ABSTRACT
The short shoot phenomenon is an important phenotype underlying the economic value of roses – node number can directly affect shoot length. To elucidate the molecular mechanism of how day length affects shoot length, the effect of 8, 10, and 12 h day lengths on node number were studied. The number of nodes between the 8 and 12 h treatment groups significantly differed. Node development in the 12 h treatment group occurred significantly earlier than that in the 8 and 10 h treatment groups and the number of nodes reached a maximum on the third day. Using RNA-seq analysis, we identified candidate genes that regulated shoot length; these were involved in the abscisic acid synthesis pathway and auxin signal transduction and synthesis pathways. Furthermore, the abscisic acid content in the shoot tip decreased with an increasing day length, while the auxin content increased with day length.
Introduction
Roses (Rosa sp.) are one of the main cut flowers sold worldwide (Halevy Citation1986; Niu and Rodriguez Citation2009). The grading standards of roses differ slightly depending on the region, but shoot length is the main grading standard for commercial roses (Zhang et al. Citation2020). Heilongjiang Province, located in the cold, high-altitude region of China, had an annual planting area of 370 ha in 2019, with an annual output of 250 million rose shoots. Thus, it is one of the main summer rose production areas of roses in China. However, the short shoot phenomenon in summer has become a challenge in commercial cut flower production.
The shorter shoot phenomenon exhibited by roses during summer is related to seasonal climate, and studies on the effects of seasonal climate on plant growth usually focus on temperature and sunshine (In and Lim Citation2018). However, it is difficult to separate the effects of temperature and sunshine, which are usually correlated. Previous studies have demonstrated that the seasonal difference in rose shoot length might be influenced by temperature, sunshine duration, or a combination of the two factors (Plaut and Zieslin Citation1977; Plaut et al. Citation1979). Furthermore, other studies have shown that light is an important climatic factor in the interseasonal differences in rose shoot length. It is well known that day length plays an essential role in seasonal plant growth of plants (Triozzi PM et al. Citation2018); however, the regulatory mechanism of day length in rose shoot development remains unclear, and there are few relevant local and international studies in this regard.
The node number is an important phenotype for studyies on rose shoot lengths (Moe and Kristoffersen Citation1969; De Vries and Smeets Citation1979; Leiv and Mortensen Citation1991). Horridge and Cockshull (Citation1974) dissected the shoot tips of the cultivars ‘Baccara,’ ‘Pink Sensation,’ ‘Super Star,’ and ‘Sonia’ under a microscope, charting the developmental process from vegetative growth to flowering. Their results showed that the number of nodes reached a maximum at the flower bud differentiation stage (Horridge and Cockshull Citation1974). The same conclusion has also been made for maize. Another study reported that the number of nodes was related to flowering time in the maize internode length phenotypes (Liu et al. Citation2021). Therefore, the flower bud differentiation period can be used as a key characteristic to study the number of rose nodes.
Auxin is a key hormone that affects the growth and development of plant shoots. Chen et al. (Citation2011) showed that indole-3-acetic acid (IAA) is a major auxin in plants that is related to shoot elongation (Chen et al. Citation2011). Furthermore, Wang and Yao (Citation2020) demonstrated that IAA had a significant effect on promoting the formation of rooting seedlings and shoots of the Masson pine and Sairanen et al. (Citation2012) indicated that light interacts with IAA metabolism in Arabidopsis. In addition, Lilley et al. (Citation2012) reported that IAA was mediated by a photochromatic interaction factor (PIF) transcriptional regulator and influenced early seedling growth. Evidence suggests an interaction between abscisic acid and IAA. Shuai et al. (Citation2017) reported that exogenous auxin inhibited soybean seed germination by mediating abscisic acid and gibberellin biosynthesis. Furthermore, Suzuki et al. (Citation2001) showed that the ectopic expression of size VP1 revealed a new interaction between auxin and abscisic acid in roots. There are numerous studies on the effects of plant growth regulators on the growth and development of rose species (Djennane et al. Citation2014; Roman et al. Citation2016; Jing et al. Citation2020), but there are relatively few studies on the correlation between endogenous hormone levels and the shoot length of rose plants.
To understand the short shoot phenomenon that occurs in roses during summer, we used the main cut rose cultivars to observe the shoot length difference between summer and autumn and determined the key phenotypes and sensitive cultivars for further investigations. Furthermore, the mechanism of action of the day length on the short shoot phenomenon of summer roses was preliminarily explained by phenotypic analyses, microscopic observations, and transcriptome sequencing. The results provide a theoretical basis for overcoming the production bottleneck caused by the short shoot phenomenon in summer roses and help to enhance the market competitiveness of cut roses in Heilongjiang Province.
Materials and methods
Plant material and experimental procedures
In this study, rose cultivars ‘Carola’ and ‘Evening Glow’ were selected. In a plastic greenhouse at the Northeast Agricultural University Experimental Station (45°75′ N, 126°63′ E), 1-year-old seedling cuttings were planted in 4-inch pots, one per pot. The matrix comprised charcoal and vermiculite (3:1) and 2 g Oryzol slow-release fertiliser 318S in each pot. The seedling treatment was as follows: each plant was pruned 0.5 cm above the third leaflet from the base of the shoot. The axillary buds of the third leaflet of the shoots were tested ((a)), and buds were removed once a week.
Figure 1. Test materials and sample preparation. (a) Test seedling treatment. (b) Sample production. Scale bars = 1 cm.
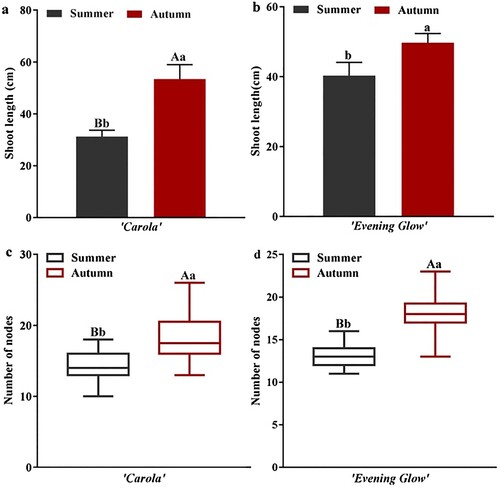
In April 2017, the rose cultivars ‘Carola’ and ‘Evolving Glow’ were planted in the greenhouse to observe the morphological characteristics of shoot development in summer (June 9 to July 24) and autumn (July 24 to September 19) in a natural environment (Table S4). Rose cultivar ‘Carola’ was planted during the same period in April 2017 to observe the phenotypic differences in the development of day-length treated shoots. Day length treatments included durations of 8 h (8:00–16:00), 10 h (6:00–16:00), and 12 h (6:00–18:00), while investigated phenotypes included shoot length, diameter, and dry weight (Table S5). Each treatment comprised 30 plants in triplicate; therefore, 90 plants were evaluated for each treatment. Paraffin sections were used to determine the period when the number of nodes reached a maximum, and transcriptome sequencing and hormone measurements were carried out on all samples processed in the period with the maximum number of nodes.
Microscopic observations
The samples were fixed overnight in formaldehyde glacial acetic acid (FAA) (70% ethanol, 18:1:1), dehydrated using 100% ethanol and xylene, embedded in paraffin, and sectioned at 10 μm intervals. After drying, the sections were dewaxed in xylene, washed with ethanol, and stained with a saffron and solid green stain. Thereafter, the sections were photographed using a research-grade ortho-microscopy system (Nikon Model Ci-L; Nikon, Tokyo, Japan).
IAA and ABA content assay
Ultra-performance liquid chromatography (UPLC), coupled with a tandem quadrupole mass spectrometer equipped with an electrospray interface (UPLC-ESI-QMS/MS), was used to determine the levels of IAA and ABA in shoot tip tissues (Kojima et al. Citation2009). The samples were isolated over a 10-day period following day length treatment. At 10 am on the 10th day, the shoot tips were isolated for the 8, 10, and 12 h treatments. Samples were collected in triplicate and stored at −80 °C. The IAA and ABA contents were determined at the indicated treatment points depending on the tissue analysis results. Briefly, 0.5 g of shoot tips was placed in 20 mL 80% cold methanol (4 °C) ice cubes, ground in a homogeniser, and then transferred to a 50 mL centrifuge tube. Thereafter, the homogeniser was flushed with 10 mL 80% methanol. The supernatant was then collected and heated to 45 °C for dissolution in the mobile phase. Next, 1 mL of supernatant was filtered through an organic phase membrane filter (0.22 μm). This was repeated thrice for each sample.
RNA samples, total RNA extraction, and cDNA library construction and sequencing
Scale, transitional, and compound leaves were removed, and samples of approximately 1 cm in length were taken from the shoot tips ((b)). Each treatment consisted of 5–10 shoot tips. Treatment groups included day 0 of the day length treatment as the control (CK) and the day length treatments of 8, 10, and 12 h at the same time point for the IAA and ABA analysis. After treatment, samples were placed in aluminium foil and stored at a temperature lower than −80 °C.
The TRIzol method (Invitrogen, Carlsbad, CA, USA) was used to separate and purify the RNA from the total sample. A NanoDrop ND-1000 spectrophotometer (Thermo Fisher Scientific, Wilmington, DE, USA) was used to perform quality control checks on the purification of the total RNA. The integrity of the RNA was then tested using an Agilent 2100 Bioanalyzer System (Agilent Technologies, Santa Clara, CA, USA), and RIN > 7.0 was used as the criterion to suggest integrity. The original data were stored in the NCBI database with registration no. PRJNA725601.
Beginning with a 5 µg total RNA sample, oligo (dT) magnetic beads were used to capture mRNA with polyadenylic acid (PolyA) through two rounds of purification. Subsequently, the captured mRNA was fragmented under high-temperature conditions using divalent cations. Thereafter, the fragmented RNA was reverse transcribed into cDNA using reverse transcriptase. Next, Escherichia coli DNA polymerase I was used with RNase H to convert these complex duplexes of DNA and RNA into DNA duplexes while adding dUTP to the DNA duplexes to supplement and flatten the ends of the duplex DNA fragments. Afterward, an A base was added to each end to allow it to be ligated to the connector with a T end. Next, single- or dual-index adapters were ligated to the fragments, and size selection was conducted using AMPureXP beads. UDG enzymes were then used to digest the duplexes, and PCR was run to create a library with a fragment size of 300 bp (±50 bp). Finally, we used a standard procedure with an Illumina Hiseq 4000 System (Illumina, San Diego, CA, USA) to perform double-ended with a read length of 150 bp.
RNA-seq data analysis
Perl scripts were used for library assembly. The raw reads were filtered by removing adapter sequences, reads containing poly-N sequences, and low-quality sequences. Clean reads were de novo assembled using Trinity (Grabherr et al. Citation2011), and the transcriptome reference database was obtained. FPKM was used to obtain the relative expression levels (Mortazavi et al. Citation2008). The DESeq R package was used for the differential expression analysis between groups. The resulting P-values were adjusted using Benjamini and Hochberg’s approach for controlling the false discovery rate.
The differentially expressed genes (DEGs) were identified with a |fold change ≥1.5 and false discover rate < 0.05 between each comparison. The Mercator web tool was used to annotate the DEGs (Lohse et al. Citation2014). The results were then loaded to MapMan software for functional enrichment analysis (Thimm et al. Citation2004). Subsequently, Gene Ontology (GO) and Kyoto Encyclopaedia of Genes and Genomics (KEGG) pathway analyses were performed (Moriya et al. Citation2007; Young et al. Citation2010). Venn diagrams and hierarchical clustering heat maps were generated using gmodels, Venn diagram, and Pheatmap packages in R based on the gene list and gene expression levels for each tissue type. To identify the candidate genes underlying the number of nodes, the DEGs found in different regions of Venn diagrams were selected depending on the analysis results of phenotypes under different day length treatments.
qRT-PCR
The transcript abundance of selected genes was analyzed using qRT-PCR to validate the RNA sequencing results (Ma CW et al. Citation2015). Total RNA was extracted using Trizol, and cDNA was synthesised using a TUREscript 1st Stand cDNA Synthesis Kit (AidLab Biotechnologies Co., Ltd., Beijing, China; 1 μg total RNA, 4 μL 5× RT Reaction Mix, 1 μL Rondam primer/oligodT, 1 μL TUREscript H- RTase/RI Mix, and RNase Free dH2O up to 20 μL) according to the manufacturer’s instructions. Real-time PCR amplification reactions were performed in 94-well plates (Bio-Rad Laboratories, Hercules, CA, USA) in an Analytik-Jena-qTOWER2.2 Quantitative Real-Time PCR Thermal Cycler (Analytic Jena AG, Jena, Germany). Each PCR reaction mix was 10 μL and included 5 μL 2× SYBR® Green Master Mix (DBI), 1 μL cDNA template (100 ng), 0.5 μL forward primer (10 μm), 0.5 μL reverse primer (10 μm), and 3 μL dH2O. The programme was conducted according to the three-step reaction procedure with pre-denaturation at 95 °C for 5 min, 40 cycles of 95 °C for 10 s, 55 °C for 20 s, and 72 °C for 30 s. Finally, a dissociation curve was generated by increasing the temperature from 65 to 95 °C to determine the specificity of reactions. The Ct value of each PCR well was automatically calculated using Analytik-Jena-q TOWER2.2 software. The RhUbi2 housekeeping gene was used as a standard, according to previous studies (Meng YLL et al. Citation2013; Zhang SZ et al. Citation2019). The primers used to determine transcript abundance are listed in Table S5.
Data analysis
The data were analyzed using SPSS 17.0 (SPSS Inc., Chicago, IL, USA), and data mapping was performed using GraphPad Prism 6.02 (GraphPad Software Inc., San Diego, CA, USA).
Results
Morphological characteristics of roses affected by the short shoot phenomenon in summer
The difference in shoot length between the two main local cultivars, ‘Carola’ and ‘Evening Glow’ in summer and autumn, is shown in . The shoot lengths of ‘Carola’ and ‘Evening Glow’ were significantly shorter in summer than those in autumn (p < 0.01 and p < 0.05, respectively; (a and b)). The results showed that the short shoot phenomenon in summer was ubiquitous in rose production and the difference in shoot length between summer and autumn was significantly greater in ‘Carola’ than that in ‘Evening Glow.’ Therefore, ‘Carola’ was considered to be a sensitive cultivar and selected to study the short shoot phenomenon further. Meteorological data showed that the day length in summer was longer than that in autumn during the 10 days following the bud burst of roses (Figure S1). These results support the theory that extended day lengths may be the environmental factor resulting in the short shoot phenomenon in summer.
Figure 2. Influence of summer and autumn on the shoot length of roses. (a) The shoot length of Rose cultivars ‘Carola’. (b) The shoot length of Rose cultivars ‘Evening Glow’. Significant differences are indicated at the level of p < 0.05 with lowercase letters. Highly significant differences are indicated with uppercase letters at p < 0.01. Error bars represent (SD). Three biological replicates were used (n = 10). PE, Petal primordium. ST, Stamen primordium.
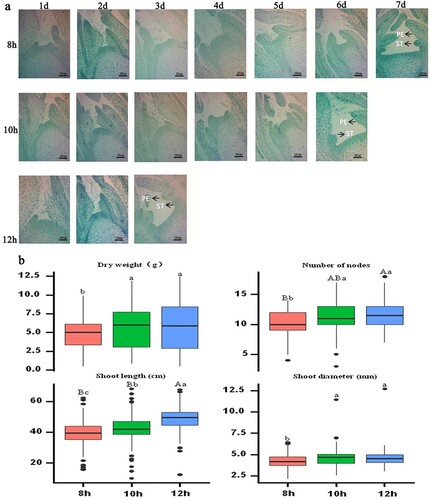
Interestingly, the number of nodes in both cultivars in autumn significantly increased compared with that in summer ((c, d)), implying a relationship between the short shoot phenomenon and the number of nodes in roses. To verify this relationship, we conducted a correlation analysis of node number and shoot length for the ‘Carola’ and ‘Evening Glow’ cultivars in summer (). The node numbers of ‘Carola’ and ‘Evening Glow’ were significantly correlated with shoot length (R2 = 0.583**, p = 0.001 and R2 = 0.365*, p = 0.047, respectively). Based on these results, we speculated that node development might be related to rose shoot length.
Table 1. Correlation coefficient of internode length, number of nodes and shoot length of rose in summer.
Effect of day length on morphological characteristics and microscopic observations of shoot development in roses in summer
The influence of day length on the growth of summer rose shoots is still unclear. We observed the shoot development of the rose cultivar ‘Carola’ under controlled conditions, including the stages from budding to harvest.
The microstructure of shoot development under different day length treatments is shown in (a). Node development in the 12 h treatment group occurred significantly earlier than that in the 8 and 10 h treatment groups, and the node number reached a maximum on the third day. However, the node number in the 8 h treatment group reached a maximum on the seventh day. The results showed that prolonging the day length could accelerate node development.
Figure 3. Effect of day length on shoot development of rose in summer. (a) Histological analysis of the shoot development of roses under day length treatment. PE: Petal primordium. ST: Stamen primordium. (b) Effect of day length on shoot of rose at harvest time. Significant differences are indicated at the level of p < 0.05 with lowercase letters. Highly significant differences are indicated with uppercase letters at p < 0.01. Error bars represent standard deviation (SD). Three biological replicates were used (n=30).
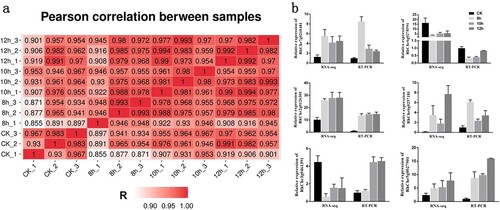
The effects of day length on rose shoot characteristics at the harvest stage are shown in (b). The shoot dry weight of all treatment groups was in the following order: 12 h > 10 h > 8 h, with a significant difference between the 12 and 8 h treatment groups (p < 0.05). The influence of day length on shoot length and node number was similar; that is, 12 h > 10 h > 8 h, with a significant difference between the 12 and 8 h treatment groups (p < 0.01). The effect of day length on shoot diameter significantly differed between the 8 and 12 h treatment groups (p < 0.05).
The results indicated that day length played an important role in the short shoot phenomenon during summer in roses, and the number of nodes positively regulated the shoot length.
RNA-sequencing of rose shoot development by day length in summer
Based on the phenotype and paraffin microstructure results, the shoot tips were sampled on the third day in the 8, 10, and 12 h treatment groups, using day zero samples as the control group. The samples were sequenced to reveal the molecular mechanism of node development. Total reads from the RNA sequencing of each library ranged from 6.73–8.27 G with total valid data from 6.64–8.16 G (Table S1). Effective reads accounted for 97.94% to 98.74%, Q20% was 99.99%, Q30% was above 97.95%, and GC content accounted for more than 45.5% of all reads.
To confirm the reliability of the RNA-seq data, we performed a Pearson’s correlation coefficient analysis of transcriptome profiles between the 12 libraries and biological copies, and the results indicated that the RNA sequence datasets were highly correlated ((a)). To further validate the expression profiles of the RNA-seq data, we randomly selected six transcripts for qRT-PCR analysis. The results showed a general consistency with the expression profiles obtained by RNA-seq, indicating that the RNA-seq results were reliable ((b)).
Figure 4. Validation of transcriptome data. (a) Pearson correlation between 12 samples. (b) Relative expression of the following six selected genes was determined qRT-PCR for validation of RNA-seq results: RhChr7g0210481, auxin-induced protein 15A; RhChr6g0278591, monooxygenase 3-like; Rhchr2g0126301, ethylene-responsive transcription factor ERF054; RhChr6g0257181, ethylene-responsive transcription factor ERF109; RhChr3g0461391, hypothetical protein; RhChr5g0027901, 9-cis-epoxycarotenoid dioxygenase NCED1. RhUbi2 was used as reference gene. Error bars represent SD. Three biological replicates were performed.
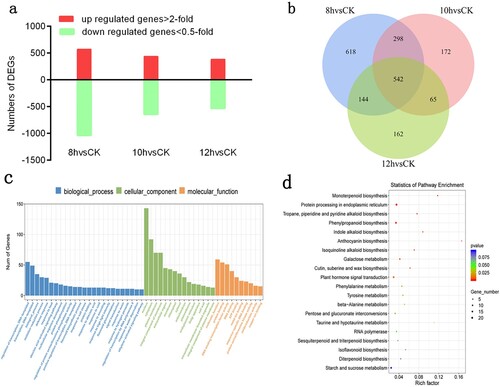
Dynamic transcriptome of shoot development related to day length in summer
In the transcriptomic data, we used the difference multiplier FC ≥ 2 or FC ≤ 0.5 (absolute value of log2FC ≥ 1) as the change threshold and p-value < 0.05 as the criteria for screening DEGs in the set comparison group. A total of 3,592 DEGs were identified in the 8, 10, and 12 h and CK groups. The comparison between the 8 h and CK groups revealed 569 upregulated DEGs and 1,032 downregulated DEGs, while that between the 10 h and CK groups indicated 437 upregulated DEGs and 640 downregulated DEGs. In addition, the comparison between the 12-h and CK groups indicated 382 upregulated DEGs and 531 downregulated DEGs ((a)). The results showed that the number of DEGs decreased with increasing day length.
Figure 5. Numbers of DEGs between day length treatment and corresponding GO and KEGG enrichment data. (a) Numbers of up-regulated and down-regulated genes identified from comparison of 8 h vs CK, 10 h vs CK and 12 h vs CK. (b) Venn diagram of the data in A. (c) GO function enrichment analysis of DEGs. (d) KEGG pathway enrichment analysis of DEGs.
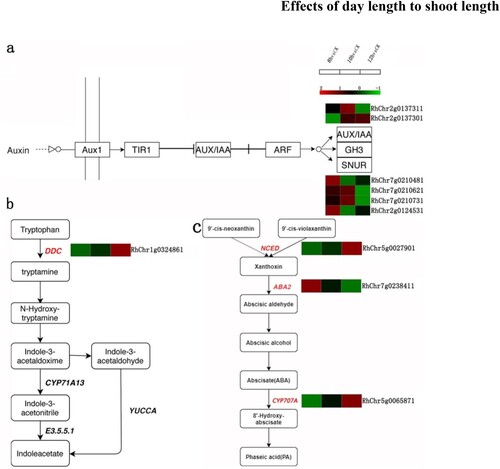
In addition to the morphological differences of the influence of day length on rose shoot development, we focused on the difference in gene expression between the 8 and 12 h treatment groups, and a total of 686 DEGs were identified from the sprouting to budding stages ((b)). To assess the effect of DEGs on shoot node number development, GO analysis was performed, and the results showed enrichment in 24 pathways. More significantly GO-enriched signalling pathways were activated by abscisic acid, multicellular biogenesis, flavonoid biosynthetic processes, responses to abscisic acid, high light intensity, and growth hormones, flavonoid glucuronidation, and signalling pathways activated by ethylene ((c)). In addition, DEGs were classified as genes enriched in 20 pathways found in the KEGG database. These results indicated that phytohormone signalling and starch and sucrose metabolism-related genes were significantly enriched in the identified DEGs ((d)).
Abscisic acid and auxin are related to rose shoot development in summer
We focused on 686 genes to investigate the regulation of plant hormones in shoot number development. Of the 686 genes, 94 hormone-related DEGs were identified. These DEGs involved abscisic acid, auxin, ethylene, jasmonic acid, oleuropein lactone, salicylic acid, gibberellin, and cytokinin. The two hormones regulated by most DEGs were abscisic acid and auxin (Figure S2a). There were 34 abscisic acid DEGs (Table S2) distributed in 9 GO-functionally enriched pathways (Figure S2b) and 15 auxin DEGs (Table S3) distributed in 5 GO-functionally enriched pathways (Figure S2c). The sum of the two accounted for more than 50% of the total hormone DEGs. Therefore, we hypothesised that abscisic acid and auxin could influence rose shoot development
To clarify the functions of abscisic acid- and auxin-related differential genes, we mapped the abscisic acid synthesis pathway, auxin signal transduction pathway, and synthesis pathway (). In the auxin signalling pathway, significant differences were found in AUX/IAA (RhChr2g0137311, RhChr2g0137301) and SNUR (RhChr7g0210481, RhChr7g0210621, RhChr7g0210731, RhChr2g0124531). AUX/IAA (RhChr2g0137301) was upregulated at 12 h relative to that at 8 h. SNUR (RhChr7g0210621, RhChr7g0210731) was downregulated at 12 h relative to that at 8 h, and RhChr7g0210481, RhChr2g0124531 was downregulated at 12 h relative to that at 8 h ((a)). Interestingly, in the auxin synthesis pathway, DDC (RhChr1g0324861) was found to be significantly upregulated at 12 h relative to that at 8 h (, b). With respect to the shedding acid synthesis pathways NCED (RhChr5g0027901), ABA2 (RhChr7g0238411), and CYP707A (RhChr5g0065871) were all significantly differentially expressed when comparing the 8 and 12 h treatment groups ((c)).
Figure 6. Heat maps of DEGs in the signal transduction and synthesis pathways of plant hormones involved in the development of shoot under day length treatment. Red and green colours indicate up- and down- regulated transcripts, respectively, from the three comparisons (log2 fold change). (a) Auxin signal transduction pathway related gene expression map. AUX/IAA: RhChr2g0137311; RhChr2g0137301. SNUR: RhChr7g0210481, RhChr7g0210621, RhChr7g0210731, RhChr2g0124531. (b) Gene expression map of auxin synthesis pathway. DDC: RhChr1g0324861. (c) Gene expression map of abscisic acid synthesis pathway. NCED: RhChr5g0027901; ABA2: RhChr7g0238411; CYP707A: RhChr5g0065871.
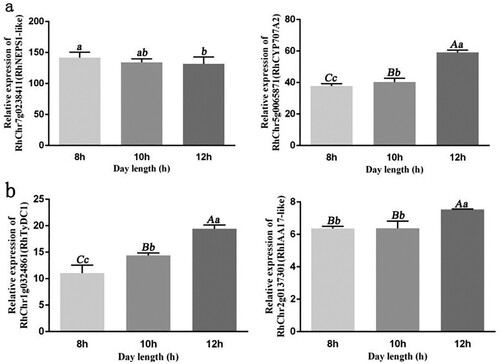
To further test our hypothesis, qRT-PCR was used to determine the expression of genes related to abscisic acid and auxin under 8, 10, and 12 h periods of day length. The results indicated a negative correlation between the expression of abscisic acid synthesis pathway gene RhChr7g0238411 (RhNEPS1-like) and day length, with a significant difference between day lengths of 8 and 12 h. In contrast, there was a positive correlation between the expression of the abscisic acid decomposition gene RhChr5g0065871 (RhCYP707A2) and day length. Furthermore, there were significant differences between day lengths of 8, 10, and 12 h ((a)). The expression of the auxin biosynthesis pathway gene RhChr1g0324861 (RhTyDC1) positively correlated with day length, with significant differences between the treatments with day lengths of 8, 10, and 12 h. Similarly, the expression of the auxin signal transduction gene RhChr2g0137301 (RhIAA17-like) also positively correlated with day length, with significant differences between day lengths of 8 and 12 h ((b)). The results showed that auxin and abscisic acid were important hormones for regulating rose shoot development.
Figure 7. qRT-PCR analysis of abscisic acid and auxin related genes related to shoot node number development. (a) Abscisic acid synthesis related genes RhChr7g0238411 (RhNEPS1-like) and RhCr5g0065871 (RhCYP707A2). (b) Auxin synthesis gene RhChr1g0324861 (RhTyDC1) and auxin signal transduction gene RhChr2g0137301 (RhIAA17-like). Significant differences are indicated at the level of p < 0.05 with lowercase letters. Highly significant differences are indicated with uppercase letters at p < 0.01. Error bars represent standard deviation (SD). Three biological replicates were used (n=3).
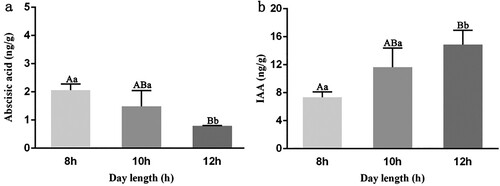
To verify the effect of day length on abscisic acid and IAA levels in rose plants, the content of endogenous IAA and abscisic acid in shoot tips on the third day after treatment was determined. Determining abscisic acid and IAA levels at the shoot tips indicated a change in the gradient for abscisic acid between treatment groups, with day length being negatively correlated with abscisic acid levels. The differences in the abscisic acid levels between the 8, 10, and 12 h treatment groups were significant ((a)). Day length was positively correlated with IAA levels, with significant differences between the 8, 10, and 12 h treatment groups ((b)). The results showed that the abscisic acid content in shoots decreased with increasing day length, while the auxin content increased.
Figure 8. Determination of hormone content in rose shoots by day length. (a) Abscisic acid content of shoot tip. (b) IAA content of shoot tip. Significant differences are indicated at the level of p < 0.05 with lowercase letters. Highly significant differences are indicated with uppercase letters at p < 0.01. Error bars represent standard deviation (SD). Three biological replicates were used (n=3).
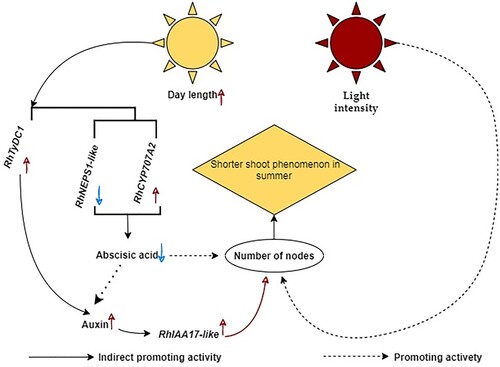
Discussion
Short shoot phenomenon in summer is a physiological disorder with high frequency in roses
Seasonal differences in shoot length are common for roses production globally. By observing the shoot length of tetraploid roses in Kenyan summer and winter periods, Gitonga et al. (Citation2014) concluded that the shoot length in winter was significantly longer than that in summer. However, in an experiment conducted by In and Lim (Citation2018) using the rose cultivar ‘Lovely Lydia’ in South Korea, shoot length was observed to be higher in summer than that in winter. The present study also proved that the shoot length of cut roses was shorter in summer than that in autumn in Heilongjiang Province, China (). However, we found that node number was an important parameter that affected the summer shoot length ().
Most studies on the seasonal differences of cut rose shoots focus on the effects of temperature and day length (Plaut and Zieslin Citation1977; Plaut et al. Citation1979; Triozzi PM et al. Citation2018). Previous studies have shown that during summer in Korea, the temperature can be lowered by mist treatment, resulting in a 10–20% increase in cut rose shoot length (Chon et al. Citation2013; Lee et al. Citation2020). Nevertheless, the increase in humidity can mask the effect of temperature as the main factor for seasonal differences. Harada and Komagata (Citation2014) and Shi et al. (Citation2018) applied supplementary light to extend the day length, and as a result, shoot length also increased. Our research has been revised based on previous research, and day length was processed under summer climatic conditions. The results further showed that the day length was related to the short shoot phenomenon in summer.
Day length is involved in the regulation of the short shoot phenomenon in summer
The present study indicated that increasing the day length could increase the rose shoot length during summer ((b)), consistent with previous studies. For example, Moe (Citation1971) reported that increasing the day length could promote first flowering and shoot growth in roses. At present, the mechanism by which external factors affect the node number and internode length in roses remains unclear (De Vries and Smeets Citation1979). Our research shows that the day length is positively correlated with the number of nodes in cut roses during summer ((b)), indicating that the short shoot phenomenon in roses during summer is related to day length, which regulates the number of nodes, consequently affecting shoot length.
In the current study, the transcriptome analysis of summer rose shoots affected by day length showed that the GO-enriched signalling pathways of DEGs, including ‘signal pathway activated by abscisic acid,’ ‘response to abscisic acid,’ and ‘response to auxin’ have significant effects on the node number ((c)). Interestingly, the regulation of abscisic acid and auxin involved in floral transition has been reported by Guo et al. (Citation2017) for the transcriptome of the analysis of rose floral transition (Guo et al. Citation2017). Furthermore, prior studies have revealed that the ABA signalling pathway is a key factor for inducing floral transition in apples (Foster et al. Citation2003; Xing et al. Citation2015). However, when we analyzed the transcriptome, we selected the DEGs between 12 and 8 h day lengths ((b)), but when combined with the findings of Guo et al. (Citation2017), our results showed that some genes simultaneously regulated the node number growth and flower transition.
Other environmental factors are also involved in regulating the short shoot phenomenon during summer in roses. We showed that the number of nodes reached a maximum on the third day of treatment, with a day length of 12 h ((b)), and the number of nodes reached a maximum on the seventh day for plants experiencing a day length of 8 h ((a)). This result is consistent with a previous study where all leaves and flower buds had formed approximately 10 days after the axillary bud germination of the rose (Acker Citation1993). According to the analysis of day length data after 10 days of axillary bud germination in summer and autumn (Figure S1), the extension of day length may be one of the most important environmental factors resulting in the short shoot phenomenon in summer. However, our results show that extending the day length in summer promoted rose shoot growth ((b)). This indicates that the short shoot phenomenon in summer is affected not only by day length but also by other environmental factors. For example, reducing indoor temperatures through atomisation effectively increased rose shoot length during summer (In and Lim Citation2018). A similar method has been used in rose production in Korea (Chon et al. Citation2013; Lee et al. Citation2020). Nevertheless, lowering the temperature also increases the air humidity, so it is unknown whether temperature and humidity have interactive effects on shoot length in summer.
Abscisic acid and auxin are involved in regulating the short shoot phenomenon in summer
We found that abscisic acid-related genes were the most common hormone-related genes associated with day length extension (Figure S2a); these genes included RhChr5g0027901 (RhChrNCED1) and RhChr7g0238411 (RhNEPS1-like), indicating that the shoot tip might have the ability to synthesise abscisic acid. Previous studies have also shown that abscisic acid in angiosperms is synthesised in leaves rather than in roots (Xiong et al. Citation2001). qRT-PCR confirmed that the gene RhChr7g0238411 (RhNEPS1-like), which is related to the abscisic acid synthesis pathway, was negatively correlated with day length, and the abscisic acid decomposition gene RhChr5g0065871 (RhChrCYP707A2) was positively correlated with day length ((c)). In addition, the content of endogenous abscisic acid was negatively correlated with day length, showing significant differences among all day length treatments ((a)), consistent with the gene expression trend – this indicated that abscisic acid was correlated with day length. Bittner et al. (Citation2001) also found that abscisic acid was involved in the effect of seasonal day length on plant growth. Therefore, abscisic acid plays an important role in summer shoot development.
Previous studies have demonstrated that endogenous abscisic acid might play a positive role in shoot growth (Sharp et al. Citation2000; Cheng et al. Citation2002), providing evidence that abscisic acid directly affects node development. Interestingly, another study identified four AO genes from Arabidopsis thaliana and verified that Arabidopsis aldehyde oxidase 1 (AAO1) can transform IAAld into IAA (Seo et al. Citation1998). Furthermore, Xiong et al. (Citation2001) and Bittner et al. (Citation2001) showed that in the AO family, molybdenum cofactor sulfidase is required to exert its activity, whereas molybdenum cofactor sulfurase was encoded by ABA DEFICIENT 3 (ABA3). Other studies also showed that the main role of abscisic acid is to adjust plant growth through an enhanced auxin signal (Belin et al. Citation2009). Recently, the potential molecular mechanism of ABA-AUXIN crosstalk has been revealed. Previous studies have demonstrated that abscisic acid-induced ARF2 acts as a transcriptional inhibitor of the homologous domain gene HB33. The decrease in ARF2 or increase in HB33 expression leads to the oversensitivity of root growth to abscisic acid (Wang et al. Citation2011). This provides evidence for an indirect effect of abscisic acid on the number of nodes by acting on auxin.
The biosynthesis pathway of rose auxin was obtained through the KEGG database ((b)). Previous studies have shown that L-Trp-dependent IAA biosynthesis is the main pathway for plant IAA biosynthesis (Ouyang et al. Citation2000). However, in the synthetic pathway, indole-3-acetaldoxime has two pathways: one to synthesise indole-3-acetaldehyde and then indoleacetate, while the other synthesises indole-3-acetonitrile and then indoleacetate. The specific pathway could not be determined because there were no comments in the transcript group data. Interestingly, the expression of the DDC (RhChr1g0324861) gene was upregulated at 12 h compared with that at 8 h ((b)). Therefore, it was inferred that the content of auxin in shoots after a day length of 12 h was higher than that for the 8 h treatment group. The measurement of endogenous hormones also confirmed this inference ((b)). The results were consistent with previous studies, particularly with Chen et al. (Citation2013), who found a positive feedback loop between auxin and its transporter PIN-FORMED1 (PIN1)-induced leaf priming in A. thaliana. Braybrook and Kuhlemeier (Citation2010) have also shown that the occurrence of leaf primordia depends on the formation of the maximum amount of auxin at the starting point in A. thaliana. The results showed that auxin affected shoot development by regulating the number of nodes.
As changing day length can lead to differences in rose shoot length, abscisic acid plays an important role in regulating shoot development. It has been demonstrated that exogenous abscisic acid is important for plant growth and development (Xiong et al. Citation2001; Ahmed Khadr et al. Citation2020). Using a combination of hormone and transcriptome analyses, the key genes RhChr7g0238411 (RhNEPS1-like) and RhCr5g0065871 (RhCYP707A2) were identified for the regulation of rose shoot length. At the same time, auxin-related genes RhChr1g0324861 (RhTyDC1) and RhChr2g0137301 (RhIAA17-like) may cooperate with abscisic acid to regulate shoot development. The genes and hormones that we identified in the present study can be used for further research for rose development.
The short shoot phenomenon in roses during summer may be related to node development (). RNA-seq analysis shows that abscisic acid and auxin may be related to the development of nodes (leaf) (Figure S2a), while the auxin concentration is positively correlated with node number (leaf number). Studies in Arabidopsis have shown that the occurrence of leaf primordium depends on the formation of the maximum amount of auxin at the starting point (Braybrook and Kuhlemeier Citation2010). Furthermore, several studies have identified growth vitamins to be beneficial for the elongation of plant shoots; for example, in rice (Chen et al. Citation2012), sorghum (Hilley et al. Citation2017), tomato (Ohtaka et al. Citation2020), and cowpea (Sharma et al. Citation2020). Abscisic acid often negatively correlates with plant shoot length, such as in Arabidopsis (Li et al. Citation2019) and tomato (Martinez-Andujar et al. Citation2021), but no relevant research has been conducted on the relationship of plant leaf development with abscisic acid. Abscisic acid may indirectly regulate rose shoot length by affecting auxin. Studies have shown that auxin regulates growth through cell elongation and division, and it is logical that auxin acts downstream of abscisic acid to regulate the growth process (Emenecker and Strader Citation2020). Therefore, we speculate that auxin positively regulates rose shoot length during summer, while abscisic acid plays a negative regulatory role.
Here, we propose a model mechanism for the regulation of shoot development in cultivated roses by day length during summer (). Day length regulated the expression of the abscisic acid synthesis gene, and the auxin synthesis gene affected the content of abscisic acid and auxin in shoots through positive effects on the number of nodes, negatively affecting the short shoot phenomenon of cut roses in summer. However, the two hormones have different effects on node number. Auxin affects node number development through a signal transduction pathway; however, it is unclear whether abscisic acid directly affects node number or affects it through interactions with auxin.
Supplemental Material
Download MS Word (410.5 KB)Acknowledgment
Thanks to Lianchuan Biotechnology Company for providing sequencing services.
Disclosure statement
No potential conflict of interest was reported by the author(s).
Additional information
Funding
Notes on contributors
Li Wang
Li Wang is a Ph.D student in the College of Horticulture at Northeast Agricultural.
Wan Jiang
Wan Jiang is a Ph.D student in the College of Horticulture at Northeast Agricultural.
Wuhua Zhang
Wuhua Zhang is a Ph.D student in the College of Horticulture at Northeast Agricultural.
Weitong Chen
Weitong Chen is a graduate student in the College of Horticulture at Northeast Agricultural.
Yu Mo
Yu Mo is a graduate student in the College of Horticulture at Northeast Agricultural.
Tong Zhou
Tong Zhou is a graduate student in the College of Horticulture at Northeast Agricultural.
Jinzhu Zhang
Jinzhu Zhang is a research associate in the College of Horticulture at Northeast Agricultural.
Daidi Che
Daidi Che is a Professor of ornamental plants breeding in the College of Horticulture at Northeast Agricultural.
References
- Acker C-v. 1993. Ontogeny of axillary buds and shoots in roses: leaf initiation and pith development. Sci Hortic. 57(1-2):111–122.
- Ahmed Khadr YW, Que F, Li T, Xu Z, Xiong A. 2020. Exogenous abscisic acid suppresses the lignification and changes the growth, root anatomical structure and related gene profiles of carrot. Acta Biochim Biophys Sin. 52(1):97–100.
- Belin C, Megies C, Hauserova E, Lopez-Molina L. 2009. Abscisic acid represses growth of the Arabidopsis embryonic axis after germination by enhancing auxin signaling. Plant Cell. 21(8):2253–2268.
- Bittner F, Oreb M, Mendel RR. 2001. ABA3 is a molybdenum cofactor sulfurase required for activation of aldehyde oxidase and xanthine dehydrogenase in Arabidopsis thaliana. J Biol Chem. 276(44):40381–40384.
- Braybrook SA, Kuhlemeier C. 2010. How a plant builds leaves. Plant Cell. 22(4):1006–1018.
- Chen MK, Wilson RL, Palme K, Ditengou FA, Shpak ED. 2013. ERECTA family genes regulate auxin transport in the shoot apical meristem and forming leaf primordia. Plant Physiol. 162(4):1978–1991.
- Chen ML, Huang YQ, Liu JQ, Yuan BF, Feng YQ. 2011. Highly sensitive profiling assay of acidic plant hormones using a novel mass probe by capillary electrophoresis-time of flight-mass spectrometry. J Chromatogr B. 879(13-14):938–944.
- Chen Y, Fan X, Song W, Zhang Y, Xu G. 2012. Over-expression of OsPIN2 leads to increased tiller numbers, angle and shorter plant height through suppression of OsLAZY1. Plant Biotechnol J. 10(2):139–149.
- Cheng WH, Endo A, Zhou L, Penney J, Chen HC, Arroyo A, Leon P, Nambara E, Asami T, Seo M, et al. 2002. A unique short-chain dehydrogenase/reductase in Arabidopsis glucose signaling and abscisic acid biosynthesis and functions. Plant Cell. 14(11):2723–2743.
- Chon YS, Ha SH, Jeong KJ, Choi KO, Yun JG. 2013. Effect of mist treatment on the growth and quality of Cut rose ‘hanmaum’during summer. Korean J Hortic Sci Technol. 31(5):538–543.
- De Vries DP, Smeets L. 1979. Effects of temperature on growth and development of hybrid tea-rose seedlings. Sci Hortic. 11(3):261–268.
- Djennane S, Hibrand-Saint Oyant L, Kawamura K, Lalanne D, Laffaire M, Thouroude T, Chalain S, Sakr S, Boumaza R, Foucher F, et al. 2014. Impacts of light and temperature on shoot branching gradient and expression of strigolactone synthesis and signalling genes in rose. Plant Cell Environ. 37(3):742–757.
- Emenecker RJ, Strader LC. 2020. Auxin-Abscisic acid interactions in plant growth and development. Biomolecules. 10(2):281.
- Foster T, Johnston R, Seleznyova A. 2003. A morphological and quantitative characterization of early floral development in apple (Malus x domestica borkh.). Ann Bot. 92(2):199–206.
- Gitonga VW, Koning-Boucoiran CF, Verlinden K, Dolstra O, Visser RG, Maliepaard C, Krens FA. 2014. Genetic variation, heritability and genotype by environment interaction of morphological traits in a tetraploid rose population. BMC Genet. 15(1):1–14.
- Grabherr MG, Haas BJ, Yassour M, Levin JZ, Thompson DA, Amit I, Adiconis X, Fan L, Raychowdhury R, Zeng Q, et al. 2011. Full-length transcriptome assembly from RNA-Seq data without a reference genome. Nat Biotechnol. 29(7):644–652.
- Guo X, Yu C, Luo L, Wan H, Zhen N, Xu T, Tan J, Pan H, Zhang Q. 2017. Transcriptome of the floral transition in Rosa chinensis ‘Old blush’. BMC Genomics. 23. 18(1):199.
- Halevy AH. 1986. Rose research - current situation and future needs. Acta Hortic. 189(1986):11–20.
- Harada T, Komagata T. 2014. Effects of long-Day treatment using fluorescent lamps and supplemental lighting using white LEDs on the yield of Cut rose flowers. Jpn Agric Res Q. 48(4):443–448.
- Hilley JL, Weers BD, Truong SK, McCormick RF, Mattison AJ, McKinley BA, Morishige DT, Mullet JE. 2017. Sorghum Dw2 encodes a protein kinase regulator of stem internode length. Sci Rep. 7(1):4616.
- Horridge JS, Cockshull KE. 1974. Flower initiation and development in the glasshouse rose. Sci Hortic. 2(3):273–284.
- In BC, Lim JH. 2018. Potential vase life of cut roses: seasonal variation and relationships with growth conditions, phenotypes, and gene expressions. Postharvest Biol Technol. 135(2018):93–103.
- Jing W, Zhang S, Fan Y, Deng Y, Wang C, Lu J, Sun X, Ma N, Shahid MO, Li Y, et al. 2020. Molecular evidences for the interactions of auxin, gibberellin, and Cytokinin in bent peduncle phenomenon in rose (Rosa sp.). Int J Mol Sci. 21(4):1360.
- Kojima M, Kamada-Nobusada T, Komatsu H, Takei K, Kuroha T, Mizutani M, Ashikari M, Ueguchi-Tanaka M, Matsuoka M, Suzuki K, et al. 2009. Highly sensitive and high-throughput analysis of plant hormones using MS-probe modification and liquid chromatography-tandem mass spectrometry: an application for hormone profiling in oryza sativa. Plant Cell Physiol. 50(7):1201–1214.
- Lee JH, Yoon JW, Oh SI, Lee AK. 2020. Relationship between cultivation environment and Postharvest Quality of Cut Rose ‘Lovely lydia’. Hortic Sci Technol. 38(2):263–270.
- Leiv M, Mortensen RM. 1991. Effects of CO2 enrichment and different day/night temperature combinations on growth and flowering of Rosa L. and kalanchoe blossfeldiana v. poelln. elsevier. Science Publishers BV. 51(1-2):145–153.
- Li X, Wu L, Qiu Y, Wang T, Zhou Q, Zhang Q, Zhang W, Liu Z. 2019. Abscisic acid receptors modulate Metabolite levels and phenotype in Arabidopsis under normal growing conditions. Metabolites. 9(11):249.
- Lilley JL, Gee CW, Sairanen I, Ljung K, Nemhauser JL. 2012. An endogenous carbon-sensing pathway triggers increased auxin flux and hypocotyl elongation. Plant Physiol. 160(4):2261–2270.
- Liu W, Liu G, Yang Y, Guo X, Ming B, Xie R, Liu Y, Wang K, Hou P, Li S. 2021. Spatial variation of maize height morphological traits for the same cultivars at a large agroecological scale. Eur J Agron. 130(2021):126349.
- Lohse MNA, Herter T, May P, Schroda M, Zrenner R, Tohge T, Fernie AR, Stitt M, Usadel B. 2014. Mercator: a fast and simple web server for genome scale functional annotation of plant sequence data. Plant Cell Environ. 37(5):1250–1258.
- Ma CW H, Macnish AJ, Estrada-Melo AC, Lin J, Chang YH, Reid MS, Jiang CZ. 2015. Transcriptomic analysis reveals numerous diverse protein kinases and transcription factors involved in desiccation tolerance in the resurrection plant myrothamnus flabellifolia. Hortic Res. 2(2015):15034.
- Martinez-Andujar C, Martinez-Perez A, Albacete A, Martinez-Melgarejo PA, Dodd IC, Thompson AJ, Mohareb F, Estelles-Lopez L, Kevei Z, Ferrandez-Ayela A, et al. 2021. Overproduction of ABA in rootstocks alleviates salinity stress in tomato shoots. Plant Cell Environ. 44(9):2966–2986.
- Meng YLL N, Tian J, Gao JP, Zhang CQ. 2013. Identification and validation of reference genes for gene expression studies in postharvest rose flower (Rosa hybrida). Sci Hortic. 158(2013):16–21.
- Moe R. 1971. The relationship between flower abortion and endogenous auxin content of rose shoots. Physiol Plant. 24(3):374–379.
- Moe R, Kristoffersen T. 1969. The effect of temperature and light on growth and flowering of rosa‘Baccara’in greenhouses. Int Soc Hortic Sci. 1969(14):157–166.
- Moriya Y, Itoh M, Okuda S, Yoshizawa AC, Kanehisa M. 2007. KAAS: an automatic genome annotation and pathway reconstruction server. Nucleic Acids Res. 35(2007):W182–W185.
- Mortazavi A, Williams BA, McCue K, Schaeffer L, Wold B. 2008. Mapping and quantifying mammalian transcriptomes by RNA-Seq. Nat Methods. 5(7):621–628.
- Niu G, Rodriguez DS. 2009. Growth and physiological responses of four rose rootstocks to drought stress. J Amersochortsci. 134(2):202–209.
- Ohtaka K, Yoshida A, Kakei Y, Fukui K, Kojima M, Takebayashi Y, Yano K, Imanishi S, Sakakibara H. 2020. Difference between Day and night temperatures affects stem elongation in tomato (solanum lycopersicum) seedlings via regulation of gibberellin and auxin synthesis. Front Plant Sci. 11(2020):577235.
- Ouyang J, Shao X, Li J. 2000. Indole-3-glycerol phosphate, a branchpoint of indole-3-acetic acid biosynthesis from the tryptophan biosynthetic pathway in Arabidopsis thaliana. Plant J. 24(3):327–333.
- Plaut Z, Zieslin N. 1977. The effect of canopy wetting on plant water status, CO2 fixation, Ion content and growth rate of ‘Baccara’ roses. Physiol Plant. 39(4):317–322.
- Plaut Z, Zieslin N, Grawa A, Gazit M. 1979. The response of rose plants to evaporative cooling: flower production and quality. Sci Hortic. 11(2):183–190.
- Roman H, Girault T, Barbier F, Peron T, Brouard N, Pencik A, Novak O, Vian A, Sakr S, Lothier J, et al. 2016. Cytokinins Are initial targets of light in the control of Bud outgrowth. Plant Physiol. 172(1):489–509.
- Sairanen I, Novák O, Pěnčík A, Ikeda Y, Jones B, Sandberg G, Ljung K. 2012. Soluble carbohydrates regulate auxin biosynthesis via PIF proteins in arabidopsis. Plant Cell. 24(12):4907–4916.
- Seo M, Akaba S, Oritani T, Delarue M, Bellini C, Caboche M, Koshiba T. 1998. Higher activity of an aldehyde oxidase in the auxin-overproducing superroot1 mutant of Arabidopsis thaliana. Plant Physiol. 116(2):687–693.
- Sharma P, Kumar V, Khosla R, Guleria P. 2020. Exogenous naringenin improved digestible protein accumulation and altered morphology Via VrPIN and auxin redistribution in vigna radiata. 3 Biotech. 10(10):431.
- Sharp RE, LeNoble ME, Else MA, Thorne ET, Gherardi F. 2000. Endogenous ABA maintains shoot growth in tomato independently of effects on plant water balance: evidence for an interaction with ethylene. J Exp Bot. 51(350):1575–1584.
- Shi L, Wang Z, Kim WS. 2018. Effect of drought stress on shoot growth and physiological response in the cut rose ‘charming black’ at different developmental stages. horticulture. Environ Biotechnol. 60(1):1–8.
- Shuai H, Meng Y, Luo X, Chen F, Zhou W, Dai Y, Qi Y, Du J, Yang F, Liu J, et al. 2017. Exogenous auxin represses soybean seed germination through decreasing the gibberellin/abscisic acid (GA/ABA) ratio. Sci Rep. 7(1):12620.
- Suzuki M, Kao CY, Cocciolone S, McCarty DR. 2001. Maize VP1 complements Arabidopsis abi3 and confers a novel ABA/auxin interaction in roots. Plant J. 28(4):409–418.
- Thimm O, Blasing O, Gibon Y, Nagel A, Meyer S, Kruger P, Selbig J, Muller LA, Rhee SY, Stitt M. 2004. MAPMAN: a user-driven tool to display genomics data sets onto diagrams of metabolic pathways and other biological processes. Plant J. 37(6):914–939.
- Triozzi PM R-S, Hernandez-Verdeja T JM, Moreno-Cortes A, Allona I, Perales M. 2018. Photoperiodic regulation of shoot apical growth in poplar. Front Plant Sci. 9(5):1030.
- Wang L, Hua D, He J, Duan Y, Chen Z, Hong X, Gong Z. 2011. Auxin response Factor2 (ARF2) and its regulated homeodomain gene HB33 mediate abscisic acid response in arabidopsis. PLoS Genet. 7(7):e1002172.
- Wang Y, Yao R. 2020. Increased endogenous gibberellin level inhibits root growth of pinus massoniana lamb. plantlets during long-term subculture. In Vitro Cell Dev Bio - Plant. 56(4):470–479.
- Xing LB, Zhang D, Li YM, Shen YW, Zhao CP, Ma JJ, An N, Han MY. 2015. Transcription profiles reveal sugar and hormone signaling pathways mediating flower induction in apple (Malus domestica borkh.). Plant Cell Physiol. 2015(10):2052–2068.
- Xiong L, Ishitani M, Lee H, Zhu JK. 2001. The Arabidopsis LOS5/ABA3 locus encodes a molybdenum cofactor sulfurase and modulates cold stress- and osmotic stress-responsive gene expression. Plant Cell. 13(9):2063–2083.
- Young MD, Wakefield MJ, Smyth GK, Oshlack A. 2010. Gene ontology analysis for RNA-seq: accounting for selection bias. Genome Biol. 11(2):R14.
- Zhang N, Westreenen A, Evers JB, Anten NPR, Marcelis LFM. 2020. Quantifying the contribution of bent shoots to plant photosynthesis and biomass production of flower shoots in rose (Rosa hybrida) using a functional-structural plant model. Ann Bot. 126(4):587–599.
- Zhang SZ QC, Zeng DX, Xu JH, Zhou HG, Wang FL, Ma N, Li YH. 2019. RhMYB108, an R2R3-MYB transcription factor, is involved in ethylene- and JA-induced petal senescence in rose plants. Hortic Res. 6(2019):131.