Abstract
This study investigated the plant growth promotion and stress mitigation effects of Penicillium species RDA01, NICS01, and DFC01 on sesame (Sesamum indicum L.) plants. The fungal isolates NICS01 and DFC01 significantly enhanced shoot length, root length, and fresh and dry seedling weight, due to the secretion of various concentrations of amino acids (Asp, Thr, Ser, Asn, Glu, Gly, Ala, Val, Met, Ile, Leu, Tyr, Phe, Lys, His, Try, and Arg). Penicillium sp. NICS01 increased the amount of chlorophylls, proteins, amino acids, and lignans in the sesame plants more so than in controls. Sesame plant growth was stunted by high soil salinity, and application of the three fungal isolates increased plant survival. The RDA01 and NICS01 strains significantly increased shoot length and fresh and dry seedling weights under salt stress conditions. In addition, an in vitro study of the Penicillium spp. revealed their antagonistic activity toward the pathogenic fungi Fusarium spp. Fusarium spp. reduce shoot length; co-inoculation with the NICS01 or DFC01 isolates significantly increased shoot length in infected plants. Our results suggest that exogenous application of the Penicillium sp. NICS01 can act as a biofertilizer and a biocontrol agent to improve plant growth and enhance plant survival against salt stress and Fusarium infection.
Introduction
Crop productivity is severely reduced by environmental stress from both biotic and abiotic factors. Approximately one-third of the irrigated agricultural land is affected by salinity (Munns & Tester Citation2008). Plants have the capability to cope with limited levels of salinity stress through antioxidants and other secondary metabolic pathways. Exogenous treatment with plant growth regulators, such as polyamines, gibberellin (GA), auxin, and zeatin, has been used to protect plants from salt stress damage (Egamberdieva Citation2009; Hamayun et al. Citation2010; Radhakrishnan & Lee Citation2014), and beneficial fungal associations increase plant tolerance to salt stress (Khan, Hamayun, Ahmad et al. Citation2011). We recently identified GA-producing endophytic fungi that can ameliorate salt stress effects in soybean (Glycine max) plants, through the regulation of antioxidants and endogenous phytohormones (Radhakrishnan, Khan, Lee Citation2013). Phytopathogens are also a major cause of declining crop yields. Harmful fungi in soil can cause plant diseases, and among these, Fusarium spp. infect the roots of crop plants (Gajbhiye et al. Citation2010; Hariprasad et al. Citation2011). Fusarium spp. survive for several years as a large group of filamentous fungi that are abundant in the soil (Leslie & Summerell Citation2006). Some pathogenic virulent Fusarium strains produce mycotoxin in the infected tissue and grain, which leads to yield losses and poor grain quality, and the consumption of diseased grain causes serious health problems in humans and animals (Kang et al. Citation2013). Soil-borne pathogenic fungi have a broad range of host plants, and can survive under different environmental conditions (Hammami et al. Citation2013). The application of chemical fungicides can control Fusarium infections in crop plants, but large quantities of these are deposited in the soil and inhibit the growth of beneficial microbes and other organisms (Hariprasad et al. Citation2011). In contrast, nonpathogenic and plant growth-promoting microbes in the soil can interfere with pathogens through parasitism, competition for nutrients, and antibiosis (Alabouvette et al. Citation2009).
Soil is a primary source of fungal growth, and is associated with the roots of all plant species. Fungi produce a wide range of bioactive metabolites, which can improve plant growth (Khan, Hamayun, Ahmad et al. Citation2011; Waqas et al. Citation2014). In addition, fungi supply inorganic nutrients to plants, such as ammonium, nitrate, and phosphate (Seastedt et al. Citation2008), and they are used as biofertilizers. Rhizosphere microorganisms can overcome competition with other soil factors and survive under variable environmental conditions (Ferrara et al. Citation2012). Species of Penicillium are ubiquitous soil fungi and their conidia are easily distributed in nature through the atmosphere and soil. Several reports have suggested that Penicillium spp. interact with roots of crop plants to enhance the plant growth (Hyakumachi Citation1994; Shivanna et al. Citation1994; Khan et al. Citation2008). They are potent plant growth-promoting fungus, and secrete the plant hormones, indole-3-acetic acid (IAA) and GA, and involve in phosphate solubilization, which may be a reason to increase the plant growth (Khan et al. Citation2008; Khan, Hamayun, Kim et al. Citation2011; Radhakrishnan, Shim et al. Citation2013). Some species of Penicillium are well known for their antagonistic activity against pathogen by producing antibiotics and induce resistance in plants by activating multiple defense signals (Hossain et al. Citation2007). They can survive under environmental stress condition such as saline soil and promote plant growth against salt stress (Khan, Hamayun, Ahmad et al. Citation2011; Khan, Hamayun, Kim et al. Citation2011). Several antagonistic microorganisms, Bacillus spp., Pseudomonas spp., Trichoderma spp., and Sebacinales spp., have been studied extensively and proved their biocontrol activity against the growth of Fusarium (Saikia et al. Citation2006; Shoresh et al. Citation2010; Hariprasad et al. Citation2011). Recently, Sartaj et al. (Citation2011) reported that Penicillium sp. EU0013 inoculation is capable to enhancing growth and protecting tomato plants against Fusarium wilt.
Sesame (Sesamum indicum L.) is an economically important cash crop, and is widely grown in tropical and subtropical countries. Sesame seeds contain 45–60% oil, 18–28% protein, and other phytocompounds. The productivity of sesame is reduced by low genetic yield potential, and is susceptible to many biotic and abiotic stresses (Jyothi et al. Citation2011). Fusarium oxysporum and Macrophomina phaseolina are the causal agents of wilt and charcoal rot disease, and are the most serious and destructive pathogens in reducing sesame yield (Kumar et al. Citation2011). Currently, farmers are using chemical fertilizers to enhance sesame yield, and chemical pesticides, fungicides, and bactericides to control plant diseases. These chemicals are expensive and harmful to other beneficial biological organisms and pollute the environment (Hariprasad et al. Citation2011). Sesame can moderately tolerate saline soils (Yousif et al. Citation1972), and biofertilizers may be useful in remediating salt stress effects and increase plant yield. To date, there have been no studies that have investigated the presence of amino acids in Penicillium fungal cultures, and their role in plant growth promotion. Therefore, the aim of the present study was to evaluate the ability of selected amino acid-producing fungi to enhance plant growth, and to assess their biological activity against salt stress and Fusarium infection in sesame plants.
Materials and methods
Isolation and identification of rhizosphere soil fungi
Three dominant fungal strains were isolated from the rhizosphere soil of peanut (Arachis hypogaea) plants grown in a greenhouse at the National Institute of Crop Science, Miryang, South Korea, and cultured in potato dextrose broth (PDB). Genomic DNA was extracted from the fungal isolates using a fungal genomic DNA preparation kit (Solutions for Genetic Technologies, Daejeon, South Korea) and polymerase chain reaction was performed according to an established protocol (Radhakrishnan, Shim et al. Citation2013). The fungal isolates were identified by sequencing the internal transcribed region (ITS) of 18s rDNA using universal primers: ITS-1 (5′-TCC GTA GGT GAA CCT GCG G-3′) and ITS-4 (5′-TCC TCC GCT TAT TGA TAT GC-3′) as described by Radhakrishnan, Khan, Lee (Citation2013). The BLAST search program (http://blast.ncbi.nlm.nih.gov) was used to compare the nucleotide sequence similarity of the ITS region in related fungi. E-value of three fungal isolates was zero and length of sequence amplicon was 542–556. On the basis of the sequence similarity, the fungal isolates were identified as Penicillium sp. RDA01 (accession no: KC710943), Penicillium sp. NICS01 (accession no: JX481980), and Penicillium sp. DFC01 (accession no: KC852152).
Amino acid analyses of the fungal cultures
The culture filtrates were separated from the fungal isolates of Penicillium spp. RDA01, NICS01, and DFC01 cultured at 28 ± 2°C for 14 days, and filtrated through a nylon 66 syringe filter (0.452 µm). The amino acids Asp, Thr, Ser, Asn, Glu, Gly, Ala, Val, Met, Ile, Leu, Tyr, Phe, Lys, His, Try, and Arg were identified and quantified according to the method described by Radhakrishnan, Pae et al. (Citation2013). Briefly, 1 mL of culture filtrate was filtered through a nylon 66 syringe filter (0.452 µm) and 0.1 mL was then mixed with 0.9 mL lithium citrate-loading buffer (pH 2.2). The amino acids were determined using a Biochrom 30 (Cambridge, UK) amino acid analyzer on a single lithium cation-exchange resin column (4.6 × 200 mm), using ninhydrin as the color reactant. Chromatography data were analyzed using Ezchrom E software. The quantity of amino acids was calculated by comparison with a known concentration of amino acid standard.
Fungal treatments and sesame plant growth
Sesame seeds (Pyeongang, Kangbaek, and 90 Day) were surface sterilized with 0.5% sodium hypochloride, and rinsed several times with sterile distilled water. The culture filtrates of RDA01, NICS01, and DFC01 were applied to the seeds and shaken at 120 rpm for 4 hours; seeds without fungal treatment served as controls. The soaked seeds were placed in an autoclaved Petri dish containing three layers of filter paper and maintained in the dark at 27°C for 3 days; distilled water was applied at regular intervals. The experiment was repeated for three times with 30 seeds. The lengths of the roots and shoots and the fresh and dry seedling weights were measured after 3 days. In a pot experiment, RDA01, NICS01, and DFC01 were applied to autoclaved Baroker soil, and seeds (cv. 90 Day) were inoculated on fungi-pretreated or untreated soil. The experiment was conducted with a randomized block design, with six replications. The aerial parts of the plants were harvested at 65 days. The collected plants were maintained at −80°C for biochemical analyses.
Photosynthetic pigments were extracted from leaves ground with 80% acetone. Chlorophylls and carotenoids were estimated according to the methods of Arnon (Citation1949) and Lichtenthaler (Citation1987), respectively. The nitrogen and protein content of the freeze-dried sesame plant samples were analyzed using the Rapid N Cube method. For the amino acid analysis, plant samples (50 mg) were dissolved in water and centrifuged at 13,000 rpm at 4°C for 30 min. The supernatants were filtered through a nylon 66 syringe filter (0.452 µm) and the amino acids were quantified according to the method of Radhakrishnan, Pae et al. (Citation2013). Lignans (sesamin and sesamolin) were estimated from the methanol extracted by high-performance liquid chromatography, according to the method described by Radhakrishnan, Pae et al. (Citation2013). The powdered plant sample (1 g) was extracted with methanol for 24 h and filtered through a nylon syringe filter (0.45 µm), and 0.7 mL of filtrate was injected into a LiChrospher® 100 RP-18 column (5 µm) at 30°C. The mobile phase was 60% methanol at 0.8 mL/min and lignans were detected at 361 nm and quantified by their standard peak area.
Fungal treatments and salt stress in sesame plants
The fungi-pretreated seeds were placed in autoclaved Baroker soil and distilled water was applied at regular intervals. After 15 days, uniformly sized seedlings were transplanted into pots containing sterilized Baroker soil. The 45-day-old sesame plants were treated with 150 mM NaCl for salinity stress. The aerial parts of the plants were harvested, and shoot length and fresh and dry shoot weights were measured at 50 days.
Evaluation of the antagonistic effects of Penicillium spp. on Fusarium disease in sesame plants
The antagonistic effects of the Penicillium spp. on Fusarium were assayed using the method of Latha et al. (Citation2011). Seven-day-old RDA01, NICS01, DFC01 and Fusarium sp. cultures in PDB broth were applied to a 4-mm disc placed on the opposite side of Petri dishes containing potato dextrose agar (PDA) medium with three replicates. The Petri dishes were incubated for 14 days at 28 ± 2°C and Fusarium mycelium growth was measured. The surface-sterilized seeds were sown in pots containing RDA01-, NICS01-, and DFC01-treated Baroker soil in a greenhouse. Fusarium culture was applied to the Penicillium-inoculated plants at 50 days. The experiment had a randomized block design with five replications, each consisting of four plants per pot. Shoot length was measured 15 days after the first appearance of wilting.
Statistical analyses
Growth (n = 12) and biochemical (n = 3) parameters of the sesame plants were analyzed, and statistical analyses were performed using SPSS 11.5 software (SPSS Inc., Chicago, IL, USA). Data were subjected to analysis of variance, and mean values between treatments were compared using Duncan's multiple-range test at a significance level of P < 0.05. Means followed by the same letter were not significantly different.
Results and discussion
Effect of Penicillium spp. RDA01, NICS01, and DFC01 on sesame seedling growth
The fungi isolated from the rhizosphere soil promote plant growth, and could be used as biocontrol agents, but amino acid changes during the fungal interaction have rarely been studied. Several reports have suggested that plant growth is enhanced by the application of soil fungi (Ruanpanun et al. Citation2010; Tchameni et al. Citation2011; Dutt et al. Citation2013). In the present study, three soil fungal isolates, Penicillium spp. RDA01, NICS01, and DFC01, were tested to ascertain their effect on sesame plant growth. We found significant variations in the growth of fungi-inoculated sesame (cv. Pyeongang, Kangbaek, and 90 Day) compared to untreated plants ( and ). The growth promotion of sesame plants was differentially influenced by cultivars and fungal isolates. The Pyeongang cultivar seeds associated with Penicillium spp. RDA01 and NICS01 exhibited a remarkable increase in shoot and root length and fresh and dry seedling weight, whereas the fungal inoculations RDA01 and NICS01 enhanced the growth of the Kangbaek cultivar (shoot length, root length, fresh and dry seedling weight) compared to the controls. A positive interaction between RDA01, NICS01, and the 90 Day cultivar sesame plants was observed, by increasing shoot length (4.0%, 7.2%, and 2.2%, respectively) and root length (3.3%, 2.9%, and 8.9%, respectively), whereas the fungal isolate RDA01 increased fresh (4.7%) and dry (4.5%) seedling weight. DFC01 inhibited the seedling growth of the three sesame cultivars compared to the controls. The results of this preliminary study suggest that exogenous treatment with Penicillium spp. RDA01 and NICS01 may be an effective bio-inoculant strategy.
Secretion of amino acids from Penicillium spp. RDA01, NICS01, and DFC01 in the fungal medium
Plant growth-promoting substances produced by fungi are important in promoting plant growth. In a previous study, we found IAA in the culture filtrate of fungi, which may enhance seedling growth (Radhakrishnan, Shim et al. Citation2013). Khan, Hamayun, Kim et al. (Citation2011) reported that the production of GAs in a Penicillium minioluteum culture caused an increase in soybean plant growth. In the current study, fungi-treated sesame seeds exhibited a significant enhancement in seedling root growth, possibly due to the presence of amino acids in the culture medium (). The concentration of secreted amino acids differed between RDA01, NICS01, and DFC01. The amino acid Cys (0.27 mg/L) was only found in the NICS01 culture, whereas Asp, Thr, Ser, Glu, Ala, Val, Met, Ile, Leu, Tyr, Phe, Lys, His, and Arg were found in all the fungal cultures. The highest concentration of amino acids was found in the RDA01 (0.12 mg/L Asp, 0.11 mg/L Thr, 0.1 mg/L Ser, 0.22 mg/L Glu, 0.27 mg/L Ala, 0.07 mg/L Met, 0.25 mg/L Leu, 0.41 mg/L Lys, 0.14 mg/L His, and 0.38 mg/L Arg) and DFC01 (0.11 mg/L Asp, 0.12 mg/L Thr, 0.11 mg/L Ser, 0.33 mg/L Glu, 0.14 mg/L Gly, 0.26 mg/L Ala, 0.11 mg/L Val, 0.07 mg/L Met, 0.17 mg/L Ile, 0.24 mg/L Leu, 0.18 mg/L Tyr, 0.17 mg/L Phe, and 0.42 mg/L Lys) fungal cultures. The exogenous application of amino acids is well known to improve the plant growth (El-Samad et al. Citation2011; Sudadi Citation2012). Very few studies have been conducted to ascertain the role of fungal amino acids on plant growth (Whiteside, Garcia, Treseder Citation2012). Arginine and lysine are abundant in Penicillium culture medium. Arginine pretreatment increases seed germination, plant growth, and tolerance of low temperatures. Lysine is synthesized from aspartic acid, and regulates various plant processes. The secretion of plant growth-regulating compounds from fungi is one of the reasons they increase crop productivity; such growth-regulating compounds can influence plant growth in stressful environments (Khan, Hamayun, Ahmad et al. Citation2011). The current study suggests that the presence of amino acids in the culture medium of RDA01, NICS01, and DFC01 increases seedling growth.
Effect of fungal treatments on the primary and secondary metabolites of sesame plants
Fungal associations with plants influence the primary and secondary metabolism of plants at all developmental stages. Photosynthesis is an important primary mechanism, and the main source of energy for plants. Its efficiency is related to photosynthetic pigments such as chlorophylls and carotenoids. Leaf chlorophyll a was increased in fungi-treated plants more so than in the controls; there were no significant changes to chlorophyll b and the total chlorophyll content in plants exposed to RDA01 and NICS01 (). Recently, Vafadar et al. (Citation2014) reported that plant growth-promoting bacteria and fungi increased chlorophyll content in Stevia rebaudiana. An increase in chlorophyll content accelerates photosynthesis, which leads to enhanced plant growth (Dutt et al. Citation2013). DFC01-treated plants exhibited a lower level of chlorophyll and carotenoid contents. NICS01 increased nitrogen, protein, and the amino acids Thr, Ser, Asn, Gly, Ala, Val, Met, Ile, Leu, Tyr, Phe, Lys, His, Try, and Arg ( and ). Soil fungi supply inorganic nutrients, which are essential for the biological activities of plants. Soil microorganisms release nitrogen in the form of amino acids, resulting from N2 fixation (Ferrara et al. Citation2012), and these amino acids are absorbed by plants (Whiteside, Digman et al. Citation2012). Recently, Tchameni et al. (Citation2011) also reported significantly greater amino acid synthesis in fungi-inoculated plants compared to control plants. Sesamin and sesamolin are major lignans in sesame plants, and these exhibit their antioxidant activity against pathogen infection (Jeng & Hou Citation2005) by suppressing reactive oxygen species generation and mitogen-activated protein kinases (Gill et al. Citation2002). In the present study, both sesamin and sesamolin content were higher in the fungi-associated plants than in the control plants (). Sesamin content was increased 7-fold by RDA01 and 15-fold by NICS01 compared to the controls, whereas NICS01-inoculation resulted in a 6-fold increase in sesamolin levels. An increase in lignans by soil organisms may confer health benefits to sesame plants.
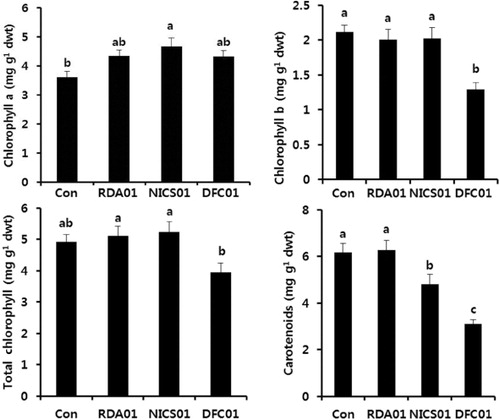
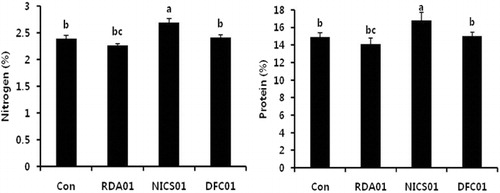
Effect of Penicillium spp. on salt stress and Fusarium disease in sesame plants
Plant growth regulator-producing microorganisms stimulate a greater uptake of nutrients by plants, thereby improving plant health under stressful conditions (Egamberdieva & Kucharova Citation2009). High soil salinity decreases sesame plant growth, while the association of fungi with sesame plants resulted in considerable increases in shoot length and plant fresh and dry weight under salt stress conditions (). The external application of RDA01 and NICS01 fungal cultures completely halted shoot length reduction in sesame plants damaged by salt stress. The fresh and dry weights of salt-affected plants also significantly increased after RDA01 and NICS01 application. A similar response of fungi against abiotic stress was reported in soybean (Khan, Hamayun, Ahmad et al. Citation2011) and rice (Redman et al. Citation2011). The results of the present study suggest that the growth enhancement of sesame plants in saline soil may be due to fungi secreting amino acids.
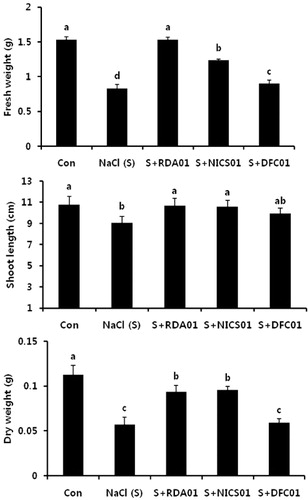
Antagonistic organisms induce systemic resistance in plants against pathogen attack. The production of antibiotic substances and secondary metabolites in these organisms causes an inhibition of phytopathogenic fungal growth (Ruanpanun et al. Citation2010). Fusarium wilt is an important disease in sesame, and causes stunted plant growth and reduced yield (Kumar et al. Citation2011). Our in vitro study revealed that RDA01, NICS01, and DFC01 inhibit Fusarium fungal growth by 49% in PDA medium (). The antagonistic fungi produced volatile secondary metabolites inhibit the growth of Fusarium and protect the plant disease against pathogens (Minerdi et al. Citation2008, Citation2009, Citation2011). The results of the pot experiment under greenhouse conditions confirmed the biocontrol ability of NICS01 and DFC01 against Fusarium, as evidenced by increases in plant growth (). Our results are consistent with those of previous studies that show that Penicillium spp. can prevent Fusarium infection in crop plants (Alam et al. Citation2011; Ting et al. Citation2012). The secretion of a wide range of antibiotics, such as 2,4-diacetylphloroglucinol, pyoluteorin, pyrrolnitrin, pyocyanin, oligomycin, and phenazine, by biocontrol agents may inhibit pathogenic fungi (Nielson et al. Citation1998).
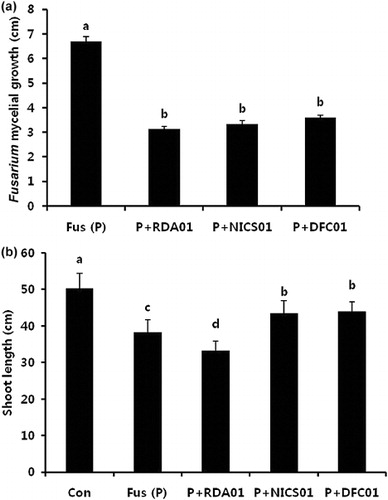
In conclusion, the amino acid-producing Penicillium spp. RDA01, NICS01, and DFC01 increased sesame plant growth. Moreover, NICS01 mitigated the lethal effects of salt stress and protected sesame plants from Fusarium infection. The results of this study suggest that NICS01 can be used as a biofertilizer and a biocontrol agent, because it increases plant growth under biotic and abiotic stress conditions. Therefore, the application of NICS01 would be favorable for the cultivation of crop plants, even in salt stress environments.
Funding
This study was supported by a grant from the Post-doctoral Fellowship Program of the National Institute of Crop Science, Rural Development Administration, Republic of Korea, and by the Korean Ministry of the Environment as an Eco-Innovation Project.
Additional information
Funding
References
- Alabouvette C, Olivain C, Migheli Q, Steinberg C. 2009. Microbiological control of soil-borne phytopathogenic fungi with special emphasis on wilt-inducing Fusarium oxysporum. New Phytol. 184:529–544. 10.1111/j.1469-8137.2009.03014.x
- Alam SS, Sakamoto K, Inubushi K. 2011. Biocontrol efficiency of fusarium wilt diseases by a root-colonizing fungus Penicillium sp. Soil Sci Plant Nut. 57:204–212. 10.1080/00380768.2011.564996
- Arnon DI. 1949. Copper enzyme in isolated chloroplasts and polyphenoloxidase in Beta vulgaris. Plant Physiol. 24:1–15. 10.1104/pp.24.1.1
- Dutt S, Sharma SD, Kumar P. 2013. Inoculation of apricot seedlings with indigenous arbuscular mycorrhizal fungi in optimum phosphorus fertilization for quality growth attributes. J Plant Nutr. 36:15–31. 10.1080/01904167.2012.732648
- Egamberdieva D. 2009. Alleviation of salt stress by plant growth regulators and IAA producing bacteria in wheat. Acta Physiol Plant. 31:861–864. 10.1007/s11738-009-0297-0
- Egamberdieva D, Kucharova Z. 2009. Selection for root colonizing bacteria stimulating wheat growth in saline soils. Biol Fert Soils. 45:563–571. 10.1007/s00374-009-0366-y
- El-Samad HMA, Shaddad MAK, Barakat N. 2011. Improvement of plants salt tolerance by exogenous application of amino acids. J Med Plants Res. 5:5692–5699.
- Ferrara FIS, Oliveira ZM, Gonzales HHS, Floh EIS, Barbosa HR. 2012. Endophytic and rhizospheric enterobacteria isolated from sugar cane have different potentials for producing plant growth-promoting substances. Plant Soil. 353:409–417. 10.1007/s11104-011-1042-1
- Gajbhiye A, Rai AR, Meshram SU, Dongre AB. 2010. Isolation, evaluation and characterization of Bacillus subtilis from rhizospheric soil with biocontrol activity against Fusarium oxysporum. World J Microbiol Biotechnol. 26:1187–1194. 10.1007/s11274-009-0287-9
- Gill R, Soriano M, Blomgren K, Hagberg H, Wybrecht R, Miss MT, Hoefer S, Adam G, Niederhauser O, Kemp JA, Loetscher H. 2002. Role of caspase-3 activation in cerebral ischemia-induced neurodegeneration in adult and neonatal brain. J Cereb Blood Flow Metab. 22:420. 10.1097/00004647-200204000-00006
- Hamayun M, Khan SA, Khan AL, Shin JH, Ahmad B, Shin DH, Lee IJ. 2010. Exogenous gibberellic acid reprograms soybean to higher growth and salt stress tolerance. J Agric Food Chem. 58:7226–7232. 10.1021/jf101221t
- Hammami I, Hsouna AB, Hamdi N, Gdouraf R, Triki MA. 2013. Isolation and characterization of rhizosphere bacteria for the biocontrol of the damping-off disease of tomatoes in Tunisia. C R Biol. 336:557–564. 10.1016/j.crvi.2013.10.006
- Hariprasad P, Divakara ST, Niranjana SR. 2011. Isolation and characterization of chitinolytic rhizobacteria for the management of Fusarium wilt in tomato. Crop Prot. 30:1606–1612. 10.1016/j.cropro.2011.02.032
- Hossain MM, Sultana F, Kubota M, Koyama H, Hyakumachi M. 2007. The plant growth-promoting fungus Penicillium simplicissimum GP17-2 induces resistance in Arabidopsis thaliana by activation of multiple defense signals. Plant Cell Physiol. 48:1724–1736. 10.1093/pcp/pcm144
- Hyakumachi M. 1994. Plant-growth-promoting fungi from turfgrass rhizosphere with potential for disease suppression. Soil Microorg. 44:53–68.
- Jeng KCG, Hou RCW. 2005. Sesamin and sesamolin: nature's therapeutic lignans. Curr Enzym Inhib. 1:11–20. 10.2174/1573408052952748
- Jyothi B, Ansari NA, Vijay Y, Anuradha G, Sarkar A, Sudhakar R, Siddiq EA. 2011. Assessment of resistance to fusarium wilt disease in sesame (Sesamum indicum L.) germplasm. Aust Plant Pathol. 40:471–475. 10.1007/s13313-011-0070-x
- Kang W-R, Hwang D-J, Bae S-C, Lee T, Kim S, Ahn, I-P. 2013. Evaluation of fusarium head blight in barley infected by Fusarium graminearum. J Microbiol. 51:540–543. 10.1007/s12275-013-3338-8
- Khan AL, Hamayun M, Ahmad N, Hussain J, Kang SM, Kim YH, Adnan M, Tang DS, Waqas M, Radhakrishnan R, et al. 2011. Salinity stress resistance offered by endophytic fungal interaction between Penicillium minioluteum LHL09 and Glycine max. L. J Microbiol Biotechnol. 21:893–902. 10.4014/jmb.1103.03012
- Khan AL, Hamayun M, Kim YH, Kang SM, Lee IJ. 2011. Ameliorative symbiosis of endophyte (Penicillium funiculosum LHL06) under salt stress elevated plant growth of Glycine max L. Plant Physiol Biochem. 49:852–861. 10.1016/j.plaphy.2011.03.005
- Khan SA, Hamayun M, Yoon H, Kim HY, Suh SJ, Hwang SK, Kim JM, Lee IJ, Choo YS, Yoon UH, et al. 2008. Plant growth promotion and Penicillium citrinum. BMC Microbiol. 8:231– 10.1186/1471-2180-8-231
- Kumar S, Aeron A, Pandey P, Maheshwari DK. 2011. Ecofriendly management of charcoal rot and fusarium wilt diseases in sesame (Sesamum indicum L.). In: Maheshwari DK, editor. Bacteria in agrobiology: crop ecosystems. Heidelberg: Springer; p. 387–405.
- Latha P, Anand T, Prakasama V, Jonathan EI, Paramathma M, Samiyappan R. 2011. Combining Pseudomonas, Bacillus and Trichoderma strains with organic amendments and micronutrient to enhance suppression of collar and root rot disease in physic nut. Appl Soil Ecol. 49:215–223. 10.1016/j.apsoil.2011.05.003
- Leslie JF, Summerell BA. 2006. Fusarium verticillioides (Saccardo) Nirenberg. In: Leslie JF, Summerell BA, editors. The fusarium laboratory manual. Oxford: Blackwell; p. 274–279.
- Lichtenthaler HK. 1987. Chlorophylls and carotenoids: pigment of photosynthetic biomembranes. Method Enzymol. 148:350–382.
- Minerdi D, Bossi S, Gullino ML, Garibaldi A. 2009. Volatile organic compounds: a potential direct long-distance mechanism for antagonistic action of Fusarium oxysporum strain MSA 35. Environ Microbiol. 11:844–854. 10.1111/j.1462-2920.2008.01805.x
- Minerdi D, Bossi S, Maffei ME, Gullino ML, Garibaldi A. 2011. Fusarium oxysporum and its bacterial consortium promote lettuce growth and expansin A5 gene expression through microbial volatile organic compound (MVOC) emission. FEMS Microbiol Ecol. 76:342–351. 10.1111/j.1574-6941.2011.01051.x
- Minerdi D, Moretti M, Gilardi G, Barberio C, Gullino ML, Garibaldi A. 2008. Bacterial ectosymbionts and virulence silencing in a Fusarium oxysporum strain. Environ Microbiol. 10:1725–1741. 10.1111/j.1462-2920.2008.01594.x
- Munns R, Tester M. 2008. Mechanisms of salinity tolerance. Ann Rev Plant Biol. 59:651–681. 10.1146/annurev.arplant.59.032607.092911
- Nielson MN, Sorensen J, Fels J, Pdersen HC. 1998. Secondary metabolite and endochitinase dependent antagonism toward plant pathogenic microfungi of Pseudomonas fluorescens isolates from sugar beet rhizosphere. Appl Env Microbiol. 64:3563–3569.
- Radhakrishnan R, Khan AL, Lee IJ. 2013. Endophytic fungal pre-treatments of seeds alleviates salinity stress effects in soybean plants. J Microbiol. 51:850–857. 10.1007/s12275-013-3168-8
- Radhakrishnan R, Lee IJ. 2014. Effect of low dose of spermidine on physiological changes in salt stressed cucumber plants. Russ J Plant Physiol. 61:90–96. 10.1134/S1021443714010129
- Radhakrishnan R, Pae S-B, Shim K-B, Baek I-Y. 2013. Penicillium sp. mitigates Fusarium-induced biotic stress in sesame plants. Biotech Let. 35:1073–1078. 10.1007/s10529-013-1177-7
- Radhakrishnan R, Shim KB, Lee BW, Hwang CD, Pae SB, Park CH, Kim SU, Lee CK, Baek IY. 2013. IAA-producing Penicillium sp. NICS01 triggers plant growth and suppresses Fusarium sp.-induced oxidative stress in sesame (Sesamum indicum L.). J Microbiol Biotechnol. 23:856–863. 10.4014/jmb.1209.09045
- Redman RS, Kim YO, Woodward CJDA, Greer C, Espino L, Doty SL, Rodriguez RJ. 2011. Increased fitness of rice plants to abiotic stress via habitat adapted symbiosis: a strategy for mitigating impacts of climate change. PLoS One. 6:e14823. 10.1371/journal.pone.0014823
- Ruanpanun P, Tangchitsomkid N, Hyde KD, Lumyong S. 2010. Actinomycetes and fungi isolated from plant-parasitic nematode infested soils: screening of the effective biocontrol potential, indole-3-acetic acid and siderophore production. World J Microbiol Biotechnol. 26:1569–1578. 10.1007/s11274-010-0332-8
- Saikia R, Singh K, Arora DK. 2006. Suppression of Fusarium wilt and charcoal rot of chickpea by Pseudomonas aeruginosa RsB29. Indian J Microbiol. 44:181–184.
- Sartaj AS, Sakamoto K, Inubushi K. 2011. Effect of Penicillium sp. EU0013 inoculation on tomato growth and Fusarium wilt. Hort Res. 65:69–73.
- Seastedt TR, Hobbs RJ, Suding KN. 2008. Management of novel ecosystems: are novel approaches required? Front Ecol Environ. 6:547–553. 10.1890/070046
- Shivanna MB, Meera MS, Hyakumachi M. 1994. Sterile fungi from zoysiagrass rhizosphere as plant growth promoters in spring wheat. Can J Microbiol. 40:637–644. 10.1139/m94-101
- Shoresh M, Harman GE, Mastouri F. 2010. Induced systemic resistance and plant responses to fungal biocontrol agents. Annu Rev Phytopathol. 48:21–43. 10.1146/annurev-phyto-073009-114450
- Sudadi MP. 2012. Exogenous application of tryptophan and indole acetic acid (IAA) to induce root nodule formation and increase yield of soybean. Agr Sci Res J. 2:134–139.
- Tchameni SN, Ngonkeu MEL, Begoude BAD, Nana LW, Fokom R, Owona AD, Mbarga JB, Tchana T, Tondje PR, Etoa FX, Kuate J. 2011. Effect of Trichoderma asperellum and arbuscular mycorrhizal fungi on cacao growth and resistance against black pod disease. Crop Prot. 30:1321–1327. 10.1016/j.cropro.2011.05.003
- Ting ASY, Mah SW, Tee CS. 2012. Evaluating the feasibility of induced host resistance by endophytic isolate Penicillium citrinum BTFO8 as a control mechanism for Fusarium wilt in banana plantlets. Biol Control. 61:155–159. 10.1016/j.biocontrol.2012.01.010
- Vafadar F, Amooaghaie R, Otroshy M. 2014. Effects of plant-growth-promoting rhizobacteria and arbuscular mycorrhizal fungus on plant growth, stevioside, NPK, and chlorophyll content of Stevia rebaudiana. J Plant Interact. 9:128–136.
- Waqas M, Khan AL, Lee IJ. 2014. Bioactive chemical constituents produced by endophytes and effects on rice plant growth. J Plant Interact. 9:478–487.
- Whiteside MD, Digman MA, Gratton E, Treseder KK. 2012. Organic nitrogen uptake by arbuscular mycorrhizal fungi in a boreal forest. Soil Biol Biochem. 55:7–13. 10.1016/j.soilbio.2012.06.001
- Whiteside MD, Garcia MO, Treseder KK. 2012. Amino acid uptake in arbuscular mycorrhizal plants. PLoS One. 7:e47643. 10.1371/journal.pone.0047643
- Yousif YH, Binghane FT, Yermonas DM. 1972. Growth, mineral composition and seed oil of sesame as affected by boron and exchangeable sodium. Proc Am Soil Soc. 36:923–926. 10.2136/sssaj1972.03615995003600060026x