ABSTRACT
The aim of this study was to investigate the optimum time for γ-aminobutyric acid (GABA) application to improve the yield and quality in fragrant rice. Pot and field experiments were conducted during 2016–17 with two fragrant rice cultivars (for pot experiment), and five fragrant rice cultivars (for field experiment) which were applied with five GABA levels i.e. no GABA application (CK), application of GABA at 250 mg l−1 with 25 ml pot−1 at tillering stage (S1), panicle initiation stage (S2), heading stage (S3), and at tillering, panicle initiation and heading stages (S4) in the pot experiment. Similarly, the same treatments with 100 ml m−2 were applied to all rice cultivars in the field experiment. The S3 treatment significantly increased the 2-acetyl-1-pyrroline (2AP) contents in Meixiangzhan2 (14.76%) and Yuxiangyouzhan (20.19%) in pot experiment, Meixiangzhan2 (27.27%), Yuxiangyouzhan (40.24%), Basmati (43.07%) and Yungengyou14 (13.66%) in field experiment owing to regulations in the contents of proline, Δ1-pyrroline-5-carboxylic acid (P5C), GABA and the activities of enzymes involved in 2AP formation. The GABA treatments improved yield and modulated the antioxidant enzyme activities. This study provides a reference for the GABA application to improve yield and quality in fragrant rice.
1. Introduction
Rice is an important cereal crop that feeds billions of people around the world (Mahajan et al. Citation2010). Fragrant rice is a unique rice type and world-famous due to its special aroma, appearance, and taste, thus regarded as a superfine grain (Giraud Citation2013). In recent years, it has become more popular and its demand is increasing in international markets worldwide (Hashemi et al. Citation2013). However, the grain yield was generally lower in fragrant rice than non-fragrant rice cultivars. Thus, some effective strategies are needed and have also been previously employed to improve grain yield production in fragrant rice (Pan et al. Citation2013; Mo et al. Citation2015, Citation2017).
Rice aroma was regarded as one of the most critical quality characters (Das et al. Citation2018), aroma intensities are closely related to the mixture of aroma compounds in rice (Sansenya et al. Citation2018). The 2-acetyl-1-pyrroline (2AP) was reported as a crucial volatile compound involved in the intricate volatile chemistry of aroma in fragrant rice (Ren et al. Citation2017; Boontakham et al. Citation2019), which can be determined in all parts of fragrant rice excluding root (Maraval et al. Citation2010; Mo et al. Citation2015). In recent times, some studies focusing on the formation of aroma have revealed that the betaine-aldehyde dehydrogenase (BADH)-related gene is responsible for the biosynthesis and up–down regulation of 2AP (Bradbury et al. Citation2005; Chen et al. Citation2008). Previous studies reported that catalytic activities of proline dehydrogenase (PDH), Δ1-pyrroline-5-carboxylic acid synthetase (P5CS) and ornithine aminotransferase (OAT) convert the proline, glutamate, and ornithine into a common metabolite i.e. pyrroline-5-carboxylic acid (P5C) and thus act as precursors for several related physiological processes involved in the formation of 2AP in fragrant rice (Mo et al. Citation2016a; Ghosh and Roychoudhury Citation2018). Moreover, diamine oxidase (DAO) and soluble protein have also been found to be associated with grain 2AP production in fragrant rice (Li et al. Citation2016b; Deng et al. Citation2018).
Indeed, the biosynthesis and regulations of 2AP in fragrant rice are genetic-controlled mechanisms, however, agronomic measures and crop management practices can also affect the yield, enzyme activities, and genes involved in 2AP biosynthesis to a great extent (Bao et al. Citation2018). For example, Mo et al. (Citation2019a, Citation2019b) showed that the regulations of 2AP were linked to the dynamics in water and nitrogen application in fragrant rice. Additionally, it appears that the production and accumulation of 2AP can also be linked to the external environmental conditions and abiotic stress factors, e.g. an increment of 2AP contents in rice was detected in response to salt stress (Poonlaphdecha et al. Citation2012). Similarly, Mo et al. (Citation2015) reported that the accumulation of GABA and 2AP were enhanced simultaneously by shading or low light intensity during the grain filling stage in fragrant rice. Besides, Bradbury et al. (Citation2008) indicated that both the biosynthesis of γ-aminobutyric acid (GABA) and the accumulation of Δ1-pyrroline were largely affected by Badh2 gene. Furthermore, it was reported that GABA was closely associated with the plant growth and grain yield (Li et al. Citation2017; Li et al. Citation2019b). Consequently, it is quite feasible to regulate 2AP biosynthesis and grain yield by the exogenous application of GABA in fragrant rice.
GABA is recognized as a signaling molecule that is involved in various physio-biochemical processes to regulate plant stress responses (Ramesh et al. Citation2017; Routray and Rayaguru Citation2018). In general, the endogenous concentration of GABA in plants is relatively low but its levels may be enhanced in response to the various stress conditions (Li et al. Citation2017). Previous studies confirmed that GABA is endowed with the ability to regulate physio-biochemical metabolism in different plants (Yu and Sun Citation2007; Song et al. Citation2010). Li et al. (Citation2016a) suggested that exogenous GABA improved the salt stress tolerance in growing wheat plants due to the enhancement of photosynthesis and antioxidant enzyme activities. Exogenous GABA application improved the chlorophyll contents and other photosynthetic attributes in rice seedlings (Li et al. Citation2017). Xie et al. (Citation2019) revealed that GABA application improved the nutrient uptake in rice. Shang et al. (Citation2011) showed that GABA application played an important role in alleviating chilling injury in cold-stored peach fruit. Li et al. (Citation2019b) demonstrated that GABA application could regulate rice yield and yield-related traits under different nitrogen levels. Hence, exogenous GABA applications can regulate either aroma biosynthesis or plant growth and even the grain yield by affecting the physiological metabolism. Previous studies have well documented the GABA-induced regulations in different plant species, however, a little is known about the effects of GABA application during the different growth stages of fragrant rice to find out the suitable application period or growth stage in fragrant rice. Therefore, the present study was conducted to investigate the effects of exogenous GABA application at different growth stages of fragrant rice on the 2AP contents and the physiological and biochemical attributes involved in 2AP biosynthesis, grain yield formation, grain quality as well as antioxidant defense system in fragrant rice.
2. Materials and methods
2.1. Experimental description
The pot and field experiments were performed in the greenhouse and under field conditions at Experimental Research Farm, College of Agriculture, South China Agricultural University (SCAU), Guangzhou, P.R. China from 2016 to 2017. The experimental region has a humid subtropical-monsoon type climate with an average air temperature of 24.4°C and average humidity of 79.3% in the growing season of rice. The seeds were collected from the College of Agriculture, South China Agricultural University, Guangzhou, China. The soil of pot experiment was sandy loam with 21.65 g kg−1 organic matter, 152 g kg−1 total N, 1.02 g kg−1 total P, 19.34 g kg−1 total K, and 6.40 soil pH whereas the soil of the field experiment was sandy loam containing 21.74 g kg−1 organic matter, 1.70 g kg−1 total N, 1.30 g kg−1 total P, 20.10 g kg−1 total K, and 6.34 soil pH.
2.2. Experimental treatments and design
The experiments were conducted with two fragrant rice cultivars (Meixiangzhan2 and Yuxiangyouzhan) for pot experiment, and with five fragrant rice cultivars (Meixiangzhan2, Yuxiangyouzhan, Basmati, Xiangyaxiangzhan and Yungengyou14) for field experiment. Those cultivars were globally /regionally popular due to their special aroma and better cooking qualities. The five GABA levels i.e. no GABA application (CK), application of GABA at 250 mg l−1 with 25 ml pot−1 at tillering stage (S1), panicle initiation stage (S2), heading stage (S3), and at tillering, panicle initiation and heading stages (S4) were applied in the pot experiment. Similarly, the same treatments with 100 ml m−2 were applied to all rice cultivars in the field experiment. The crop management practices and pest control were carried out according to standard cultural practices.
The pot experiment was arranged in randomized complete block design (RCBD) with seven replications for each treatment. The commercial compound fertilizer (N: P2O5: K2O = 15:15:15) was applied in all the pots with 3.5 g pot−1 as basal fertilizer. Each pot (32 cm in diameter and 24 cm in height) was filled with 12 kg of sun-dried soil and transplanted with 30 days old rice seedlings with 3 seedings per hill and five hills per pot at two days after application of basal fertilizer. An additional dose of fertilizer was applied with 3.5 g per pot at the tillering stage.
The field experiment was arranged in spilt-plot design with three replications, with rice cultivars were assigned to the main plot and GABA treatments were kept in the sub-plot. The net plot size was 5 × 3 m. The NPK at 90–150–180 kg hm−2 was applied as basal fertilizer and no additional fertilizer was applied later on. The rice seedlings were transplanted by using rice transplanter (2Z-8A2-PZ80-HDRT25) at 30 × 14 cm planting distance.
2.3. Sampling and measurement
Plant sampling was done during 9:00–11:00 am at the heading stage (HS), 15 days after heading stage (15 d AH), and maturity stage (MS). At HS, 15 d AH and MS thirty flag leaves of the main stem, and at 15 d AH and MS fifteen panicles were sampled randomly. The panicles were divided into two parts: one was stored at −20°C for determination of 2AP content and the other together with all the flag leaves were frozen by liquid nitrogen and stored at −80°C for physio-biochemical assays.
2.3.1. Determination of grain 2AP contents
The grain 2AP contents were determined by the synchronization distillation and extraction method (SDE) combined with GCMS-QP 2010 Plus (Shimadzu Corporation) as described by Huang et al. (Citation2012). The chromatographic condition was described as following: gas chromatograph equipped with a RESTEK Rxi-5 ms (Shimadzu, Japan) silica capillary column (30 m × 0. 32 mm × 0. 25 μm). The temperature of the GC oven was kept at 45°C (1 min), increased at the rate of 2°C per min to 65°C and kept at 65°C for 1 min, and then increased to 220°C at the rate of 10°C per min, and kept at 220°C for 10 min. The auto-injector was AOC-20i, SPL1. High purity helium gas (99.999%, Guangzhou Gases Co., LTD., China) was the carrier gas at a constant flow rate of 2 ml per min. The temperature of the ion source was 200°C. Under these conditions, the retention time of 2AP was 7.5 min. The contents of 2AP in grains were defined as μg g−1.
2.3.2. Determination of proline, P5C, GABA and soluble protein contents in leaves and grains
The proline contents in leaves and grains were determined according to Bates et al. (Citation1973) and the contents of proline were expressed as μg g−1 FW, whereas the P5C contents were determined by Miller et al. (Citation2009) and were defined as μmol g−1 FW. The GABA contents in leaves and grains were determined according to Zhao et al. (Citation2009) and expressed as mg g−1 FW, whereas the soluble protein contents were detected by using the method as described by Kong et al. (Citation2017) and expressed as μg g−1 FW.
2.3.3. Determination of PDH, P5CS, OAT and DAO activities in leaves and grains
Crude enzymes were extracted according to the method represented previously (Tripathi et al. Citation2013; Naliwajski and Skłodowska Citation2014). The PDH activity was assayed by the method devised by Ncube et al. (Citation2013) and was expressed as U g−1 min−1 FW, while the P5CS activity was determined according to Sánchez et al. (Citation2002) and was expressed as U g−1 min−1 FW. The OAT activity was measured by using the method of Umair et al. (Citation2011) and was expressed as U g−1 min−1 FW whereas the DAO activity was estimated according to the method of Su et al. (Citation2005) and was expressed as U g−1 min−1 FW.
2.3.4. Determination of antioxidant enzyme activities and malondialdehyde (MDA) contents
The antioxidant enzyme activities i.e. superoxide dismutase (SOD), peroxidase (POD), and catalase (CAT), as well as MDA contents, were assayed according to the procedures of Li et al. (Citation2019a). In brief, fresh leaves (0.3 g) were homogenized with 5 ml of 50 mM sodium phosphate buffer (pH 7.8) and centrifuged at 8000 rpm at 4°C for 15 min and the supernatant was the crude enzyme extract. The SOD activity was defined as U g−1 FW, while the POD and CAT activities were defined as U g−1 min−1 FW, as well as the MDA contents were expressed as μmol g−1 FW.
2.3.5. Determination of grain yield and yield related traits
For the pot experiment, six pots from each treatment at maturity stage were sampled to determine grain yield and yield-related traits. The panicle number per pot was measured and averaged from the six pots. The filled grain number per pot and total grain number per pot were recorded from the panicles threshed manually, the 1000-grain weight was recorded by counting and weighing five random samples from filled grains (Mo et al. Citation2017).
For the field experiment, at maturity stage, the panicle number from the fifty representative plants was investigated in each plot. Four hills from different locations of each plot were sampled to determine the total grain number, the filled grain number, grain-filling percentage, and the 1000-grain weight (Mo et al. Citation2015, Citation2017).
2.3.6. Determination of grain quality
Grains were stored at room temperature for three months to measure grain quality attributes after sun drying. About 1.5 kg grains from each treatment were weighed and brown rice rate was estimated by using FC2 K rice huller (Jiangsu, China), whereas milled and head milled rice rates were assayed by JNMJ6 rice polishing machine (Zhejiang, China). The percentage of chalky grain and chalkiness degree were analyzed by using SDE-A lightbox (Guangzhou, China). The Infratec1241 grain analyzer (FOSS-TECATOR) was used to determine amylose content, protein content, and alkali of grains (Mo et al. Citation2015).
2.3.7. Statistical analysis
Microsoft office 2013 was used as a tool to log, dispose, and calculate data. The data analysis and the evaluation of relationships among the indexes were conducted using Statistix version 8 (Analytical Software, Tallahassee, Florida, USA). The significance of differences amongst means of different treatments was determined by the least significant difference (LSD) test at the 0.05 probability level.
3. Results
3.1. 2AP contents
For pot experiment, compared with CK, the GABA application improved the grain 2AP contents in both cultivars. The S3 treatment increased the grain 2AP contents of MX2 and YX by 14.76% and 20.19% as compared to CK whereas the highest 2AP contents were recorded under the S3 and S4 treatments for MX2 and YX, respectively.
For field experiment, the application of GABA led to substantial improvements in grain 2AP contents of YX, XY, and YG14. Compared to CK, the grain 2AP contents of MX2, YX, BS, and YG14 were increased by 27.27%, 40.24%, 43.07%, and 13.66% under the S3 treatment. Moreover, the highest grain 2AP contents were recorded under S3 for MX2 and YX, under S4 for BS and XY as well as under S2 for YG14 ().
Table 1. Effects of different γ-aminobutyric acid application periods on the contents of 2AP in grains.
3.2. The proline, P5C, GABA and soluble protein contents in leaves
For pot experiment, the proline contents were significantly affected by the GABA application, all the GABA treatments substantially increased the proline contents for MX2 at HS and MS. The S1 and S3, S2 and S4 treatments significantly improved the proline contents for YX at HS and MS, respectively. However, the GABA application marginally affected the P5C contents for MX2 and YX, for MX2, the S1, S3 and S4 treatments significantly reduced the P5C contents at HS, while the S1, S2 and S4 treatments significantly improved the P5C contents at 15 d AH. Furthermore, for MX2, the S2, S3 and S4 treatments significantly increased the GABA contents, while for YX, the GABA contents were found higher under the S1 and S4 treatments at MS. Likewise, the S1 and S4 treatments significantly increased the soluble protein contents for YX at HS, however, the S3 and S4 treatments significantly reduced the soluble protein contents for MX2 at HS and 15 d AH, respectively ().
Table 2. Effects of different γ-aminobutyric acid application periods on the contents of proline, P5C, GABA and soluble protein in leaves.
For field experiment, all the GABA treatments significantly reduced the proline contents for YX at MS, BS at 15 d AH and MS as well as YG14 at HS and MS, and the S1 and S3 treatments reduced the proline contents for MX2 and XY at HS and MS as well as YX at HS, MX2 at HS, respectively. Additionally, the S3 and S4 treatments significantly increased the proline contents for YX at HS and 15 d AH. Furthermore, all the GABA treatments, S2 and S3 treatments significantly increased the P5C contents for BS at MS and YG14 at HS, YX and YG14 at 15 d AH, respectively. Additionally, the S4 treatment significantly improved the P5C contents for MX2 at MS, BS at HS and YG14 at 15 d AH. Likewise, all the GABA treatments, as well as S2 and S3, S3 and S4 significantly increased the GABA contents for BS at HS, YG14 at 15 d AH and MS, YX and BS at 15 d AH and MS, respectively. Additionally, the S1 treatment significantly improved the GABA contents for YX at MS, XY at HS and 15 d AH as well as YG14 at HS and MS. For the soluble protein contents, the S2 and S3 treatments significantly increased the soluble protein contents for MX2 at MS and YG14 at 15 d AH, while the S1 and S3 treatments significantly increased the soluble protein contents for YX and BS at 15 d AH as well as YG14 at MS, YX and XY at 15 d AH and MS, respectively ().
3.3. The proline, P5C, GABA and soluble protein contents in grains
Significant effects of GABA application on the proline contents were detected. All the GABA treatments significantly increased the proline contents for YX2 and XY at 15 d AH, YG14 at MS, whereas the S1, S2 and S3 treatments significantly improved the proline contents for BS at 15 d AH. Additionally, the S2 and S4 treatments significantly improved the proline contents for MX2 at MS and YX at 15 d AH, YX at 15 d AH and BS at MS, respectively. However, all the GABA treatments significantly decreased the P5C contents for YX at 15 d AH, while the S1, S2 and S4 treatments significantly increased the P5C contents for MX2 and XY at MS, MX2 at MS, BS at 15 d AH and MS as well as MX2 at MS. Furthermore, significant effects were noted on the GABA contents for MX2, YX, BS and YG14. All the GABA treatments, S3 and S4 treatments significantly increased the GABA contents for MX2 at MS as well as BS and YG14 at 15 d AH, YX at 15 d AH and BS at MS, respectively. Likewise, significant effects were recorded regarding the soluble protein contents for YX, BS, XY and YG14. The S1, S2 and S4 treatments improved the soluble protein contents for BS at 15 d AH and MS as well as YG14 at 15 d AH, BS and XY at 15 d AH, YX and BS at 15 d AH as well as XY and YG14 at 15 d AH, respectively, while the S3 treatment significantly decreased the soluble protein contents for YX at MS and YG14 ().
Table 3. Effects of different γ-aminobutyric acid application periods on the contents of proline, P5C, GABA and soluble protein in grains in field experiment.
3.4. The PDH, P5CS, OAT and DAO activities in leaves
For pot experiment, all the GABA treatments significantly increased the PDH activity for MX2 at HS and MS whereas the S2 and S3 treatments significantly decreased the PDH activity for YX at 15 d AH. For the P5CS activity, the S1 treatment significantly increased the P5CS activity for MX2 at MS as well as YX at HS and MS whereas the S3 and S4 treatments significantly increased the P5CS activity for MX2 at HS, YX at MS and MX2 at HS, respectively. Furthermore, the S3, both the S2 and S4 treatments significantly increased the OAT activity for YX at HS, MX2 at 15 d AH, respectively. Likewise, the GABA treatments showed a significant effect on the DAO activity. All the GABA treatments significantly increased the DAO activity for MX2 at MS, while the S3 and S4 treatments significantly increased the DAO activity for YX at 15 d AH. Additionally, the S1, S2 and S4 treatments substantially improved the DAO activity for YX at HS and MS, MX2 at HS and YX at MS, YX at MS, respectively ().
Table 4. Effects of different γ-aminobutyric acid application periods on the activities of PDH, P5CS, OAT and DAO in leaves.
For field experiment, all the GABA treatments significantly decreased PDH activity for XY at HS and the S1 and S4 treatments significantly reduced the PDH activity for BS at HS, YX at 15 d AH and XY at MS, respectively, while the S3 and S4 treatments significantly increased the PDH activity for XY at 15 d AH and YG14 at HS. Moreover, GABA treatments significantly affected the P5CS activity for MX2, YX, BS and XY. All the GABA treatments significantly increased the P5CS activity for MX2 at HS, and the S1 and S3 treatments significantly increased the P5CS activity for XY at HS and 15 d AH as well as YG14 at HS, YX at HS and 15 d AH as well as XY at HS, respectively, while the S2, S3 and S4 treatments significantly decreased P5CS activity for BS at HS, and the S2 and S4 treatments significantly reduced the P5CS activity for MX2, YX and XY at MS. Furthermore, the S3 and S4 treatments significantly increased the OAT activity for MX2 at HS and MS as well as XY at HS, while the S2 treatment significantly decreased the OAT activity for YX at 15 d AH and MS. However, all the GABA treatments significantly decreased the DAO activity for XY at HS whereas the S1, S2, S3 and S4 treatments significantly increased the DAO activity for YG14 at HS and BS at MS, MX2 at 15 d AH, YX at 15 d AH and BS at MS, MX2 and YX at 15 d AH, respectively ().
3.5. The PDH, P5CS, OAT and DAO activities in grains
The GABA application marginally affected the PDH and DAO activities, however, the S1 and S2 treatments substantially improved the PDH activity for MX2 at MS whereas the S2 and S3, S3 and S4 treatments decreased the PDH activity significantly for BS at MS and YG14 at 15 d AH, respectively. All the GABA treatments significantly decreased the DAO activity for BS at 15 d AH. Furthermore, all the GABA treatments significantly increased the P5CS activity for BS and YG14 at 15 d AH and XY at MS whereas the S1, S2 and S4 treatments significantly decreased the P5CS activity for YX at MS. The S2 and S3, S3 and S4 treatments led to decline in the P5CS activity for MX2 at 15 d AH, MX2 at MS and XY at 15 d AH, respectively. Moreover, the S1 treatment reduced the P5CS activity significantly for MX2 at 15 d AH and MS. Likewise, the OAT activities were significantly affected by the GABA treatments. All the GABA treatments significantly decreased the OAT activity for MX2 at 15 d AH and YG14 at MS whereas the S1 and S4, S3 and S4 treatments significantly increased the OAT activity for BS at 15 d AH and MS, XY at 15 d AH, respectively, and the S2 and S3 treatments significantly increased the OAT activity for YX at 15 d AH and BS at MS, MX2 at MS and BS at 15 d AH, respectively ().
Table 5. Effects of different γ-aminobutyric acid application periods on the activities of PDH, P5CS, OAT and DAO in grains in field experiment.
3.6. Grain yield and yield related traits
Significant variations were noted in both yield and yield traits under the different GABA treatments. For MX2 and YX, the S4 treatment enhanced the grains per panicle by 14.40% and 25.06%, respectively, whereas for BS, the 1000-grain weight under the S4 treatment was significantly higher as compared with CK. The grain yield was found higher in YX under the S4 treatment than CK. Moreover, both the S2 and S3 treatments produced 16.70% and 24.77% higher grains per panicle for XY whereas the S3 and S4 treatments improved the filled grain percentage for YG14 by 16.87% and 11.05%, respectively. In addition, the S2 treatment significantly increased the grain yield for MX2 and YX by 16.90% and 22.93% as compared with CK ().
Table 6. Effects of different γ-aminobutyric acid application periods on yield and yield related traits in field experiment.
3.7. The SOD, POD, CAT activities and MDA contents
Significant effects of the GABA treatments on the antioxidant enzyme activities and the MDA contents were noted. All the GABA treatments improved the SOD activity for MX2 and XY at HS whereas the S1 and S3 treatments significantly improved the SOD activity for XY and YG14. The S1 and S4, S2 and S3 treatments substantially enhanced the SOD activity for MX2 and YX at 15 d AH, YX at HS, respectively, while the S2 and S4 treatments decreased the SOD activity for MX2 and BS, BS, respectively. Likewise, all the GABA treatments resulted in higher POD activities for YX and BS at MS and MX2 at HS. The S1 and S2, S3 and S4 treatments significantly increased the POD activity for YX at HS and BS at 15 d AH as well as YG14 at MS, XY and YG14, respectively, nevertheless, the S1 and S2 treatments reduced the POD activity for MX2 at 15 d AH and MS as well as XY at MS. Additionally, the S4 treatment significantly increased the POD activity for YX and BS at HS and 15 d AH. Furthermore, the CAT activity was substantially increased under GABA application for YX, BS and YG14 at MS as well as MX2 at 15 d AH, whereas the S3 and S4, S2 and S4 treatments significantly increased the CAT activity for XY at 15 d AH and MS, MX2 at MS and BS at 15 d AH, respectively. The S1 and S3 treatments significantly reduced the CAT activity for MX2 at MS and YX at HS. Moreover, the S2, S3 and S4 treatments significantly reduced the CAT activity for YX and YG14 at 15 d AH as well as XY, YX at 15 d AH and XY at HS, YX at HS and YG14 at MS, respectively. Moreover, the higher contents of MDA were detected for BS and YG14 at HS under the GABA treatments and the S3 and S4 treatments significantly increased the MDA contents for MX2 at MS, YX at HS and 15 d AH as well as XY at 15 d AH, but the S1 and S2, S2 and S3 treatments significantly decreased the MDA contents for YG14 at MS and MX2 at 15 d AH, respectively ().
Table 7. Effects of different γ-aminobutyric acid application periods on the activities of SOD, POD, CAT and the content of MDA in field experiment.
3.8. Grain quality
Significant effects were noted on the grain quality traits under the GABA treatments. For instance, the S4 treatment significantly increased the grain protein contents in MX2, YX and BS whereas all the GABA treatments significantly reduced the rate of chalky grains and the degree of chalkiness for MX2 and BS as well as the alkali for YG14. The S2, S3 and S4 treatments substantially reduced the rate of chalky grains and the degree of chalkiness for XY. Moreover, higher amylose contents for BS were found under the S2 and S4 treatments, while for YG14, the amylose contents were remained lower under the same treatments. Additionally, the S4 treatment significantly improved the brown and milled rice rate for MX2, BS and XY, whilst both the S2 and S3 treatments caused a significant improvement in brown and milled rice rate for XY ().
Table 8. Effects of different γ-aminobutyric acid application periods on grain quality in field experiment.
3.9. Correlation analysis
In field experiment, non-significant associations were observed of grain 2AP contents with proline contents in leaves at HS and 15 d AH, GABA contents in leaves, P5CS and OAT activities in grains and OAT activities in leaves at HS and 15 d AH. The grain 2AP contents showed significant and positive correlations with grain P5C contents at 15 d AH, grain DAO activity at 15 d AH, proline contents in leaves at MS, grain proline and GABA contents. However, significant and negative correlations were observed between grain 2AP with DAO activities in leaves at 15 d AH and MS, OAT, P5CS activities and P5C contents in leaves at MS (). In pot experiment, no significant correlation between 2AP content and other physiological-biochemical attributes were observed (data not shown). Moreover, grain yield was significantly and positively associated with filled grains percentage, and grains per panicle ().
Figure 1. Correlation analyses between grain yield and the investigated parameters in field experiment. MX2: Meixiangzhan2; YX: Yuxiangyouzhan; BS: Basmati; XY: Xiangyaxiangzhan; YG14: Yungengyou 14. * Significant at P < 0.05; ** Significant at P < 0.01; ns nonsignificant at P > 0.05 level.
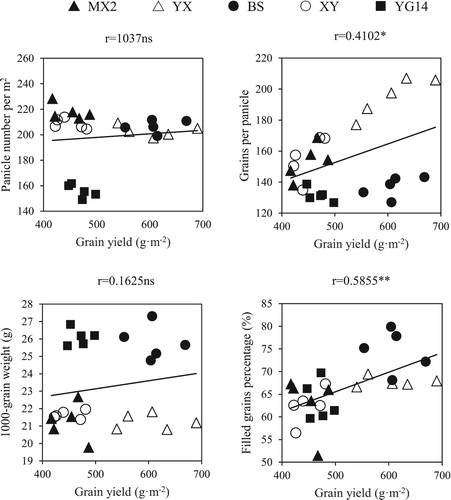
Table 9. Correlation analyses between 2AP contents in grains at maturity and the investigated parameters in field experiment.
4. Discussion
GABA is a non-protein amino acid that acts as a signaling molecule that regulates growth and development, metabolism and stress response in plants (Ramesh et al. Citation2017). It was found that the GABA concentration tends to be higher in response to environmental stresses such as drought, UV irradiation, mechanical damage, extreme temperature, salinity, and hypoxic conditions (Bouché and Fromm Citation2004). The positive effects of GABA on different plants under adverse conditions have also been widely studied. For instance, the application of GABA could significantly improve the growth and productivity of black cumin under water deficit conditions (Rezaei-Chiyaneh et al. Citation2018). Exogenous application of GABA could transiently increase the tolerance to root hypoxia of Prunus rootstock (Salvatierra et al. Citation2016). In the present study, GABA-induced modulations in 2AP contents and enzymes involved in its biosynthesis as well as grain yield of rice (, , and ) were estimated.
GABA application during the different growth stages of fragrant rice could result in regulations in physiological metabolism and growth. For example, the application of GABA at panicle initiation stage could regulate the aroma formation for three aromatic rice cultivars (Xie et al. Citation2019). Xie et al. (Citation2020) indicated that spraying GABA at the initial heading stage could modulate the grain 2AP contents, grain yield and grain quality in fragrant rice. In the present study, application of GABA at heading stage (S3) or at all the three stages (i.e. tillering stage, panicle initiation stage and heading stage) (S4) promoted the grain 2AP contents whereas application of GABA at panicle initiation stage (S2) improved grain yield of rice ( and ).
The 2AP is considered one of the most important volatiles responsible for the fragrance of aromatic rice (Buttery et al. Citation1983). Previous studies reported proline as the most important precursor of 2AP (Daygon et al. Citation2017). The grain 2AP contents are directly related to the enhancement in proline contents in grains (Wang et al. Citation2013). Besides, proline or 2AP is likely to transport from leaves to grains (Buttery et al. Citation1983; Maraval et al. Citation2010). In the present study, GABA application significantly reduced the proline contents in leaves at MS and the contents of proline at MS were substantially decreased as compared with proline contents at 15 d AH (), the result in field experiment showed the transference of proline from leaves to grains during grain filling thus resulted in more proline contents in grains which involved in 2AP formation. The significant and positive correlation between grain proline and 2AP contents () confirms the involvement of proline in 2AP biosynthesis. However, Tang and Wu (Citation2006) revealed the differences in proline contents in leaves at MS and the 2AP contents from 14 days after full heading stage to MS under zinc, iron, and lanthanum application. In the pot experiment, a significant increase or relative stability of proline contents in leaves at HS and MS was noted under the GABA treatments (), whereas the S3 and S4 treatments also increased grain 2AP contents that were significantly related to the proline contents in leaves at MS.
Moreover, the 2AP contents were also associated with the GABA contents in grains but not with the GABA contents in the leaves of aromatic rice (Poonlaphdecha et al. Citation2012). Mo et al. (Citation2015) reported that the 2AP contents were significantly and positively correlated with grain GABA contents in fragrant rice. The application of exogenous GABA resulted in more GABA accumulation in grains. In general, the GABA in grains is synthesized via GABald or by the conversion of glutamate (Bouché and Fromm Citation2004; Fait et al. Citation2008). Moreover, the biosynthesis and accumulation of 2AP are associated with glutamate and GABald (Xie et al. Citation2020). In the present study, it was detected that both the S3 and S4 treatments significantly enhanced the GABA contents in grains () whereas the leaf GABA contents were significantly increased under the S3 treatment at 15 d AH (). Therefore, regulation in glutamate and GABald involved in the up/down regulation of aroma formation in rice. Furthermore, a significant and positive correlation between 2AP and GABA contents in grains was noted (). Therefore, the increase in grain 2AP contents under the S3 and S4 treatments may be attributed to the improvement of GABA contents in grains.
In addition to proline, the P5C and the enzymes i.e. PDH, P5CS, OAT, and DAO were also found to be involved in the biosynthesis of 2AP (Chen et al. Citation2008; Kaikavoosi et al. Citation2015). Previously, Li et al. (Citation2016a) and Mo et al. (Citation2016b) reported a significant and positive association between 2AP and P5C contents in rice. Mo et al. (Citation2019a) suggested that P5C content and DAO activity were closely related to grain 2AP contents. In the present study, it was found that the GABA application improved the P5C contents in grains and leaves at MS ( and ), whereas the S2 and S4 treatments substantially increased the P5C contents in leaves at 15 d AH and MS, respectively (). Moreover, the P5CS and OAT activities in leaves at HS reminded higher under the S3 treatment () whilst the P5CS and OAT activities in grains were varied depending upon GABA treatments and rice genotypes (). Furthermore, the significant and positive correlation between 2AP and P5C contents in grains was also observed (). Thus, application of exogenous GABA caused higher P5C content and led to more 2AP accumulation in grains. However, further studies at molecular and metabolic levels are still needed to better understand the positive role of GABA application at suitable growth stage in aroma formation in fragrant rice.
Previously, exogenous GABA applications improved the growth, development, and photosynthesis in plants (Luo et al. Citation2011; Li et al. Citation2016a). GABA affected grain yield which on the other hand was significantly associated with grain weight (Li et al. Citation2019b). In the present study, the S2 treatment substantially improved the grain yield owing to an improvement in grains per panicle (). For example, grains per panicle as well as grain yield were increased under the S2 treatment for YX and BS. Moreover, grain yield was significantly and positively associated with grains per panicle and filled grains percentage (). Furthermore, GABA-induced modulations in antioxidant enzyme activities i.e. SOD, POD, and CAT and MDA contents were previously noted (Li et al. Citation2016a). Malekzadeh et al. (Citation2014) indicated an increase in antioxidant enzyme activities under GABA application which confirms our findings that application of GABA significantly increased the SOD activity at 15 d AH, CAT activity at 15 d AH and MS, as well as POD activity at HS and MS (). Interestingly, contrary to the reports of Priya et al. (Citation2019), exogenous GABA application enhanced the MDA contents, which indicates that exogenous GABA application might cause stress in fragrant rice. However, AL-Quraan et al. (Citation2015) demonstrated that the significant increase in GABA and MDA accumulation occurs simultaneously under treatment with the three 1,2,3-thiadiazole compounds and discovered GABA molecule may act as a defense mechanism to alleviate oxidative damages. Further, GABA can trap MDA directly and indirectly, as reported by Deng et al. (Citation2010).
Additionally, GABA also plays an important role in ion absorption, nitrate uptake and nitrogen metabolism in plants (Ma et al. Citation2016). For instance, GABA could regulate the nitrate concentration and metabolism in the leaves of Pakchoi (Brassica campestris ssp. chinensis Makino) (Li et al. Citation2018). In the present study, different rice cultivars differed in their grain quality attributes, for example, significant influences in grains with chalkiness, chalkiness degree were observed under the GABA treatments, thereinto, down-regulation was noted in MX2, YX, and XY, but in YX and YG14, the two attributes were on the increase, while for protein, a substantial increase was observed in MX2 and BS whereas significant reduction was noted in XY and YG14 (). The growth and productivity of rice varies among different rice genotype (Li et al. Citation2019b). These results suggested that the interactive effects of GABA and cultivar influenced grain quality attributes, however, the molecular mechanisms about GABA-cultivar interaction need to be further studied. Overall, exogenous GABA applications during different growth stages of fragrant rice modulated the grain 2AP contents, enzymes involved in its formation and grain yield.
5. Conclusions
In conclusion, GABA application could improve the grain yield and 2AP contents in fragrant rice. Application of GABA at heading stage or at all (tillering, panicle initiation, and heading) stages significantly increased the grain 2AP contents across all cultivars due to the improvement of proline contents in leaves, grain proline and GABA contents. A significant increase in grain yield was noted under the GABA treatments owing to an increment in filled grains percentage, grains per panicle. Besides yield, GABA application also affected the grain quality attributes. Overall, our result suggested that the application of GABA regulate the grain yield formation and 2AP accumulation in fragrant rice.
Authors’ contributions
Z.M. designed the experiments; Z.G., W.X., Y.L., L.M. and R.G. investigated the traits; Z.G., W.X. and U.A. analyzed the data and wrote the manuscript; Z.M., U.A., G.P., H.T., M.D., S.W., and X.T. revised and edited the manuscript. X.T. was the leader of our research team. All authors read and approved the final manuscript.
Disclosure statement
No potential conflict of interest was reported by the author(s).
Additional information
Funding
Notes on contributors
Zifeng Gao
Zifeng Gao is an undergraduate student of the Ding Ying Innovation class of Agronomy, College of Agriculture, South China Agricultural University.
Wenjun Xie
Wenjun Xie is an undergraduate student of the College of Agriculture, South China Agricultural University. Wenjun Xie research directions are rice cultivation and physiology.
Umair Ashraf
Umair Ashraf is an assistant professor at the Department of Botany, Division of Science and Technology, University of Education, Lahore, Punjab, Pakistan. His works include the research on crop production and physiology.
Yuzhan Li
Yuzhan Li is an undergraduate student of the College of Agriculture, South China Agricultural University. Li research directions are rice cultivation and physiology.
Lin Ma
Lin Ma is an undergraduate student of the College of Agriculture, South China Agricultural University. Ma research directions are rice cultivation and physiology.
Runfei Gui
Runfei Gui is an undergraduate student of the College of Agriculture, South China Agricultural University. Gui research directions are rice cultivation and physiology.
Shenggang Pan
Shenggang Pan is faculty of College of Agriculture, South China Agricultural University. Pan main works include the research on crop production and physiology, and the physiology and ecology response of fragrant rice and super rice.
Hua Tian
Hua Tian is faculty of College of Agriculture, South China Agricultural University. Tian main works include the research on crop production and physiology, and the physiology and ecology response of fragrant rice and super rice.
Meiyang Duan
Meiyang Duan is faculty of College of Agriculture, South China Agricultural University. Duan main works include the research on crop production and physiology, and the physiology and ecology response of fragrant rice and super rice.
Shuli Wang
Shuli Wang is faculty of College of Agriculture, South China Agricultural University. Wang main works include the research on crop production and physiology, and the physiology and ecology response of fragrant rice and super rice.
Xiangru Tang
Xiangru Tang is faculty of College of Agriculture, South China Agricultural University. Tang main works include the research on crop production and physiology, and the physiology and ecology response of fragrant rice and super rice.
Zhaowen Mo
Zhaowen Mo is faculty of College of Agriculture, South China Agricultural University. Mo main works include the research on crop production and physiology, and the physiology and ecology response of fragrant rice and super rice.
References
- AL-Quraan NA, AL-Smadi ML, Swaleh AF. 2015. GABA metabolism and ROS induction in lentil (Lens culinaris Medik) plants by synthetic 1,2,3-Thiadiazole compounds. J Plant Interact. 10:185–194. doi:10.1080/17429145.2015.1056262.
- Bao G, Ashraf U, Wang C, He L, Wei X, Zheng A, Mo Z, Tang X. 2018. Molecular basis for increased 2-acetyl-1-pyrroline contents under alternate wetting and drying (AWD) conditions in fragrant rice. Plant Physiol Biochem. 133:149–157. doi:10.1016/j.plaphy.2018.10.032.
- Bates LS, Waldren RP, Teare ID. 1973. Rapid determination of free proline for water-stress studies. Plant Soil. 39:205–207. doi:10.1007/BF00018060.
- Boontakham P, Sookwong P, Jongkaewwattana S, Wangtueai S, Mahatheeranont S. 2019. Comparison of grain yield and 2-acetyl-1-pyrroline (2AP) content in leaves and grain of two Thai fragrant rice cultivars cultivated at greenhouse and open-air conditions. Aust J Cereal Sci. 13:159–169. doi:10.21475/ajcs.19.13.01.p1431.
- Bouché N, Fromm H. 2004. GABA in plants: just a metabolite? Trends Plant Sci. 9:110–115. doi:10.1016/j.tplants.2004.01.006.
- Bradbury LM, Fitzgerald TL, Henry RJ, Jin Q, Waters DL. 2005. The gene for fragrance in rice. Plant Biotechnol J. 3:363–370. doi:10.1111/j.1467-7652.2005.00131.x.
- Bradbury LM, Gillies SA, Brushett DJ, Waters DL, Henry RJ. 2008. Inactivation of an aminoaldehyde dehydrogenase is responsible for fragrance in rice. Plant Mol Biol. 68:439–449. doi:10.1007/s11103-008-9381-x.
- Buttery RG, Ling LC, Juliano BO, Turnbaugh JG. 1983. Cooked rice aroma and 2-acetyl-1-pyrroline. J Agric Food Chem. 31:823–826. doi:10.1021/jf00118a036.
- Chen S, Yang Y, Shi W, Ji Q, He F, Zhang Z, Cheng Z, Liu X, Xu M. 2008. Badh2, encoding betaine aldehyde dehydrogenase, inhibits the biosynthesis of 2-acetyl-1-pyrroline, a major component in rice fragrance. Plant Cell. 20:1850–1861. doi:10.1105/tpc.108.058917.
- Das B, Sengupta S, Kole B, Ghose TK. 2018. Elucidation of aroma levels within a set of rice landraces by means of aroma linked markers. J Pharmacogn Phytochem. 7:1756–1760.
- Daygon VD, Calingacion M, Forster LC, De Voss JJ, Schwartz BD, Ovenden B, Alonso DE, McCouch SR, Garson MJ, Fitzgerald MA. 2017. Metabolomics and genomics combine to unravel the pathway for the presence of fragrance in rice. Sci Rep. 7:8767. doi:10.1038/s41598-017-07693-9.
- Deng QQ, Ashraf U, Cheng SR, Sabir SR, Mo ZW, Pan SG, Tian H, Duan MY, Tang XR. 2018. Mild drought in interaction with additional nitrogen dose at grain filling stage modulates 2acetyl-1-pyrroline biosynthesis and grain yield in fragrant rice. Appl Ecol Environ Res. 16(6):7741–7758. doi:10.15666/aeer/1606_77417758.
- Deng Y, Xu L, Zeng X, Li Z, Qin B, He N. 2010. New perspective of GABA as an inhibitor of formation of advanced lipoxidation end-products: it’s interaction with malondiadehyde. J Biomed Nanotechnol. 6:318–324. doi:10.1166/jbn.2010.1130.
- Fait A, Fromm H, Walter D, Galili G, Fernie AR. 2008. Highway or byway: the metabolic role of the GABA shunt in plants. Trends Plant Sci. 13:14–19. doi:10.1016/j.tplants.2007.10.005.
- Ghosh P, Roychoudhury A. 2018. Differential levels of metabolites and enzymes related to aroma formation in aromatic indica rice varieties: comparison with non-aromatic varieties. 3 Biotech. 8:25. doi:10.1007/s13205-017-1045-6.
- Giraud G. 2013. The world market of fragrant rice, main issues and perspectives. Inter Food Agribus Manag Rev. 16:1–20. doi:10.22004/ag.econ.148577.
- Hashemi FG, Rafii MY, Ismail MR, Mahmud TMM, Rahim HA, Asfaliza R, Malek MA, Latif MA. 2013. Biochemical, genetic and molecular advances of fragrance characteristics in rice. Crit Rev Plant Sci. 32:445–457. doi:10.1080/07352689.2013.807716.
- Huang ZL, Tang XR, Wang YL, Chen MJ, Zhao ZK, Duan MY, Pan SG. 2012. Effects of increasing aroma cultivation on aroma and grain yield of aromatic rice and their mechanism. Scientia Agricultura Sinica. 45:1054–1065 (in Chinese with English abstract). doi:10.3864/j.issn.0578-1752.2012.06.003.
- Kaikavoosi K, Kad TD, Zanan RL, Nadaf AB. 2015. 2-Acetyl-1-pyrroline augmentation in scented indica rice (Oryza sativa L.) varieties through Δ 1-pyrroline-5-carboxylate synthetase (P5CS) gene transformation. Appl Biochem Biotechnol. 177:1466–1479. doi:10.1007/s12010-015-1827-4.
- Kong L, Ashraf U, Cheng S, Rao G, Mo Z, Tian H, Pan S, Tang X. 2017. Short-term water management at early filling stage improves early-season rice performance under high temperature stress in South China. Eur J Agron. 90:117–126. doi:10.1016/j.eja.2017.07.006.
- Li M, Ashraf U, Tian H, Mo Z, Pan S, Anjum SA, Duan M, Tang X. 2016b. Manganese-induced regulations in growth, yield formation, quality characters, rice aroma and enzyme involved in 2-acetyl-1-pyrroline biosynthesis in fragrant rice. Plant Physiol Biochem. 103:167–175. doi:10.1016/j.plaphy.2016.03.009.
- Li Y, Fan Y, Ma Y, Zhang Z, Yue H, Wang L, Li J, Jiao Y. 2017. Effects of exogenous γ-aminobutyric acid (GABA) on photosynthesis and antioxidant system in pepper (Capsicum annuum L.) seedlings under low light stress. J Plant Growth Regul. 36:436–449. doi:10.1007/s00344-016-9652-8.
- Li MF, Guo SJ, Yang XH, Meng QW, Wei XJ. 2016a. Exogenous gamma-aminobutyric acid increases salt tolerance of wheat by improving photosynthesis and enhancing activities of antioxidant enzymes. Biol Plantarum. 60:123–131. doi:10.1007/s10535-015-0559-1.
- Li S, Jiang H, Wang J, Wang Y, Pan S, Tian H, Duan M, Wang S, Tang X, Mo Z. 2019a. Responses of plant growth, physiological, gas exchange parameters of super and non-super rice to rhizosphere temperature at the tillering stage. Sci Rep. 9:10618. doi:10.1038/s41598-019-47031-9.
- Li Y, Lai R, Li W, Liu J, Huang M, Tang Y, Tang X, Pan S, Duan M, Tian H, et al. 2019b. γ-Aminobutyric acid regulates grain yield formation in different fragrant rice genotypes under different nitrogen levels. J Plant Growth Regul. 38:1–13. doi:10.1007/s00344-019-10016-z doi: 10.1007/s00344-018-9799-6
- Li J, Tian Z, Wu X, Lv G, Ma W, Zhang Y, Gao H. 2018. Gamma-aminobutyric acid (GABA) modulates nitrate concentrations and metabolism in the leaves of Pakchoi (Brassica campestris ssp. chinensis Makino) treated with a nitrogen-rich solution. Plant Mol Biol Rep. 36:530–542. doi:10.1007/s11105-018-1092-0.
- Luo HY, Gao HB, Xia QP, Gong BB, Wu XL. 2011. Effects of exogenous GABA on reactive oxygen species metabolism and chlorophyll fluorescence parameters in tomato under NaCl stress. Scientia Agricultura Sinica. 44:753–761 (in Chinese with English abstract). doi:10.3864/j.issn.0578-1752.2011.04.013.
- Ma X, Zhu C, Yang N, Gan L, Xia K. 2016. γ-Aminobutyric acid addition alleviates ammonium toxicity by limiting ammonium accumulation in rice (Oryza sativa) seedlings. Physiol Plant. 158:389–401. doi:10.1111/ppl.12473.
- Mahajan G, Sekhon NK, Singh N, Kaur R, Sidhu AS. 2010. Yield and nitrogen-use efficiency of aromatic rice cultivars in response to nitrogen fertilizer. J New Seeds. 11:356–368. doi:10.1080/1522886X.2010.520145.
- Malekzadeh P, Khara J, Heydari R. 2014. Alleviating effects of exogenous Gamma-aminobutiric acid on tomato seedling under chilling stress. Physiol Mol Biol Plants. 20:133–137. doi:10.1007/s12298-013-0203-5.
- Maraval I, Sen K, Agrebi A, Menut C, Morere A, Boulanger R, Gay F, Mestres C, Gunata Z. 2010. Quantification of 2-acetyl-1-pyrroline in rice by stable isotope dilution assay through headspace solid-phase microextraction coupled to gas chromatography–tandem mass spectrometry. Anal Chim Acta. 675:148–155. doi:10.1016/j.aca.2010.07.024.
- Miller G, Honig A, Stein H, Suzuki N, Mittler R, Zilberstein A. 2009. Unraveling Δ1-pyrroline-5-carboxylate-proline cycle in plants by uncoupled expression of proline oxidation enzymes. J Biol Chem. 284:26482–26492. doi:10.1074/jbc.M109.009340.
- Mo Z, Fan P, Pan S, Wang Z, Tian H, Duan M, Tang X. 2016a. Effect of fertilizer types and fertilization methods on 2-acetyl-1-pyrroline content in aromatic rice. Acta Agriculturae Boreali-Sinica. 31:152–158 (in Chinese with English abstract). doi:10.7668/hbnxb.2016.05.023.
- Mo Z, Huang J, Xiao D, Ashraf U, Duan M, Pan S, Tian H, Xiao L, Zhong K, Tang X. 2016b. Supplementation of 2-Ap, Zn and La improves 2-acetyl-1-pyrroline concentrations in detached aromatic rice panicles in vitro. PLoS ONE. 11:e0149523. doi:10.1371/journ al.pone.0149523 doi: 10.1371/journal.pone.0149523
- Mo Z, Lei S, Ashraf U, Khan I, Li Y, Pan S, Duan M, Tian H, Tang X. 2017. Silicon fertilization modulates 2-acetyl-1-pyrroline content, yield formation and grain quality of aromatic rice. J Cereal Sci. 75:17–24. doi:10.1016/j.jcs.2017.03.014.
- Mo Z, Li Y, Nie J, He L, Pan S, Duan M, Tian H, Xiao L, Zhong K, Tang X. 2019a. Nitrogen application and different water regimes at booting stage improved yield and 2-acetyl-1-pyrroline (2AP) formation in fragrant rice. Rice. 12:74. doi:10.1186/s12284-019-0335-5 doi: 10.1186/s12284-019-0328-4
- Mo Z, Li W, Pan S, Fitzgerald TL, Xiao F, Tang Y, Wang Y, Duan M, Tian H, Tang X. 2015. Shading during the grain filling period increases 2-acetyl-1-pyrroline content in fragrant rice. Rice. 8:9. doi:10.1186/s12284-015-0040-y.
- Mo Z, Tang Y, Ashraf U, Pan S, Duan M, Tian H, Wang S, Tang X. 2019b. Regulations in 2-acetyl-1-pyrroline contents in fragrant rice are associated with water-nitrogen dynamics and plant nutrient contents. J Cereal Sci. 88:96–102. doi:10.1016/j.jcs.2019.05.013.
- Naliwajski MR, Skłodowska M. 2014. Proline and its metabolism enzymes in cucumber cell cultures during acclimation to salinity. Protoplasma. 251:201–209. doi:10.1007/s00709-013-0538-3.
- Ncube B, Finnie JF, Van Staden J. 2013. Dissecting the stress metabolic alterations in in vitro Cyrtanthus regenerants. Plant Physiol Biochem. 65:102–110. doi:10.1016/j.plaphy.2013.01.001.
- Pan S, Rasul F, Li W, Tian H, Mo Z, Duan M, Tang X. 2013. Roles of plant growth regulators on yield, grain qualities and antioxidant enzyme activities in super hybrid rice (Oryza sativa L.). Rice. 6:9. doi:10.1186/1939-8433-6-9.
- Poonlaphdecha J, Maraval I, Roques S, Audebert A, Boulanger R, Bry X, Gunata Z. 2012. Effect of timing and duration of salt treatment during growth of a fragrant rice variety on yield and 2-acetyl-1-pyrroline, proline, and GABA levels. J Agric Food Chem. 60:3824–3830. doi:10.1021/jf205130y.
- Priya M, Sharma L, Kaur R, Bindumadhava H, Nair RM, Siddique KHM, Nayyar H. 2019. GABA (γ-aminobutyric acid), as a thermo-protectant, to improve the reproductive function of heat-stressed mungbean plants. Sci Rep. 9:7788. doi:10.1038/s41598-019-44163-w.
- Ramesh SA, Tyerman SD, Gilliham M, Xu B. 2017. γ-Aminobutyric acid (GABA) signalling in plants. Cell Mol Life Sci. 74:1577–1603. doi:10.1007/s00018-016-2415-7.
- Ren Y, Ashraf U, He LX, Mo ZW, Wang F, Wan XC, Kong H, Ran XY, Tang XR. 2017. Irrigation and nitrogen management practices affect grain yield and 2-acetyl-1-pyrroline content in aromatic rice. Appl Ecol Environ Res. 15:1447–1460. doi:10.15666/aeer/1504_14471460.
- Rezaei-Chiyaneh E, Seyyedi SM, Ebrahimian E, Moghaddam SS, Damalas CA. 2018. Exogenous application of gamma-aminobutyric acid (GABA) alleviates the effect of water deficit stress in black cumin (Nigella sativa L.). Ind Crops Prod. 112:741–748. doi:10.1016/j.indcrop.2017.12.067.
- Routray W, Rayaguru K. 2018. 2-Acetyl-1-pyrroline: a key aroma component of aromatic rice and other food products. Food Rev Int. 34:539–565. doi:10.1080/87559129.2017.1347672.
- Salvatierra A, Pimentel P, Almada R, Hinrichsen P. 2016. Exogenous GABA application transiently improves the tolerance to root hypoxia on a sensitive genotype of Prunus rootstock. Environ Exp Bot. 125:52–66. doi:10.1016/j.envexpbot.2016.01.009.
- Sánchez E, Ruiz JM, Romero L. 2002. Proline metabolism in response to nitrogen toxicity in fruit of French Bean plants (Phaseolus vulgaris L. cv Strike). Sci Hortic. 93:225–233. doi:10.1016/S0304-4238(01)00342-9.
- Sansenya S, Hua Y, Chumanee S. 2018. The correlation between 2-acetyl-1-pyrroline content, biological compounds and molecular characterization to the aroma intensities of Thai local rice. J Oleo Sci. 67:893–904. doi:10.5650/jos.ess17238.
- Shang H, Cao S, Yang Z, Cai Y, Zheng Y. 2011. Effect of exogenous γ-aminobutyric acid treatment on proline accumulation and chilling injury in peach fruit after long-term cold storage. J Agric Food Chem. 59:1264–1268. doi:10.1021/jf104424z.
- Song H, Xu X, Wang H, Wang H, Tao Y. 2010. Exogenous γ-aminobutyric acid alleviates oxidative damage caused by aluminium and proton stresses on barley seedlings. J Sci Food Agric. 90:1410–1416. doi:10.1002/jsfa.3951.
- Su G, An Z, Zhang W, Liu Y. 2005. Light promotes the synthesis of lignin through the production of H2O2 mediated by diamine oxidases in soybean hypocotyls. J Plant Physiol. 162:1297–1303. doi:10.1016/j.jplph.2005.04.033.
- Tang X, Wu M. 2006. Effects of application of Zinc, Iron and Lanthanum on contents of aroma in brown rice and proline in flag leaf of aromatic rice. Hybrid Rice. 21:69–72 (in Chinese with English abstract). doi:10.16267/j.cnki.1005-3956.2006.06.028.
- Tripathi BN, Singh V, Ezaki B, Sharma V, Gaur JP. 2013. Mechanism of Cu-and Cd-induced proline hyperaccumulation in Triticum aestivum (wheat). J Plant Growth Regul. 32:799–808. doi:10.1007/s00344-013-9343-7.
- Umair S, Leung YM, Bland RJ, Simpson HV. 2011. Enzymes of the ornithine–glutamate–proline pathway in the sheep abomasal nematode parasites Haemonchus contortus and Teladorsagia circumcincta. Exp Parasitol. 129:115–119. doi:10.1016/j.exppara.2011.07.006.
- Wang P, Tang X, Tian H, Pan S, Duan M, Nie J, Luo Y, Xiao L. 2013. Effects of different irrigation modes on aroma content of aromatic rice at booting stage. Guangdong Agric Sci. 8:1–3. (in Chinese with English abstract).
- Xie W, Ashraf U, Zhong D, Lin R, Xian P, Zhao T, Feng H, Wang S, Duan M, Tang X, et al. 2019. Application of γ-aminobutyric acid (GABA) and nitrogen regulates aroma biochemistry in fragrant rice. Food Sci Nutr. 7:3784–3796. doi:10.1002/fsn3.1240.
- Xie W, Kong L, Ma L, Ashraf U, Pan S, Duan M, Tian H, Wu L, Tang X, Mo Z. 2020. Enhancement of 2-acetyl-1-pyrroline (2AP) concentration, total yield, and quality in fragrant rice through exogenous γ-aminobutyric acid (GABA) application. J Cereal Sci. 91:102900. doi:10.1016/j.jcs.2019.102900.
- Yu GH, Sun MX. 2007. Deciphering the possible mechanism of GABA in tobacco pollen tube growth and guidance. Plant Signal Behav. 2:393–395. doi:10.4161/psb.2.5.4265.
- Zhao D, Pu X, Zeng Y, Li B, Du J, Yang S. 2009. Determination of γ-aminobutyric acid in barley. J Triticeae Crops. 29:69–72 (in Chinese with English abstract).