ABSTRACT
Many plants exhibit systemic induced resistance (SIR) coordinated among numerous branches after damage to a single localized tissue. Recently, it has been revealed that damaged leaves release a variety of volatile organic compounds (VOCs) into their surroundings that induce SIR. The phenomenon has been described for only four species in field experiments. We investigated induced resistance to herbivory in beech, a dominant tree in Japan. We examined whether volatile cues were required for SIR by determining natural levels of leaf damage for assay branches that were on the same plant as treatment branches. We found when a local branch was mechanically clipped, the proportion of leaf segments that were damaged by herbivores was reduced. However, when a clipped branch was covered with a plastic bag, the proportion of leaf segments that were damaged was the same as that of the control. These results suggested that SIR in beech trees required air contact.
Introduction
It is known that many plants exhibit systemic induced resistance coordinated among numerous branches after damage on a single localized tissue (Karban and Baldwin Citation1997).
The phenomenon is called systemic acquired resistance (SAR) or systemic induced resistance (SIR), and has been reported for various plants such as for hybrid poplar (Populus × euroamericana), tomato (Solanum lycopersicum), arabidopsis (Arabidopsis thaliana), and rice (Oryza sativa). Salicylic acid (SA) and jasmonic acid (JA) are known as signals of SIR through an internal pathway (Heil and Ton Citation2008).
Artificial damage to local leaves resulted in SIR in non-adjacent, orthostichous leaves (vertically aligned on the stem) with direct vascular connections, both up and down the shoot; but no SIR was reported in adjacent, non-orthostichous leaves with less direct vascular connections in eastern cottonwood (Populus deltoides) (Jones et al. Citation1993). For other plants, local damage induced expression of defense genes in non-damaged leaves regardless of the directionality of the phyllotaxis (Arimura et al. Citation2004).
After one part of a plant is attacked, other parts of the same individual may be at higher risk than other individuals (Karban Citation2015). Indeed, it is predicted that intra-plant signaling transmits more rapidly and reliably than the signal transmission to other individuals (Heil and Adame-Álvarez Citation2010).
Recently, it has been revealed that damaged leaves of many species release a variety of volatile organic compounds (VOCs) into their surroundings that induce SIR in undamaged leaves (Arimura et al. Citation2004; Heil and Ton Citation2008; Li and Blande Citation2017b). SIR signal transmission occurs not only through vascular connections but also via volatile organic compounds (VOCs) (Frost et al. Citation2008).
For example, in sagebrush (Artemisia tridentata) external volatile cues may be used instead of limited vascular signaling among branches within an individual (Shiojiri and Karban Citation2008). For several plant species, only those branches that received volatile cues from neighboring clipped branches experienced less damage by herbivores than control branches (Dolch and Tscharntke Citation2000; Karban et al. Citation2006; Shiojiri and Karban Citation2006; Dong et al. Citation2011; Shiojiri et al. Citation2017).
The phenomenon of intra-plant signaling via VOCs has been described only in eight plant species (Li and Blande Citation2017b). Only four of these studies were field experiments: sagebrush (Artemisia tridentata) (Karban et al. Citation2006), lima bean (Phaseolus lunatus) (Heil and Silva Bueno Citation2007), California mugwort (Artemisia douglasiana) (Shiojiri and Karban Citation2008), hybrid aspen (Populus tremula × tremuloides) (Li and Blande Citation2017b).
Although volatile signals, unlike vascular signals, move rapidly and can be detected by all leaves that have air contact with the damaged leaves, the transport of volatiles through air is influenced by many environmental factors such as wind, humidity, and temperature (Blande et al. Citation2014). Therefore, it is very difficult to study using field experiments or natural condition (Karban et al. Citation2014). However, field studies provide important insights into whether and how plants detect and respond to VOCs in the natural environment.
Beech is a dominant tree in many parts of the world, e.g. Fagus sylvatica in Europe, Fagus orientalis in East Asia, Fagus grandifolia in North America. Japanese beech (Fagus crenata Blume) is a dominant tree species throughout cool-temperate mesic forests in Japan (Kon et al. Citation2005) It was reported that herbivores (Quadricalcartfera puncttell or Lymantria dispar) feeding on beech trees induce not only indirect defense but also increased the carbon/nitrogen ratio, and tannin and phenolic compounds (Kamata et al. Citation1996; Aoyama and Koike Citation2011).
Here we asked the following questions: (1) When a branch is mechanically clipped, does beech exhibit SIR and change herbivore damage during the same growing season leaf-cycle under natural conditions? (2) Which pathway, volatile, vascular, or both, is responsible for SIR in intra-plant signaling?
Material and methods
Plant and study site
The study was conducted in the Tomakomai Experimental Forest, Hokkaido University (42°40′N, 141°36′E). The monthly mean temperature ranges from −3.2°C to 19.1°C. Annual rainfall is 1200 mm.
Seeds of Japanese beech (Fagus crenata) were collected from 5 source sites: Kuromatsunai (42.7°N), Ohirayama (42.6°N), Kikonai (41.6°N), Iwate (38.5°N), and Yabe (32.6°N) in Japan. The seedlings were sown in a common garden in 1991. The other trees were propagated from cuttings in the Tomakomai Experimental Forest. The original scions were collected from a planted tree (height = 15.2 m; DBH = 53.3 cm) within the Hokkaido Forestry Research Institute Donan Branch Station, which has been previously used for temperature manipulation experiments (Kon and Noda Citation2007). The root stocks were from 5-year-old seedlings collected from Moheji (41°76 N, 140°59 E) in 1997 (Miyazaki et al. Citation2014). The grafts were transplanted to Tomakomai Experimental Forest (42°40 N, 141°36 E, 40 m a.s.l.) from in the Hokkaido Forestry Research Institute Donan Branch Station (41°50 N, 140°43 E, 50 m a.s.l.) in April 2009 (Miyazaki et al. Citation2014).
All sample trees were grown in a high-light environment under similar soil conditions and tree height was approximately 10 m (the heights of Clone trees were approximately 3 m). Leaf expansion started in early May and leaf abscission started in the middle of October.
Systemic-induced resistance in plants
We examined systemic-induced resistance for plants by determining natural levels of leaf damage for assay branches that were on the same plant as treatment branches, treatments were either: (1) unclipped controls, or (2) mechanically clipped ((a)).
Figure 1. Schematic diagram of the experimental design for systemic-induced resistance for plant. (a) Experiment1 (1) an unclipped branch and five assay branches, (2) a clipped branch and five assay branches. (b)Experiment2 (1) an unclipped control branch and five assay branches, (2) a clipped branch enclosed in a plastic bag and five assay branches, (3) clipped branch and assay branch.
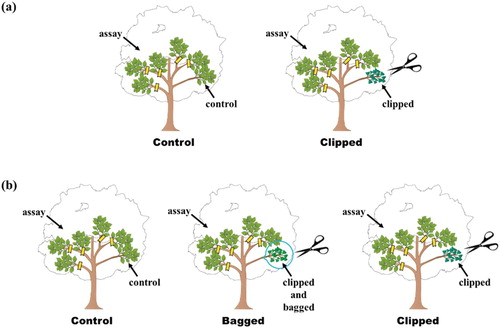
On 3 June 2018, we clipped one treatment branch for plants in the clipped treatment and marked unclipped controls, we marked five assay branches for plants in the treatments. We chose assay branches located 50 cm to 6 m distance along a straight line from clipped branch (1 m–15 m following branches the connected the clipped and assay branches). We included 10 trees from all 5 sites expect for Ohirayama which only had 8 trees. For branches that were assigned to be mechanically clipped, we clipped the distal edge of approximately half of 20–30 leaves with scissors. On 2 September 2018, the number of leaf segments damaged by chewing herbivores and pathogens were recorded for each assay branch. Each leaf segments was a portion of the leaf blade that was surrounded by parallel leaf veins.
Are volatile cues required in systemic induced response?
We examined whether volatile cues are required for systemic induced resistance by determining natural levels of leaf damage for assay branches that were on the same plant as treatment branches. Treatment branches were either: (1) unclipped controls, (2) mechanically clipped, or (3) mechanically clipped but with blocked air contact between the clipped and assay branches ((b)).
For branches that were assigned to be mechanically clipped, we clipped the distal edge of approximately half of 20–30 leaves with scissors. For branches that were assigned to be mechanically clipped and bagged, we clipped leaves, covered the clipped branch with a plastic bag (500 mm × 350 mm) and then sealed the bag with a wire twist-tie. On 1 June 2019, we performed the appropriate treatment to one branch for each of the three treatments and marked the 5 other assay branches for 21 plants. After 10 days, the plastic bag was removed without giving excessive stress to branch. On 30 August 2019, the number of leaf segments damaged by chewing herbivores and pathogens were recorded for each assay branches.
Statistical analyses
To analyze the effect of the treatment on the number of leaf segments damaged, we used a one-way ANOVA and Tukey’s HSD test. The one-way ANOVA and Tukey’s HSD test were conducted using the JMP software package (version 7.0.2.; SAS Institute, Cary, NC, USA). Tukey’s HSD (honestly significant difference) test was used to determine significant differences among treatments in experiments. An alpha level of 0.05 was used for statistical significance. Since we used trees from different sources, the source sites were random effects in the models. Because the total numbers of leaf segments were different for the site sources, we calculated on 3 June 2018 the average number of leaf segments on each source site by randomly selecting ten intact leaves. The results are weighted by the total number of leaves times the average leaf segments of each site.
Results
Systemic-induced resistance in plants
Clipped assay branches got approximately 50% less damage than control branches (; F2, 90 = 26.32, p < 0.001). This result indicates that clipped treatments induced systemic-induced resistance. In this experiment, the assay branch and treatment branch (clipped or unclipped) shared vascular connections aboveground, we could not determine whether this systemic-induced resistance was induced because they shared a vascular connection or volatile cues.
Are volatile cues required in systemic induced response?
Our next experiment tested the hypothesis that air contact was necessary for systemic induced response. When air contact was allowed, damage was reduced by 51% on assay branches compared to control branches (). When air contact was not allowed by bagging the clipped branches, the number of damaged leaf segments was almost the same as controls. Tukey’s HSD (honestly significant difference) test indicated Bagged and Control treatments were not significantly different. This indicates that air contact was required for systemic induced resistance within a single plant.
Discussion
It is known that plants can induce systemic resistance following local damage. Our experiments indicate that mechanically clipped beech leaves caused systemic induced resistance, throughout the individual (Experiment 1). We focus on herbivore damage but not defense traits in this study, and we found that the clipped treatment decreased damage compared with the control and bagged treatments. For the bagged treatment, we covered the clipped branch with a plastic bag after clipping. Because the clipped branch did not continue to emit active cues for more than 7 days, the branches that were bagged for 10 days did not release active cues to neighboring branches. Therefore, volatiles from the clipped branches that were not bagged were responsible for the induced resistance in beech that we observed during this experiment. In several previous studies, volatile organic compounds emitted following actual herbivore damage differenced from these following that of mechanically clipped damage; however, experimental clipping also induced systemic resistance in these plants (Karban et al. Citation2006; Shiojiri and Karban Citation2006; Li and Blande Citation2017a).
Aoyama and Koike (Citation2011) reported that local damage by herbivores (Gypsy moth: Lymantria dispar) increased the condensed tannin, total phenolics and the C/N ratio after 10–30 days compared to undamaged beech leaves. Local damage also induced resistance against subsequent herbivores. However, these measures of plant defense became almost the same as the control treatment after 50 days. Although the quality of defense traits was not measured in this study, we found that leaf damage to the clipped treatment after 90 days was less than half the damage to the control treatment. In brief, the differences in early season defenses may have produced differences in damage that were seen throughout the season.
In other studies, early season attacks by chewers and gall makers were strong negative predictors of later season chewing and galling (Cornelissen et al. Citation2011). Dalin and Bjorkman (Citation2003) determined that Salix cinerea L. are usually attacked again later in the season by leaf beetle (Phratora vulgatissima L.) larvae; nevertheless, larvae on plants previously exposed to adult grazing consumed less total leaf area and showed more dispersed feeding than larvae on plants protected from previous grazing.
Kessler et al. (Citation2006) found that wild tobacco plants (Nicotiana attenuata) with air contact with mechanically clipped sagebrush (Artemisia tridentata), were primed for accelerated proteinase inhibitor (PI) activity when subsequently exposed to experimental feeding by Manduca sexta. Thus, defense priming by VOC exposure can accelerate responses following subsequent attack by herbivores (Kessler et al. Citation2006). In this study, we clipped leaves in the early season and examined the branches later. Our results demonstrate that clipping in the early season may be a strong negative predictor of later season damage. Although we did not examine the activation of defense genes for priming, the beeches which got damage on a local branch might be primed systemically for subsequent attack, and the priming response may lead to our results.
In this study, we considered artificially clipped leaves as damaged. However, leaves that are damaged by herbivores and pathogens produce different emissions in both timing and quantities. Herbivore damage often causes VOC emissions over a longer time than artificial clipping. Therefore, natural damage by herbivores and pathogens may induce resistance more continuously and more strongly. Future studies should focus on natural damage by herbivores via VOCs signaling.
Acknowledgements
Thanks to Associate Professor. Masae Ishihara (Kyoto Univ.) and Professor Tsutomu Hiura (Hokkaido Univ.) for their support of our field work. We also thank Professor. Junji Takabayashi (Kyoto Univ.) for providing helpful suggestions. We would also like to thank Professor Richard Karban (UC Davis) for his assistance with English language and grammatical editing of the manuscript.
Disclosure statement
No potential conflict of interest was reported by the author(s).
Additional information
Funding
Notes on contributors
Tomika Hagiwara
Tomika Hagiwara is interested in interactions by plant volatiles.
Kaori Shiojiri
Kaori Shiojiri is interested in interactions by plant volatiles.
References
- Aoyama C, Koike T. 2011. Temporal changes of induced resistance in the saplings of Fagus crenata and Qeuercus mongolica var. crispula in second year. (in Japanese) Boreal Forest Soc. 59:133–136.
- Arimura G-I, Huber DPW, Bohlmann J. 2004. Forest tent caterpillars (Malacosoma disstria) induce local and systemic diurnal emissions of terpenoid volatiles in hybrid poplar (Populus trichocarpa × deltoides): cDNA cloning, functional characterization, and patterns of gene expression of (−)-germacrene D synthase, PtdTPS1. Plant J. 37:603–616. doi: 10.1111/j.1365-313X.2003.01987.x
- Blande JD, Holopainen JK, Niinemets Ü. 2014. Plant volatiles in polluted atmospheres: stress responses and signal degradation. Plant Cell Environ. 37:1892–1904. doi: 10.1111/pce.12352
- Cornelissen T, Fernandes GW, Coelho MS. 2011. Induced responses in the neotropical shrub Bauhinia brevipes Vogel: does early season herbivory function as cue to plant resistance? Arthropod Plant Interact. 5:245–253. doi: 10.1007/s11829-011-9134-7
- Dalin P, Bjorkman C. 2003. Adult beetle grazing induces willow trichome defence against subsequent larval feeding. Oecologia. 134:112–118. doi: 10.1007/s00442-002-1093-3
- Dolch R, Tscharntke T. 2000. Defoliation of alders (Alnus glutinosa) affects herbivory by leaf beetles on undamaged neighbours. Oecologia. 125:504–511. doi: 10.1007/s004420000482
- Dong F, Yang ZY, Baldermann S, Sato Y, Asai T, Watanabe N. 2011. Herbivore-induced volatiles from tea (Camellia sinensis) plants and their involvement in intraplant communication and changes in endogenous nonvolatile metabolites. J Agric Food Chem. 59:13131–13135. doi: 10.1021/jf203396a
- Frost CJ, Mescher MC, Carlson JE, De Moraes CM. 2008. Plant defense priming against herbivores: getting ready for a different Battle. Plant Physiol. 146:818–824. doi: 10.1104/pp.107.113027
- Heil M, Adame-Álvarez RM. 2010. Short signalling distances make plant communication a soliloquy. Biol Lett. 6:843–845. doi: 10.1098/rsbl.2010.0440
- Heil M, Silva Bueno JC. 2007. Within-plant signaling by volatiles leads to induction and priming of an indirect plant defense in nature. Proc Natl Acad Sci U S A. 104:5467–5472. doi: 10.1073/pnas.0610266104
- Heil M, Ton J. 2008. Long-distance signalling in plant defence. Trends Plant Sci. 13:264–272. doi: 10.1016/j.tplants.2008.03.005
- Jones CG, Hopper RF, Coleman JS, Krischik VA. 1993. Control of systemically induced herbivore resistance by plant vascular architecture. Oecologia. 93:452–456. doi: 10.1007/BF00317892
- Kamata N, Igarashi Y, Ohara S. 1996. Induced response of the Siebold’s beech (Fagus crenata Blume) to manual defoliation. J Forest Res. 1:1–7. doi: 10.1007/BF02348331
- Karban R. 2015. Plant sensing and communication. Chicago: University of Chicago Press.
- Karban R, Baldwin IT. 1997. Induced responses to herbivory. Chicago: University of Chicago Press.
- Karban R, Shiojiri K, Huntzinger M, Mccall AC. 2006. Damage-induced resistance in sagebrush: volatiles are key to intra- and interplant communication. Ecology. 87:922–930. doi: 10.1890/0012-9658(2006)87[922:DRISVA]2.0.CO;2
- Karban R, Wetzel WC, Shiojiri K, Ishizaki S, Ramirez SR, Blande JD. 2014. Deciphering the language of plant communication: volatile chemotypes of sagebrush. New Phytol. 204:380–385. doi: 10.1111/nph.12887
- Kessler A, Halitschke R, Diezel C, Baldwin IT. 2006. Priming of plant defense responses in nature by airborne signaling between Artemisia tridentata and Nicotiana attenuata. Oecologia. 148:280–292. doi: 10.1007/s00442-006-0365-8
- Kon H, Noda T. 2007. Experimental investigation on weather cues for mast seeding of Fagus crenata. Ecol Res. 22:802–806. doi: 10.1007/s11284-006-0320-5
- Kon H, Noda T, Terazawa K, Koyama H, Yasaka M. 2005. Evolutionary advantages of mast seeding in Fagus crenata. J Ecol. 93:1148–1155. doi: 10.1111/j.1365-2745.2005.01040.x
- Li T, Blande JD. 2017a. How common is within-plant signaling via volatiles? Plant Signal Behav. 12:e1347743. doi: 10.1080/15592324.2017.1347743
- Li T, Blande JD. 2017b. Volatile-mediated within-plant signaling in hybrid aspen: required for systemic responses. J Chem Ecol. 43:327–338. doi: 10.1007/s10886-017-0826-z
- Miyazaki Y, Maruyama Y, Chiba Y, Kobayashi MJ, Joseph B, Shimizu KK, Mochida K, Hiura T, Kon H, Satake A, Knops J. 2014. Nitrogen as a key regulator of flowering in Fagus crenata: understanding the physiological mechanism of masting by gene expression analysis. Ecol Lett. 17:1299–1309. doi: 10.1111/ele.12338
- Shiojiri K, Karban R. 2006. Plant age, communication, and resistance to herbivores: young sagebrush plants are better emitters and receivers. Oecologia. 149:214–220. doi: 10.1007/s00442-006-0441-0
- Shiojiri K, Karban R. 2008. Vascular systemic induced resistance for artemisia cana and volatile communication for artemisia douglasiana. Am Midl Nat. 159:468–477, 10. doi: 10.1674/0003-0031(2008)159[468:VSIRFA]2.0.CO;2
- Shiojiri K, Ozawa R, Yamashita KI, Uefune M, Matsui K, Tsukamoto C, Tokumaru S, Takabayashi J. 2017. Weeding volatiles reduce leaf and seed damage to field-grown soybeans and increase seed isoflavones. Sci Rep. 7:8. doi: 10.1038/srep41508