ABSTRACT
Moisture stress is one of the most important constraints for crop production in arid regions. Cowpea is a vital food legume that has been cultivated in tropical and sub-tropical regions where water is scarce. Rhizobia inoculation confers resistance to water stress legumes. Two-year field experiments were conducted to assess the carbon assimilation and water use efficiencies of inoculated cowpea varieties at three sites. The treatments consist of four varieties and three levels of Bradyrhizobium inoculation arranged in a factorial randomized complete block design with four replications. The nitrogen (% N) and carbon (% C) concentrations in plant shoots were obtained directly from the mass spectrometric analysis. The results revealed considerable variation for shoot % N and % C, shoot growth, and δ13C among the varieties. Similarly, Bradyrhizobium significantly affected plant growth, % C, C and N contents, C/N ratio, and carbon isotope discrimination of the shoot. For instance, C and N contents and the C/N ratio increased by 28, 24, and 31%, respectively, due to Bradyrhizobium inoculation. In general, these results indicated that physiological performances such as carbon assimilation and water use efficiency of the crop could be, significantly improved when effective Bradyrhizobium strains and the best performing varieties are selected.
1. Introduction
Water stress represents the most important constraint for crop production in arid regions (Donohue et al. Citation2013), which is becoming a more pressing challenge during climate change (Salman et al. Citation2020). Therefore, the identification of crop varieties and agronomic practices that improve crop water use (WUE) with minimal yield reduction is particularly prominent in the context of crop production, as WUE is an important physiological parameter to assess the tradeoff between CO2 fixation and water loss (Wei et al. Citation2021). Moreover, WUE is useful to describe plant physiological responses to environmental variables, temperature, and water availability (Kooperman et al. Citation2018). Therefore, knowledge on crop WUE in response to climate change serves to improve climate predictions (Adams et al. Citation2020).
In this regard, cowpea can adapt and grow under rain-fed conditions in semiarid areas, where erratic and insufficient rain often reduces crop yield (Ismail and Hall Citation2000; Pottorff et al. Citation2012). In addition, it has the potential to fix atmospheric N, thereby improving soil fertility (Adjei-Nsiah et al. Citation2008). Therefore, cowpea is considered one of the most important crops under future climate change, with global warming and rainfall variability likely to occur, especially in the tropics (Tankari et al. Citation2019).
Cowpea is also considered among the major food security crops as it plays a pivotal role in saving lives in Africa and other developing countries (Tankari et al. Citation2019). It is characterized by having significant contents of proteins (23–32%) and carbohydrates (50–60%), fibers and vitamins, with low-fat content (1%), and bioactive compounds, such as phenols and polyamines (Moreira-Araújo et al. Citation2017). In addition to its high nutritional value, it contains an incredible source of other health-promoting components, such as soluble and insoluble dietary fiber, phenolic compounds, minerals, and many other functional compounds, including B group vitamins, and carotenoids (Bai et al. Citation2020). However, its production mostly depends on natural rainfall, which is low and erratic in nature, resulting in low and unstable yields (Makoi et al. Citation2012). Therefore, a better understanding of cowpea’s water use efficiency is essential for improving yield under future changing climate scenarios. This is because water use efficiency is a key trait to crop productivity in areas with limited water resources (Wright et al. Citation1994), and is an important factor in drought resilience (Ehdaie and Waines Citation1993).
Symbiotic fixation by rhizobia has huge importance in nutrient supply (Herridge and Herridge Citation2013). However, it is affected by soil water regimes (Serraj et al. Citation1999). Seed inoculation with tolerant rhizobia could increase the yields of legumes in contrast to the non-inoculated plants under water-deficit conditions (Yanni et al. Citation2016). On the other hand, the application of rhizobacteria and arbuscular mycorrhizal were reported to confer resistance to plants and reduce the effects of environmental stresses, including drought (Rocha et al. Citation2019). Similarly, Figueiredo et al. (Citation2008) and Bano et al. (Citation2014) reported that rhizobia inoculation could alleviate the drought stress on legume plants. Hence, the effectiveness of Rhizobium strains used largely affects the symbiotic efficiency of inoculants, and Bradyrhizobium species reported to have a capacity to resist drought or desiccation (Bano et al. Citation2014; Mouradi et al. Citation2016; Jemo et al. Citation2017).
However, how inoculation affects water use efficiency in legumes, including cowpea, is still scarce (Giller Citation2001). Therefore, investigating the impact of Bradyrhizobium inoculation on the water use efficiency of cowpea could deserve to be a topic of interest (Tankari et al. Citation2019). According to Farquhar and Richards (Citation1984) and Ehleringer et al. (Citation1991), δ13C ‰ carbon isotope discrimination is an important tool for determining water use efficiency in C3 plants. On top of the dependence of the existing knowledge of WUE on its relationship with photosynthetic 12C/13C isotope discrimination (Farquhar et al. Citation1989), the techniques explicitly describe isotopic fractionation associated with physical and biochemical processes during the photosynthetic gas exchange (Wei et al. Citation2021). As a result, it is now gaining acceptance as a potential future tool for screening and selecting crop species for higher water use efficiency and crop yields (Kumarasinghe et al. Citation1996; Wei et al. Citation2021).
Hall et al. (Citation1992) also reported the association between carbon isotope discrimination and water use efficiency response to drought. In line with this, a highly significant negative association between water use efficiency and carbon isotope discrimination was reported for cowpea, which could indicate the usefulness of carbon isotope discrimination as an indirect method of determining water use efficiency in cowpea (Ismail and Hall Citation1992). Moreover, isotope discrimination is a quick and scientific technique to select plant species with high water use efficiency (Li et al. Citation2019). In general, carbon isotopic discrimination (δ13C‰) provides important information for measuring water use efficiency (Barbour Citation2007). Therefore, the aim of this study was to assess the water use-efficiency of cowpea varieties using the carbon discrimination technique, and whether Bradyrhizobium inoculation could improve crop water use-efficiency in cowpea plants.
2. Materials and methods
2.1. Site description
Field experiments were conducted at Boricha, Dore, and Hawassa sites during the 2018 and 2019 main cropping season. The three sites are located (6°17′N and 38°04′E), (7°3′N and 38°28′E), and (7°3′N and 38°30′E), respectively, , presents the rainfall and temperature data of the sites. The areas had bimodal rainfall, with a short rainy season from March to May and a long rainy season from June to mid-October. These areas are experiencing climate change-related problems, including shortage and fluctuation in rainfall, and the population is vulnerable to food insecurity (Atara et al. Citation2019; Gezahegn Citation2018). However, these areas are suitable for legumes production (Fekadu Citation2013).
Table 1. Monthly rainfall and temperature of the sites during 2018 and 2019.
2.2. Seeds and inoculant sources
Bradyrhizobium strains were obtained from Hawassa University, Agriculture College. The strains were isolated from major cowpea growing areas and were subjected to authentication and characterization (Degefu et al. Citation2018). The symbiotic effectiveness of the strains was confirmed on a sand culture before field application (Ayalew and Yoseph Citation2020).
2.3. Soil physicochemical properties of study sites
Twenty soil samples were randomly taken from topsoil (0–30 cm depth) before sowing to establish the baseline soil properties for each site. The soil samples were pooled per site and thoroughly mixed, and the composite sample was air-dried in the laboratory and sieved (2.0 mm) for analysis. Soil samples were analyzed at HORTCOOP Soil and Water Analysis Laboratory, Addis Ababa, Ethiopia, and the result is presented in .
Table 2. Physicochemical properties of the soil at experimental sites.
The physico-chemical analyses indicated that the experimental sites had different soil chemical and textural properties (). Soil textures had clay loam in the Boricha and Hawassa sites and loam in the Dore site. The recorded pH values ranged from slightly acidic to neutral and were suitable for cowpea production (Onyibe et al. Citation2006). The soils were very low-to-low in organic carbon (OC) content and low to moderate in total nitrogen (TN) as per the limit set by Herrera (Citation2005) and Havlin et al. (Citation1999), respectively. Available phosphorus (Avail P) and cation exchange capacity (CEC) ranged from very low (at Dore) to high (at Hawassa) and low to medium, respectively. Exchangeable cations K, Ca, and Mg ranged from medium to high (FAO Citation2006). The site at Hawassa takes advantage of available P, CEC, and exchangeable cations.
2.4. Inoculation techniques
The seeds were inoculated with 10 g of peat-based inoculant per kg containing 6.5×108 viable bacterial cells g−1 peat of Bradyrhizobium strain CP-24 and CP-37. The seeds were inoculated under shade and air dry for some minutes prior to planting to maintain cells viability and avoid fungal growth, respectively (Rice et al. Citation2001). After sowing, the seeds are immediately covered with moist soil to prevent bacterial cell death due to desiccation. Ridges were made between plots and blocks to prevent bacterial movement through rainwater
2.5. Experimental design and procedures
Seeds were sown in a Randomized Complete Block Design with the factorial arrangement, having 12 total treatment combinations with four replicate plots for each treatment. A spacing of 0.5, 0.2, 0.5, and 1 m were used for row – row, plant – plant, plot – plot, and block – block arrangements, respectively, with 3.5 × 3.4 m individual plot size.
2.6. Plant sampling and processing
At the mid-flowering growth stage, shoots of five randomly selected plants were considered from each plot to process the shoot for shoot % C, % N, and carbon isotope analyses. The cowpea shoots were oven-dried at 70°C for 48 hrs, weighed, and finely ground to a fine powder (0.85 mm sieve size) for 15N and C isotopic analysis.
2.7. 13C/12C isotopic and shoot N analysis
For 13C/12C isotopic analysis, shoot % C and % N determination, the samples ground previously were further ball-milled. About 2.0 mg of ball-milled shoot samples were weighed into aluminum capsules and loaded on to mass spectrometer for Thermo 2000 Elemental Analyzer coupled via a Thermo Conflo IV to a Thermo Delta V Plus stable light isotope mass spectrometer (Thermo, Corporation, Bremen, Germany). An internal standard of Nasturtium species was included in every five runs to correct machine error during isotopic analysis.
The 13C value of the shoot samples were estimated, while % C was obtained directly from the mass spectrometer. The ratio of 13C/12C in each sample was used to calculate the 13C natural abundance or δ13C (‰) as described by (Farquhar et al. Citation1989):
where 13C/12C sample is the isotopic ratio of the sample and 13C/12C standard is the isotopic ratio of PDB, a universally accepted standard from the Pee Dee Belemnite limestone formation (Craig Citation1957).
2.8. Shoot C content
The shoot C content was calculated as the product of shoot % C (obtained from mass spectrometry) and shoot dry mass as:
2.9. Shoot N content
The N content of shoots was calculated as the product of shoot %N (obtained from mass spectrometry) and shoot dry mass as described by Pausch et al. (Citation1996):
2.10. C/N ratio
The C/N ratio of the shoot was calculated by computing the ratio between C and N concentrations in shoot.
2.11. Statistical analysis
The data were subjected to statistical analysis using a generalized linear model procedure of SAS software program version 9.4 (SAS Institute Citation2012). Inoculant and variety were considered as fixed factors and growing season and location as random variables. Either the two seasons or the three sites were combined when the test for variances is homogeneous; separate analysis was done when the variances were heterogeneous. Variety response and inoculation effects on nitrogen content g plant−1 and 13C isotope were analyzed for the 2018 and 2019 growing seasons and each site separately to compare inter-annual and site variation, since the data for those parameters were heterogeneous for both year and site upon testing homogeneity of variance. The data on shoot dry weight was combined across sites and separated for year, since the data found homogeneous and heterogeneous for sites and years respectively. The data on shoot C content was combined over sites and separated for year, since the data found homogeneous across sites and heterogeneous over years. Both year and sites were combined for percent C concentration and C/N ratio, since the parameters found homogeneous for both season and site. Treatments were compared by least significant difference method, for a significance level of P ≤ 0.05. Linear regression analysis was done for the combined data to determine relationships between the measured parameters.
3. Result
3.1. Effect of Bradyrhizobium inoculation on shoot biomass and C content
The analysis of variance revealed a significant effect on shoot biomass of cowpea varieties during the 2018 and 2019 cropping seasons. However, there were no significant differences among cowpea varieties on shoot C content in the 2019 cropping seasons (). The highest shoot biomass was recorded from the varieties White wonderer trailing followed by TVU and Black eye bean. However, the lowest shoot biomass was recorded from the Keti (IT99K-1122) variety. On the other hand, except for shoot biomass in 2019, Bradyrhizobium inoculation showed a significant effect on shoot biomass and C content in both cropping seasons (). For the data combined across three sites and over two years, inoculated plants produced higher shoot biomass and C content than the control. However, the two Bradyrhizobium strains did not differ from each other in terms of shoot C content during both cropping seasons. The variety x Bradyrhizobium interaction was significant for shoot carbon content (g plant−1) during both the 2018 and 2019 cropping seasons (). The results revealed that White wonderer trailing and TVU varieties produced more shoot C content when inoculated with Bradyrhizobium strain CP-24 than the varieties Keti and Black eye bean ((a,b)).
Figure 1. The interactive effect of variety x Bradyrhizobium on shoot C content (a) in 2018 and (b) in 2019 for data combined across three sites. Data presented are mean values; vertical lines on bars represent standard errors. Uninoc.: Un-inoculated, Keti: Keti (IT99K-1122); BEB: Black eye bean; WWT: White wonderer trailing.
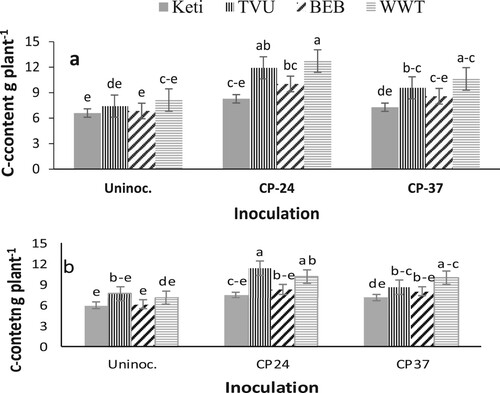
Table 3. Effects of Bradyrhizobium on shoot biomass and C content averaged across three sites during 2018 and 2019.
3.2. Effect of Bradyrhizobium inoculation on shoot C concentration and C/N ratio
The shoot C concentration and C/N ratio were similar among the cowpea varieties, but they were significantly affected by Bradyrhizobium inoculation. Moreover, the variety × Bradyrhizobium inoculation effect was significant for the C/N ratio (). Generally, plants inoculated with Bradyrhizobium strains recorded more shoot C concentration and C/N ratio than the control. Regarding the interaction effect, TVU variety inoculated with Bradyrhizobium strain CP-24 recorded a higher C/N ratio followed by the variety White wonderer trailing and strain CP-24 interaction. The lower C/N ratio was recorded from the variety Keti (IT99K-1122) without inoculation (). However, all un-inoculated, Keti inoculated with CP-24 and CP-37, and Blackeye bean with CP-37 did not differ statistically for C: N ratio ().
Figure 2. The interactive effect of variety × Bradyrhizobium on C/N ratio for data combined across three sites and over two years. Data presented are mean values; vertical lines on bars represent standard errors.
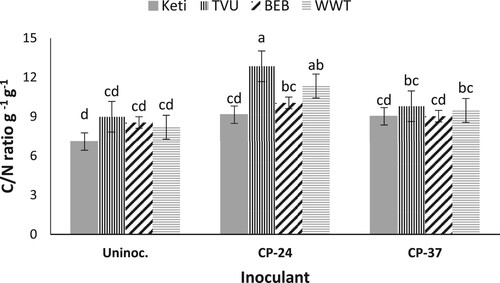
Table 4. Effect of variety and Bradyrhizobium on carbon concentration (%) and C/N ratio average over three sites in 2018 and 2019.
3.3. Effect of Bradyrhizobium inoculation on shoot N content and δ13C
The analysis of variance showed a significant effect of cowpea varieties on shoot N content during the 2018 cropping season. However, the varietal responses were significant for δ13C value during the 2018 and 2019 cropping seasons (), with about 8% higher (fewer negative values) for Keti, indicating the overperformance of this variety in terms of water use efficiency. Shoot N content was markedly lower for the Black eye bean variety. However, there were no significant differences among the other varieties regarding the shoot N content. Among the varieties, a more negative δ13C value was recorded from the White wonderer trailing followed by TVU variety. However, Keti (IT99K-1122) variety recorded a relatively less negative δ13C value than other varieties (). Bradyrhizobium inoculation, on the other hand, had a significant effect on shoot N content at all three sites and δ13C value at the Boricha site during the 2018 cropping season. The higher shoot N content and less negative δ13C value were recorded from the inoculated plants compared to the control, with a 4% advantage in δ13C, indicating improved water use with inoculation. The variety x Bradyrhizobium interaction was significant only for δ13C value at the Boricha site during the 2018 cropping season. Regardless of inoculation, TVU and White wonderer trailing varieties recorded more negative values than the Black eye bean and Keti (IT99K-1122) varieties. Moreover, inoculated plants recorded less negative δ13C values than un-inoculated plants ().
Figure 3. The interactive effect of variety × Bradyrhizobium on δ13C for data combined across three sites and over two years. Data presented are mean values; vertical lines on bars represent standard errors. Un-inoc.: Un-inoculated; Keti: Keti (IT99K-1122); BEB: Black eye bean; WWT: White wonderer trailing.
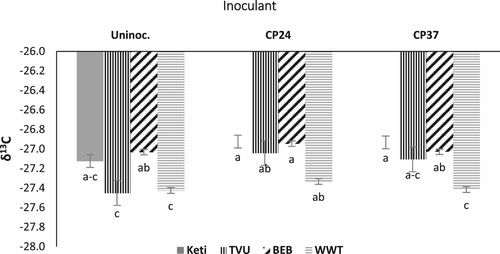
Table 5. Effect of Bradyrhizobium on shoot N content and δ13 C of three sites in 2018 and 2019.
3.4. Regression analysis
Regression analyses were conducted to determine the degree of association between different parameters. Comparing the degree of association between shoot carbon content and shoot dry matter, C/N ratio and shoot carbon content showed positive relationships. C/N ratio was inversely correlated with shoot N content. Moreover, the relation established between seed yield (the seed yield data adopted from Ayalew et al. Citation2021, the data from the same experiment with this one) and shoot carbon content was positive ((a–d)).
4. Discussion
The current study evaluated the effect of Bradyrhizobium inoculation on shoot biomass, shoot C accumulation, and δ13C (as a measuring tool for water-use efficiency) of four cowpea varieties. The results showed that Bradyrhizobium inoculation significantly increased shoot biomass, shoot C concentration, shoot C content, C/N ratio, and shoot δ13C. The magnitude of the shoot biomass and shoot C content response to Bradyrhizobium inoculation was more marked than the other parameters. For example, with strain CP-24 inoculation, shoot biomass accumulation and shoot C content increased by 31 and 28%, respectively, over the un-inoculated control. This is because the effect of symbiotic N nutrition on plants has been attributed to increased stomatal functioning (Augé et al. Citation2014), photosynthetic rate (Belane and Dakora Citation2015), CO2 assimilation, and water use efficiency (Damba et al. Citation2019; Tankari et al. Citation2019). Therefore, the increase in shoot biomass and C accumulation obtained in this study are associated with increased plant growth, photosynthesis, and C assimilation with Bradyrhizobium inoculation (Lovelock et al. Citation2006).
The C/N ratio defines the N status of plants and indicates the C-to-N relationship in organs. A C/N ratio lower than 24 g.g−1 is typical of symbiotic legumes (Hobbie et al. Citation1998), and this stems from the ability of legumes to reduce atmospheric N2 to NH3 in the root nodules. Therefore, the C/N ratio range of 8.21–10.81 g.g−1 recorded in this study matches the range reported for nodulated legumes. Shoot with a C/N ratio of much less than 24 g.g−1 decomposes rapidly, thus mineralizing N (Ronner and Franke Citation2012). Therefore, cowpea shoots with less than 24 g.g−1 C/N were likely to serve as good bio-fertilizers in increasing symbiotic N supply to the following crops.
The δ13C of C3 plants is an integrator of water-use efficiency (Condon et al. Citation2004). In this study, inoculation and interaction of the treatments significantly increased shoot δ13C in 2018. Effective N2-fixation promotes photosynthetic rates and hence greater C accumulation (Belane et al. Citation2014), which led to increased water use efficiency due to regulation in stomatal functioning thereby the transpiration process (Pule-Meulenberg et al. Citation2010). Furthermore, inoculation induces over-expression of trehalose-6-phosphate synthase, indicating the link between improved drought tolerance and grain yield (Suáre et al. Citation2008).
In addition, with the significant effect of Bradyrhizobium inoculation on δ13C during 2018, the δ13C values were generally lower in 2019 than in 2018, indicating the greater amounts of rainfall received in 2019 compared to 2018 (). Plants grown under high rainfall conditions usually exhibit lower δ13C values than those growing under water-stressed conditions (Ma et al. Citation2012). The amount of rainfall received during the growing months (June–October) was high in 2019 (2379 mm) relative to 2018 (1688 mm), therefore, the higher amounts of rainfall during the 2019 cropping season might have increased 13C discrimination and decreased the δ13C values, leading to lower water-use efficiency during this same season. This argument is supported by negative correlations reported between mean annual precipitation and δ13C values in C3 plants (Hartman and Danin Citation2010).
Cowpea varieties also varied significantly in shoot biomass, shoot C content, shoot N content, and δ13C but not for shoot C concentration and C/N ratio, with varieties TVU and White wonderer trailing exhibiting higher shoot biomass, shoot N, and C contents. However, the varieties Keti and Black eye bean registered higher δ13C values (less negative values). The variety White wonderer trailing recorded relatively higher C content followed by TVU. As expected, crops that exhibit greater C assimilation from photosynthesis can accumulate high shoot biomass (Belane and Dakora Citation2015; Taiz and Zeiger Citation2003). The current result confirms the same, where the higher shoot biomass recorded by White wanderer trailing and TVU, and the same varieties also fixed a higher amount of N (data not shown). This was further confirmed by the positive correlation between shoot biomass and carbon content ((a)). In nodulated legumes, the level of photosynthetic C accumulation is regulated by N nutrition (Mohale et al. Citation2014). A relatively higher C/N ratio in TVU and White wonderer trailing varieties was associated with the higher shoot C content recorded in these varieties, while the lower C/N ratio in Keti variety was attributed to the lower shoot C content. The positive relation between the C/N ratio and C content observed in the current study ((c)) confirmed this.
The findings of this study have shown that varieties greater in biomass production and N content showed lower water use efficiency (more negative δ13C values) and vice versa. For instance, the variety White wonderer trailing produced higher shoot biomass and N content but recorded lower water use efficiency (more negative δ13C value). In contrast, Keti (IT99K-1122) variety had higher water use efficiency but lower biomass and N content. Lower water use efficiency (more negative δ13C value) in varieties with higher biomass accumulation and N contribution was reported by an experiment on groundnut on the soils of Ethiopia (Muhaba and Dakora Citation2020).
Bradyrhizobium inoculation significantly increased most of the parameters considered for the cowpea varieties. For instance, shoot biomass, shoot N content, shoot C accumulation, C/N ratio, and δ13C of cowpea were greater in plants inoculated with Bradyrhizobium strain CP-24. Of the two Bradyrhizobium strains tested, strain CP-24 exhibited better performance in those parameters than strain CP-37. Generally, the results obtained in this study have shown that Bradyrhizobium inoculation through biological nitrogen fixation can improve carbon assimilation and carbon isotope discrimination performance leading to improved water use efficiency, which will have paramount importance during the climate change-driven water scarcity periods.
Disclosure statement
No potential conflict of interest was reported by the author(s).
Additional information
Funding
Notes on contributors
Tewodros Ayalew
Tewodros Ayalew is an academic staff at Hawassa University, Ethiopia with associate professor academic rank. He conducts researches in the area of agronomy, symbiosis, sustainable crop production, and crop-climate interaction. Running a number of projects focusing on the legume symbiosis, sustainable intensification, legume response to bio-inoculants.
Tarekegn Yoseph
Tarekegn Yoseph is an associate professor holding a dean position at College of Agriculture, Hawassa University, Ethiopia.
Georg Cadisch
Prof Dr Georg Cadisch is a full professor and holding a chair in the Institute of Agronomy in the Tropics and Subtropics, Hohenheim University, Germany.
References
- Adams MA, Buckley TN, Turnbull TL. 2020. Diminishing CO2-driven gains in water-use efficiency of global forests. Nat Clim Change. 10:466–471.
- Adjei-Nsiah S, Kuyper TW, Leeuwis C, Abekoe M, Cobbinah J, Sakyi-Dawson O, Giller K. 2008. Farmers’ agronomic and social evaluation of productivity, yield and N2-fixation in different cowpea varieties and their subsequent residual N effects on a succeeding maize crop. Nutr Cycl Agroecosyst. 80:199. doi:10.1007/s10705-007-9133-3.
- Atara A, Tolossa D, Denu B. 2019. Assessment of food security situation of the rural households: the case of Boricha Woreda of Sidama Zone, Ethiopia. Geo J. 1–17.
- Augé RM, Saxton AM, Toler HD. 2014. Arbuscular mycorrhizal symbiosis alters stomatal conductance of host plants more under drought than under amply watered conditions: a meta-analysis. Front Plant Sci. 5(562):1–14.
- Ayalew T, Yoseph T. 2020. Symbiotic effectiveness of inoculation with Bradyrhizobium isolates on Cowpea (Vigna unguiculata (L.) Walp) varieties. Cogent Food Agric. 6(1):1845495.
- Ayalew T, Yoseph T, Petra H, Cadisch G. 2021. Yield response of field-grown cowpea (Vigna unguiculata (L.) Walp) varieties to Bradyrhizobium inoculation. Agron J. 113:3258–3268.
- Bai Z, Huang X, Meng J, Kan L, Nie S. 2020. A comparative study on nutritive peculiarities of 24 Chinese cowpea cultivars. Food Chem Toxicol. 146:111841.
- Bano A, Batool R, Dazzo F. 2014. Adaptation of chickpea to desiccation stress is enhanced by symbiotic rhizobia. Symbiosis. 50:129–133.
- Barbour MM. 2007. Stable oxygen isotope composition of plant tissue: a review. Funct Plant Biol. 34:83–94.
- Belane A, Dakora F. 2015. Assessing the relationship between photosynthetic C accumulation and symbiotic N nutrition in leaves of field-grown nodulated cowpea (Vigna unguiculata L. Walp) genotypes. Photosynthetica. 53:562–571.
- Belane AK, Pule-Meulenberg F, Makhubedu IT, Dakora FD. 2014. Nitrogen fixation and symbiosis-induced accumulation of mineral nutrients by cowpea (Vigna unguiculata L. Walp.). Crop and Pasture Science. 65(3):250.
- Condon A, Richards R, Rebetzke G, Farquhar G. 2004. Breeding for high water-use efficiency. J Exp Bot. 55(407):2447–2460.
- Craig H. 1957. Isotopic standards for carbon and oxygen and correction factors for mass-spectrometric analysis of carbon dioxide. Geochim Cosmochim Acta. 12(1–2):133–149.
- Damba Y, Nicholas D, Mustapha M, Blair MW. 2019. Screening cowpea (Vigna unguiculata (L.) Walp.) genotypes for Enhanced N2 fixation and water use efficiency under field conditions in Ghana. Am J Plant Sci. 10:640–658.
- Degefu T, Wolde-Meskel E. Rasche, F. 2018. Genetic diversity and symbiotic effectiveness of Bradyrhizobium strains nodulating selected annual grain legumes growing in Ethiopia Int J Syst Evol Microbiol. 68:449–460.
- Donohue RJ, Roderick ML, McVicar TR, Farquhar GD. 2013. Impact of CO2 fertilization on maximum foliage cover across the globe’s warm, arid environments. Geophys Res Lett. 40:3031–3035.
- Ehdaie B, Waines JG. 1993. Variation in water use efficiency and its components in wheat: I. Well-watered pot experiment. Crop Sci. 33:294–299.
- Ehleringer JR, Phillips SL, Schuster WSF, Sandquist DR. 1991. Differential utilization of summer rains by desert plants. Oecologia. 88:430–443.
- [FAO] Food and Agriculture Organization. 2006. Core production data. http://faostat.fao.org.
- Farquhar GD, Hubick KT, Condon AGD, Richards RA. 1989. Carbon isotope fractionation and plant water-use efficiency. In: Rundel PW, Ehleringer JR, Nagy KA, editors. Stable isotope in ecological research. New York: Springer-Verlag; p. 21–40.
- Farquhar GD, Richards RA. 1984. Isotopic composition of plant carbon correlates with water-use efficiency of wheat genotypes. Funct Plant Biol. 11:539–552.
- Fekadu G. 2013. Assessment of farmers' criteria for common beanvariety selection: The case of Umbullo Watershed in Sidama Zoneof the southern region of Ethiopia. Ethiop E-J Res Innov Foresight. 5(2):4–13.
- Figueiredo MV, Burity HA, Martínez C, Chanway CP. 2008. Alleviation of drought stress in the common bean (Phaseolus vulgaris L.) by co-inoculation with Paenibacillus polymyxa and Rhizobium tropici. Appl Soil Ecol. 40:182–188.
- Gezahegn A. 2018. Household food insecurity in the Sidama Zone of southern Ethiopia: factors, coping and adaptation strategies. J Food Secur. 6(3):99–106.
- Giller KE. 2001. Nitrogen fixation in tropical cropping systems. 2nd ed. Wallingford: CABI Publishing; p. 253–270.
- Hall AE, Mutters RG, Farquhar GD. 1992. Genotypic and drought-induced differences in carbon isotope discrimination and gas exchange of cowpea. Crop Sci. 32:1–6.
- Hartman G, Danin A. 2010. Isotopic values of plants in relation to water availability in the Eastern Mediterranean region. Oecologia. 162(4):837–852.
- Havlin JL, Tisdale SL, Nelson WL, Beaton JD. 1999. Soil fertility and fertilizers. 5th ed. New York: Macmillan Publishing Company; p. 428–455.
- Herrera E. 2005. Soil test interpretations Guide A-122. College of Agriculture and Home Economics New Mexico State University.
- Herridge DF, Herridge D. 2013. Managing legume and fertiliser N for northern grains cropping, 2nd ed. Armidale, NSW: the GRDC and the University of New England.
- Hobbie EA, Macko SA, Shugart H. 1998. Patterns in N dynamics and N isotopes during primary succession in Glacier Bay, Alaska. Chem Geol. 152(1):3–11.
- Ismail AM, Hall AE. 1992. Correlation between water-use efficiency and carbon isotope discrimination in diverse cowpea genotypes and isogenic lines. Crop Sci. 32:7–12.
- Ismail AM, Hall AE. 2000. Semi-dwarf and standard-height cowpea responses to row spacing in different environments. Crop Sci. 40:1618–1623.
- Jemo M, Sulieman S, Bekkaoui F, Olomide OAK., Hashem A, Abd Allah EF, Alqarawi AA, Tran L-SP. 2017. Comparative analysis of the combined effects of different water and phosphate levels on growth and biological nitrogen fixation of nine cowpea. Front Plant Sci. 8:2111.
- Kooperman GJ, Chen Y, Hoffman FM, Koven ChD, Lindsay K, Pritchard MS, Swann ALS, Randerson JT. 2018. Forest response to rising CO2 drives zonally asymmetric rainfall change over tropical land. Nat Clim Change. 8:434–440.
- Kumarasinghe KS, Kirda C, Bowen GD. 1996. Carbon isotope discrimination as a selection tool for high water use efficiency and high crop yields. (Joint FAO/IAEA Div. of Nuclear Techniques in Food and Agriculture, Vienna (Austria). Soil Fertil Irrig Crop Prod Sect. 155–170.
- Li C, Huang M, Liu J, Ji S, Zhao R, Zhao D, Sun R. 2019. Isotope-based water-use efficiency of major greening plants in a sponge city in northern China. PLoS One. 14(7):e0220083.
- Lovelock CE, Feller IC, Ball MC, Engelbrecht BMJ, Ewe ML. 2006. Differences in plant function in phosphorus- and nitrogen-limited mangrove ecosystems. New Phytol. 172(3):514–522.
- Ma JY, Sun W, Liu XN, Chen FH. 2012. Variation in the stable carbon and nitrogen isotope composition of plants and soil along a precipitation gradient in northern China. PLoS One. 12:e51851.
- Makoi JH, Chimphango SB, Dakora FD. 2012. Photosynthesis, water-use efficiency and 13C of five cowpea genotypes grown in mixed culture and at different densities with sorghum. Photosynthetica. 48:143–155.
- Mohale KC, Belane AK, Dakora FD. 2014. Symbiotic N nutrition, C assimilation, and plant water use efficiency in Bambara groundnut (Vigna subterranea L. Verdc) grown in farmers’ fields in South Africa, measured using 15N and 13C natural abundance. Biol Fertil Soils. 50(2):307–319.
- Moreira-Araújo RS, Sampaio GR, Manólio-Soares RA, Silva CP, Arêas JAG. 2017. Identification and quantification of antioxidant compounds in cowpea. Revista Ciencia Agronomica. 48:799–805.
- Mouradi M, Farissi M, Bouizgaren A, Makoudi B, Kabbadj A, Very A-A, Sentenac H, Qaddourya A, Ghoulam C. 2016. Effects of water deficit on growth, nodulation and physiological and biochemical processes in Medicago sativa-rhizobia symbiotic association. Arid Land Res Manag. 30:193–208.
- Muhaba SK, Dakora FD. 2020. Symbiotic performance, shoot biomass and water-use efficiency of three groundnut (Arachis hypogaea L.) genotypes in response to phosphorus supply under field conditions in Ethiopia. Frontiers of Agricultural Science and Engineering. 455–466.
- Onyibe JE, Kamara AY, Omoigui LO. 2006. Guide to cowpea production in Borno State, Nigeria. Ibadan: Promoting Sustainable Agriculture in Borno State (PROSAB); p. 36.
- Pausch RC, Mulchi CL, Lee EH, Meisinger JJ. 1996. Use of 13C and 15N isotopes to investigate O3 effects on C and N metabolism in soybeans. Part II. Nitrogen uptake, fixation, and partitioning. Agric Ecosyst Environ. 60(1):61–69.
- Pottorff M, Ehlers JD, Fatokun C, Roberts PA, Close TJ. 2012. Leaf morphology in cowpea [Vigna unguiculata (L.) Walp]: QTL analysis, physical mapping and identifying a candidate gene using synteny with model legume species. BMC Genomics. 13:234.
- Pule-Meulenberg F, Belane AK, Krasova-Wade T, Dakora FD. 2010. Symbiotic functioning and bradyrhizobial biodiversity of cowpea (Vigna unguiculata L. Walp.) in Africa. BMC Microbiol. 10(1):89.
- Rice WA, Clayton GW, Lupwayi NZ, Olsen PE. 2001. Evaluation of coated seeds as a Rhizobium delivery system for field pea. Can J Plant Sci. 81(2):247–253.
- Rocha IM, Carvalho MF, Magalhaes C, Janoušková M, Vosátka M, Freitas H, Oliveira RS. 2019. Seed coating with inocula of arbuscular mycorrhizal fungi and plant growth-promoting rhizobacteria for nutritional enhancement of maize under different fertilisation regimes. Archives of Agronomy and Soil Science. 65:31–43.
- Ronner E, Franke A. 2012. Quantifying the impact of the N2Africa project on biological nitrogen fixation. [Online], N2Africa; p. 29.
- Salman A, Shahid S, Afan A, Shiru MS, Al-Ansari N, Yaseen ZM. 2020. Changes in climatic water availability and crop water demand for Iraq region. Sustainability. 12(8):3437–3420.
- SAS. 2012. Institute inc. SAS user’s guide, statistics version 9.0 ed. Cary, NC: SAS Institute.
- Serraj R, Sinclair TR, Purcell LC. 1999. Symbiotic N2-fixation response to drought. J Exp Bot. 50:143–155.
- Suáre R, Wong A, Ramírez M, Barraza A, Orozco Mdel C, Cevallos MA, Lara M, Hernández G, Iturriaga G. 2008. Improvement of drought tolerance and grain yield in common bean by overexpressing trehalose-6-phosphate synthase in rhizobia. Mol Plant-Microbe Interact. 21(7):958–966.
- Taiz L, Zeiger E. 2003. Photosynthesis: carbon reactions. Plant physiology. Sunderland: Sinauer Associates, p. 145–168.
- Tankari M, Wang C, Ximei ZX, Li L, Soothar R, Ma H, Xing H, Yan C, Zhang Y, Liu F, Wang Y. 2019. Leaf gas exchange, plant water relations and water use efficiency of Vigna unguiculata L. Walp. inoculated with rhizobia under different soil water regimes. Water (Basel). 11(3):498–415.
- Wei T, Guillaume T, Xu M, Schäufele R, Schnyder H, Yang Y, Gong XY. 2021. Accounting for mesophyll conductance substantially improves 13C-based estimates of intrinsic water-use efficiency. New Phytol. 229:1326–1338.
- Wright GC, Nageswara R, Farguhar GD. 1994. Water-use efficiency and carbon isotope discrimination in peanut under water deficit conditions. Crop Sciences. 34: 92–97.
- Yanni Y, Zidan M, Dazzo F, Rizk R, Mehesen A, Abdelfattah F, Elsadany A. 2016. Enhanced symbiotic performance and productivity of drought stressed common bean after inoculation with tolerant native rhizobia in extensive fields. Agric Ecosyst Environ. 232:119–128.