Abstract
To reduce the risk of excessive arsenic (As) in rice by using agronomic methods applicable to common farming in uncontaminated soil with As, we examined the effect of different start periods of drainage after heading and the application of iron (Fe) or silicate (Si) materials in concrete frames filled with soil collected from an area surrounding a former As-polluted region. The concentration of As in the grain decreased with the early start of drainage, whereas the cadmium (Cd) concentration increased. Thus, maintaining the oxidative condition after heading significantly decreases the concentration of As in the grain. Fe material application significantly decreased the concentration of As in the grain compared to the control. The phosphorus (P) concentration ratio of grain to straw (which we regarded as the P distribution rate of grain and straw) was significantly lower with the delay of the start of drainage. Similar results were obtained in the solution culture experiment in which the P level was decreased by half in the culture solution. A negative correlation between the P concentration ratio of grain to straw and the concentration of As in the grain was observed, indicating that an increase in the P translocation rate from straw to grain would inhibit the accumulation of As in the grain due to the competition of P and As. Further evaluations of the effects of Fe, Si, and P on water management and fertilization are needed to reduce the risk of As accumulation in rice grain.
Introduction
Contamination of soil accompanied by crop growth inhibition in Japan has rarely been attributed to residues from pesticides that used to contain arsenic (As; Quazi et al. Citation2013) or to geological features that are found in Southeast Asian countries (Williams et al. Citation2006). Rather, such contamination and crop inhibition are believed to be caused mostly by As that has eluted due to flooding or spontaneously over a long period of time from muck, slag, and pit wastewater from mine development, and then accumulated in agricultural lands (Yamane et al. Citation1976). When the Agricultural Land Soil Contamination Prevention Law was enacted in Japan in 1971, 391 hectare (ha) in 14 regions of the country were found to require control measures for As pollution. In such As-affected regions, the crop growth inhibition is comparatively mild among dry field crops, but it is known to be severe for paddy field rice (Yamane et al. Citation1976). This is because As changes its state depending on the state of the oxidoreduction of the soil. More specifically, the reduction of soil by flooding leads to the reduction of the arsenate (a form of As) to the form arsenite, which has markedly increased solubility, plant availability, and toxicity (Takahashi et al. Citation2004).
Among the techniques for preventing the growth inhibition of rice in As-affected paddy fields, civil engineering methods (Yamane Citation1989) have been used, including (1) dilution with additional soil and (2) the removal of contaminated topsoil and replacement with uncontaminated topsoil. By 2008, of the 391 ha specified by the Agricultural Land Soil Contamination Prevention Law in Japan, 324 ha (83%) had been restored through means such as the removal and admixture of soil (Ministry of the Environment Government of JapanCitation2010).
However, the international situation regarding As has changed markedly in recent years. The issue of As in food is on the agenda for the Codex Committee. In 1988, the Joint Food and Agriculture Organization/World Health Organization Expert Committee on Food Additives (JECFA) proposed an interim acceptable As intake of 15 μg/kg (body weight)/week (JECFA Citation1988), and the discussion is continuing today from an epidemiological point of view (JECFA Citation2010). In addition, the Codex Committee adopted the maximum level 0.2 mg kg−1 for inorganic As in polished rice by the 37th Session of the Commission (Codex Alimentarius Commission Citation2014). It is thus necessary to establish agronomic management practices for reducing the accumulation of As in rice grain even in As-uncontaminated soil.
Many studies have reported methods to reduce As concentration in rice grain. These methods can be classified as those (1) maintaining soil oxidation by water control and preventing the elution of As (Arao et al. Citation2009; Li et al. Citation2009; Talukder et al. Citation2011, Citation2012; Shaheen et al. Citation2014), (2) facilitating the formation and precipitation of poorly soluble As compounds by adding iron (Fe) materials to the soil (Yamane Citation1989; Liu et al. Citation2005; Sun et al. Citation2006; Nath et al. Citation2014), and (3) inhibiting the root uptake by adding silicate (Si) substances that compete with As even when it has been eluted off (Guo et al. Citation2005; Seyfferth & Fendorf Citation2012; Hu et al. Citation2013). Most of these published papers on the As transfer from soil or culture solution to plants referred to pot experiments, heavily As-contaminated soils, and culture solution amended with high levels of As.
However, most of the food consumed originates from crops grown in fields that are not heavily contaminated with As, and it is thus necessary to study the effects of agronomic practices on As transfer from uncontaminated soil to rice under actual field conditions. There are many paddy fields in Japan with relatively high As concentrations in areas that have not been subjected to countermeasures because their As levels were below the criterion value. We therefore examined the water-saving culture and the ability of the application of Fe or Si materials to inhibit As uptake by rice in soil collected from an area surrounding a former As-polluted region, with the goal of establishing agronomic methods applicable to common paddy field farming.
Materials and methods
Concrete frame experiment
Experimental design
Bottomless concrete frames (0.8 × 0.9 × 0.6 m each) were used for each replication. The concrete frame experiment was performed in 2010 in a field at Shimane University, Matsue, Japan. Each concrete frame was filled with 400 kg of soil collected from the plow layer (0−15 cm) of paddy fields in an area surrounding a former As-polluted region. The tested soil contained 2.3% total C, 0.22% total N, 0.4 mg kg−1 total Cd, and 39.5 mg kg−1 total As, and it had a pH of 5.5. The total As concentration in the soil was higher than the background As concentration in Japanese soil (Iimura & Ito Citation1978).
To understand the accumulation of As in the rice grain and straw, we conducted the concrete frame experiment using two types of application of materials and three start periods of drainage after heading. The application of materials was comprised of three levels with nine replicates: (1) no application of materials as a control, (2) Fe material application, and (3) Si material application. The start period of drainage after heading was also comprised of three levels with nine replicates: the drainage started from (1) heading, (2) 10 days after heading, and (3) 20 days after heading. Each of the nine replicates of the application of material were divided into three levels with three replicates of the treatment of the start period of drainage. That is, this experiment was carried out by two-way layout.
Rice cultivation
Seedlings of rice (Oryza sativa L. cv. Koshihikari) were germinated on raising seedling culture soil (Green Soil, Izumo Green Co., Izumo, Japan). Two rice seedlings per hill were transplanted at the spacing of 13 cm by 16 cm (33.3 hills m−2) in each frame on 30 May 2010. A compound fertilizer containing 2.16 g of N, 0.94 g of P, and 1.79 g of K was supplied to each frame by basal application before the seedlings were transplanted. Ammonium sulfate containing 1.44 g of N was also supplied to each frame by top dressing 45 days after the rice seedlings were transplanted.
The flooding condition of the frames was maintained from the transplanting to heading period, except for a midseason drainage carried out from June 30 to July 10. The heading days occurred between August 5 and August 10. The water management after heading was described above. After the seeds had matured, the stems of the plants were cut at 2 cm above the soil surface on September 14.
Application of materials and water management after heading
Two types of commercially available materials were used in this experiment. For the Fe material, we used converter furnace slag (FM GOLD, Yoneda Industry Co., Okayama, Japan) which was obtained as Fe manufacturing waste and had been used as an agricultural soil conditioner (Fe% ≑ 20%). The application rate of the Fe material was 0.5 kg m−2 with nine replicates. For the Si material, calcium silicate slag (SiO2% ≑ 30%) was also applied at the rate of 0.5 kg m−2 with nine replicates.
These materials were applied to the soil in the concrete frames 14 days before the transplanting of rice seedlings, and then the soils were immediately plowed. The soils were sufficiently flooded and tilled for the transplanting of rice seedlings 10 days after the application of the Fe materials and calcium silicate slag. Frames without the application of Fe or Si materials were used as the control.
Three different start periods of drainage were examined after the heading period. The treatments were comprised of three levels as described above.
Solution culture experiment
A solution culture experiment was performed in a greenhouse under sunlight. Rice seeds were germinated on perlite without the addition of any nutrients. Two rice seedlings of similar growth were selected, and two hills including two seedlings were transplanted into a 30-L plastic container on June 12 and grown in a standard nutrient solution containing the macronutrients 0.18 mM (NH4)2SO4, 0.27 mM MgSO4·7H2O, 0.09 mM KNO3, 0.18 mM Ca(NO3)2·4H2O, and 0.09 mM KH2PO4, and the micronutrients 20 μM NaEDTA-Fe·3H2O, 6.7 μM MnCl2·4H2O, 9.4 μM H3BO3, 0.015 μM (NH4)6Mo7O24·4H2O, 0.15 μM ZnSO4·7H2O, and 0.16 μM CuSO4·5H2O. The 30-L plastic containers were used for each replication.This culture solution was renewed fortnightly, and the pH was adjusted daily to 5.5 until the harvest on October 3.
To evaluate the effect of different P supply rates after the heading stage on the accumulation of As in the grain and straw, this solution culture experiment was conducted with two factors: the adding period of As, and the P level in culture solution after the heading stage. The treatment of adding period of As was comprised of three levels with six replicates each: As (0.4 mg L−1, as H3AsO3) was amended during (1) the whole growth period, (2) before heading, and (3) after heading. The As amendment to the culture solution was started from July 1 in the plot of (1) the whole growth period and (2) before heading. Heading occurred on August 22, and then the As amendment stopped in the plot of (2) and started in the plot of (3).
The P level treatment in the culture solution after the heading stage was comprised of two levels with nine replications each: 0.09 mM and 0.045 mM. KCl was added instead of KH2PO4 to compensate for the reduction in potassium concentration. Each of the six replicates of the As adding period treatment were divided into two levels with three replicates of the P level treatment in the culture solution after heading stage. That is, this experiment was also carried out with a two-way layout.
After the seeds had matured, the stems of the plants were cut at the aerial part on October 3.
Chemical analysis of plant tissues
We harvested rice plant samples from each frame and solution culture, and the harvested samples were air-dried in a greenhouse for 7 days. The air-dried samples were divided into the ears and straw. Husks and unfilled grains were removed from the ears, and the filled grains and the straw were oven-dried at 75°C for 3 days. The grains and straw samples were each ground to a fine powder, and 0.5-g samples were digested in 5:1 (v/v) HNO3/H2O2 (5 mL) in a microwave oven (UNI8300, Uniflex Co., Nagano, Japan).
The concentration of As in the digested samples was determined by atomic absorption spectrophotometry (Z5010, Hitachi High-Technologies, Tokyo) with a hydride generation apparatus (HFS-3, Hitachi High-Technologies). The concentration of Cd in the digested samples was determined using the furnace mode of the atomic absorption spectrophotometer. After dilution of the digested samples with distilled water, the P in the samples was measured by the vanadate-molybdate method (yellow method).
Statistical analysis
The data were analyzed using a one-way or two-way analysis of variance (ANOVA). The mean separation between treatments was then determined using the Ryan significant difference test. Regression analyses were performed between the As concentrations in rice grain and straw and the chemical properties of the plants.
Results
Growth and concentration of As, Cd, and P of rice plants at the heading stage in the concrete frame experiment
The plant growth parameters at the heading stage are shown in . There were no significant differences in the plant length or number of tillers due to the application of materials. The soil plant analysis development (SPAD) value was significantly higher in the Fe application than in the control and Si application. shows the concentrations of As, Cd, and P in the tiller at the heading stage. Although the concentrations of Cd and P in the tiller grown in the soil to which Fe was applied tended to be lower than that grown in the control and Si application, no significant differences were observed among the treatments. The application of materials showed significant effects on the concentration of As in the tiller at the heading stage; the application of Fe material significantly reduced the As concentration in the tiller compared to the control (p< 0.05).
Table 1. Effect of the material application on the growth of rice at heading stage in the concrete frame experiment.
Table 2. Concentrations of As, Cd, and P in the tiller at heading stage in the concrete frame experiment.
Grain yield, biomass production and concentrations of As, Cd, and P in the grain and straw in the concrete frame experiment
The grain yield and biomass production of straw at harvest are shown in . No significant effect of the start period of drainage or the application of materials was observed on the grain yield or the biomass production of straw. The concentration of As in the soil tested in this experiment was considerably higher than the average concentration observed in Japan, but it was under the criterion value. The data in this experiment were equivalent to an above-average yield in Japan. Therefore, the rice cultivation in this experiment can be carried out normally without growth inhibition by As.
Table 3. The grain yield and biomass production of straw at harvest in the concrete frame experiment.
The results of the statistical analyses of the concentrations of As and Cd in the rice plants are shown in . The ANOVA revealed that the start period of drainage had significant effects on the concentration of As in the grain and that of Cd in the grain and straw. The concentration of As in the straw was not significantly affected by the start period of drainage. The significant effect of the material application was observed only for the concentration of As in the grain. There was no significant effects of the interaction between the start period of drainage and the application of materials on the concentrations of As or Cd in the rice plants.
Table 4. Analysis of variance (two-way ANOVA) on the concentration of As, Cd, and P in the grain and straw at harvest in the concrete frame experiment.
The concentration of Cd in the grain was greatly decreased with the delay of the drainage period (). The concentration of Cd in the grain was sixfold lower when the drainage was started 20 days after heading than when it was started from heading. Thus, maintaining the flooded condition after heading must be effective to reduce the Cd concentration in the grain. However, the concentration of As in the grain showed a reaction opposite to that of Cd (). The concentration of As in the grain increased with the delay in the start period of drainage. There was a significant difference between the drainage started 20 days after heading and that started at heading. Thus, maintaining the flooded condition after heading significantly increased the concentration of As in the grain.
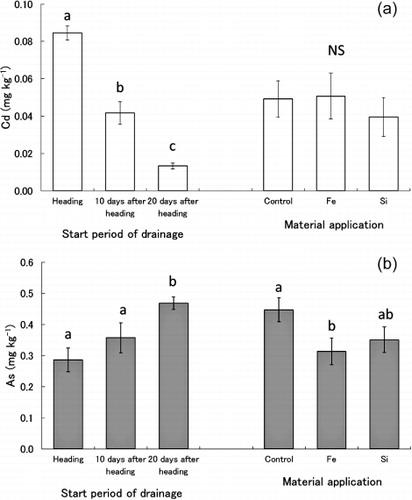
As described above, the concentration of As in the grain was significantly affected by the application of materials (). Especially, the Fe material application significantly decreased the concentration of As in the grain compared to the control, whereas the Si application did not produce a significant difference (). No significant difference was observed on the concentration of Cd in the grain among the material applications ().
shows the concentration of P in the grain and straw and the concentration ratio of grain to straw. There was no significant effect of the interaction between the start period of drainage and the application of materials on these values. The start period of drainage and the material application did not have significant effects on the concentration of P in the grain. The concentration of P in the straw tended to be higher at 20 days after heading than at heading and 10 days after heading, but no significant difference was observed among the treatments.
Table 5. The concentration of P in the grain and straw and the concentration ratio of grain to straw at harvest in the concrete frame experiment.
There was a significant effect of the start period of drainage on the P concentration ratio of grain to straw. The P concentration ratio of grain to straw was significantly lower in the drainage started from 20 days after heading than in the other plots. We regarded the P concentration ratio of grain to straw as a P distribution rate of grain and straw. A significant negative correlation (p < 0.05) was observed between the P concentration ratio of grain to straw and the concentration of As in the grain in each of the Fe () and Si () application plots, whereas no correlation was observed in the control plot ().
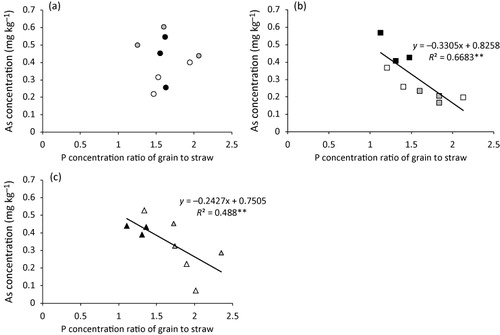
Effect of P level in culture solution on the As accumulation of rice grain
To evaluate the effect of different P supply rates after the heading stage on the accumulation of As in the grain and straw, we conducted a solution culture experiment with the two factors of the adding period of As and the P concentration in culture solution after the heading stage.
The concentrations of As in the grain and straw were significantly changed by the adding period of As in the culture solution (). The highest concentration was observed with the adding of As throughout the entire growth period, followed by the adding of As after the heading growth period. The concentration of As in the grain and straw grown in the culture solution added with As before the heading growth period was the lowest among the treatments. Reducing the P level in the culture solution after the heading growth period significantly decreased the As accumulation in the grain.
Table 6. Effects of As application period and P level in culture solution after heading on the concentration of As and P in the grain and straw at harvest in solution culture experiment.
The concentration of P in the grain did not show a significant difference despite the reduction of P in the culture solution after the heading growth period (). On the other hand, the concentration of P in the straw was significantly decreased by the reduction of P in the culture solution. These results indicate that the P concentration in the grain would be maintained at a certain concentration by the translocation of P from straw to grain. In fact, the P concentration ratio of the grain to straw was significantly higher in the culture solution with a reduced P level compared to that with the normal P level, indicating that a different rate of P translocation from straw to grain had occurred with the reduction of the P supply rate after the heading growth period.
A significant negative correlation was observed between the concentration of As in the grain and the P concentration ratio of grain to straw when the P level was decreased by half in the culture solution (), while the correlation was not observed when the P level was maintained with normal (). Thus, the increase in the rate of P translocation from straw to grain (i.e. a high rate of the P concentration ratio of grain to straw) induced by the reduction of P supply rate significantly decreased the accumulation of As in the grain. This result was similar to that of the concrete frame experiment using soil.
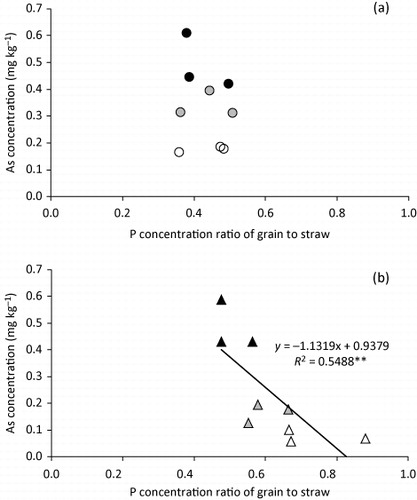
Discussion
Effect of the start period of drainage on the accumulation of As in the grain
Water-saving cultivation has been practiced to mitigate damages in paddy fields in As-affected regions (Maejima et al. Citation2008). This is because As changes its state depending on the state of the oxidoreduction of the soil. More specifically, the oxidation of soil by drainage leads to the oxidation of the arsenite to the form of arsenate, which has markedly decreased solubility, plant availability, and toxicity (Takahashi et al. Citation2004). In areas suffering from rice yield reduction caused by As contamination, the cultivation of rice under aerobic conditions has been recommended (Yamane et al. Citation1976; Sarkar et al. Citation2012). On the other hand, soil flooding has a variety of benefits for rice cultivation, such as enhancing the quality and grade of brown rice, weed control, and the prevention of damage by continuous cropping (Kyuma Citation2004). Water-saving throughout the cultivation period is thus difficult to apply in common paddy field farming.
For this reason, water control to reduce the As concentration in rice while maintaining yield and quality has not been investigated in detail and has remained largely unknown. Arao et al. (Citation2009) cultivated paddy field rice in 1/5000a Wagner pots with two types of soil with differing concentrations of 1 M HCl-soluble As; they investigated the effects of seven water control regimes on the As concentration in the grain. In both soil types, the As concentration in grain was reduced to 1/9 to 1/10 by water-saving management for 3 weeks before and after heading compared to the concentration in rice cultivated under continuous flooding conditions. However, the Cd concentrations in the grain were highest under this condition. Although Cd becomes insoluble and less prone to uptake by paddy field rice when the soil is reductive due to flooding, oxidative conditions promote the solubilization of Cd as well as its uptake by paddy field rice. Arao et al. (Citation2009) thus concluded that it was difficult to simultaneously reduce As and Cd, which are in a trade-off relationship, with water control alone.
In the present study, the results in the concrete frame experiment agreed with the study by Arao et al. (Citation2009). The results show that the As level in brown rice was reduced by 40%, but the Cd level was about sixfold higher when water was drained starting from the heading period compared to the concentrations in cultivation where flooding was maintained for 20 days after the heading period (). Thus, although early drainage presents the risk of increasing the Cd concentration in grain in regions that show relatively high Cd concentrations in the soil, it is effective to reduce the As concentration in grain.
Effect of material application on the accumulation of As in grain
We also observed that the application of materials had a significant effect on the As accumulation in the grain (). Many researchers have reported that Si application was effective to reduce the As uptake by rice grown on As-polluted soil or culture solution supplied with a high concentration of As. Li et al. (Citation2009) reported that Si fertilization decreased the total As concentration in straw and grain by 78% and 16%, respectively, and that it specifically decreased the uptake of arsenite.
One of the primary factors in the reduction of As uptake by rice is thought to be competition between As and Si because of their chemical similarities; that is, the uptake of arsenite and methylated As into shoots is mediated by Si uptake mechanisms. It was also reported that a high silicate concentration in soil solution and in nutrient solution reduced the plant As uptake (Bogdan & Schenk Citation2008; Guo et al. Citation2005, Citation2007; Seyfferth & Fendorf Citation2012). In the present study, however, Si application did not show a significant difference from the control on the concentration of As in both grain and straw ( and ), although the As concentrations in the grain and straw tended to be lower than those of the control.
We speculated that the application of silicate prevented As uptake by paddy field rice competitively because of the chemical similarities between As and silicate. Bogdan and Schenk (Citation2009) characterized the contribution of soil characteristics to the As content of rice, and they concluded that Na-acetate extracted silicate was only weakly related to the Si content in rice straw and did not contribute to the As concentration in rice. Therefore, the effect of silicate application should be considered when determining the application rate and the type of silicate materials.
We found in the present study that the application of Fe materials resulted in an extremely significant reduction of the As concentration in grain and straw ( and ). The As concentration in grain to which Fe material was applied was reduced by 42% compared to the control. These results demonstrate that the application of Fe materials strongly retarded the uptake of As by the rice plants. It was reported that the application of Fe materials decreased the absorption of As by paddy field rice (Yamane Citation1989; Nath et al. Citation2014).
The possible effects of the application of Fe materials include: (1) the increased deposition of Fe oxide in an oxidized band around rice roots and within rice roots and the inhibition of the penetration of As into the roots (Taylor et al. Citation1984; Liu et al. Citation2004); (2) co-precipitation around neutral pH when the ratio of Fe to As increases (Yamaguchi et al. Citation2011); and (3) suppression of the release of soluble arsenite by the adsorption of arsenate by Fe (Goldberg Citation2002; Takahashi et al. Citation2004; Xu et al. Citation2008; Bogdan & Schenk Citation2009). Although most of these reports were conducted using As-polluted soil or solution culture supplied with a high concentration of As, the results of the present study demonstrated the effectiveness of Fe application for reducing the As concentration in rice grain grown on nonpolluted soil. In other words, Fe application is useful to reduce the risk of As accumulation in rice grain even in common paddy field farming. The application of Fe materials is a common practice in rice production systems in Southeast Asia. Further studies are needed to determine the optimal application rate and type of Fe materials and to identify the amount of Fe oxide in soil that is closely related to the availability of As.
Effect of phosphorus translocation on the accumulation of As in grain
In the present study, the Cd concentration in the grain was greatly changed by the different start periods of drainage. The Cd concentration in the grain with drainage starting at heading was sixfold higher than that started from 20 days after heading. On the other hand, the difference in the As concentration in the grain among the start period of drainage treatments was lower than that of the Cd concentration. The availability of Cd and As was changed by the state of the oxidoreduction of the soil as described above.
These results indicate that accumulation of As in the grain may be less susceptible to the oxidoreduction of the soil than that of Cd. The marked change in the Cd concentration in grain by the water management may be explained by the reports that transport of Cd to the grain can occur from roots via the xylem as well as remobilization from leaves and culm after heading (Kashiwagi et al. Citation2009; Ishikawa et al. Citation2011). Thus, Cd accumulation in the grain would well reflect the increase in the amount of available Cd in soil that is derived from the early period drainage after heading.
It has been reported that As enters the roots either as arsenite taken up by rice roots through the highly efficient Si transport pathway (Ma et al. Citation2008) or as arsenate via arsenate/phosphate cotransporters (Abedin et al. Citation2002). Wu et al. (Citation2011) demonstrated that As transported in the xylem sap consisted of both arsenate and arsenite, with their proportions changing over the time course of arsenate exposure; arsenate was initially the dominant species, but by 24 h the majority of As was arsenite in the wild-type cv Nipponbare. Furthermore, even if some arsenate is taken up from the relatively oxygenated rhizosphere of rice roots, the pentavalent As is efficiently reduced to arsenite in roots and shoots, so that arsenite became the dominant form transported in the xylem and phloem. Arsenite was reported to be the predominant chemical species of As in rice shoot and grain (Naito et al. Citation2015). Therefore, many researchers have reported the relationship between Si and As (Guo et al. Citation2005; Seyfferth & Fendorf Citation2012; Hu et al. Citation2013) because arsenite shares the Si transport pathway (Ma et al. Citation2008). However, Si is not readily accumulated in the grain but rather deposited in the husk, which is primarily xylem fed (Lombi et al. Citation2009).
Li et al. (Citation2009) demonstrated that the application of Si fertilization decreased the total As concentration in straw and grain by 78% and 16%, respectively, and specifically decreased the arsenite uptake. These results indicate that the suppression of As accumulation in the grain by Si fertilization would be much less than that in shoots. Norton et al. (Citation2010) reported that no correlation was observed between shoot Si and grain As, and suggest that although the uptake of arsenite and methylated As into shoots is mediated by the Si uptake mechanisms, the translocation of As from shoots to grain – especially the unloading of As into the grain – may involve the system of P transport. If indeed arsenate may be the main form of As transported to the grain because arsenate would be readily translocated from shoot to grain during P mobilization (Zhang et al. Citation2007; Norton et al. Citation2008; Jiang et al. Citation2014).
Lou-Hing et al. (Citation2011) demonstrated that As uptake and translocation from root to shoot in rice grown on culture solution applied with arsenite or arsenate were decreased by high phosphate treatments (≥50 µM). Both tissue phosphorus and As concentrations did not respond differently to arsenate or arsenite treatments. They supposed that both As species were involved in a shared pathway responsible for reduced As sensitivity. Arsenite is more reactive than arsenate and these ions exist in equilibrium with each other, driven by redox status. Alternatively, arsenite reduced to arsenate at the cellular level would allow phosphate to out-compete arsenate in metabolism due to the higher tissue phosphate status. Norton et al. (Citation2010) also considered that free arsenate and arsenite were in equilibrium that was driven toward arsenite by the reducing conditions of plant cells, maintained by arsenate reductases or other nonenzymatic pathways.
Recently, the relationship between As and phosphate was demonstrated at the genetic level. Norton et al. (Citation2008) showed that genes involved in rice phosphate transport were regulated by arsenate exposure. Zhang et al. (Citation2007) identified a region on chromosome 8 of rice that is responsible for increasing shoot P concentration and decreasing grain As concentration by the mapping of quantitative trait loci.
These reports suggest that the reduction of As accumulation in grain is not as large as the decrease in available As in the soil by the early period of drainage. Thus, the relationship between P and As on the translocation from shoots to grain must be also considered for the accumulation of As in the grain.
Generally, it is well known that P availability in soil is decreased by oxidation with drainage. Fe and Si applications also decrease P availability in soil due to the fixation of As with Fe oxide and competition with As and Si (Bogdan & Schenk Citation2009). Therefore, the early start of drainage after heading and the Fe or Si application in the present study would inhibit P supply to the rice plant. However, there was little difference in the P concentrations in the grain among the present treatments, whereas the P concentration in straw tended to be lower in the early period of drainage ().
The concentration of P in grain stays at a certain level because grain is a strong sink of P, and the P translocation from shoots to grain would accelerate in rice plants when the P supply from soil is restricted. We regarded the P concentration ratio of grain to straw as the P distribution rate of grain and straw. The P concentration ratio of grain to straw was significantly lower in the drainage started from 20 days after heading than in the other plots (). A similar result was also obtained in the solution culture experiment in which the P level was decreased by half in the culture solution ().
These results indicate that the P translocation from shoot to grain is stimulated when the P supply from soil or culture solution is restricted. In that situation, we observed a negative correlation between the P concentration ratio of grain to straw and the concentration of As in the grain in both the concrete frame experiment ( and ) and the solution culture experiment (). These results suggest that the increasing of the P translocation rate from straw to grain would inhibit the accumulation of As in the grain due to the competition of P and As.
Phosphate transporters have been identified as mechanisms of arsenate assimilation in rice, as the two ions are analogs (Abedin et al. Citation2002; Meharg & Jardine Citation2003). Norton et al. (Citation2010) reported that As utilizes the P transport mechanisms in rice for transport into the grain, and P then outcompetes As in the translocation from shoots to grain. Our results should be in agreement with those studies, and thus further studies of the P interaction with As accumulation in the grain are needed. After their survey of plant samples from 68 fields, Bogdan and Schenk (Citation2009) suggested that fertilizer management such as low P fertilization plus Fe and Si application may decrease the As concentration in grain.
To reduce the risk of excessive As accumulation in rice grain by using agronomic methods applicable to common farming, detailed evaluations of the effects of Fe, Si, and P on water management and fertilization are needed.
Additional information
Funding
References
- Abedin MJ, Cotter-Howells J, Meharg A. 2002. Arsenic uptake and accumulation in rice (Oryza sativa L.) irrigated with contaminated water. Plant Soil. 240:311–319.10.1023/A:1015792723288
- Arao T, Kawasaki A, Baba K, Mori S, Matsumoto S. 2009. Effects of water management on cadmium and arsenic accumulation and dimethylarsinic acid concentrations in Japanese rice. Environ Sci Technol. 43:9361–9367.10.1021/es9022738
- Bogdan K, Schenk MK. 2008. Arsenic in rice (Oryza sativa L.) related to dynamics of arsenic and silicic acid in paddy soils. Environ Sci Technol. 42:7885–7890.10.1021/es801194q
- Bogdan K, Schenk MK. 2009. Evaluation of soil characteristics potentially affecting arsenic concentration in paddy rice (Oryza sativa L.). Environ Pollut. 157:2617–2621.10.1016/j.envpol.2009.05.008
- Codex Alimentarius Commission. 2014. Thirty-seventh Session CICG, Geneva; [cited 2014 Jul 14–18]. Available from: http://www.fao.org/news/story/en/item/238558/icode/.
- Goldberg S. 2002. Competitive adsorption of arsenate and arsenite on oxides and clay minerals. Soil Sci Soc Am J. 66:413–421.10.2136/sssaj2002.4130
- Guo W, Hou YL, Wang SG, Zhu YG. 2005. Effect of silicate on the growth and arsenate uptake by rice (Oryza sativa L.) seedlings in solution culture. Plant Soil. 272:173–181.10.1007/s11104-004-4732-0
- Guo W, Zhu YG, Liu WJ, Liang YC, Geng CN, Wang SG. 2007. Is the effect of silicon on rice uptake of arsenate (AsV) related to internal silicon concentrations, iron plaque and phosphate nutrition? Environ Pollut. 148:251–257.
- Hu H, Zhang J, Wang H, Li R, Pan F, Wu J, Feng Y, Ying Y, Liu Q. 2013. Effect of silicate supplementation on the alleviation of arsenite toxicity in 93-11 (Oryza sativa L.indica). Environ Sci Pollut Res. 20:8579–8589.10.1007/s11356-013-1811-x
- Iimura K, Ito H. 1978. Behavior and balance of contaminant heavy metals in paddy soils: studies on heavy metal pollution of soils (Part 2). Bull Hokuriku Natl Agric Exp Stn. 21:95–145. Japanese.
- Ishikawa S, Suzui N, Tanabata SI, Ishii S, Igura M, Abe T, Kuramata M, Kawachi N, Fujimaki S. 2011. Real-time imaging and analysis of differences in cadmium dynamics in rice cultivars (Oryza sativa) using positron-emitting107Cd tracer. BMC Plant Biol. 11:172. 10.1186/1471-2229-11-172
- [JECFA] Joint FAO/WHO Expert Committee on Food Additives. 1988. Summary of evaluations performed by the Joint FAO/WHO Expert Committee on Food Additives: Arsenic. [cited 2013 Sep 17]. Available from: http://www.inchem.org/documents/jecfa/jeceval/jec_159.htm.
- [JECFA] Joint FAO/WHO Expert Committee on Food Additives. 2010. Summary report of the 73rd JECFA meeting. [cited 2013 Sep 17]. Available from: http://www.fao.org/ag/agn/agns/jecfa/JECFA73%20Summary%20Report%20Final.pdf.
- Jiang W, Hou Q, Yang Z, Zhong C, Zheng G, Yang Z, Li J. 2014. Evaluation of potential effects of soil available phosphorus on soil arsenic availability and paddy rice inorganic arsenic content. Environ Pollut. 188:159–165.10.1016/j.envpol.2014.02.014
- Kashiwagi T, Shindoh K, Hirotsu N, Ishimaru K. 2009. Evidence for separate translocation pathways in determining cadmium accumulation in grain and aerial plant parts in rice. BMC Plant Biol. 9:8.10.1186/1471-2229-9-8
- Kyuma K. 2004. The paddy soil/rice system in the environment. In: Kyuma K, editor. Paddy soil sciences. Kyoto, Melbourne: Kyoto University Press, Transpacific Press; p. 255–277.
- Li RY, Stroud JL, Ma JF, McGrath SP, Zhao FJ. 2009. Mitigation of arsenic accumulation in rice with water management and silicon fertilization. Environ Sci Technol. 43:3778–3783.10.1021/es803643v
- Liu WJ, Zhu YG, Smith FA. 2005. Effects of iron and manganese plaques on arsenic uptake by rice seedlings (Oryza sativa L.) grown in solution culture supplied with arsenate and arsenite. Plant Soil. 277:127–138.
- Liu WJ, Zhu YG, Smith FA, Smith SE. 2004. Do phosphorus nutrition and iron plaque alter arsenate (As) uptake by rice seedlings in hydroponic culture? New Phytol. 162:481–488.
- Lombi E, Scheckel KG, Pallon J, Carey AM, Zhu YG, Meharg AA. 2009. Speciation and distribution of arsenic and localization of nutrients in rice grains. New Phytol. 184:193–201.10.1111/j.1469-8137.2009.02912.x
- Lou-Hing D, Zhang B, Price AH, Meharg AA. 2011. Effects of phosphate on arsenate and arsenite sensitivity in two rice (Oryza sativa L.) cultivars of different sensitivity. Environ Exp Bot. 72:47–52.
- Ma JF, Yamaji N, Mitani N, Xu XY, Su YH, McGrath SP, Zhao FJ. 2008. Transporters of arsenite in rice and their role in arsenic accumulation in rice grain. PNAS. 105:9931–9935.10.1073/pnas.0802361105
- Maejima Y, Arao T, Baba K. 2008. Arsenic contamination in soils and crops in Japan. In: Hseu ZY, Chen TC, Chao HR editors. 2008 International symposium of soil heavy metal pollution and remediation. Pingtung: National Pintung University of Science and Technology Press; p. 76–86.
- Meharg AA, Jardine L. 2003. Arsenite transport into paddy rice (Oryza sativa) roots. New Phytol. 157:39–44.10.1046/j.1469-8137.2003.00655.x
- Ministry of the Environment Government of Japan. 2010. Enforcement status of Agricultural Land-Soil Pollution Prevention Law in fiscal 2008. Japan: Ministry of the Environment. [cited 2013 Sep 17]. Available from: http://www.env.go.jp/press/file_view.php?serial=16311&hou_id=12979. Japanese.
- Naito S, Matsumoto E, Shindoh K, Nishimura T. 2015. Effects of polishing, cooking, and storing on total arsenic and arsenic species concentrations in rice cultivated in Japan. Food Chem. 168:294–301.10.1016/j.foodchem.2014.07.060
- Nath S, Panda P, Mishra S, Dey M, Choudhury S, Sahoo L, Panda SK. 2014. Arsenic stress in rice: redox consequences and regulation by iron. Plant Physiol Biochem. 80:203–210.10.1016/j.plaphy.2014.04.013
- Norton GJ, Islam MR, Duan GL, Lei M, Zhu YG, Deacon CM, Moran AC, Islam S, Zhao FJ, Stroud JL, et al. 2010. Arsenic shoot-grain relation-ships in field grown rice cultivars. Environ Sci Technol. 44:1471–1477.
- Norton GJ, Lou-Hing DE, Meharg AA, Price AH. 2008. Rice-arsenate interactions in hydroponics. Whole genome tran-scriptional analysis. J Exp Bot. 59:2267–2276.
- Quazi S, Sarkar D, Datta R. 2013. Human health risk from arsenical pesticide contaminated soils: a long-term greenhouse study. J Hazard Mater. 262:1031–1038.
- Sarkar S, Basu B, Kundu CK, Patra PK. 2012. Deficit irrigation: an option to mitigate arsenic load of rice grain in West Bengal, India. Agri Ecosyst Environ. 146:147–152.
- Seyfferth AL, Fendorf S. 2012. Silicate mineral impacts on the uptake and storage of arsenic and plant nutrients in rice (Oryza sativa L.). Environ Sci Technol. 46:13176–13183.
- Shaheen SM, Rinklebe J, Rupp H, Meissner M. 2014. Lysimeter trials to assess the impact of different flood–dry-cycles on the dynamics of pore water concentrations of As, Cr, Mo and V in a contaminated floodplain soil. Geoderma. 228–229:5–13.
- Sun H, Wang L, Zhang R, Sui J, Xu G. 2006. Treatment of groundwater polluted by arsenic compounds by zero valent iron. J Hazard Mater. 129:297–303.10.1016/j.jhazmat.2005.08.026
- Takahashi Y, Minamikawa R, Hattori KH, Kurishima K, Kihou N, Yuita K. 2004. Arsenic behavior in paddy fields during the cycle of flooded and non-flooded periods. Environ Sci Technol. 38:1038–1044.10.1021/es034383n
- Talukder ASM, Meisner CA, Sarkar MAR, Islam MS. 2011. Effect of water management, tillage options and phosphorus status on arsenic uptake in rice. Ecotoxicol Environ Saf. 74:834–839.10.1016/j.ecoenv.2010.11.004
- Talukder ASM, Meisner CA, Sarkar MAR, Islam MS, Sayre KD, Duxbury JM, Lauren JG. 2012. Effect of water management, arsenic and phosphorus levels on rice in a high-arsenic soil–water system: II. Arsenic uptake. Ecotoxicol Environ Saf. 80:145–151.10.1016/j.ecoenv.2012.02.020
- Taylor GJ, Crowder AA, Rodden R. 1984. Formation and morphology of an iron plaque on the roots of Typha latifolia L. grown in solution culture. Am J Bot. 71:666–675.10.2307/2443363
- Williams PN, Islam MR, Adomako EE, Raab A, Hossain SA, Zhu YG, Feldmann J, Meharg AA. 2006. Increase in rice grain arsenic for regions of Bangladesh irrigating paddies with elevated arsenic in groundwaters. Environ Sci Technol. 40:4903–4908.10.1021/es060222i
- Wu Z, Ren H, McGrath SP, Wu P, Zhao FJ. 2011. Investigating the contribution of the phosphate transport pathway to arsenic accumulation in rice. Plant Physiol. 157:498–508.10.1104/pp.111.178921
- Xu XY, McGrath SP, Meharg AA, Zhao FJ. 2008. Growing rice aerobically markedly decreases arsenic accumulation. Environ Sci Technol. 42:5574–5579.10.1021/es800324u
- Yamaguchi N, Nakamura T, Dong D, Takahashi Y, Amachi S, Makino T. 2011. Arsenic release from flooded paddy soils is influenced by speciation, Eh, pH, and iron dissolution. Chemosphere. 83: 925−932.10.1016/j.chemosphere.2011.02.044
- Yamane T. 1989. The mechanisms and countermeasures of arsenic toxicity to rice plant. Bull Shimane Agr Exp. 24:1–95. In Japanese with English abstract.
- Yamane T, Yamaji T, Takami Y. 1976. Mechanism of rice plant injury in arsenic contaminated paddy soils and its preventive measures: I. Influence of arsenite and arsenate in growth media on the nutrient uptake, growth and yield of rice plant. Bull Shimane Agr Exp. 14:1–17. In Japanese with English abstract.
- Zhang J, Zhu YG, Zeng DL, Cheng WD, Qian Q, Duan GL. 2007. Mapping quantitative trait loci associated with arsenic accumulation in rice (Oryza sativa). New Phytol. 177:350–355.