ABSTRACT
Abiotic stresses limit the plant growth and productivity. Plants have developed various responsive mechanisms to survive these adverse environmental conditions. To better understand plants how to adapt environmental stress, we retrieved the Arabidopsis root responsive genes related to eight abiotic stresses (salt, cold, drought, osmotic, UV, wounding, heat, and heavy metal stresses) from the Gene Expression Omnibus (GEO) database. After quantile normalization of microarray data, a robust rank aggregation (RRA) algorithm was applied to determine the differentially expressed genes (DEGs). The resultant 213 DEGs, including 139 up-regulated and 74 down-regulated genes out of more than 20,000 genes, were further analyzed by the integrated bioinformatics approaches of PUBMED, PANTHER, DAVID, and STRING. The results indicated that the up-regulated DEGs were more positively involved in response to oxidative stress, regulation of transcription, and structure organization than the down-regulated DEGs. The former also had more complicated PPI interactions than the latter. Furthermore, qRT-PCR and enzyme activity have been done to validate the outcome of integrated bioinformatics analysis. Our work will facilitate to demonstrate the common molecular mechanisms responsive to differential abiotic stresses and these DEGs might be potential biomarkers for future abiotic stress-resistance studies.
Introduction
Agricultural production has been severely disrupted by various abiotic stresses such as salinity, drought, climate changes, and heavy metal around the world, which threatens the food security required for the growing global population. To improve plant resistance on these abiotic stresses and increase crop yield, many researches have been done to explore the adaption mechanisms plant evolved under variable field conditions for the past decades (Gong et al. Citation2020).
Microarray technology has been used to measure the abundance of thousands of mRNAs. But microarray is still cost-prohibitive experiment for each lab. It’s difficult for an individual lab to determine the Arabidopsis expression profilings in development stages, and growth responses under different stages. Therefore, the public repositories such as Gene Expression Omnibus provide an important platform to disclose the plant physiological mechanism using the available thousands of expression profiles unloaded by different labs. In order to effectively compare these microarray data and obtain the plant insights, integrated bioinformatic method has been applied to predict the molecular functions and biological pathways involved in Arabidopsis genes, or visualize the coexpressed genes based on these public data. In the previous research, more than 6000 microarray data have been selected from GEO to reveal the expression patterns of different tissues in Arabidopsis. Based on the analysis of integrated bioinformatics, the expression profiles of the same tissue are more similar to each other than these from other tissues in Arabidopsis (He et al. Citation2016). Another bioinformatic analysis of salt-responsive expression profilings have been done using the data from GEO. A rank aggregation method (RRA) was applied to screen 452 upregulated and 377 down-regulated DEGs under salt stress in Arabidopsis. Gene ontology enrichment analysis showed these DEGs were involved in signaling, transcription, and development (Zhang et al. Citation2017). Furthermore, integrated bioinformatics analysis was also used to reveal the DEGs under heavy metal through Arabidopsis GEO datasets. The results demonstrated that 168 DEGs were associated with the response under As, Cd, Pb and Cu. GO and KEGG pathway enrichments found these DEGs were related to responses to stress, responses to chemical, and responses to abiotic stimulus (Niu et al. Citation2019).
Arabidopsis is an ideal plant model for stress response research. The DEGs under salt, UV light, wounding, heat and cold stresses have been explored using microarray technique to disclose the interaction networks in A. thaliana root (Kilian et al. Citation2007). The drought-responsive DEGs in A. thaliana root have been studied, which were indicated to be related to DNA methylation, osmotic balance and apoptosis (Pandey et al. Citation2013). A novel DEG (IAR3) under high osmotic stress also have been documented by microarray analysis in A. thaliana root (Kinoshita et al. Citation2012). The DEGs responsive to heavy metal (Cd) were found to be associated with antioxidant capacity in A. thaliana root (López-Martín et al. Citation2008). In our previous work, we focused on the salt-responsive molecules using A. thaliana as the model plant. The results indicated the differential molecules were involved in cell wall metabolisms and reactive oxygen species (ROS) scavenging (Guo et al. Citation2014a). More importantly, the complicated cross-tolerances to differential stresses such as osmosis, water deprivation, heat, UV-light and wounding has been shown in A. thaliana (Guo et al. Citation2019).
In the present work, to demonstrate the details of eight abiotic stresses (salt, cold, drought, osmotic, UV, wounding, heat, and heavy metal stresses) in Arabidopsis root, the DEGs were retrieved from the gene expression omnibus database (GEO). From more than 20,000 Arabidopsis genes, 213 DEGs were obtained using a rank aggregation method. Then, the molecular function was analyzed by the integrated bioinformatics tools, followed by experimental validation. These altered genes will facilitate better understanding on the stress-response mechanisms, and might be useful targets for promoting crop output and plant growth.
Materials and methods
Retrieval of salt-responsive genes in Arabidopsis roots from GEO database
The responsive gene expression profiling of the microarray data (GSM131257, GSM131258, GSM131469, GSM131470, GSM131281, GSM131282, GSM984521, GSM984522, GSM984523, GSM984527, GSM984528, GSM984529, GSM901072, GSM901073, GSM901074, GSM901078, GSM901079, GSM901080, GSM131409, GSM131410, GSM131437, GSM131438, GSM131469, GSM131470, GSM476827, GSM476828, GSM476829, GSM476839, GSM476840, GSM476841) related to eight stresses (salt, cold, drought, osmotic, UV, wounding, heat, and heavy metal (Cd) stresses) in Arabidopsis roots were downloaded from the GEO database (https://www.ncbi.nlm.nih.gov/geo/). Briefly the raw data were qualified by quantile normalization, and then the DEGs were screened by the limma package. Genes with P-value < 0.05 and |log2fold change (FC)|> 1 were defined as DEGs. The resultant DEGs were further integrated by a robust rank aggregation (RRA) algorithm. The hypothesis of RRA method was that each gene is randomly ordered in experiment. If a gene has a high rank among all experiments, then its P-value is small (Niu et al. Citation2019; Zhang et al. Citation2017).
Gene ontology classification and PPI network
The online bioinformatic tools of PANTHER (Protein Analysis THrough Evolutionary Relationships) classification system (Version 15.0 released 2019_04) (http://pantherdb.org/) and DAVID (Database for annotation, visualization and integrated discovery, Version 6.8) (https://david.ncifcrf.gov/) were applied for gene ontology enrichment analysis of the DEGs. Each gene was classified into one category. The analysis of PPI interaction networks was performed by the online STRING (search tool for recurring instances of neighboring genes) database (Version 11.0, released January 19, 2019) (http://string-db.org/).
Growth conditions and harvest of Arabidopsis root
According to previous publications (Guo et al. Citation2014a; Kilian et al. Citation2007), the ecotype Col-0 Arabidopsis seeds were germinated on the normal medium plate with 22/20°C day/night, 8/16 h light/dark cycle, 60 μmol·m−2s−1 light intensity. After 7 days, the A. thaliana seedlings separately underwent the following stresses: the cold-stress condition (8°C), the osmotic stress condition (200 mmol·L−1 mannitol), the salt stress condition (150 mmol·L−1 NaCl), the wounding stress condition by punctuation of the leaves with needles, the drought stress condition by removing the lid of dish and exposing to a stream of air for 15 min, the UV stress condition by removing the lid of dish and irradiated with UV light for 15 min, the heat stress condition by exposing to a temperature of 35°C for 3 h, and the heavy metal condition (100 μmol·L−1 CdCl2), followed by another 3 days growth. Then the Arabidopsis roots were harvested and stored at –80°C.
qRT-PCR analysis
According to the previous method of RNA isolation and qRT-PCR analysis (Li et al. Citation2015; Li et al. Citation2019), the relative expression levels of Arabidopsis gene ascorbate peroxidase 2 (AT3G09640) was determined and Arabidopsis gene actin 2 (AT3G18780) was used as endogenous reference in Arabidopsis root. All experiments were performed in triplicate.
Enzyme activity assay
Peroxidase (POD) activity in Arabidopsis root was assayed using a commercial kit (Nanjing Jiancheng Bioengineering Institute) according to the previous publication (Guo et al. Citation2019). Briefly, the POD activity was determined by the catalyzing H2O2 of absorbance change at 420 nm. The plots were done by Graphpad Prism for windows (Version 8.0). All experiments were performed in triplicate.
Results
Identification of stress-responsive DEGs in Arabidopsis root
Dataset from eight stress-responsive experiments in Arabidopsis root were retrieved from the GEO database (). After the microarray data were qualified by quantile normalization (), DEGs in Arabidopsis roots under eight abiotic stress were shown in . Then, the RRA method was used to integrate the above DEGs. As a result, 139 up-regulated and 74 down-regulated genes were screened as the stress-responsive DEGs of Arabidopsis root ().
Figure 1. Standardization for all samples included GEO datasets. A. salt stress (GSM131257, GSM131258, GSM131469, GSM131470); B. cold stress (GSM131257, GSM131258, GSM131281, GSM131282); C. drought stress (GSM984521, GSM984522, GSM984523, GSM984527, GSM984528, GSM984529); D. osmotic stress (GSM901072, GSM901073, GSM901074, GSM901078, GSM901079, GSM901080); E. UV stress (GSM131257, GSM131258, GSM131407, GSM131410); F. wounding stress (GSM131257, GSM131258, GSM131437, GSM131438); G. heat stress (GSM131257, GSM131258, GSM131469, GSM131470); H. heavy metal Cd stress (GSM476827, GSM476828, GSM476839, GSM476839, GSM476840, GSM476841).
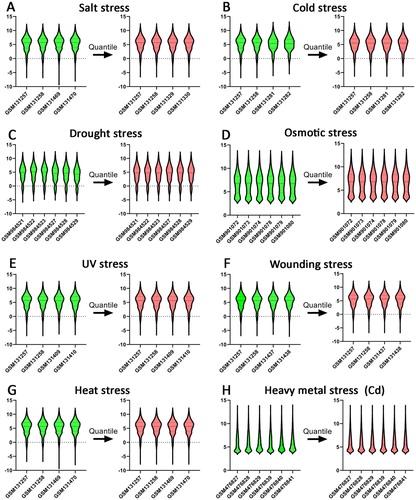
Figure 2. Differential expression genes of Arabidopsis roots responding to abiotic stress. A. salt stress (GSM131257, GSM131258, GSM131469, GSM131470); B. cold stress (GSM131257, GSM131258, GSM131281, GSM131282); C. drought stress (GSM984521, GSM984522, GSM984523, GSM984527, GSM984528, GSM984529); D. osmotic stress (GSM901072, GSM901073, GSM901074, GSM901078, GSM901079, GSM901080); E. UV stress (GSM131257, GSM131258, GSM131407, GSM131410); F. wounding stress (GSM131257, GSM131258, GSM131437, GSM131438); G. heat stress (GSM131257, GSM131258, GSM131469, GSM131470); H. heavy metal Cd stress (GSM476827, GSM476828, GSM476839, GSM476839, GSM476840, GSM476841). The red points represent up-regulated genes (fold change > 2.0, P-value < 0.05). The green points represent down-regulated genes (fold change < 0.5, P-value < 0.05). The black points represent unchanged genes difference.
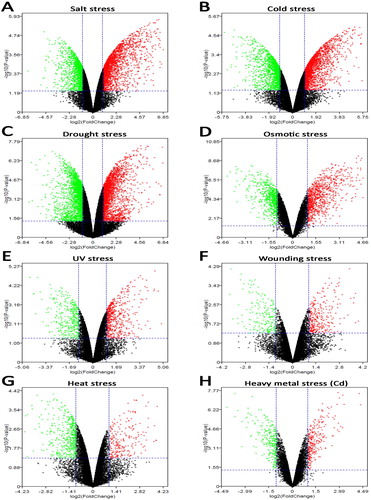
Table 1. Summary of the datasets of stress-responsive experiments in Arabidopsis root from the GEO database.
Table 2. Differential DEGs in Arabidopsis root under eight abiotic stresses.
Bioinformatics enrichment of stress-responsive DEGs
Bioinformatics tools were used to analyze the 213 DEGs. For biological process, the main processes that the up-regulated DEGs related to were regulation of biological process (34.5%), metabolic process (22.3%), regulation of transcription (16.5%), and response to oxidative stress (14.3%) ((A)). For molecular function, the major molecular functions involved were binding activity (43.2%), catalytic activity (22.3%), and antioxidant activity (13.7%) ((C)). Whereas, the percentage of antioxidant activity involvement for down-regulated DEGs was 2.7% ((C)).
PPI network of salt-responsive DEGs
Totally, 139 up-regulated stress-responsive DEGs were connected with 226 edges (PPI enrichment p-value: < 1.0e−16), and 74 down-regulated stress-responsive DEGs were connected with 27 edges (PPI enrichment p-value: < 5.22e−15) by STRING ((B)).
qRT-PCR analysis
Our results showed Arabidopsis gene ascorbate peroxidase 2 (AT3G09640) was up-regulated among salt stress, cold stress, drought stress, osmotic stress, UV stress, wounding stress, heat stress, and heavy metal stress. Therefore, the relative expression of Arabidopsis gene ascorbate peroxidase 2 (AT3G09640) in Arabidopsis roots were assayed under the above stresses to validate the confidence of the bioinformatics outcome. The results indicated that the relative expression level of Arabidopsis gene ascorbate peroxidase 2 (AT3G09640) in Arabidopsis roots were significantly increased under different stresses ().
Enzyme activity determination stress conditions
Our results showed peroxidase was up-regulated among salt stress, cold stress, drought stress, osmotic stress, UV stress, wounding stress, heat stress, and heavy metal stress. Therefore, POD activities of Arabidopsis roots were assayed under the above stresses to validate the confidence of the bioinformatics outcome. The results indicated that POD activities were all increased under different stresses ().
Discussion
Abiotic stress negatively affects crop output and plant growth. To disclose the salt-tolerance mechanism in plant, many researches has used A. thaliana as a model plant (Zhang et al. Citation2018). In the past decades, some molecular targets and stress-responsive mechanisms have been demonstrated (Carrera et al. Citation2018), and cross-talking genes responsive to different stress have been reported. Our previous work indicated that there were complicated pathways, protein interactions and cross-talks under different stresses in A. thaliana (Guo et al. Citation2019). Many genes or proteins participated in response to more than one stress, which is called cross-tolerance to multiple stresses. Cross-tolerance is an important strategy for plants to survive under different stresses (Tuteja Citation2007). But it is still not well understood how plants respond to different stresses. Therefore, more details are needed to provide more information during the plant responses under multiple stresses.
In the present work, we retrieved DEGs under eight different stresses (salt stress, cold stress, drought stress, osmotic stress, UV stress, wounding stress, heat stress, and heavy metal stress), and integrated the DEGs using RRA method. The resultant 213 DEGs, including 139 up-regulated and 74 down-regulated genes out of more than 20,000 genes, were further analyzed by the integrated bioinformatics approaches. Among the up-regulated DEGs, the blue light-regulated COR27 gene was associated with cold stress (Wang et al. Citation2017). Under heat stress, HSP70 was involved in thermotolerance by interacting with HsfA1 and inhibiting its nuclear localization (Hahn et al. Citation2011). HsfA9 also modulates the heat stress-related genes as a specific transcription repressor (Baniwal et al. Citation2007). Under cold stress, MYB15, a member of MYB family, were found to bind to the CBF genes promoters as another transcriptional repressor (Agarwal et al. Citation2006), and late elongated hypocotyl (LHY) also participated in the regulation of CBF expression as a transcriptional factor (Dong et al. Citation2011).
Based on the integrated bioinformatics analysis, response to oxidative stress was the main biological process. In the present work, POD, glutathione S-transferase U5 (GSTU5), L-ascorbate peroxidase 2 (APX2) were all up-regulated under eight stresses. Usually, the electron transport chain will be attenuated under salt stress and excessive ROS, such as hydrogen peroxide, hydroxyl radicals, and superoxide radicals produced (Miller et al. Citation2010). As the result, ROS scavenging enzymes such as the glutathione S-transferase family (Chan and Lam Citation2014) and POD (Islam et al. Citation2015) are activated to balance the redox homeostasis in plant cell. To validate the DEG cross-talk among different stresses, the enzyme activity of POD in Arabidopsis root was analyzed under all eight stress conditions. The results showed the stress cross-talk prediction agreed with the outcome of integrated bioinformatics analysis.
Conclusion
This work provided new light on the understanding of stress-tolerance DEGs under multiple stresses in Arabidopsis root, which improved our information on stress-response mechanisms in plant, and might become useful targets for promoting crop output and plant growth in the future.
Acknowledgements
MG conceived the study, and designed the experiments. MG, XL, YJ, XY, and TM performed the experiments.
MG, XL, JW, LL, YJ, XY and TM analyzed the raw data, and drafted the manuscript. All the authors participated in the revision of the manuscript.
Disclosure statement
No potential conflict of interest was reported by the author(s).
Additional information
Funding
Notes on contributors
Meili Guo
Meili Guo is an Associate Professor of Food and Biochemistry Engineering Department at Yantai Vocational College. Her research interest focuses on Arabidopsis growth and development, and the responses to abiotic stress responses.
Xin Liu
Dr Xin Liu is an Associate Professor of Central Laboratory at the Affiliated Yantai Yuhuangding Hospital of Qingdao University. His research interest focuses on genomics, proteomics and bioinformatics.
Jiahui Wang
Dr Jiahui Wang is a lecturer of Central Laboratory at the Affiliated Yantai Yuhuangding Hospital of Qingdao University. Her work focuses on statistics and bioinformatics.
Lei Li
Lei Li is a lecturer of Medical Experiment and Test Center at Capital Medical University. Her research interest focuses on genomics, proteomics and bioinformatics.
Yusu Jiang
Yusu Jiang is a lecturer of Food and Biochemistry Engineering Department at Yantai Vocational College. Her work focuses on investigations of plant growth and development, and stress physiology in plants.
Xuejuan Yu
Xuejuan Yu is an Associate Professor of Food and Biochemistry Engineering Department at Yantai Vocational College. Her research interest focuses on plant physiology.
Tao Meng
Tao Meng is an assistant lecturer of Food and Biochemistry Engineering Department at Yantai Vocational College. His work focuses on plant physiology and plant abiotic stress responses.
References
- Agarwal M, Hao Y, Kapoor A, Dong CH, Fujii H, Zheng X, Zhu JK. 2006. A R2R3 type MYB transcription factor is involved in the cold regulation of CBF genes and in acquired freezing tolerance. J Biol Chem. 281:37636–37645. doi: 10.1074/jbc.M605895200
- Baniwal SK, Chan KY, Scharf KD, Nover L. 2007. Role of heat stress transcription factor HsfA5 as specific repressor of HsfA4. J Biol Chem. 282:3605–3613. doi: 10.1074/jbc.M609545200
- Carrera DÁ, Oddsson S, Grossmann J, Trachsel C, Streb S. 2018. Comparative Proteomic analysis of plant Acclimation to Six different Long-Term environmental changes. Plant Cell Physiol. 59(3):510–526. doi: 10.1093/pcp/pcx206
- Chan C, Lam HM. 2014. A putative lambda class glutathione S-transferase enhances plant survival under salinity stress. Plant Cell Physiol. 55(3):570–579. doi: 10.1093/pcp/pct201
- Dong MA, Farré EM, Thomashow MF. 2011. Circadian clockassociated 1 and late elongated hypocotyl regulate expression of the Crepeat binding factor (CBF) pathway in Arabidopsis. Proc Natl Acad Sci USA. 108:7241–7246. doi: 10.1073/pnas.1103741108
- Gong Z, Xiong L, Shi H, Yang S, Herrera-Estrella LR, Xu G, Chao DY, Li J, Wang PY, Qin F, et al. 2020. Plant abiotic stress response and nutrient use efficiency. Sci China Life Sci. 63(5):635–674.
- Guo ML, Li H, Li L, Cheng XM, Gao WX, Xu YL, Zhou CX, Liu FJ, Liu X. 2014a. Comparative proteomic analysis of Arabidopsis thaliana roots between wild type and its salt-tolerant mutant. J Plant Interact. 9(1):330–337. doi: 10.1080/17429145.2013.833653
- Guo ML, Liu X, Wang JH, Li L, Zhang WD, Gong BJ, Zhang CL, Zhou CX. 2019. Investigation on salt-response mechanisms in Arabidopsis thaliana from UniProt protein knowledgebase. J Plant Interact. 14(1):21–29. doi: 10.1080/17429145.2018.1551581
- Hahn A, Bublak D, Schleiff E, Scharf KD. 2011. Crosstalk between Hsp90 and Hsp70 chaperones and heat stress transcription factors in tomato. Plant Cell. 23:741–755. doi: 10.1105/tpc.110.076018
- He F, Yoo S, Wang DF, Kumari S, Gerstein M, Ware D, Maslov S. 2016. Large-scale atlas of microarray data reveals the distinct expression landscape of different tissues in Arabidopsis. Plant J. 86:472–480. doi: 10.1111/tpj.13175
- Islam T, Manna M, Reddy MK. 2015. Glutathione peroxidase of Pennisetum glaucum (PgGPx) Is a functional Cd2+ Dependent Peroxiredoxin that Enhances tolerance against salinity and drought stress. PLoS One. 10(11):1–18. doi: 10.1371/journal.pone.0143344
- Kilian J, Whitehead D, Horak J, Wanke D, Weinl S, Batistic O, D'Angelo C, Bornberg-Bauer E, Kudla J, Harter K. 2007. The AtGenExpress global stress expression data set: protocols, evaluation and model data analysis of UV-B light, drought and cold stress responses. Plant J. 50(2):347–363. doi: 10.1111/j.1365-313X.2007.03052.x
- Kinoshita N, Wang H, Kasahara H, Liu J, Macpherson C, Machida Y, Kamiya Y, Hannah MA, Chua NH. 2012. IAA-Ala Resistant3, an evolutionarily conserved target of miR167, mediates Arabidopsis root architecture changes during high osmotic stress. Plant Cell. 24(9):3590–3602. doi: 10.1105/tpc.112.097006
- Li ZQ, Li JT, Bing J, Zhang GF. 2019. The role analysis of APX gene family in the growth and developmental processes and in response to abiotic stresses in Arabidopsis thaliana. Yi Chuan. 41(6):534–547.
- Li SH, Li N, Zhu P, Wang YW, Tian YJ, Wang XT. 2015. Decreased β-catenin expression in first-trimester villi and decidua of patients with recurrent spontaneous abortion. J Obstet Gynaecol Res. 41(6):904–911. doi: 10.1111/jog.12647
- López-Martín MC, Becana M, Romero LC, Gotor C. 2008. Knocking out cytosolic cysteine synthesis compromises the antioxidant capacity of the cytosol to maintain discrete concentrations of hydrogen peroxide in Arabidopsis. Plant Physiol. 147(2):562–572. doi: 10.1104/pp.108.117408
- Miller G, Suzuki N, Ciftci-Yilmaz S, Mittler R. 2010. Reactive oxygen species homeostasis and signalling during drought and salinity stresses. Plant Cell Environ. 33(4):453–467. doi: 10.1111/j.1365-3040.2009.02041.x
- Niu C, Jiang M, Li N, Cao J, Hou M, Ni DA, Chu Z. 2019. Integrated bioinformatics analysis of As, Au, Cd, Pb and Cu heavy metal responsive marker genes through Arabidopsis thaliana GEO datasets. PeerJ. 7:1–25.
- Pandey N, Ranjan A, Pant P, Tripathi RK, Ateek F, Pandey HP, Patre UV, Sawant SV. 2013. CAMTA 1 regulates drought responses in Arabidopsis thaliana. BMC Genomics. 14:1–23. doi: 10.1186/1471-2164-14-216
- Tuteja N. 2007. Abscisic Acid and abiotic stress signaling. Plant Signal Behav. 2(3):135–8. doi: 10.4161/psb.2.3.4156
- Wang P, Cui X, Zhao C, Shi L, Zhang G, Sun F, Cao X, Yuan L, Xie Q, Xu X. 2017. COR27 and COR28 encode nighttime repressors integrating Arabidopsis circadian clock and cold response. J Integr Plant Biol. 59:78–85. doi: 10.1111/jipb.12512
- Zhang S, Yang R, Huo Y, Liu S, Yang G, Huang J, Zheng C, Wu C. 2018. Expression of cotton PLATZ1 in transgenic Arabidopsis reduces sensitivity to osmotic and salt stress for germination and seedling establishment associated with modification of the abscisic acid, gibberellin, and ethylene signalling pathways. BMC Plant Biol. 18(1):1–11. doi: 10.1186/s12870-019-1719-9
- Zhang LY, Zhang XJ, Fan SJ. 2017. Meta-analysis of salt-related gene expression profiles identifies common signatures of salt stress responses in Arabidopsis. Plant Syst Evol. 303:757–774. doi: 10.1007/s00606-017-1407-x