Abstract
Peony seed oil has been known to have a high value for health due to the high content of unsaturated fatty acid. However, few studies have investigated which factors impact the oil quality and composition of seeds in the traditional mining cultivation area. In the present study, the investigation was conducted in 30 cultural field sites. Significantly negative relationships were found between Cu content in soils or leaves and linoleic and unsaturated fatty acids. Further tests revealed that Cu evidently accumulated in leaves. This demonstrated Cu content in soils was the important factor that influenced linoleic acid content and unsaturated fatty acid content. Such proposition must be considered when cultivating groups of plant toward expanding oil production.
Introduction
Phoenix White (Paeonia ostii) is a hardy shrub in the peony family, Paeoniaceae. And now it is widely cultivated in the region of Fenghuang mountain and Ya mountain in Anhui Province, China. Conventional cultivating methods had mainly focused on improving the bark of their roots yield and paeonol content which is used as an anti-spasmodic throughout Asia (Sevim et al. Citation2013). Recently, it have been proved to be a reliable crop for seed oil production because its seeds are rich in high unsaturated fatty acid content, especially γ-linolenic acid content for edible and health oil (Kim et al. Citation2014). Five main fatty acid (the minimum peak area of more than 0.1) were found in seed oil with the average content of the fatty acids being: Palmitic acid (C16:0) 5.6; Stearic acid (C18:0) 1.9; Oleic acid (C18:1) 24.6; Linoleic acid (C18:2) 29.8; and α-linolenic acid (C18:3) 38.1. In 2011, peony seed oil was approved as a new resource of food by the Ministry of health of China. An increasing market of peony seed oil has given farmer an opportunity to expand the planting area. But Fenghuang mountain-Ya mountain region also is a famous Cu mining accompanied by associated elements, such as Zn, Fe and S (Xu et al. Citation2013). Earlier studies have suggested that P. ostii could be grown as high-value crops in heavy metal polluted agricultural soils without pollution on the root bark (Zhou et al. Citation2011). Furthermore, medicinal crops may offer aphytoremediation option for mildly metal polluted agricultural soils (Zheljazkov et al. Citation2008; Dutta & Maharia Citation2012). However, the effect of these metal contaminants on seed oil of P. ostii is not well known.
In the present study, we conducted a survey of seed oil and soil chemical factors, especially the soil metal contents of Paeonia ostia in the traditional cultural mining area. Our objectives were to discover relationships between fatty acid composition of the seed oils and soil factors to provide information on the influence of soil metals on fatty acid composition of P. ostii.
Materials and methods
Study sites and sampling
This study was conducted in July 2013 at Fenghuang Mountain-Ya Mountain Region (30°49′–30°52′N, 117°59′–118°02′E). The region is located at the junction of Tongling City and Nanling County in the southern part of Anhui Province, China. The field has an elevation of 200–m and features yellow red soil. The average annual temperature in the region is 16.2°C, with an average annual precipitation of 1370 mm, average annual evaporation capacity of 1517 mm, annual average relative humidity of 77, and annual frost free period of 230 d. The region receives sufficient light, with illumination time of approximately 2000–2050h.
In this study, 30 cultural fields were investigated with a uniform distribution of maximum effort ((a)). Ten points were collected and mixed as one sample for each field ((b)). The samples were collected from the mature fruit, leaves, and soils of the crop. However, only 25 fruit samples (farmers harvested in advance; hence, 5 samples of typical fruit were not collected). All soil samples, excluding small stones and plant roots, were air-dried. The samples were then passed through a 0.25 mm nylon sieve and were used for laboratory analysis. Plant samples were dried at 80°C to a constant weight.
Figure 1. Visualization of sampling sites in which survey work was conducted during 2013. Field sites were located in Fenghuang mountain-Ya mountain regions, which belongs to the border of Tongling city and Nanling County. (a) Distribution of 30 cultural fields. (b) Map of one cultural field. (c) Plantlet of P. ostii. (d) Collected fruit of P. ostii before natural cracking. (e) Seed and natural cracking of fruit.373 x 148 mm (150 x 150 DPI)
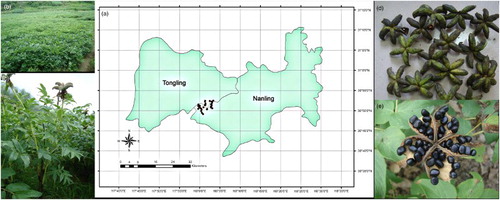
Determination of soil physicochemical Properties
Soil pH was measured in a solution of 1.0 g of soil and 5 mL of deionized water by using a Sartorius PB10 pH meter (Sartorius, Germany). Soil electrical conductivity (EC) was determined with the use of an EC meter (DDS-307, Shanghai Precision Scientific Instruments Co., Ltd., China). Meanwhile, the total concentrations of Cu, Zn, Fe, Mg, and K in soils were measured by using the HCl-HNO3-HF-HClO4 digestion method, followed by the use of an atomic absorption spectrometer (NOVAA350, Germany). Soil samples (0.3 g) were introduced into a Teflon crucible (50 mL) containing 8 mL of aqua regia. The solution was heated on a hot plate at 190°C for approximately 10 min. The temperature was then increased to 240°C until the solution was nearly dry. Subsequently, 3 mL of hydrogen fluoride (HF) was added into the crucible and was heated at 240°C until the solution turned into viscous liquid. Approximately 3 mL of HClO4 was also added into the crucible to maintain the viscous state of the solution. The metal concentrations in the solution were quantified by dissolving the residues in 25 mL of 1 HNO3. The obtained value represented the total metal concentrations.
Meanwhile, the total concentrations of Cu, Zn, Fe, Mg, and K in leaves were measured by employing the HCl-HNO3-HClO4 digestion method. The digestive process of plant samples (0.5 g) was similar to that of the soils, only the HF digestion process was excluded.
GC-MS analysis of peony seed oil
In accordance with the slightly modified method proposed by Phippen et al. (Citation2004), 4.0 g peony seed oil was placed in a round flask with 40 mL methanol and 1 M KOH methanol solution (0.5 mL). The solution was refluxed for 10 min and then cooled. It was then taken from the flask and transferred into a separatory funnel, to which hexane (20 mL) and H2O (40 mL) were added. The solution was vortexed and the layers were allowed to separate. The hexane layer was removed with a glass pipette and transferred into a gas chromatographic vial until application.
GC-MS analysis was performed on an Agilent 6890N Network GC system combined with an Agilent 5973 Network Mass Selective Detector. The GC-MS operating parameters were: chromatographic column: HP – 5MS 30 m × 0.25 mm × 0.25 μm fused silica capillary column; carrier gas: He; column temperature: 70°C held for 1 min, heated up to 100°C at 5°C/min, held for 2 min, heated up to 175°C at 10°C/min, held for 40 min, then heated up to 225°C at 15°C/min, held for 40 min, spitless injection volume: 1.0 µL; injection temperature: 250°C. The specific components of peony seed oil are shown in .
Data analysis
All samples were performed in triplicate and data were processed using SPSS 13.0 software (SPSS, USA). Mean, standard deviation, and coefficient of variation were calculated. The Pearson correlation was used to analysis correlation between composition of fatty acid and soil factors. Regression analysis was used to find linear relations between fatty acid content and variable soil physicochemical properties. The test was considered significant when the p value was lower than .05.
Results and discussion
Variations of peony seed
demonstrates the variations of seed weight, oil content, and of the five major fatty acids in the seed. The greatest variation was identified in the ratio of seed and capsule weights, in which the coefficient of variation was 16.61 (range of ratio from 0.68 to 1.31; mean = 0.99). The coefficient of variation in the five major fatty acids, saturated, and unsaturated fatty acids in the seed ranged from 5.66 to 9.72. Which is similar variation and reported for various species from environmental effect (Younis et al. Citation2000; Boschin et al. Citation2008; Yang et al. Citation2011; Chouaibi et al. Citation2012; Mao et al. Citation2012; Sabir et al. Citation2012; Solis et al. Citation2013; Alves et al., Citation2014; Nederal et al. Citation2014). The variation of C18:2 contents were high in five kinds of fatty acid, varied from 41.68–57.92 g/kg (25.97–36.82) with an average of 49.76 g/kg. The smallest variation was observed in C18:1 (CV 5.66).
Table 1. The variation of seed traits in various field condition.
Variations in basic soil chemical characteristics
showed the variations of Mg, Cu, Fe, K, and Zn concentrations, as well as organic carbon contents, pH, and electrical conductivity, obtained from 30 cultivated fields. The pH value of the soils ranged from acidic to mildly alkaline, with most soils having a pH value of 6.5–7.5. For the garden soils sampled in Tongling mining area, the pH values determined by this study were similar to those reported by Xu et al. (Citation2013). A wide range of Cu, Zn, and Fe concentrations was observed across the study area. The findings indicated that the average Zn contents were 2.7 times compared with that of Chinese Standard for Agricultural Soils (250 mg/kg, Chinese Environmental Quality Standard for soil GB 15618–1995). The average concentrations of Cu (84.68 mg/kg) were significantly higher than an average global value (30 mg/kg) in uncontaminated (Adriano Citation2001) and were slightly lower than the Chinese Standard for Agricultural Soils (100 mg/kg), but Cu content in soils had the highest coefficient of variation 83.06, which is markedly higher variation than other metals in this study area. The soil copper content of 28 samples exceeded the Chinese Standard for Agricultural Soils. Fe is one of the major constituents of the lithosphere and pedosphere. The content of Fe in natural soils ranges between 7 and 42 mg/g (Wei et al. Citation2010). However, plant available Fe is low in most soils because the low solubility product of iron minerals makes the inorganic form of iron unavailable to plants and forms the most common widespread nutritional disorder world over (Chatterjee et al. Citation2006). The average Fe content were 40.42 mg/g with range 26.67–58.89 mg/g in this study, it was slight higher than the natural background values.
Table 2. The variation of metal element, pH, and electrical conductivity in soils in various cultural regions.
Effect of variable soil metal on composition of seed oil
Regression analysis was conducted to estimate the relationships of seed oil composition with essential soil metals. The result of the analysis showed that in soils, C18:2 and USFA had a significantly negative linear correlation with Cu content (). Meanwhile, other factors exhibited a weak correlation with the composition of seed oil (data are shown in the ). The value of R2 for the linear correlation between Cu content in soils and C18:2 (R2 = 0.350) was larger than that for the correlation of Cu content in soils with USFA (R2 = 0.229). This finding may demonstrate Cu content in soils was the important factor that influenced linoleic acid content and unsaturated fatty acid content. C18:2 is much more sensitive parameter affected by Cu content of soil compared with other four fatty acids, and unsaturated fatty acid was significantly affected than saturated fatty acid by the Cu content in soil.
Accumulation of Fe, Zn, and Cu in leaves and its effect on the composition of seed oil
In particular, the linear Pearson correlation between the metal contents in soil and leaves was explored (). In plants, normal Fe concentrations vary between 30 and 300 mg/kg dry weight (Adamski et al. Citation2011), and concentrations above 500 mg/kg are generally considered toxic (Pugh et al. Citation2002). In this study, the Fe concentration in P. ostii leaves ranged from 70 to 150 mg/kg, which falls within the moderate value of plant growth. No linear correlation was determined between Fe content in leaves and soil. Excess Fe concentration in P. ostii is yet to be reported.
Table 3. The Pearson correlations between Fe, Zn, and Cu content in leaves and in soils.
Zn is the second most abundant transition metal after iron (Fe) and is involved in various biological processes in organisms (Broadley et al. Citation2007). Zinc is normally present at concentration of 10–200 mg/kg for leaves of plants (Ghaderian et al. Citation2007). Balsberg Påhlsson (Citation1989) suggested that Zn concentrations of leaves above 200 mg/kg may impair plant performance, Zn accumulates in the plant tissues is toxic concentrations (300 mg/kg) for most plants, lowering the content in essential nutrients (Sagardoy et al. Citation2009). The Zn content of P. ostii leaves in this study ranged from 42.91 to 104.50 mg/kg. This was an appropriate value to most plants. The linear correlation did not found between Zn content in leaves and in soil. Although P. ostii was still needed to study for the zinc tolerance, but this study was difficult to determine whether there was excess Zn in leaves.
Cu concentrations in plants are regulated within a remarkably narrow range, normally from 5 to 25 mg/kg, and concentrations >100 mg/kg are rare even in the presence of high soil Cu concentrations (Reeves & Baker Citation2000). In this study, the Cu content of P. ostii in its leaf dry matter ranged from 3.78 to 6.49 mg/kg. Shen et al. (Citation2005) reported that Cu content in the leaves of P. ostii is approximately 30 mg/kg from Cu-tailings of 1212 mg/kg soil Cu, and such content increases with cultivation time. A control experiment revealed that Cu could accumulate in the roots, stems, shoots, and leaves of P. ostii. In particular, Cu content in leaves could reach 80 mg/kg (Zhou et al. Citation2011). These findings indicated that Cu concentration in the leaves of P. ostii depends on soil Cu concentration and accumulation times. Such proposition conforms to the results of this study.
Significant correlations were detected among C18:2 (p < .01), USFA (p < .01), and Cu content in leaves (). However, no correlations were established among the Fe content of leaves, Zn content of leaves, and all compositions of fatty acid (data are shown in Appendix). With a correlation coefficient of 0.348 (R2 = 0.348), linoleic acid content showed a significant negative correlation with Cu content in leaves. Such measure was slightly lower than the correlation coefficient of the linoleic acid and Cu contents in soil (R2 = 0.350). Meanwhile, the correlation coefficient between the unsaturated fatty acids and Cu content in leaves (R2 = 0.264) was higher than that between the unsaturated fatty acids and Cu content in soil (R2 = 0.229). This particular observation clarifies that the Cu content of leaves induced a significant inhibition of unsaturated fatty acid storage.
Figure 4. The influence of Cu content in leaves on composition of seed oils. 170 x 80mm (150 x 150 DPI)
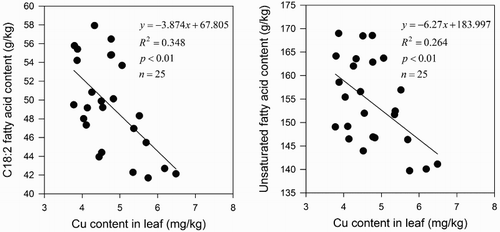
In their study, Sinha et al. (Citation2010) considered that the plant parts (i.e. fruit and seed), which can be considered as storage organs and have low transpiration rates, do not accumulate heavy metals. Similarly, in the present study, Cu did not accumulate in the seeds of P. ostii (data are not shown). One probable reason for such condition is the fact that the embryo occupies a remarkably small proportion of seed dry weight. This particular matter must be further explored. The seeds of P. ostii contain almost exclusively 16-carbon and 18-carbon fatty acid, which is similar to the fatty acid composition of the plant cell membrane (Millar et al. Citation2000). Seed oil and membrane lipids simultaneously occur and involve common precursors (Ohlrogge & Browse Citation1995). Plastid is a major organelle involved in the synthesis of fatty acids (Hills Citation2004) and is also the main site for Cu accumulation in higher plants (Sinha et al. Citation2010). Previous studies noted that photosynthesis decreases in Cu-treated plants (Fernandes & Henriques Citation1991) and That Cu accumulation may affect fatty acid content. This study, however, did not observe a significant correlation between Cu accumulation and gross fatty acid content (data are shown in the Appendix). By using various species, recent studies investigated the changes in fatty acid composition induced by Cu (Baryla et al. Citation2000; Chaffai et al. Citation2007). In the study of Chaffai et al. (Citation2007), the unsaturation level of total fatty acids decreased from 1.42 to 1.28 in maize roots under Cu stress. Baryla et al. (Citation2000) reported that both peroxides of linoleic acid and linolenic acid had a significant positive correlation with the Cu concentration in the plantlets. We also found that linoleic acid of seeds oil from P. ostii was more sensitive to excess Cu.
Consequently, P. ostii seed oil composition can definitely be affected by Cu content in soil via the accumulation of plantlet. Hence, Cu content must be considered in cultivating P. ostii for producing seed oil, although metal availability to plants may also be strongly influenced by pH and other factors. Over the past two years, P. ostii has been cultivated from 30° north latitude to 38° north latitude. The effects of environmental variables, such as light, temperature, and rainfall, must not be discounted in selecting an appropriate habitat for cultivating P. ostii to improve the quality of peony seed oil further. These still need further research.
Disclosure statement
No potential conflict of interest was reported by the authors.
Additional information
Funding
References
- Adamski JM, Peters JA, Danieloski R, Bacarin, MA. 2011. Excess iron-induced changes in the photosynthetic characteristics of sweet potato. J Plant Physiol. 168:2056–2062.
- Adriano DC. 2001. Trace elements in terrestrial environment: biogeocemistry, Bioavailability and Risks of Metals, 2nd ed. New York: Springer-Verlag.
- Alves AAC, Manthey C, Manthey L, Isbell T, Ellis D. 2014. Diversity in oil content and fatty acid profile in seeds of wild cassava germplasm. Ind Crop Prod. 60:310–315.
- Balsberg Påhlsson AM. 1989. Toxicity of heavy metals (Zn, Cu, Cd, Pb) to vascular plants. A literature review. Water Air Soil Pollut. 47:287–319.
- Baryla A, Laborde C, Montillet JL, Triantaphylidès C, Chagvardieff P. 2000. Evaluation of lipid peroxidation as a toxicity bioassay for plants exposed to copper. Environ Pollut. 109:131–135.
- Boschin GD, Agostina A, Annicchiarico P, Arnoldi A. 2008. Effect of genotype and environment on fatty acid composition of Lupinus albus L. seed. Food Chem. 108:600–606.
- Broadley M.R. White PJ, Hammond JP, Zelko I, Lux A. 2007. Zinc in plants. New Phytol. 173:677–702.
- Chaffai R, Elhammadi MA, Seybou TN, Tekitek A, Marzouk B, Ferjani EE. 2007. Altered fatty acid profile of polar lipids in maize seedlings in response to excess copper. J Agron Crop Sci. 193:207–217.
- Chatterjee C, Gopal R, Dube BK. 2006. Impact of iron stress on biomass, yield, metabolism and quality of potato (Solannum tuberosum L.). Sci Hortic (Amsterdam). 108:1–6.
- Chouaibi M, Mahfoudhi N, Rezig L, Donsi F, Ferrari G, Hamdi S. 2012. Nutritional composition of Zizyphus lotus L. seeds. J Sci Food Agric. 92:1171–1177.
- Dutta RK, Maharia RS. 2012. Antioxidant responses of some common medicinal plants grown in copper mining areas. Food Chem. 131:259–265.
- Fernandes JC, Henriques FS. 1991. Biochemical, physiological and structural effect of excess copper in plants. Bot Rev. 57:246–273.
- Ghaderian SM, Hemmat GR, Reeves RD, Baker AJM. 2007. Accumulation of lead and zink by plants colonizing a metal mining area in central Iran. J Appl Bot Food Qual. 81:145–150.
- Hills MJ. 2004. Control of storage-product synthesis in seeds. Curr Opin Plant Biol. 7:302–308.
- Kim KB, Nam YA, Kim HS, Hayes AW, Lee BM. 2014. α-Linolenic acid: nutraceutical, pharmacological and toxicological evaluation. Food Chem Toxicol. 70:163–178.
- Mao ZX, Fu H, Nan ZB, Wang J, Wan CG. 2012. Fatty acid content of common vetch (Vicia sativa L.) in different regions of Northwest China. Biochem Syst Ecol. 44:347–351.
- Millar AA, Smith MA, Kunst L. 2000. All fatty acids are not equal: discrimination in plant membrane lipids. Trends plant sci. 5(3):95–101.
- Nederal S, Petrović M, Vincek D, Pukec D, Škevin D, Kraljić K, Obranović M. 2014. Variance of quality parameters and fatty acid composition in pumpkin seed oil during three crop seasons. Ind Crop Prod. 60:15–21.
- Ohlrogge J, Browse J. 1995. Lipid biosynthesis. Plant Cell. 7:957–970.
- Phippen WB, Isbell TA, Phippen ME. 2004. Total seed oil and fatty acid methyl ester contents of Cuphea accessions. Ind Crop Prod. 24:52–59.
- Pugh RE, Dick DG, Fredeen AL. 2002. Heavy metal (Pb, Zn, Cd, Fe and Cu) contents of plant foliage near the Anvil range lead/zinc mine, Faro, Yukon territory. Ecotoxicol Environ Saf. 52:273–279.
- Reeves RD, Baker AJM. 2000. Metal accumulating plants. In: Raskin, I., Ensley, B.D. editor. Phytoremediation of toxic metals: using plants to clean up the environment. New York: John Wiley and Sons; pp. 193–229.
- Sabir A, Unver A, Kara Z. 2012. The fatty acid and tocopherol constituents of the seed oil extracted from 21 grape varieties (Vitis spp.). J Sci Food Agric. 92:1982–1987.
- Sagardoy R, Morales F, López-Millán AF, Abadía A, Abadía J. 2009. Effects of zinc toxicity on sugar beet (Beta vulgaris L.) plants grown in hydroponics. Plant Biol. 11:339–350.
- Sevim D, Senol FS, Gulpinar AR, Orhan IE, Kaya MK, Sener B. 2013. Discovery of potent in vitro neuroprotective effect of the seed extracts from seven Paeonia L. (peony) taxa and their fatty acid composition. Ind Crop Pro. 49:240–246.
- Shen ZJ, Wang YB, Wang GL, Yan M, Li Z, Liu DY. 2005. Heavy metals pollution of Paeonia ostii land at copper-tailings reservoir of Tongling city: a preliminary study. Chin J Appl Ecol. 16(4):673–677. (in Chinese, with abstract in English).
- Sinha S, Sinam G, Mishra RK, Mallick S. 2010. Metal accumulation, growth, antioxidants and oil yield of Brassica juncea L. exposed to different metals. Ecotoxicol Environ Saf. 73:1352–1361.
- Solis MIV, Patel A, Orsat V, Singh J, Lefsrud M. 2013. Fatty acid profiling of the seed oils of some varieties of field peas (Pisum sativum) by RP-LC/ESI-MS/MS: towards the development of an oilseed pea. Food Chem. 139:986–993.
- Wei XR, Shao MG, Zhuang J, Horton R. 2010. Soil iron fractionation and availability at selected landscape positions in a loessial gully region of northwestern China. Soil Sci Plant Nutr. 56:617–626.
- Xu DC, Zhou P, Zhan J, Gao Y, Dou CM, Sun QY. 2013. Assessment of trace metal bioavailability in garden soils and health risks via consumption of vegetables in the vicinity of Tongling mining area, China. Ecotosicol Environ Saf. 90:103–111.
- Yang ZN, Luo SQ, Yu ZW, Zhao C. 2011. Quality evaluation of Semen persicae (seed of Prunus persica L. Batsch) by gas chromatography-mass spectrometry. Biochem Syst Ecol. 39:183–192.
- Younis YMH, Ghirmay S, Al-Shihry SS. 2000. African Cucurbita pepo L.: properties of seed and variability in fatty acid composition of seed oil. Phytochemistry. 54:71–75.
- Zheljazkov V, Jeliazkova EA, Kovacheva N, Dzhurmanski A. 2008. Metal uptake by medicinal plant species grown in soils contaminated by a smelter. Environ Exp Bot. 64:207–216.
- Zhou Y, Zhang GH, Xu YC, Cheng JG, Chen YH, Li X. 2011. Effects of Cu on Paeonia ostii growth, physiology and element absorption and allocation. Chin J Ecol. 30(3):415–423. (in Chinese, with abstract in English).