ABSTRACT
Plants can be severely affected by heat stress due to rapid environmental changes. Use of endophytic fungi is a new tool to protect crops from the environmental stresses. Here we claim a potent endophyte isolated from Sonchus asper L. The aim was to explore the stress adaptive mechanism of sunflower and soybean mediated by Aspergillus niger (SonchL-7) under high temperature. Inoculation with A. niger boosted plant height, biomass and chlorophyl contents, while significantly curtailed the concentration of lipid peroxidation and reactive oxygen species (ROS) under thermal stress at 40°C. Moreover, the ROS-scavenging activities, like ascorbic acid oxidase (AAO), catalase (CAT), glutathione reductase (GR), superoxide dismutase (SOD) and peroxidase (POD) were augmented. Also, proline and phenolics were enhanced in the tested crops, while ABA concentration was significantly reduced. These positive results suggested that A. niger can be used as a heat-stress ameliorative tool for crops in the future.
Introduction
Thermal stress is a persistent abiotic stress in the present age, causing severe loss to agricultural crops (Ismail et al. Citation2018; Ismail et al. Citation2019). Rising in average global temperature also results in drought and saline conditions by speed up vaporization from the soil surface. Being sessile in nature, plants are exposed to stresses (abiotic and biotic) leading to an enlarged accumulation of reactive oxygen species (ROS). The ROS cause oxidative damages to the chlorophylls, macro-molecules, and disturb cell functional-integrity resulting in premature cell death and apoptosis (Ashraf and Harris Citation2004). These ROS species are defused by local antioxidants, located in biological membranes except H2O2 (Abd_Allah et al. Citation2018). To avoid oxidative damages, all plants have self-activated-defense-mechanisms (SADMs) like compatible osmolytes and antioxidant systems (Mhamdi et al. Citation2010). Antioxidant systems work in synchronization in order to detoxify reactive oxygen species.
Plant hormones can be worked as signaling molecules during various stresses (Hamayun et al. Citation2015; Hamayun et al. Citation2017; Bilal et al. Citation2018; Khan et al. Citation2018; Gul Jan et al. Citation2019; Jan et al. Citation2019). Abscisic acid (ABA) is known to be the foremost hormone respond to lack of water and heat stress by regulating stomatal opening and closing (Yoon et al. Citation2009). High atmospheric humidity, low concentration of CO2 and light cause stomatal opening while, dry air, high concentration of CO2, darkness and stress-induced ABA result in closing of stomata (Lorenzo et al. Citation2004). It also plays significant role in seed germination, maturation and senescence of vascular plants, including Arabidopsis thaliana (Waqas et al. Citation2012).
Proline and phenolics are accumulated in higher plants during abiotic stresses and function as osmolytes, protein and membrane stabilizers, buffer of cellular redox reactions and as ROS foragers (Khushdil et al. Citation2019; Muhammad et al. Citation2019; Nusrat et al. Citation2019). Moreover, proline also helps in balancing the ratio of NADP+/NADPH necessary for cellular metabolism, maintenance of cellular-acidosis and protein-compatible-hydrotrope. Proline breaks down after stress that generates huge amount of reducing agents used for ATP synthesis in mitochondrial oxidative phosphorylation. ATPs are then used in stress retrieval and refurbishing of damages (Ashraf and Foolad Citation2007). Phenolics are known to function as herbivore restriction, antioxidants and attract plant pollinating insects (Rodriguez et al. Citation2009).
Endophytes are endosymbiotic partners in majority of plants, which reside inside the plant tissues permanently or for the time being with no visible sign of harm (Mehmood et al. Citation2019a, Citation2019b). They are branded to have a role in the refurbishment of restraint plant growth under various abiotic and biotic stresses by providing resistance, reduce ailment severity, accelerate mineral absorption and improve biomass synthesis of their host plant (Mehmood et al. Citation2019a, Citation2019b). Plants without hosting endophytic fungi are more prone to environmental constraints, like variation in temperatures, salinity and drought (Ismail et al. Citation2019; Kang et al. Citation2019; Khushdil et al. Citation2019). Aspergillus species were formerly known to have the capacity to tolerate arsenic toxicity by modulating the activities of SOD, CAT, GR and the contents of malondialdehyde, proline and thiol (Ismail et al. Citation2018; Ismail et al. Citation2019). The present work was an attempt to investigate the stress adaptive tools for sunflower and soybean arbitrated by endophytic fungus A. niger under heat stress.
Materials and methods
Rice (Oryza sativa) cultivar Fakhr-e-Malakand was cultivated in pots having 30 ml of 0.8% (w/v) water-agar medium. The pots were placed in a growth chamber with day/night cycle of 14 h at 28 ± 0.3°C and 10 h at 25 ± 0.3°C. The relative humidity of the growth chamber was set at 70%. We used rice seedlings (a quick replier) for preliminary screening to see the effect of endophytic fungi (Misra and Dwivedi Citation2004). The potent species were separated and applied to the sunflower and soybean. Sunflower and soybean were chosen as these are the two best oil producing crops grown worldwide, facing heat stress nowadays. Soybean (Swat 84) and sunflower (ICI Hyson 33 Australia) varieties were grown in autoclaved sand for two weeks in growth chambers set at 25°C and 40°C. The setting of the growth chamber was same as mentioned earlier. All experiments were performed in three biological replicates.
Fungal isolation
Endophytes were isolated from the Sonchus asper in District Nowshera Khyber Pakhtunkhwa, Pakistan using standardized protocol of Benizri et al. (Citation1998). S. asper is a wild plant that has the ability to stand the hot summer of the area, therefore, it was explored to isolate potent endophytic fungi for the amelioration of heat stress. After isolation on Hagem media, endophytic fungi were purified on potato dextrose agar (PDA) plates. Fungal isolates were inoculated on Czapek media in a shaking incubator and their biomass was collected after 7 days of incubation.
Isolation of fungal DNA
Isolation of A. niger DNA was carried according to an established protocol of Khan et al. (Citation2008). The mycelium (200 mg) of A. niger was suspended in micro-centrifuge tube containing 500 μl of a bead beating solution and 5% of sodium dodecyl sulfate. The tube was vortexed and centrifuged for 10 min at 11,000 g and 4°C. The supernatant was transferred to a new tube and equal volumes of phenol: chloroform: isoamyl alcohol (25:24:1) was added to it. The sample was vortexed and centrifuged for 5 min. Again, the collected supernatant was transferred to a new tube and added an equal volume of chloroform: isoamyl alcohol (24:1). The tube was then centrifuged for 5 min at 10,000 g. The supernatant was transferred to a new eppendorf tube, and 2.5 volumes of isopropanol were added to precipitate DNA. The tube was incubated in a refrigerator for 1 h, and centrifuged for 10 min at 14,000 g. The pellet was washed twice with cold 70% ethanol, air-dried and added 40 µl TE buffer. The purity of the extracted DNA and its quantity was measured by Thermo Scientific Nano Drop spectrophotometer at 260 nm (Chen and Kuo Citation1993). The experiment was done in triplicate.
Identification of potent species
Fungal isolate was identified by following the method of Khan et al. (Citation2008) with some modifications. 18S rRNA was amplified by using primers ITS1 (5′-TCC GTA GGT GAA CCT GCG G-3′) and ITS4 (5′-TCC TCC GCT TAT TGA TAT GC-3′). The sequence attained, was then subjected to BLASTn1 for sequence homology approximation. Neighbor Joining (NJ) tree was constructed for the phylogenetic analysis using MEGA 7 software.
Preliminary screening of isolated fungus
Preliminary screening was carried out on rice seedlings at two leaf stage. A 100 µl of fungal culture filtrate was applied on the tip of rice seedlings grown in water-agar medium (0.8%) in a growth-chamber (operated with settings as mentioned earlier) for one week. Growth attributes, including total chlorophyl content, root and shoot length, fresh and dry weight of root and shoot, were measured and compared with distilled water- and Czapek broth-treated controlled plants (Warrier et al. Citation2013).
Inoculation of sunflower and soybean with A. niger
Fresh biomass of A. niger was grown in a 250 ml conical flask with 50 ml of Czapek broth and transferred to shaking-incubator set at 28°C for 1 week at 120 rpm. Filter paper was used to separate the fungal biomass from the Czapek broth. One mg fresh endophytic fungi bio-mass per 100 g of autoclaved sand, was applied to pot comprising H. annuus (variety Hysun-33) and G. max (variety Swat-84) seeds (9 seeds/pot), then transferred for 14 days into growth chambers fixed at normal temperature at 25°C and high temperature at 40°C. Ten milliliter Hoagland Solution (half strength) was given to plants at two days interval. Growth attributes were assessed after 14 days of cultivation in growth chambers (Misra and Dwivedi Citation2004). The chlorophyl contents of wheat leaves were quantified by chlorophyl meter (Spad-502 plus, Japan). The experiment was accomplished in triplicates.
Determination of antioxidants
The activity of catalase (CAT, EC 1.11.1.6) in the seedlings of H. annuus and G. max was determined using Luck (Citation1974) protocol. Fresh leaves of sunflower and soybean (2 g each) were ground in 10 ml of phosphate buffer (phosphate buffer consisted of NaCl = 8 g/L, KCl = 0.2 g/L, Na2HPO4 = 1.44 g/L and KH2PO4 = 0.24 g/L) and then spun at 10,000 rpm for 5 min. About, 40 µl of supernatant was mixed with 3 ml of H2O2-phosphate buffer. Optical density was measured at a wavelength of 240 nm. H3PO4 buffer was used as blank. The quantity of enzyme needed to lower OD by 0.05 per gram of plant biomass at 240 nm was considered as one unit. Kar and Mishra (Citation1976) method was used for the activity of peroxidase (POD, EC 1.11.1.7). The mixture contained H2O2 (50 μmoles), phosphate buffer (125 μmoles pH 6.8), pyrogallol (50 μmoles) and 1 ml of the 20X diluted enzyme extract in a final volume of 5 ml. The amount of purpurogallin developed was measured at 420 nm and the concentration of POD was calculated as EU mg−1 protein. Oberbacher and Vines (Citation1963) method was used for the estimation of ascorbate oxidase (AAO, EC 1.10.3.3) activity in soybean and sunflower seedlings. Fresh leaves (0.1 g) of soybean and sunflower were ground in phosphate buffer (2 ml) and centrifuged for five minutes at 3000 g. Approximately, 3 ml of the substrate solution (8.8 mg of ascorbic acid in 300 ml phosphate buffer, pH 5.6) was mixed with 100 µl of supernatant. The OD265 was finally observed at an interval of 30 s for 5 min. One unit of AAO was calculated by a decrease in OD265 of 0.05 per minute. Beyer and Fridovich (Citation1987) protocol was adopted for the analysis of superoxide dismutase (SOD, EC 1.15.1.1) activity by measuring decrease in the absorbance of nitroblue tetrazolium (NBT). The amount of SOD was determined as enzyme unit (EU) mg−1 protein and 1 unit of enzyme was defined as the amount of protein causing a 50% inhibition of NBT reduction. Carlberg and Mannervik (Citation1985) method was applied for the activity of glutathione reductase (GR, EC 1.6.4.2). The GR activity is assayed as NADPH dependent reduction of oxidized glutathione (GSSG), which is followed as the decrease in absorbance at 340 nm for 2 min. The 0.8-ml reaction mixture contains 0.125 mM NADPH, 1 mM GSSG, 1 mM EDTA in 100 mM potassium phosphate buffer, pH 7.0. The amount of GR was measured by applying extinction co-efficient of 0.12 mM NADPH, which was 6.2 mM−1 cm−1, and was shown as EU mg−l protein.
Determination of ABA
Methodology of Yoon et al. (Citation2009) was used to investigate ABA concentration in H. annuus and G. max. seedlings. Soybean and sunfloweŕs fresh leaves (0.5 g) were crushed in liquid N2 and mixed with 2 ml of glacial acetic acid (GAA, 28.5 ml) and isopropanol (1.5 ml) mixture. Then via rotary evaporator, the mixture was filtered and dehydrated. Then we added diazomethane to this mixture and examined via GC MS SIM (6890N setup GC Scheme furnished with 5973 System Mass Selective Detector; Agilent Technologies, Palo Alto, CA, USA). The Lab Base, Thermo Quset, Manchester, UK, Data System Software (DSS) was used to monitor retorts to ions with m/z standards of 190 and 162 for Me-ABA and 194 and 166 for Me-[2H6]-ABA. ABA ([2H6]-ABA) was applied as standard.
Determination of phenolics and proline
Methodology of Cai et al. (Citation2004) was applied to investigate total phenolics in H. annuus and G. max. Sample (0.2 ml) was mixed with Folin–Ciocalteu reagent (0.5 N) and kept for 4 min at 25°C. Sodium carbonate (75 g/L) was added to the mixture for neutralization and heated at 100°C for 1 min. This mixture was then kept in the dark for 2 h. Optical density was taken at 650 nm. Different concentrations of gallic acid (Sigma Aldrich; 100, 200, 300, 500, 600, 700, and 900 mg/ml) were made to draw a standard curve. Bates et al. (Citation1973) procedure was used with minor modifications, to analyze total proline. Fresh leaves (0.1 g) of G. max and H. annuus were grinded in 4 ml sulpho-salicylic acid (3%) and incubated at 5°C for 24 h. The mixture was then centrifuged at 3000 rpm for 5 min. Then 2 ml supernatant was mixed with 2 ml of acid ninhydrin and heated at 100°C for 1 h. Toluene (4 ml) was added to the mixture and OD was taken at 520 nm. Various concentrations of proline (Sigma Aldrich; 2, 4, 6, 8, and 10 μg/ml) were used to plot standard curve. Optical density was noted at 520 nm.
Determination of Primary metabolites
Proteins were determined in H. annuus and G. max seedlings using methodology of Lowry et al. (Citation1951). Homogenate of filtered leaf sample (0.1 ml) was mixed with 0.1 ml of 2M NaOH. The mixture was heated at 100 °C to hydrolyse. The resulted hydrolysate was cooled and mixed with 1 ml of complex-forming solution [complex-forming solution = 2% (w/v) Na2CO3 in distilled H2O, 1% (w/v) CuSO4.5 H2O in distilled H2O, 2% (w/v) sodium potassium tartrate in distilled H2O] and let the mixture stand for 10 min at room temperature. About, 1 ml of Folin reagent was added and the mixture was vortexed. The mixture was allowed to stand for 30 min at room temperature. The absorbance was finally read at 650 nm using spectrophotometer. Different concentrations of bovine serum albumin (BSA) (Sigma Aldrich; 20, 40, 60, 80, and 100 µg/ml) was applied to draw a standard curve. Van Handel (Citation1985) methodology was followed for the analysis of total lipids. Crushed leaf sample was centrifuged and about 1 ml of the supernatant was taken and transferred to the reaction tube containing 1 ml chloroform/methanol (1:2 v/v) solution. The mixture was heated in a water bath at 90°C until complete evaporation. Concentrated sulfuric acid (2 ml) was added to the precipitate formed and mixed with the help of vortex for 2 min and then heated on a water bath at 90°C. The mixture was cooled and added 5 ml vanillin-phosphoric acid reagent. The tube containing mixture was allowed to stand for 30 min at room temperature for color development. Finally the tube was mixed and the absorbance was measured at 525 nm. Various concentrations of pure canola oil (10, 40, 70, 100, 130, and 160 µg/ml) was used to draw a standard curve. Mohammadkhani and Heidari (Citation2008) protocol was applied to determine soluble sugar in H. annuus and G. max leaves. Homogenate of filtered leaf sample (1 ml) was mixed with 10 µl of 5% phenol and 1 ml of 98% sulfuric acid. The mixture was vortexed and allowed to stand for 1 h. The absorbance was finally measured at 485 nm by using spectrophotometer. Different concentrations of glucose (Sigma Aldrich; 20, 40, 60, 80, and 100 µg/ml) were taken to plot a standard curve.
Statistical analysis
Experiments were accomplished in triplicates. ANOVA was applied to analyze the data. Means of all values were equated by DMRT (Duncan Multiple Range Test) at p < 0.05, via SPSS-20 (SPSS Inch., Chicago, IL, USA).
Results
Fungal isolation and their screening on Oryza sativa seedlings
A total of ten different endophytic fungi were isolated from S. asper that was initially screened on rice seedlings for their growth promoting potential. SonchL-7-treated rice seedlings have more chlorophyl content (4.9%), shoot (11.7%) and root length (21.9%), fresh shoot (11.9%) and root weight (61.8%) and, dry shoot (30.5%) and root (15.7%) weight as compared to Czapek-treated control seedlings ().
Table 1. Effect of A. niger filtrate on the growth of rice seedlings.
Molecular identification of isolated fungus
Nucleotide sequence of ITS region of SonchL-7 isolate was compared with allied strains by applying BLAST search program. 18S rRNA sequence revealed maximum resemblance (49%) with A. niger. Phylogenetic consensus tree was constructed from 12 taxa (11 reference and 1 clone) by neighbor joining (NJ) method using MEGA 7 software (). Our endophyte SonchL-7 formed a clad with A. niger reinforced by 49% bootstrap value in the harmony tree. Sequence homology and phylogenetic analysis confirmed that A. niger was our isolate. ITS sequence of 18S rRNA was submitted to GenBank database under accession No. MH577053.
Growth features of sunflower and soybean co-cultured with A. Niger
Growth attributes, including total chlorophyl content, root and shoot length, fresh and dry weight of root and shoot of experimental plants (A. niger-co-cultured) grown for two weeks, at 25°C and 40°C were measured. A significant increase in most of the growth attributes were found in sunflower and soybean seedlings inoculated with A. niger as compared to A. niger-free seedlings at 25°C and 40°C. A. niger-associated sunflower has enhanced chlorophyl (5.9%), shoot length (17%), root length (35.5%), fresh shoot (2.8%) and root weights (23.5%) and shoot (30%) and root dry weights (50%), when exposed to thermal stress as compared to the control seedlings (). Similarly, the increase was also recorded in the chlorophyl content (9.9%), shoot (32.3%) and root length (33%), fresh shoot (47.6%) and fresh root weight (23%), dry shoot (5%) and dry root weight (16.7%) of A. niger-inoculated soybean at heat stress as compared to control plants ().
Table 2. Effect of A. niger on the growth of sunflower seedlings.
Table 3. Effect of A. niger on the growth of soybean seedlings.
Analysis of physiological features
We found an enhanced level of H2O2 and lipid peroxidation in endophyte-free sunflower and soybean seedlings, subjected to heat stress (i.e. 40°C). High concentration of H2O2 is known to have negative impact on membrane integrity. Quantification of lipid peroxidation was measured in terms of malondialdehyde (MDA) production. During normal temperature (25°C), A. niger reduced H2O2 levels in sunflower and soybean by 21% and 67% respectively while, at thermal stress (40°C) this decrease was 45.8% and 37% respectively. A significant decrease was also found in the amount of MDA in A. niger-inoculated sunflower and soybean. At 25°C this decrease in the amount of MDA was equal (61%) in the sunflower and soybean seedlings, while at 40°C the decrease was more in sunflower (49%) than in soybean (30%) seedlings ().
Figure 2. (A) Hydrogen peroxide (H2O2) and (B) lipid peroxidation (MDA) B in soybean or sunflower seedlings grown at 25°C and 40°C inoculated with and without A. niger. Data represent mean of biological triplicates. Similar bars labeled with different letters are significantly different (p < 0.05) as estimated by Duncan’s Multiple Range Test (DMRT).
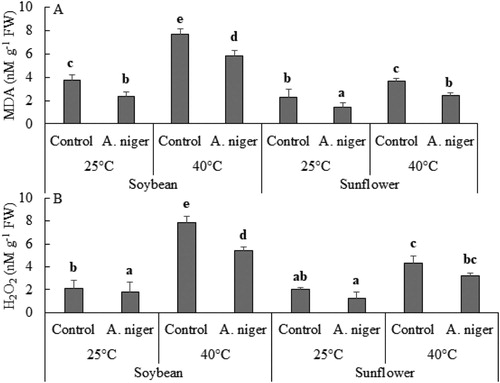
Soybean and sunflower both showed an enhanced level of proline and phenolic contents under heat stress when co-cultured with A. niger as compared to control plants. Maximum increase (61%) in proline content was noted in the seedlings of sunflower whereas, the fungal strain was less responsive in soybean seedlings in terms of increase in proline content and the increase was 26% as compared to inoculated sunflower plants. In contrast the total phenolics content was significantly augmented (34%) in the seedlings of soybean as compared to sunflower (14%) ().
Figure 3. (A) Total proline and (B) phenolics in soybean or sunflower seedlings grown at 25°C and 40°C inoculated with and without A. niger. Data represent mean of biological triplicates. Similar bars labeled with different letters are significantly different (p < 0.05) as estimated by Duncan’s Multiple Range Test (DMRT).
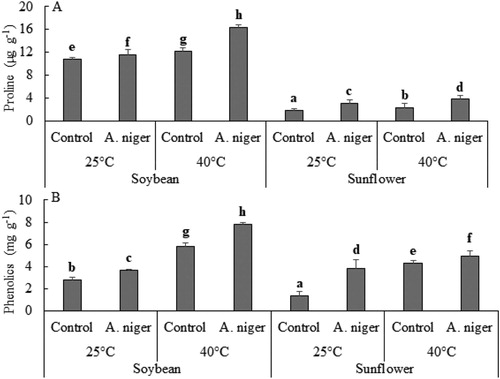
A significant increase was detected in the activities of antioxidants including CAT, POD, AAO, SOD and GR in A. niger-inoculated sunflower and soybean at 40°C as compared to endophyte-free seedlings. A. niger-inoculated soybean seedlings have enhanced concentrations of CAT (32%), POD (58%), AAO (18.9%), SOD (24.8%) and GR (18.9%) and sunflower has CAT (34.6%) POD (64%), AAO (3%), SOD (27.8%) and GR (42.8%) as compared to endophyte-free seedlings ().
Figure 4. Activity of (A) CAT; (B) POD; (C) AAO; (D) SOD and (E) GSH in soybean or sunflower seedlings grown at 25°C and 40°C inoculated with and without A. niger. Data represent mean of biological triplicates. Similar bars labeled with different letters are significantly different (p < 0.05) as estimated by Duncan’s Multiple Range Test (DMRT).
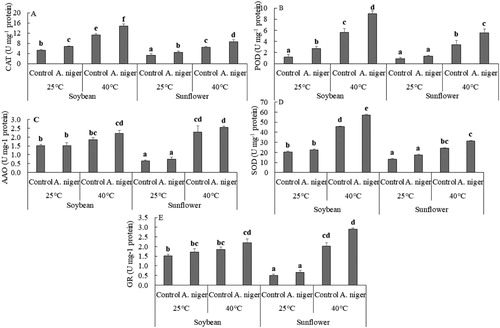
A decrease was also noted in the amount of ABA, in the leaves of endophyte-inoculated soybean and sunflower seedlings at normal as well as at high temperature (40°C) as compared to non-inoculated plants. Soybean seedlings co-cultured with A. niger has 15% lesser ABA as compared to control plants at room temperature while, at 40°C, endophyte-inoculated soybean has 18% lower of ABA content as compared to control plants. Similarly, A. niger-inoculated sunflower has 7% lesser ABA content at 25°C while, 17% lesser ABA content at 40°C as compared to endophyte-free plants ().
Figure 5. GC MS analysis of ABA content of soybean or sunflower seedlings grown at 25°C and 40°C inoculated with and without A. niger. Data represent mean of biological triplicates. Similar bars labeled with different letters are significantly different (p < 0.05) as estimated by Duncan’s Multiple Range Test (DMRT).
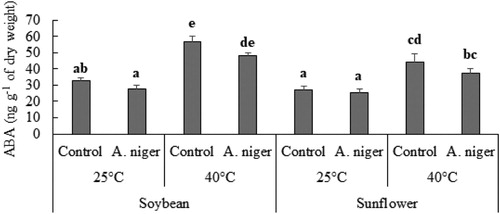
Total lipids, proteins and soluble sugars of sunflower and soybean were determined via spectrophotometer. We noticed an increase of 21% and 23% in the total lipid contents of A. niger-inoculated soybean and sunflower respectively, at 25°C as compared to uninoculated plants. At 40°C, A. niger-associated soybean (48%) and sunflower (39%) also has more lipids as compared to control seedlings (A). Whereas, endophyte-inoculated soybean has 51% and sunflower has 35% more protein at 40°C while, at 25°C soybean has36% and sunflower has 44% more proteins as compared to endophyte-free plants (B). At 40°C, A. niger-aligned soybean has 11% and sunflower has 29% more soluble sugars while, at 25°C soybean has 11% and sunflower has 15% more soluble sugars as compared to control plants (C).
Figure 6. Analysis of total lipids (A), proteins (B) and soluble sugars (C) of soybean or sunflower seedlings grown at 25°C and 40°C inoculated with and without A. niger. Data represent mean of biological triplicates. Similar bars labeled with different letters are significantly different (p < 0.05) as estimated by Duncan’s Multiple Range Test (DMRT).
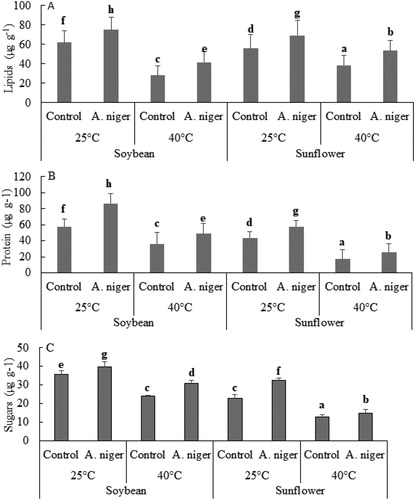
Discussion
Higher plants, subjected to heat stress, are known to pledge some intricate biosynthetic responses like antioxidant systems, adjustments in membrane lipids, osmotic potential, and synthesis of heat-shock-proteins (Rodriguez et al. Citation2004). In present study, we detected that A. niger-associated soybean and sunflower seedlings are more resistant to thermal stress than uninoculated seedlings because of improved ROS scavenging antioxidant system (including, CAT, POD, SOD, AAO, GR, proline and phenolics). Thermal stress affects plant´s biochemical, physiological and transcriptional mechanisms. High concentration of MDA in control plants indicates the amount of lipids peroxidation as a result of oxidative stress. In our study, both sunflower and soybean control seedlings exposed to heat stress, have elevated levels of MDA as compared to A. niger-associated plants. High concentrations of MDA cause membrane damaging while, endophytes including bacteria and fungi are known to reduce the impacts of abiotic stresses by reducing the level of MDA in their host plants. In a similar type of study, 27.5% decrease in the levels of MDA was found in Chickpea plants inoculated with Bacillus subtilis BERA 71 facing 200 mM NaCl salt stress (Abd_Allah et al. Citation2018). Our results confirmed the study of Abd_Allah et al. (Citation2018) on chickpea that endophytes ameliorate abiotic stresses by reducing the levels of MDA and H2O2. Furthermore, thermal stress also boosts up H2O2 in plants, which eventually affect membrane structural integrity and directs lipids peroxidation and hence more MDA synthesis as indicated in control soybean and sunflower seedlings (Ismail et al. Citation2019). We also observed high contents of chlorophyl in A. niger-inoculated sunflower and soybean at 25 °C and 40 °C as compared to their respective control seedlings, but the increase was not significantly different except for A. niger-inoculated sunflower seedlings kept at 25 °C. The increase in chlorophyl contents of A. niger-inoculated sunflower and soybean seedlings might be due the cytokinins production by the A. niger, which has been demonstrated previously by Morrison et al. (Citation2015). However, the production of cytokinins by the A. niger was not high enough to make a significant difference. Also, A. niger might help the host plants to uptake the Mg from the surroundings, thus enhanced the chlorophyl contents. Similar observations were made by Abd_Allah et al. (Citation2018). The high rate of photosynthesis is associated with high content of chlorophyl contents as well as increase in leaf area in endophyte-colonized plants as compared to endophyte-free plants (Dastogeer and Wylie Citation2017). An endophytic fungus Piriformospora indica was also found to be growth promoter of Arabidopsis by increasing chlorophyl contents and rate of photosynthesis under abiotic stress (Sherameti et al. Citation2008). Endophytes help in the maintenance of photosynthetic rate of host plant by protecting their thylakoid-membrane-proteins and chlorophyl molecules from denaturation, caused by abiotic stresses (Sun et al. Citation2010).
Phenolics are defensive compounds accumulated in higher plants against biotic and abiotic stresses (Ismail et al. Citation2018). The accumulation of high content of phenolics in endophyte-inoculated soybean and sunflower plants under thermal stress help in mitigating the accretion of ROS and lowering of stress effects on plants (Ismail et al. Citation2018). Our results are similar to the work of Abd_Allah et al. (Citation2018) that endophytes enhance phenolics content of their host plant under abiotic stress. Proline is known to function as organic osmolyte that accrue in various plants in response to different environmental stresses including high temperature. We found high content of proline in A. niger-inoculated soybean and sunflower that has encouraging effects on enzymes, membrane integrity, free radical scavenging, buffering of cellular redox potential as well as in arbitrating osmotic modification in plants facing stress conditions. Proline has role in relieving cytoplasmic acidosis, protein compatible hydrotrope and keep suitable ratio of NADP+/NADPH harmonious with metabolism (Hare and Cress Citation1997). It also help in providing stress reducing agents as a result of rapid breaking after stress. These stress reducing agents are used in repairing of stress-induced damages and generate ATP (Hare et al. Citation1998).
Our results showed the ameliorative role of endophytic fungus A. niger against heat stress, that might be due to the up-regulation of nutrient uptake and antioxidant system, and decline of ROS (Chang et al. Citation2016). The enzymatic antioxidant system of plants include CAT, POD, SOD, AAO and GR. The up-regulation of these antioxidants protect plant´s membrane from the hazardous effects of free radicals generated under various stresses (Abd_Allah et al. Citation2018). SOD is one of the crucial antioxidant enzyme having key role in scavenging of H2O2 and superoxide radicals for the synthesis of hydroxyl (OH–) radicals and decline of Haber–Weiss reaction. In a similar study it was known that up-regulation of CAT, POD, SOD and GR reduced membrane dysfunction in chickpea induced by free radicals. High content of POD might have role in reducing stress toxicity by accelerating lignin biosynthesis and other protective compounds (Boerjan et al. Citation2003). Enhanced activity of CAT boost up immunity against abiotic stress in Brassica juncea (Mittal et al. Citation2012). CAT function as eliminator of H2O2 to protect membranes and organelles damage because H2O2 is known as signaling molecule which quickly diffuse through membrane (Bienert and Chaumont Citation2014). A series of redox reactions occur in the ascorbate-glutathione cycle leading to eliminate H2O2 in cytosol and chloroplasts to reduce the toxic effects of oxidative-stress on plants under abiotic stress. Abscisic acid, a phytohormone accumulates in higher plants under abiotic stresses including high temperature. Elevated content of ABA was observed in Oryza sativa under thermal stress (Raghavendra et al. Citation2010). Thermal stress causes up-regulation of ABA biosynthesis genes while, down-regulates those genes which are responsible for ABA catabolism (Toh et al. Citation2008). In our study we detected low amount of ABA in sunflower and soybean inoculated with A. niger under heat stress as compared to endophyte-free seedlings. This reduction in ABA content in experimental plants might be due to down-regulation of genes responsible for ABA synthesis or acceleration of ABA degradation. Low amount of ABA in fungal endophyte associated soybean and sunflower confirmed the results of Hamayun et al. (Citation2017). In present study enhanced concentration in total nutritious value like total lipids, proteins and sugars, of A. niger-associated soybean and sunflower was found under thermal stress, indicating the positive role of endophytic fungi for crops and suggesting their use as bio-fertilizers. In a similar study by Rodriguez and Redman (Citation2008) pointed out the protective role of A. flavus for host plant against heat stress.
Conclusion
Thermal stress prevailing in the atmosphere is amongst the crucial constraints to agricultural crops, including soybean and sunflower. The present work testified a thermo-tolerant fungal strain, A. niger (MH577053), that not only enhanced growth in sunflower and soybean, but also facilitated it to resist heat stress. A. niger helped the host species to ameliorate the heat stress by boosting ROS scavenging antioxidant system, such as CAT, POD, SOD, AAO, GR, proline and phenolics. It also modulated endogenous level of ABA in sunflower and soybean as well as enhanced their nutritious values. The present study suggests the use of A. niger as a heat stress alleviating bio-agent and bio-fertilizer in the future for sustainable agriculture.
Author Contributions
Formal analysis, Muhammad Hamayun, AmjadIqbal, Sumera Afzal Khan; Funding acquisition, In-Jung Lee; Investigation, Ismail, Anwar Hussain; Project administration, In-Jung Lee, Muhammad Hamayun, Anwar Hussain; Resources, In-Jung Lee, Muhammad Hamayun, Anwar Hussain; Supervision, Muhammad Hamayun, Anwar Hussain; Writing – original draft, Ismail, Anwar Hussain; Writing – review & editing, Muhammad Hamayun, AmjadIqbal and In-Jung Lee.
Ethics approval and consent to participate
Our study doesn’t involve any human, animal or endangered species.
Consent for publication
No consent/approval at the national or international level or appropriate permissions and/or licenses for the study was required.
Availability of data and material
All the data are included in the manuscript.
Acknowledgement
This research was supported by the Basic Science Research Program through the National Research Foundation of Korea (NRF) funded by the Ministry of Education (2017R1D1A1B04035601).
Disclosure statement
No potential conflict of interest was reported by the author(s).
Additional information
Funding
Notes on contributors
Ismail
Dr. Ismail is an Assistant professor in Colleges and published papers on plant-microbe interactions in reputed journal.
Muhammad Hamayun
Prof. Muhammad Hamayun is a well known botanist/endophytologist. He is currently serving as a Head of the Department of Botany, Abdul Wali Khan University Mardan.
Anwar Hussain
Dr. Anwar Hussain is an Associate Professor at the Department of Botany, Abdul Wali Khan University Mardan, Who has broad experience in the field of endophytes.
Amjad Iqbal
Dr. Amjad Iqbal is an Associate Professor and Head of Food Science and Technology department at Abdul Wali Khan University Mardan and an expert in isolation and purification of bioactive compounds from various sources.
Sumera Afzal Khan
Dr. Sumera Afzal Khan is an Associate Professor, expert microbiologist and the Head of Center of Biotechnology and Microbiology, University of Peshawar.
In-Jung Lee
Prof. In-Jung Lee is a well known plant physiologist/endophytologist and a group leader at the Kyungpook National University, Korea.
References
- Abd_Allah EF, Alqarawi AA, Hashem A, Radhakrishnan R, Al-Huqail AA, Al-Otibi FON, Malik JA, Alharbi RI, Egamberdieva D. 2018. Endophytic bacterium Bacillus subtilis (BERA 71) improves salt tolerance in chickpea plants by regulating the plant defense mechanisms. J Plant Interac. 13:37–44. doi: 10.1080/17429145.2017.1414321
- Ashraf M, Foolad M. 2007. Roles of glycine betaine and proline in improving plant abiotic stress resistance. Environ Exp Bot. 59:206–216. doi: 10.1016/j.envexpbot.2005.12.006
- Ashraf M, Harris P. 2004. Potential biochemical indicators of salinity tolerance in plants. Plant Sci. 166:3–16. doi: 10.1016/j.plantsci.2003.10.024
- Bates LS, Waldren RP, Teare I. 1973. Rapid determination of free proline for water-stress studies. Plant Soil. 39:205–207. doi: 10.1007/BF00018060
- Benizri E, Courtade A, Picard C, Guckert A. 1998. Role of maize root exudates in the production of auxins by Pseudomonas fluorescens M. 3.1. Soil Biol Biochem. 30:1481–1484. doi: 10.1016/S0038-0717(98)00006-6
- Beyer Jr WF, Fridovich I. 1987. Assaying for superoxide dismutase activity: some large consequences of minor changes in conditions. Anal Biochem. 161:559–566. doi: 10.1016/0003-2697(87)90489-1
- Bienert GP, Chaumont F. 2014. Aquaporin-facilitated transmembrane diffusion of hydrogen peroxide. Biochimica et Biophysica Acta (BBA)-General Subjects. 1840:1596–1604. doi: 10.1016/j.bbagen.2013.09.017
- Bilal L, Asaf S, Hamayun M, Gul H, Iqbal A, Ullah I, Lee I-J, Hussain A. 2018. Plant growth promoting endophytic fungi Asprgillus fumigatus TS1 and Fusarium proliferatum BRL1 produce gibberellins and regulates plant endogenous hormones. Symbiosis. 76:117–127. doi:10.1007/s13199-018-0545-4.
- Boerjan W, Ralph J, Baucher M. 2003. Lignin biosynthesis. Annu Rev Plant Biol. 54:519–546. doi: 10.1146/annurev.arplant.54.031902.134938
- Cai Y, Luo Q, Sun M, Corke H. 2004. Antioxidant activity and phenolic compounds of 112 traditional Chinese medicinal plants associated with anticancer. Life Sci. 74:2157–2184. doi: 10.1016/j.lfs.2003.09.047
- Carlberg I, Mannervik B. 1985. [59] glutathione reductase. Method Enzymol. 113:484–490. doi: 10.1016/S0076-6879(85)13062-4
- Chang J, Wang Y, Shao L, Laberge R-M, Demaria M, Campisi J, Janakiraman K, Sharpless NE, Ding S, Feng W. 2016. Clearance of senescent cells by ABT263 rejuvenates aged hematopoietic stem cells in mice. Nat Med. 22:78–83. doi: 10.1038/nm.4010
- Chen W-p, Kuo T-T. 1993. A simple and rapid method for the preparation of gram-negative bacterial genomic DNA. Nucleic Acids Res. 21:2260. doi: 10.1093/nar/21.9.2260
- Dastogeer KM, Wylie SJ. 2017. Plant–fungi Association: role of fungal endophytes in improving plant tolerance to water stress. plant-microbe interactions in agro-ecological perspectives. Singapore: Springer.
- Gul Jan F, Hamayun M, Hussain A, Iqbal A, Jan G, Khan SA, Khan H, Lee I-J. 2019. A promising growth promoting Meyerozyma caribbica from Solanum xanthocarpum alleviated stress in maize plants. Biosci Rep. 39:1–15.
- Hamayun M, Hussain A, Khan SA, Irshad M, Khan AL, Waqas M, Shahzad R, Iqbal A, Ullah N, Rehman G. 2015. Kinetin modulates physio-hormonal attributes and isoflavone contents of soybean grown under salinity stress. Front Plant Sci. 6:377. doi: 10.3389/fpls.2015.00377
- Hamayun M, Hussain A, Khan SA, Kim H-Y, Khan AL, Waqas M, Irshad M, Iqbal A, Rehman G, Jan S. 2017. Gibberellins producing endophytic fungus Porostereum spadiceum AGH786 rescues growth of salt affected soybean. Front Microbiol. 8:1–13. doi:10.3389/fmicb.2017.00686.
- Hare P, Cress W. 1997. Metabolic implications of stress-induced proline accumulation in plants. Plant Growth Regul. 21:79–102. doi: 10.1023/A:1005703923347
- Hare PD, Cress WA, Van Staden J. 1998. Dissecting the roles of osmolyte accumulation during stress. Plant Cell Environ. 21:535–553. doi: 10.1046/j.1365-3040.1998.00309.x
- Ismail, Hamayun M, Hussain A, Afzal Khan S, Iqbal A, Lee I-J. 2019. Aspergillus flavus promoted the growth of soybean and sunflower seedlings at elevated temperature. BioMed Res Int. 2019:1–13. doi:10.1155/2019/1295457.
- Ismail, Hamayun M, Hussain A, Iqbal A, Khan SA, Lee I-J. 2018. Endophytic fungus Aspergillus japonicus mediates host plant growth under normal and heat stress conditions. BioMed Res Int. 2018. doi:10.1155/2018/7696831.
- Jan FG, Hamayun M, Hussain A, Jan G, Iqbal A, Khan A, Lee I-J. 2019. An endophytic isolate of the fungus Yarrowia lipolytica produces metabolites that ameliorate the negative impact of salt stress on the physiology of maize. BMC Microbiol. 19:3. doi:10.1186/s12866-018-1374-6.
- Kang S-M, Hamayun M, Khan MA, Iqbal A, Lee I-J. 2019. Bacillus subtilis JW1 enhances plant growth and nutrient uptake of Chinese cabbage through gibberellins secretion. J Appl Bot Food Qual. 92:172–178.
- Kar M, Mishra D. 1976. Catalase, peroxidase, and polyphenoloxidase activities during rice leaf senescence. Plant Physiol. 57:315–319. doi: 10.1104/pp.57.2.315
- Khan MA, Hamayun M, Iqbal A, Khan SA, Hussain A, Asaf S, Khan AL, Yun B-W, Lee I-J. 2018. Gibberellin application ameliorates the adverse impact of short-term flooding on Glycine max L. Biochem J. 475:2893–2905. doi: 10.1042/BCJ20180534
- Khan SA, Hamayun M, Yoon H, Kim H-Y, Suh S-J, Hwang S-K, Kim J-M, Lee I-J, Choo Y-S, Yoon U-H. 2008. Plant growth promotion and Penicillium citrinum. BMC Microbiol. 8:231. doi: 10.1186/1471-2180-8-231
- Khushdil F, Jan FG, Jan G, Hamayun M, Iqbal A, Hussain A, Bibi N. 2019. Salt stress alleviation in Pennisetum glaucum through secondary metabolites modulation by Aspergillus terreus. Plant Physiol Biochem. 144:127–134. doi: 10.1016/j.plaphy.2019.09.038
- Lorenzo O, Chico JM, Sánchez-Serrano JJ, Solano R. 2004. JASMONATE-INSENSITIVE1 encodes a MYC transcription factor essential to discriminate between different jasmonate-regulated defense responses in Arabidopsis. Plant Cell. 16:1938–1950. doi: 10.1105/tpc.022319
- Lowry OH, Rosebrough NJ, Farr AL, Randall RJ. 1951. Protein measurement with the Folin phenol reagent. J Biol Chem. 193:265–275.
- Luck H. 1974. Methods in enzymatic analysis II (ed.) Bergmeyer.(publ.). New York: Academic Press.
- Mehmood A, Hussain A, Irshad M, Hamayun M, Iqbal A, Khan N. 2019a. In vitro production of IAA by endophytic fungus Aspergillus awamori and its growth promoting activities in Zea mays. Symbiosis. 77:225–235. doi:10.1007/s13199-018-0583-y.
- Mehmood A, Hussain A, Irshad M, Hamayun M, Iqbal A, Rahman H, Tawab A, Ahmad A, Ayaz S. 2019b. Cinnamic acid as an inhibitor of growth, flavonoids exudation and endophytic fungus colonization in maize root. Plant Physiol Biochem. 135:61–68. doi: 10.1016/j.plaphy.2018.11.029
- Mhamdi A, Queval G, Chaouch S, Vanderauwera S, Van Breusegem F, Noctor G. 2010. Catalase function in plants: a focus on Arabidopsis mutants as stress-mimic models. J Exp Bot. 61:4197–4220. doi: 10.1093/jxb/erq282
- Misra N, Dwivedi U. 2004. Genotypic difference in salinity tolerance of green gram cultivars. Plant Sci. 166:1135–1142. doi: 10.1016/j.plantsci.2003.11.028
- Mittal S, Kumari N, Sharma V. 2012. Differential response of salt stress on Brassica juncea: photosynthetic performance, pigment, proline, D1 and antioxidant enzymes. Plant Physiol Biochem. 54:17–26. doi: 10.1016/j.plaphy.2012.02.003
- Mohammadkhani N, Heidari R. 2008. Drought-induced accumulation of soluble sugars and proline in two maize varieties. World Appl. Sci. J. 3:448–453.
- Morrison EN, Knowles S, Hayward A, Thorn RG, Saville BJ, Emery R. 2015. Detection of phytohormones in temperate forest fungi predicts consistent abscisic acid production and a common pathway for cytokinin biosynthesis. Mycologia. 107:245–257. doi: 10.3852/14-157
- Muhammad I, Niaz A, Gul J, Amjad I, Muhammad H, Farzana GJ, Anwar H, In-Jung L. 2019. Trichoderma reesei improved the nutrition status of wheat crop under salt stress. J Plant Interact. 14:590–602. doi: 10.1080/17429145.2019.1684582
- Nusrat B, Gul J, Farzana GJ, Muhammad H, Amjad I, Anwar H, Hazir R, Abdul T, Faiza K. 2019. Cochliobolus sp. acts as a biochemical modulator to alleviate salinity stress in okra plants. Plant Physiol Biochem. 139:459–469. doi:10.1016/j.plaphy.2019.04.019.
- Oberbacher M, Vines H. 1963. Spectrophotometric assay of ascorbic acid oxidase. Nature. 197:1203–1204. doi: 10.1038/1971203a0
- Raghavendra AS, Gonugunta VK, Christmann A, Grill E. 2010. ABA perception and signalling. Trends Plant Sci. 15:395–401. doi: 10.1016/j.tplants.2010.04.006
- Rodriguez R, Redman R. 2008. More than 400 million years of evolution and some plants still can't make it on their own: plant stress tolerance via fungal symbiosis. J Exp Bot. 59:1109–1114. doi: 10.1093/jxb/erm342
- Rodriguez RJ, Redman RS, Henson JM. 2004. The role of fungal symbioses in the adaptation of plants to high stress environments. Mitig Adapt Strat Gl Chang. 9:261–272. doi: 10.1023/B:MITI.0000029922.31110.97
- Rodriguez R, White Jr J, Arnold AE, Redman aRa. 2009. Fungal endophytes: diversity and functional roles. New Phytol. 182:314–330. doi: 10.1111/j.1469-8137.2009.02773.x
- Sherameti I, Tripathi S, Varma A, Oelmüller R. 2008. The root-colonizing endophyte Pirifomospora indica confers drought tolerance in Arabidopsis by stimulating the expression of drought stress–related genes in leaves. Mol Plant-Microbe Interact. 21:799–807. doi: 10.1094/MPMI-21-6-0799
- Sun C, Johnson JM, Cai D, Sherameti I, Oelmüller R, Lou B. 2010. Piriformospora indica confers drought tolerance in Chinese cabbage leaves by stimulating antioxidant enzymes, the expression of drought-related genes and the plastid-localized CAS protein. J Plant Physiol. 167:1009–1017. doi: 10.1016/j.jplph.2010.02.013
- Toh S, Imamura A, Watanabe A, Nakabayashi K, Okamoto M, Jikumaru Y, Hanada A, Aso Y, Ishiyama K, Tamura N. 2008. High temperature-induced abscisic acid biosynthesis and its role in the inhibition of gibberellin action in Arabidopsis seeds. Plant Physiol. 146:1368–1385. doi: 10.1104/pp.107.113738
- Van Handel E. 1985. Rapid determination of total lipids in mosquitoes. J Am Mosq Control Assoc. 1:302–304.
- Waqas M, Khan AL, Kamran M, Hamayun M, Kang S-M, Kim Y-H, Lee I-J. 2012. Endophytic fungi produce gibberellins and indoleacetic acid and promotes host-plant growth during stress. Molecules. 17:10754–10773. doi: 10.3390/molecules170910754
- Warrier R, Paul M, Vineetha M. 2013. Estimation of salicylic acid in Eucalyptus leaves using spectrophotometric methods. Genetics Plant Physiol. 3:90–97.
- Yoon JY, Hamayun M, Lee S-K, Lee I-J. 2009. Methyl jasmonate alleviated salinity stress in soybean. J Crop Sci Biotechnol. 12:63–68. doi: 10.1007/s12892-009-0060-5