30.
- Chao Y, Hong CY, Kao CH. 2010. The decline in ascorbic acid content is associated with cadmium toxicity of rice seedlings. Plant Physiol Biochem. 48:374–381. 10.1016/j.plaphy.2010.01.009
- Dat JF, Pellinen R, Beeckman T, Van De Cotte B, Langebartels C, Kangasjarvi J, Inze D, Van Breusegem F. 2003. Changes in hydrogen peroxide homeostasis trigger an active cell death process in tobacco. Plant J. 33:621–632. 10.1046/j.1365-313X.2003.01655.x
- Djebali W, Gallusci P, Polge C, Boulila L, Galtier N, Raymond P, Chaibi W, Brouquisse R. 2008. Modifications in endopeptidase and 20S proteasome expression and activities in cadmium treated tomato (Solanum lycopersicum L.) plants. Planta. 227:625–639. 10.1007/s00425-007-0644-6
- Douchiche O, Soret-Morvan O, Chaïbi W, Morvan C, Paynel F. 2010. Characteristics of cadmium tolerance in ‘Hermes’ flax seedlings: contribution of cell walls. Chemosphere. 81:1430–1436. 10.1016/j.chemosphere.2010.09.011
- Downes BP, Crowell DN. 1998. Cytokinin regulates the expression of a soybean β-expansin gene by a post-transcriptional mechanism. Plant Mol Biol. 37:437–444. 10.1023/A:1005920732211
- Durner J, Klessig DF. 1996. Salicylic acid is a modulator of tobacco and mammalian catalases. J Biol Chem. 271:28492–28501. 10.1074/jbc.271.45.28492
- Ferreres F, Sousa C, Valentao P, Seabra RM, Pereira JA, Andrade PB. 2007. Tronchuda cabbage (Brassica oleracea L. var. costata DC) seeds: phytochemical characterization and antioxidant potential. Food Chem. 101:549–558. 10.1016/j.foodchem.2006.02.013
- Fielding JL, Hall J. 1978. A biochemical and cytochemical study of peroxidase activity in roots of Pisum sativum. J Exp Bot. 29:969–981. 10.1093/jxb/29.4.969
- Foyer CH, Noctor G. 2005. Redox homeostasis and antioxidant signaling: A metabolic interface between stress perception and physiological responses. Plant Cell. 1:1866–1875. 10.1105/tpc.105.033589
- Gadallah MAA, El-Enany AE. 1999. Role of kinetin in alleviation of copper and zinc toxicity in Lupinus termis plant. Plant Growth Regul. 29:151–160. 10.1023/A:1006245628188
- Gallego SM, Pena LB, Barcia RA, Azpilicueta CE, Iannone MF, Rosales EP, Zawoznik MS, Groppa MD, Benavides MP. 2012. Unravelling cadmium toxicity and tolerance in plants: insight into regulatory mechanisms. Environ Exp Bot. 83:33–46. 10.1016/j.envexpbot.2012.04.006
- Ghorbanli M, Kaveh SH, Sepehr MF. 2000. Effects of cadmium and gibberellin on growth and photosynthesis of Glycine max. Photosynthetica. 37:627–631. 10.1023/A:1007135928024
- Hasan SA, Wani AS, Irfan M, Hayat S. 2013. Brassinosteroids root drenching as an effective method of cadmium induced oxidative stress amelioration in Solanum lycopersicum. Inter J Chem Environ Biol Sci. 1:484–487.
- Hassan MJ, Zhang G, Zhu Z. 2008. Influence of cadmium toxicity on plant growth and nitrogen uptake in rice as affected by nitrogen form. J Plant Nutr. 31:251–262. 10.1080/01904160701853753
- Hayat S, Ali B, Hassan SA, Ahmad A. 2007. Effects of 28-homobrassinolide on salinity-induced changes in Brassica juncea. Turk J Biol. 31:141–146.
- Hayat S, Hayat Q, Alyemeni MN, Ahmad A. 2012. Salicylic acid enhances the efficiency of nitrogen fixation and assimilation in Cicer arietinum plants grown under cadmium stress. J Plant Interact. 9:35–42.
- Hsu YT, Kao CH. 2004. Cadmium toxicity is reduced by nitric oxide in rice leaves. Plant Growth Regul. 42:227–238. 10.1023/B:GROW.0000026514.98385.5c
- Hu, Y, Xie Q, Chua NH. 2013. The Arabidopsis auxin-inducible gene ARGOS controls lateral organ size. Plant Cell. 15:1951–1961. 10.1105/tpc.013557
- Ishimaru Y, Takahashi R, Bashir K, Shimo H, Senoura T, Sugimoto K, Ono K, Yano M, Ishikawa S, Arao T, et al. 2012. Characterizing the role of rice NRAMP5 in manganese, iron and cadmium transport. Sci Rep. 2:286. 10.1038/srep00286
- Janda T, Szalai G, Rios-Gonzalez K, Veisz O, Páldi E. 2003. Comparative study of frost tolerance and antioxidant activity in cereals. Plant Sci. 164:301–306. 10.1016/S0168-9452(02)00414-4
- Krantev A, Yordanova R, Janda T, Szalai G, Popova L. 2008. Treatment with salicylic acid decreases the effect of cadmium on photosynthesis in maize plants. J Plant Physiol. 165:920–931. 10.1016/j.jplph.2006.11.014
- Leon J, Lawton MA, Raskin I. 1995. Hydrogen peroxide stimulates salicylic acid biosynthesis in tobacco. Plant Physiol. 108:1673–1678.
- Li N, Parsons BL, Liu DR, Mattoo AK. 1992. Accumulation of wound-inducible ACC synthase transcript in tomato fruit is inhibited by salicylic acid and polyamines. Plant Mol Biol. 18:477–487. 10.1007/BF00040664
- Maksymiec W. 2007. Signaling responses in plants to heavy metal stress. Acta Physiol Plant. 29:177–187. 10.1007/s11738-007-0036-3
- Mishra S, Srivastava S, Tripathi RD, Kumar R, Seth CS, Gupta DK. 2006. Lead detoxification by coontail (Ceratophyllum demersum L.) involves induction of phytochelatins and antioxidant system in response to its accumulation. Chemosphere. 65:1027–1039. 10.1016/j.chemosphere.2006.03.033
- Mittler R, Vanderauwera S, Gollery M, Van Breusegem F. 2004. Reactive oxygen gene network of plants. Trends Plant Sci. 9:490–498. 10.1016/j.tplants.2004.08.009
- Nakano Y, Asada K. 1981. Hydrogen peroxide is scavenged by ascorbate-specific peroxidase in spinach chloroplast. Plant Cell Physiol. 22:676–690.
- Noriega G, Caggiano E, Lecube ML, Cruz DS, Batlle A, Tomaro M, Balestrasse KB. 2012. The role of salicylic acid in the prevention of oxidative stress elicited by cadmium in soybean plants. Biometals. 25:1155–1165. 10.1007/s10534-012-9577-z
- O'Kane D, Gill V, Boyd P, Burdon R. 1996. Chilling, oxidative stress and antioxidant responses in Arabidopsis thaliana callus. Planta. 198:371–377. 10.1007/BF00620053
- Pérez-Ruiz JM, Spínola MC, Kirchsteiger K, Moreno J, Sahrawy M, Cejudo FJ. 2006. Rice NTRC is a high-efficiency redox system for chloroplast protection against oxidative damage. Plant Cell. 18:2356–2368. 10.1105/tpc.106.041541
- Panda SK, Patra HK. 2007. Effect of salicylic acid potentiates cadmium-induced oxidative damage in Oryza sativa L. leaves. Acta Physiol Plant. 29:567–575. 10.1007/s11738-007-0069-7
- Prasad M. 1995. Cadmium toxicity and tolerance in vascular plants. Env Exp Bot. 35:525–545. 10.1016/0098-8472(95)00024-0
- Reznick AZ, Packer L. 1994. Oxidative damage to protein: spectrophotometric method for carbonyl assay. Methods Enzymol. 233:357–363.
- Rodriguez-Serrano M, Romero-Puertas MC, Pazmino DM, Testillano PS, Risueno MC, del Rio LA, Sandalio LM. 2009. Cellular response of pea plants to cadmium toxicity: cross talk between reactive oxygen species, nitric oxide, and calcium. Plant Physiol. 15:229–243. 10.1104/pp.108.131524
- Ruffer M, Steipe B, Zenk MH. 1995. Evidence against specific binding of salicylic acid to plant catalase. FEBS Lett. 377:175–180. 10.1016/0014-5793(95)01334-2
- Shi GR, Cai QS, Liu QQ, Wu L. 2009. Salicylic acid-mediated alleviation of cadmium toxicity in hemp plants in relation to cadmium uptake, photosynthesis, and antioxidant enzymes. Acta Physiol Plant. 31:969–977. 10.1007/s11738-009-0312-5
- Xu L, Dong Y, Fan Z, Kong J, Liu S, Bai X. 2013. Effects of the application of exogenous NO at different growth stage on the physiological characteristics of peanut grown in Cd-contaminated soil. J Plant Interact. 9:285–296.
- Yuan S, Lin HH. 2004. Transcription, translation, degradation and circadian clock. Biochem Biophys Res Commun. 321:1–6. 10.1016/j.bbrc.2004.06.093
- Zhang S, Du H, Klessig DF. 1998. Activation of tobacco SIP kinase by both a cell wall-derived carbohydrate elicitor and purified proteinaceous elicitins from Phytophthora spp. Plant Cell. 10:435–449.
- Zhang WN, Chen WL. 2011. Role of salicylic acid in alleviating photochemical damage and autophagic cell death induction of cadmium stress in Arabidopsis thaliana. Photochem Photobiol Sci. 10:947–955. 10.1039/c0pp00305k
- Zhang F, Wan X, Zhong Y. 2013. Nitrogen as an important detoxification factor to cadmium stress in poplar plants. J Plant Interact. 9:249–258.