ABSTRACT
Endophytic plant-growth-promoting bacteria help plants to cope with severe environmental stresses. A Klebsiella sp. strain San01 isolated from sweet potato roots exhibited diverse plant-growth-promoting activities, including indole acetic acid production, ammonia production, and phosphate solubilization. San01 inoculation significantly increased the growth characteristics of sweet potato. Moreover, the sweet potato inoculated with San01 resulted in the alleviation of drought and salinity stresses. In comparison with the non-inoculated plants, the wilting symptom and the inhibition of photochemical response were significantly diminished in San01-inoculated plants under stress conditions. In the presence of San01, the enhancement of 2,2-diphenyl-1-picrylhydrazyl radical scavenging ability and elevated activities of ascorbate peroxidase and superoxide dismutase were observed to combat the oxidative stress in sweet potato. Further investigation showed that the San01 inoculation enhanced the expression of the IbLEA gene in sweet potato under salinity and drought. These results confer a promising biotechnological approach through San01 interaction to develop stress-tolerant sweet potato plants.
Key message
Klebsiella sp. San01 inoculation alleviated salinity and drought stress in sweet potato by increasing antioxidant capacity and up-regulating salt- and drought-related genes.
Introduction
Sweet potato (Ipomoea batatas L.), a member of the Convolvulaceae family, is cultivated for its enlarged storage roots as the main food crop, forage crop, industrial raw material, and biofuel crop in more than 100 countries, especially in Asia and Africa (de Albuquerque et al. Citation2019). In recent decades, the tender leaves and shoots of sweet potato have become a popular green leafy vegetable due to a rich source of fiber, vitamins, micronutrients, and various bioactive compounds in diets (Kitayama et al. Citation2020; Nguyen et al. Citation2021). Thus, more and more leafy vegetable sweet potato cultivars with many tender shoots and small tubers have been bred and wildly cultivated in East Asia (Lai Citation2008; Kitayama et al. Citation2020). The yield of sweet potato is often restricted by abiotic stresses, especially for salt and drought (Ruan et al. Citation2012; Yooyongwech et al. Citation2013; Zhai et al. Citation2016; Zhang et al. Citation2019b). The development of sweet potato with elevated tolerance to salt and drought is therefore highly desirable. Several reports have suggested that overexpression of functional genes, such as the Na+/H+ antiporter gene (Wang et al. Citation2016; Zhang et al. Citation2019a), IbLEA14 (Park et al. Citation2011), IbOr (Kim et al. Citation2013), IbP5CR (Liu et al. Citation2014), IbMIPS1 (Zhai et al. Citation2016), and IbC3H18 (Zhang et al. Citation2019a), can significantly improve the salt and drought tolerances in sweet potato. However, the cultivation of genetically modified crops is restricted in many areas. It is therefore important to find a strategy to increase the growth performance under salt and drought stresses in sweet potato for maintaining productivity.
The utilization of biofertilizers containing beneficial microorganisms is one of the eco-friendly strategies for managing soil quality, improving crop yield, and enabling crops to cope with environmental challenges in sustainable agriculture (Hita et al. Citation2020). Among these beneficial microbes, plant-growth-promoting bacteria (PGPB) colonize plant rhizosphere or inside plants roots, and generally cause no negative effects on the plant hosts (Adhikari Dhungana et al. Citation2018). The interaction of PGPB can alter the growth, fitness, and stress tolerance of the plant host through a wide variety of mechanisms, including phosphate solubilization, ammonia production, siderophore synthesis, biological nitrogen fixation, phytohormone production, production of 1-aminocyclopropane-1-carboxylate (ACC) deaminase, and induction of systemic resistance (Eid et al. Citation2021). However, several crop plants and soil types may lack the effective microbial activity. Previous studies have shown that the negative effects of osmotic stress in other crop plants, including the reduction of chlorophyll (Chl) content and repression of photosynthetic reaction, can be diminished by interaction with endophytic PGPB (Marasco et al. Citation2012; Ullah et al. Citation2019). Additionally, the usage of PGPB-possessing indole acetic acid (IAA) and ACC deaminase has become a promising alternative for diminishing damage caused by water deficiency and salinity in plants (Singh et al. Citation2015; Cardoso et al. Citation2018). Accordingly, the isolation and characterization of PGPB can contribute to a new agricultural scenario for economically efficient crop production systems by decreasing the use of chemical fertilizers and enhancing the tolerance to salinity and drought.
Recently, many efforts have focused on the PGPB isolation in sweet potato. Puri et al. (Citation2018) reported that sweet potato interacted with several kinds of endophytic PGPB, such as Bacillus sp., Enterobacter sp., Pantoea sp., and Klebsiella sp., in different localization of Nepalese to help them grow in low-fertile soils. Further, Adhikari Dhungana et al. (Citation2018) evaluated that Klebsiella sp. Sal1 and Enterobacter sp. Sal3 isolated from sweet potato tubers promoted the biomass of sweet potato by producing IAA inside the roots under low nutrient conditions. These high-colonizing plant-growth-promoting microbes could be applied as biofertilizer agents or resistance inducers in sweet potato cultivation. Although some PGPB isolated from sweet potato have been identified and studied, the understanding of plant–microbe interaction influencing salinity and drought tolerance in sweet potato remains rare thus far. Furthermore, the investigations focused on the impacts of interactions with PGPB on physiological changes and molecular mechanisms in sweet potato plants are also poorly characterized. Therefore, this study aimed to isolate and characterize the endophytic PGPB with multiple beneficial traits from the roots of leafy vegetable sweet potato cultivar Fucaishu 18, thereby promoting growth performance and providing the salt and drought tolerance of the host plant. Further, the growth traits, photosynthetic parameters, antioxidant abilities, and transcriptional regulations in PGPB-inoculated plants under salt and drought stresses were also investigated and discussed.
Materials and methods
Plant materials and bacterial isolation
The sweet potato cv. Fucaishu 18 bred by the Fujian Academy of Agricultural Sciences (Fujian, China) is the most popular leafy vegetable cultivar, wildly planted in the Fujian province of China. The stems of tissue-cultured Fucaishu 18 plant were cut into 3-cm length, and cultured in 1/2 Murashige and Skoog basal (MS) medium containing 3% sucrose for 7 days to ensure the consistency of the plant materials. The rooted seedlings were planted in a commercial potting soil mixture without fertilizer in a phytotron at 25 oC under 50 μmol m –2 s –1 of light with a 16-h photoperiod for 2 weeks.
Four morphologically different bacterial endophytes were previously isolated from the roots of leafy vegetable sweet potato cultivar Fucaishu 18 growing in sandy soil in Sanming city (Fujian, China). The bacterial colonies were selected and maintained in glycerol culture stocks at −80ᵒC. The selected isolates were cultured in lysogeny broth (LB) medium at 28 °C in dark at 180 rpm for 72 h, and then collected by centrifugation. The pellet was rinsed five times in distilled deionized H2O to remove the medium interference. The optical density of 600 nm was measured using an Infinite M200 plate reader (Tecan, Switzerland) to adjust the suspension to 0.05 for plant inoculation.
Characterization of plant-beneficial traits
All strains were examined for their plant-growth-promoting activities. The isolates were examined for IAA production using the method of Gordon and Weber (Citation1951). In brief, 72-h-old bacterial culture grown in Nutrient broth (NB) containing tryptophan at 30°C with 180 rpm shaking was harvested by centrifugation. One milliliter of supernatant was mixed with 4 ml of Salkowsky’s reagent, and reacted at 25°C for 30 min. A pinkish color was considered a positive indicator of IAA production. The IAA concentration was measured from the absorbance at 530 nm using an Infinite M200 plate reader (Tecan, Switzerland), and then quantified by the standard curve of IAA (0, 0.1, 0.5, 1, 5, and 10 μg). Non-inoculated NB medium was used as a blank control. The ammonia accumulation was determined as previously described by Wu et al. (Citation2018). The isolates were grown in tube-containing peptone water with three replicates and incubated at 28 °C for 2 days. The culture supernatant was added and mixed with the Nessler’s reagent and ammonia-free deionized water. The optical density was measured at 450 nm using an Infinite M200 plate reader (Tecan, Switzerland). A standard ammonium curve was prepared with different concentrations of ammonium chloride (0, 1, 5, 10, 25, and 50 μg ml–1).
The National Botanical Research Institute’s phosphate (NBRIP) agar medium and chrome azurole sulfonate (CAS) agar medium were utilized to test the phosphate solubilization and siderophore production in isolated bacteria. The colony of each strain was inoculated on the NBRIP medium or CAS medium at 28 °C for 3 days. The clarification halo and red-orange halo zone around the colony were considered positive for phosphate solubilization and siderophore production, respectively. For ACC deaminase assay, the isolated strains were inoculated in Dworkin and Foster (DF) medium and DF medium supplemented with ACC (ADF) and then cultured at 30 °C for 7 days. The isolate that grew better in ADF medium than in medium was considered positive for ACC deaminase production.
Salt and drought tolerance of bacterial isolates
Salt and drought tolerance assays were performed as previously described by Singh et al. (Citation2015) and Komaresofla et al. (Citation2019), respectively. The salt tolerance assay was examined in an NA medium containing different NaCl concentrations (0.5%–8%). For drought tolerance assay, the growth potential of isolates was assayed in an NA medium containing 0, 202.2, 295.7, and 367.7 g/L PEG6000 (osmotic pressure of 0, −5, −10, and −15 bar, respectively). The growth rate of isolates was determined by measuring optical density at 600 nm using an Infinite M200 plate reader (Tecan, Switzerland), and was compared with the growth of the same strain in the NB medium without NaCl or PEG6000.
Identification and phylogenetic analysis of 16S rRNA gene
The genomic DNA of the selected isolate was carried out using the TIANamp Bacteria DNA kit (Tiangen, China). The PCR analysis was performed using universal primers 5’-AGAGTTTGATCMTGGCTCAG-3’ and 5’-TACGGCTACCTTGTTACGAC-3’ to amplify the 16S rRNA gene. The PCR preparations were performed essentially as described by Chiang et al. (Citation2014). The amplification program consisted of 1 cycle at 94 oC for 5 min and 30 cycles of 94 oC for 30 s, 55 oC for 30 s, and 72 oC for 1 min, followed by 1 cycle at 72 oC for 5 min (ABI7500). The amplicon was cloned into a pMD18-T vector (Takara Bio, Japan), and then verified by DNA sequencing.
Thirteen 16s rRNA gene sequences from different Klebsiella spp. retrieved from the GenBank database were aligned using the Clustal X program. The evolutionary history and a bootstrap consensus tree of bacterial 16s rRNA genes were generated by the Neighbor-joining (NJ) method (Saitou and Nei Citation1987) from 1000 replications in the MEGA4.0 program (Tamura et al. Citation2007).
Plant inoculation and stress treatments
The sweet potato materials were treated by irrigating the plants with 100 mL Klebsiella sp. San01 per plant once every 7 days for two times. The non-inoculated plants were simultaneously treated by irrigation with 100-mL ddH2O once every 7 days for two times. After 4 weeks of inoculation with the San01 strain, the second leaves from the top of inoculated and non-inoculated plants were harvested for physiological, biochemical, and gene expression analysis.
After 4 weeks of inoculation, the inoculated and non-inoculated plants were further treated with salinity and drought stresses. For stress treatments, the plants were irrigated with water containing 200-mM NaCl once every 3 days or subjected to drought stress without watering. After 7 and 14 days of treatments, the inoculated and non-inoculated plants were harvested for physiological, biochemical, and gene expression analysis.
Determination of physiological characteristics
The maximal photochemical efficiency (Fv/Fm) was measured using a MiniPAMII analyzer (Walz, Effeltrich, Germany). The Chl measurement was performed according to the method of Wintermans and De Mots (Citation1965). The contents of Chl a and Chl b were measured at the absorbance of OD665 and OD649. For carotenoids (Car) analysis, the leaf powder was extracted with methanol as described by Sumanta et al. (Citation2014). The Car content was determined at the absorbance of OD470, OD652, and OD665 by using an Infinite M200 plate reader (Tecan, Switzerland). Leaf water status was determined by measuring relative water content (RWC), and calculated as follows: RWC = (fresh weight − dry weight)/(turgid weight − dry weight) × 100% (Weatherley Citation1950).
Determination of 2,2-diphenyl-1-picrylhydrazyl (DPPH) scavenging ability and antioxidative enzyme activity
The DPPH-radical scavenging ability was performed by using the DPPH Assay Kit (Solarbio, China), and then calculated from the absorbance at 515 nm according to the manufacturer’s instruction by Infinite M200 plate reader (Tecan, Switzerland). Ascorbate solution (10 mg ml–1) was used as a positive control.
For activity analysis of ascorbate peroxidase (APX, EC 1.11.1.11) and catalase (CAT, EC 1.11.1.6), the leaf powder (100 mg) was extracted with 100-mM sodium phosphate buffer (pH 6.8) containing a 1-mM phenylmethylsulfonyl fluoride (Sigma, USA). The activities of APX and CAT were performed according to the protocols described by Nakano and Asada (Citation1981) and Hwang and VanToai (Citation1991), respectively. The superoxide dismutase (SOD, EC 1.15.1.1) activity was assayed by using the SOD Assay Kit (Solarbio, China), and then calculated from the absorption at 560 nm according to the manufacturer’s instructions by an Infinite M200 plate reader (Tecan, Switzerland).
RNA isolation and gene expression analysis
Total RNA was isolated from sweet potato plants using the pine tree method as previously described by Chang et al. (Citation1993). Two micrograms of total RNA were reverse-transcribed using the PrimeScript™ RT reagent Kit with gDNA Eraser (Takara Bio, Japan) according to the manufacturer’s instructions. The real-time quantitative PCR (RT-qPCR) program consisted of 1 cycle at 95 °C for 30 s followed by 45 cycles of 95 °C for 5 s and 60 °C for 20 s (ABI QuantStudio 3, USA), and the gene expression levels were calculated by the 2−ΔΔct method. Fold changes of expression levels were calibrated by non-inoculated plants in each treatment. To normalize the gene expression level, the sweet potato IbActin gene was co-amplified as internal controls. Gene-specific primers used in this study were referred to previous studies by Wang et al. (Citation2016) and Zhai et al. (Citation2016), and information of gene-specific primers is shown in Table S1. A melting curve analysis was conducted to confirm the quality of the RT-qPCR.
Plant colonization of San01 after inoculation
Sweet potato plants were harvested after 4 weeks of inoculation. The adhering soil was removed from the plant roots. Subsequently, the root surface was sterilized with 0.6% NaClO for 1 min and then washed with sterilized water four times. For determination of the root colonization, 0.5 g roots were manually ground with sterilized PBS buffer and 1% fungicidin. The resulting suspensions were evaluated by colony-forming unit (CFU) according to the dilution-plate method on an LB agar plate containing 20-ppm rifampicin. Other bacterial flora were eliminated from the plates by rifampicin, while San01 is a rifampicin-resistant bacterial strain. The re-isolated colonies were also verified by colony characteristics and microscopic observation against the San01 strain.
Statistical analysis
All experiments in this study are tabulated as the mean value with the standard deviation of at least three independent replications, as stated in each figure legend. Statistical significance was determined by a two-tailed Student’s t-test and one-way ANOVA at a significance of P < 0.05 using Excel 2010 and SPSS 22.0 software.
Results
Identification and characterization of endophytic PGPB in sweet potato
To identify and evaluate an endophytic PGPB strain, which is helpful to improve plant growth performance and stress tolerance of sweet potato, the plant-growth-promoting traits of four strains (San01-04) isolated from sweet potato roots were surveyed. In the IAA production assay, San01 and San02 showed positive results in the production of IAA. Among the four isolates, San01 produced IAA at the highest levels (31.61 μg ml–1) (a). Similarly, the result of the ammonia production assay showed that San01 had the maximum ability of ammonia production (35.84 μg ml–1), followed by San02 (28.53 μg ml–1) (b). In the phosphate solubilization assay, a clarification halo around San01 growth was observed on the NBRIP medium, suggesting that only the San01 strain was able to solubilize phosphate among these isolates (Figure S1a). Another important PGPB trial is the siderophore production. San01 isolate could grow on CAS agar and was considered as siderophore producers based on a red-orange halo formed around the colonies (Figure S1b). ACC deaminase activity of isolates was further examined by the DF medium supplemented with ACC. However, all isolated endophytes could not grow normally on the medium, suggesting that none of them had strong ACC deaminase activity (Figure S1c). The salt and osmotic tolerance of all the four isolates was also examined. The results showed that all of them were able to tolerate 4% NaCl, while only San01 and San02 showed growth in medium containing 6% NaCl (d). In parallel, the San01 also exhibited better tolerance to PEG-6000 than other isolates (f).
Figure 1. Plant growth promoting traits of sweet potato endophytic isolates. (a) In vitro test for IAA-producing potential of endophytes. (b) Quantitative estimation of ammonia production by bacterial isolates. (c) Growth potential of endophytes in NA medium with different NaCl concentrations. (d) Growth potential of endophytes in NA medium with different PEG-6000 concentrations. Values are the means (n = 4) with corresponding standard deviations. Different letters indicate significant differences analyzed by ANOVA (P < 0.05).
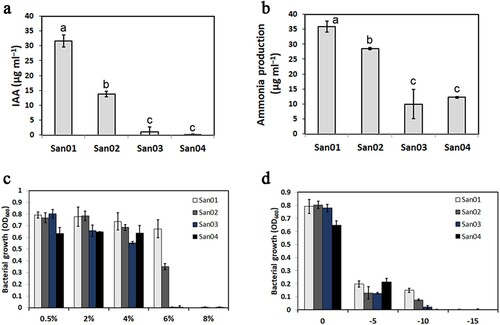
Among these isolates, San01 exhibited versatile plant-growth-promoting activities, including IAA production, ammonia production, phosphate solubilization, and siderophore production, and was tolerant to osmotic treatments. Thus, San01 was selected for detailed functional analysis in this study. For identification of San01 at the molecular level, an amplicon of the 16S rRNA gene was obtained by PCR (a), and the sequence was submitted to the NCBI Genbank under the accession number MZ734410. The strain San01 identified as Klebsiella sp. is closely related to Klebsiella quasipneumoniae 07A044 (99.79% identity) and Klebsiella pneumoniae R-70 (99.72% identity). Phylogenetic dendrogram also showed its similarity to other strains of Klebsiella spp. (b).
Figure 2. Amplification and analysis of 16S rRNA of Klebsiella sp. San01. (a) Amplification of 16s rRNA sequence of San01 by genomic PCR. (b) Phylogenetic analysis of Klebsiella sp. San01 to closely related bacteria. The 16S rRNA gene sequences of closely related species were retrieved from NCBI GenBank database.
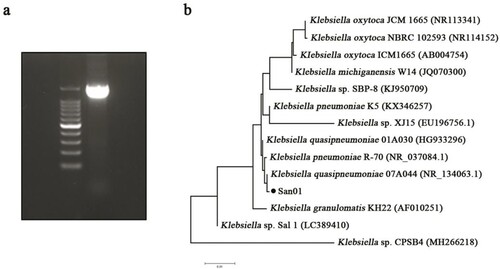
Contribution of Klebsiella sp. San01 to growth performance and abiotic stress tolerance of sweet potato
Klebsiella sp. San01 was used to evaluate the role of the leafy vegetable sweet potato cultivar. The inoculation showed a positive effect on the growth of sweet potato seedlings (a). Furthermore, a strong promotion of roots in San01-inoculated plants was also observed compared with that in non-inoculated plants (b). The biomass of sweet potato plants was further measured 4 weeks after the inoculation. c illustrates that the fresh weights of shoots and roots of inoculated plants were 1.55-fold and 1.48-fold higher, respectively than that of non-inoculated plants.
Figure 3. Effect of Klebsiella sp. San01 on the growth of the sweet potato. Phenotypic comparisons of shoot (a) and root (b) between San01-inoculated (+San01) and non-inoculated (−San01) sweet potato plants were observed after four weeks of inoculation. (c) Effect of San01 on the fresh weights of shoot and root in sweet potato plants after inoculation. Values are the means (n = 8) with corresponding standard deviations. Statistical significance between San01-inoculated and non-inoculated plants was examined by Student’s t-test (*P < 0.05; **P < 0.01).
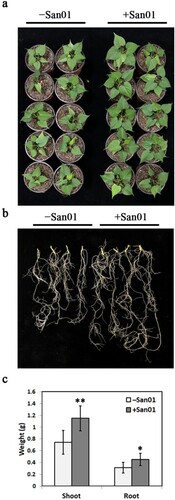
In addition to the growth promotion, the enhancement of salt and drought stress tolerance in sweet potato was also found after the San01 inoculation. After 14 days of salt and drought treatments, the plants without inoculation exhibited severe symptoms of stress, including leaf wilting and chlorosis, while the San01-inoculated sweet potato plants showed better growth and tolerances than the non-inoculated plants ((a and b)). In consistency with the tolerant phenotypes under saline conditions, the fresh weights of the shoots and roots of inoculated plants were 1.93-fold and 1.43-fold, respectively, higher than those of non-inoculated plants (c). Similarly, San01 inoculation also increased the shoot (1.97-fold) and root weights (1.51-fold) of sweet potato plants under drought treatment (d).
Figure 4. Impact of Klebsiella sp. San01 on the growth of sweet potato under salt and drought treatments. The representative photographs of the San01-inoculated (+San01) and non-inoculated (−San01) sweet potato plants were taken after 14 days of salt treatment (a) and drought treatment (b). Changes in biomass of +San01 and −San01 plants exposed to salt treatment (c) and drought treatment (d). Values are the means (n = 8) with corresponding standard deviations. Statistical significance between San01-inoculated and non-inoculated plants was examined by Student’s t-test (*P < 0.05; **P < 0.01).
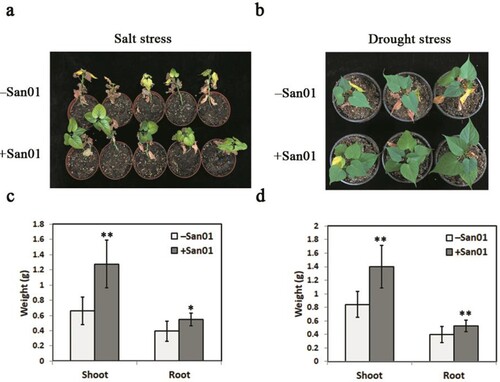
Effects of Klebsiella sp. San01 on physiological characteristics in sweet potato under salt and drought stresses
shows the impacts of San01 isolate on the physiological characteristics of sweet potato plants under different conditions. The physiological response of inoculated plants in response to drought and salt stress was determined by measuring the Fv/Fm and RWC. Although the Fv/Fm value showed no significant differences (P > 0.05) between San01-inoculated and non-inoculated plants under non-stress treatment, the Fv/Fm value of the non-inoculated plants was significantly (P < 0.05) lower than the value of inoculated plants in both salt and drought conditions (a). Similarly, the levels of RWC exhibited no significant differences (P > 0.05) between San01-inoculated and non-inoculated plants without stress treatment, while the RWC in San01-inoculated plants was significantly (P < 0.05) higher than that in non-inoculated plants under both stress conditions (b).
Figure 5. Effects of Klebsiella sp. San01 on physiological parameters, including Fv/Fm (a), RWC (b), chlorophyll (Chla and Chl b) contents (c), and carotenoid contents (d) in leaves of sweet potato plants exposed to non-stress, salt, and drought conditions. Values are the means (n ≥ 4) with corresponding standard deviations. Statistical significance between San01-inoculated plants (+) and non-inoculated plants (−) was examined by Student’s t-test (*P < 0.05; **P < 0.01)
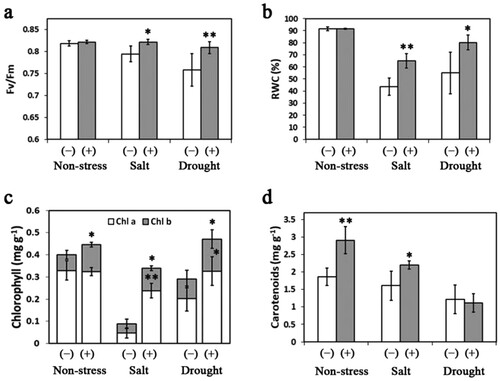
Furthermore, the contents of Chl b and Car in San01-inoculated sweet potato plants were significantly (P < 0.05) increased by 70.2% and 55.7%, respectively, compared with those in non-inoculated plants, while the Chl a content in inoculated plants did not differ from that in non-inoculated plants ((c and d)). Upon salt treatment, the Chl a, Chl b, and Car in inoculated plants were 4.9-fold, 1.5-fold, and 1.4-fold higher than those in non-inoculated plants, respectively. Similarly, compared with non-inoculated plants, San01 inoculation increased the contents of Chl a and Chl b in sweet potato plants subjected to the drought treatment. However, there were no significant differences (P > 0.05) in Car content between the inoculated and non-inoculated plants under drought stress ((c and d)).
Antioxidant activity in San01-inoculated sweet potato plants under salt and drought conditions
The DPPH radical scavenging ability and H2O2 accumulation were used as indicators to monitor the antioxidant activity and stress damage in sweet potato plants after 14 days of stress treatments. Under non-stress conditions, the San01-inoculated plants (40.5%) exhibited significantly higher DPPH radical-scavenging ability than non-inoculated plants (32.5%) (a). Differences in DPPH radical scavenging ability were also detected between the inoculated and non-inoculated plants grown under salt and drought conditions. b illustrates that San01 inoculation slowed the accumulation of H2O2 under stress conditions. The H2O2 levels in San01-inoculated plants were reduced by 46% and 56.5% compared with those in non-inoculated plants under salt and drought treatments, respectively, whereas no difference was observed between the inoculated and non-inoculated plants under non-stress conditions.
Figure 6. The impacts of Klebsiella sp. San01 on antioxidant activity and ROS accumulation of sweet potato plants exposed to salinity and drought. The DPPH-radical scavenging ability (a) and H2O2 accumulation (b) were determined in San01-inoculated (+) and non-inoculated (−) sweet potato plants under non-stress, salt, and drought conditions. Values are the means (n = 5) with corresponding standard deviations. Statistical significance between San01-inoculated plants and non-inoculated plants was examined by Student’s t-test (*P < 0.05; **P < 0.01).
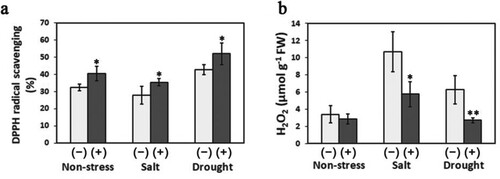
To further investigate the effects of San01 on enzymatic reactive oxygen species (ROS) scavengers, the activities of APX, CAT, and SOD were monitored. The activities of APX, CAT, and SOD in San01-inoculated plants did not differ from those in non-inoculated plants under non-stress conditions. However, higher levels of antioxidant enzyme activities were observed in San01-inoculated plants under both salt and drought treatments, except for CAT (). In the presence of San01, the activities of APX and SOD were separately increased by 22% and 26%, respectively, after 14 days of salt treatment and 34% and 43%, respectively, after 14 days of drought treatment. Therefore, San01 induced the antioxidant defense system of the sweet potato host against stress challenges.
Table 1. The activities of APX, CAT, and SOD in the leaves of San01-inoculated and non-inoculated sweet potato plants under non-stress, salt, and drought treatments.
Expression analysis of the genes related to drought and salt tolerance
The expressions of well-known salt- and drought-related genes were monitored to evaluate the transcriptional responses induced by San01 in sweet potato. Under non-stress conditions (0 h), the transcription levels of most selected genes did not change in response to San01 inoculation, except for the IbLEA gene (). Compared with the non-inoculated plants, a 5.1-fold increase in expression of the IbLEA gene was found in San01-inoculated plants.
Figure 7. Expression analysis of salt- and drought-related genes in San01-inoculated (+) and non-inoculated (−) plants under non-stress, salt, and drought treatments. Fold changes of gene expression levels were calibrated by the non-inoculated plants in each treatment. Values are the means (n = 5) with corresponding standard deviations. Statistical significance between San01-inoculated plants and non-inoculated plants was examined by Student’s t-test (*P < 0.05; **P < 0.01).
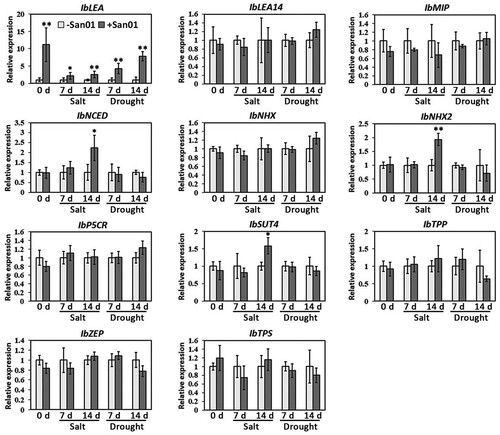
Furthermore, the expression levels of stress-responsive genes in sweet potato were also examined after stress treatments. The RT-qPCR results showed that San01 inoculation increased the IbLEA expression in sweet potato after 7 days of salinity treatment. However, the expressions of other stress-responsive genes in San01-inoculated plants did not differ from those in non-inoculated plants (). Notably, upon 14 d of salinity exposure, there was still no difference (P > 0.05) in the expressions of IbLEA14, IbMIP1, IbNHX, IbSUT4, IbTPS, IbZEP, IbP5CR, and IbTPP genes between San01-inoculated plants and non-inoculated plants, while San01 significantly (P < 0.05) enhanced the expression levels of IbLEA, IbNHX2, IbNCED, and IbSUT4 genes in sweet potato plants subjected to long-term salt stress. Similar to non-stress and salt conditions, the expression level of the IbLEA gene in San01-inoculated plants was significantly (P < 0.05) higher than that in non-inoculated plants under both 7 and 14 days of drought treatment, while there was no significant difference (P > 0.05) in transcription levels of other selected genes between San01-inoculated and non-inoculated plants.
Plant colonization of San01
The potential colonization ability of San01 in sweet potato roots was determined (). After 4 weeks of San01 colonization (non-stress), the endophytic San01 was detected in a range of 4.95×104 (log CFU/g) of the roots. Upon 7 days of salinity exposure and drought treatment, there was no significant difference (P > 0.05) in the colonized population of San01 in plant roots compared with that under non-stress conditions. However, the population of the San01 strain was decreased after 14 days of salt and drought treatment.
Table 2. Plant colonization of Klebsiella sp. San01.
Discussion
Natural bioinoculants can be utilized to promote growth and yield of crops and decrease the use of chemical fertilizers in sustainable agriculture. Although several studies have isolated the endophytic bacteria with plant-growth-promoting traits, such as Klebsiella, Bacillus, Pantoea, and Gammaproteobacteria, from sweet potato (Khan and Doty Citation2009; Marques et al. Citation2015; Puri et al. Citation2018), the endophytic bacteria contributing to enhanced tolerance against salinity and drought stress in sweet potato are still elusive. In this study, an endophytic PGPB strain, Klebsiella sp. San01, isolated from the roots of leafy vegetable sweet potato cultivar was identified and characterized. San01 inoculation not only promoted plant growth but also improved the tolerance to drought and high salinity in sweet potato. This bacterial isolate alleviated the impairments of relative water content and photosynthetic efficiency, increased the antioxidant ability, and induced significant changes in the expression of stress-related genes in sweet potato under drought and salt conditions.
The plant growth promotion and tolerance-improving potential of Klebsiella sp. have been identified in many plants, including tomato (Gupta et al. Citation2020), rice (Pramanik et al. Citation2017), and wheat (Singh et al. Citation2015). Klebsiella strains can express one or more plant beneficial traits, such as nitrogen fixation, phosphate solubilization, IAA production, siderophore production, ACC deaminase production, and ammonia production, to participate in plant physiological responses (Mukherjee et al. Citation2020). In our work, Klebsiella sp. San01 revealed the ability to IAA production, ammonia production, siderophore production, and phosphate solubilization together, and exhibited the tolerance to salinity and osmotic stress (). Previous reports indicated that PGPB could promote plant performance through nitrogen fixation and IAA production (Adhikari Dhungana et al. Citation2018; Eid et al. Citation2021). Other reports also showed that the phosphate-solubilizing bacteria are responsible for the better performance and faster growth of the plants due to the increasing phosphate uptake and organic acid accumulation (Otieno et al. Citation2015; Joe et al. Citation2016). Based on these results, Klebsiella sp. San01 may potentially be employed as biofertilizers in cultivated crop fields for enhancing nutrient uptakes, phytohormone levels, and consequent growth improvement of crops. Furthermore, Barnawal et al. (Citation2017) indicated that plant IAA content is negatively affected by abiotic stresses, such as salinity and drought, but PGPR inoculation confers the enhanced IAA level for alleviation of negative effects induced by abiotic stresses. In the present study, drought and high salinity significantly reduced the biomass in non-inoculated plants compared to San01-inoculated plants. Therefore, the tolerance improvements of the sweet potato may be attributed to the positive impact of San01 on IAA production.
In addition to plant biomass, the changes in several physiological parameters also demonstrated plant performance in response to different stresses. Photosynthetic efficiency and light-capturing pigments are widely accepted as biomarkers to examine the tolerance to abiotic stress in plants. The San01-inoculated sweet potato plants showed better Fv/Fm than non-inoculated plants under non-stress and stress conditions (a). Although the stresses slightly repressed the Fv/Fm in San01-inoculated plants, the Fv/Fm in San01-inoculated plants was greater than 0.8, suggesting that an absence of photoinhibition occurred in San01-inoculated plants under drought and salt treatments (Mevy et al. Citation2020). The increased photosynthetic capability is important for promoting plant growth and development in response to environmental challenges (Chen et al. Citation2022). Therefore, photosystem II damage in sweet potato leaves can be mitigated by San01 inoculation under abiotic stress. Exposure of plants to high salinity and dehydration reduces Chl and Car properties, which are two major light-harvesting pigments in the photosynthetic system. (Koźmińska et al. Citation2019). The elevated levels of photosynthetic pigments in plants by PGPB interaction result in a higher photosynthetic rate, which supports plant performance under salt- and drought-stressed environments (Kaushal and Wani Citation2016). Therefore, San01 mitigated the decrease in Chl and Car contents as well as photosynthetic rate, supporting the growth performance of sweet potato plants under stress treatments.
Generally, ROS overproduction is a primary consequence of photosynthetic inhibition. Since the San01-inoculated sweet potato plants exhibited lower H2O2 levels and higher DPPH scavenging activities than non-inoculated plants under stress treatments (), the inoculation alleviates the ROS-mediated damage of sweet potato against salinity and drought. The elevated DPPH-radical scavenging activity is usually based on antioxidant metabolites, including ascorbate, polyphenols, and flavonoids (Ksouri et al. Citation2007). Car is considered the major contributor to eliminating ROS (Takahashi et al. Citation2020). The elevated DPPH-radical scavenging activities by San01 may be highly associated with the carotenoids’ production in sweet potato under non-stress and stress conditions. Additionally, it has been proposed that Klebsiella sp. enhanced plant resistance by activating ROS-scavenging enzymes, including CAT, POD, and SOD in tomato plants (Gupta et al. Citation2020). As expected, San01 inoculation increased APX and SOD activity under both stress conditions (). In sweet potato, APX, and SOD have important roles in enhancing the tolerance to osmotic stress (Yan et al. Citation2016). Accordingly, these results were reflected in the lower H2O2 accumulation and tolerant phenotypes of San01-inoculated sweet potato plants under salinity and drought treatments.
PGPB interaction enhances the expressions of functional genes to improve the tolerance against salt and drought in plants (Kaushal and Wani Citation2016; Eid et al. Citation2021). Our work revealed that most salt- and drought-responsive genes in sweet potato were not influenced by San01 inoculation under non-stress conditions, except for the IbLEA gene. Similarly, the expression of IbLEA in San01-inoculated plants was also higher than in non-inoculated plants under both salt and drought treatments (). LEA genes encode small hydrophilic proteins that function as protectors of macromolecules from aggregation or desiccation to enhance resistance against osmotic stress in plants (Ke et al. Citation2018). In sweet potato, the IbLEA gene that is highly associated with resistance to salt and drought stresses has been frequently reported (Wang et al. Citation2016; Zhai et al. Citation2016; Jia et al. Citation2017). These studies suggest that the up-regulation of IbLEA expression is consistent with the salt- and drought-tolerant phenotypes mediated by San01. Notably, Liu et al. (Citation2013) noted that the expression of ZmLEA3 was also induced by pathogen inoculation, implying that sweet potato plants may increase the expression of the IbLEA gene in response to San01 inoculation, and enhance the resistance to the salt and drought stresses incidentally. In addition to the up-regulation of the IbLEA gene, the expressions of IbNHX2, IbNCED, and IbSUT4 were also increased after the inoculation under long-term salinity. It has been proposed that the up-regulation of the IbNCED gene might lead to the activation of abscisic acid signaling, and further up-regulate the expressions of stress-responsive genes, such as IbLEA, IbNHX2, and IbSUT4, in sweet potato (Wang et al. Citation2016; Wang et al. Citation2020). Accordingly, the up-regulation of IbLEA, IbNHX2, IbSUT4, and IbNCED genes suggested that San01 may participate in salt tolerance through abscisic acid signaling.
Notably, although the San01 colonization enhanced tolerance to salt and drought stress in the inoculated plants, the colonized population of San01 in plants was decreased at 14 days of both abiotic treatments (). Generally, bacterial colonization ability is determined by their vitality and survival ability in environmental constraints, especially for soils (Costa et al. Citation2020). Similarly, the colonization potential of bacterial isolates in the rhizosphere was limited by abiotic stress (Gupta et al. Citation2019; Gupta et al. Citation2020). Thus, the detrimental impacts of salinity and water deficiency on the growth of microbes and plants might be the reason for the decrease of San01 colonization in sweet potato roots.
In conclusion, this study characterizes an endophytic Klebsiella sp. strain San01 from sweet potato roots and provides information on the interaction between Klebsiella sp. San01 and sweet potato plants. Under salinity and drought stresses, Klebsiella sp. San01 inoculation promotes sweet potato growth performance by increasing the photochemical reaction and alleviates the negative impacts of drought and high salinity by increasing antioxidant abilities and upregulating the transcript levels of IbLEA, IbNHX2, IbNCED, and IbSUT4 genes. These improvements in performance mediated by San01 offer considerable potential for sustainable production of leafy vegetable sweet potato cultivars in arid and saline areas.
Supplemental Material
Download TIFF Image (8.8 MB)Supplemental Material
Download PDF (89.5 KB)Acknowledgements
The authors thank Dr. Sixin Qiu and Dr. Yongxiang Qiu (Fujian Academy of Agricultural Sciences) for providing sweet potato cv. Fucaishu 18. QL and S-PC conceived and designed the research. QL, ZH, SH, CD, and K-HL performed the experiments. QL, ZH and S-PC analyzed the data. K-HL and S-PC wrote the manuscript. All authors have read and agreed to the final version of the manuscript.
Data availability statement
The datasets generated during and/or analyzed during the current study are available from the corresponding author on reasonable request.
Additional information
Funding
Notes on contributors
Qing Li
Qing Li obtained his Master degree in Agricultural Sciences, Fujian Agriculture and Forestry University.
Zhehong Huang
Zhehong Huang obtained his Master degree in Agricultural Sciences, Fujian Agriculture and Forestry University.
Caisheng Deng
Caisheng Deng obtained his Bachelor's degree in Agricultural Sciences, Sichuan Agricultural University.
Kuan-Hung Lin
Kuan-Hung Lin is a professor at Department of Horticulture and Biotechnology, Chinese Culture University.
Shumei Hua
Shumei Hua is an associate research fellow at Institute of Dryland Crops, Sanming Academy of Agricultural Sciences.
Shi-Peng Chen
Shi-Peng Chen is an associate research fellow at Institute of Dryland Crops, Sanming Academy of Agricultural Sciences. His research interests focus on the plant defense mechanisms in response to environmental stress and the interaction between crops and beneficial endophytes.
References
- Adhikari Dhungana S, Adachi F, Hayashi S, Raj Puri R, Itoh K. 2018. Plant growth promoting effects of Nepalese sweet potato endophytes. Horticulturae. 4:53.
- Barnawal D, Bharti N, Pandey SS, Pandey A, Chanotiya CS, Kalra A. 2017. Plant growth-promoting rhizobacteria enhance wheat salt and drought stress tolerance by altering endogenous phytohormone levels and TaCTR1/TaDREB2 expression. Physiol Plant. 161:502–514.
- Cardoso P, lves AA, Silveira P, Sá C, Fidalgo C, Freitas R, Figueira E. 2018. Bacteria from nodules of wild legume species: Phylogenetic diversity, plant growth promotion abilities and osmotolerance. Sci Total Environ. 645:1094–1102.
- Chang S, Puryear J, Cairney J. 1993. A simple and efficient method for isolating RNA from pine trees. Plant Mol Biol Rep. 11 (2):113–116.
- Chen W, Lin F, Lin KH, Chen C, Kuo YW. 2022. Growth promotion and salt-tolerance improvement of Gerbera jamesonii by root colonization of Piriformospora indica. J Plant Growth Regul. 41:1219–1228.
- Chiang CM, Kuo WS, Lin KH. 2014. Cloning and gene expression analysis of sponge gourd ascorbate peroxidase gene and winter squash superoxide dismutase gene under respective flooding and chilling stresses. Hortic Environ Biotechnol. 55 (2)129–137.
- Costa P, Elsas J, Mallon C, Borges L, Passaglia L. 2020. Efficiency of probiotic traits in plant inoculation is determined by environmental constrains. Soil Biol Biochem. 148:107893.
- de Albuquerque TMR, Sampaio KB, de Souza EL. 2019. Sweet potato roots: unrevealing an old food as a source of health promoting bioactive compounds–A review. Trends Food Sci Tech. 85:277–286.
- Eid AM, Fouda A, Abdel-Rahman MA, Salem SS, Elsaied A, Oelmüller R, Hijri M, Bhowmik A, Elkelish A, Hassan SE-D. 2021. Harnessing bacterial endophytes for promotion of plant growth and biotechnological applications: An overview. Plants. 10:935.
- Gordon SA, Weber RP. 1951. Colorimetric estimation of indole acetic acid. Plant Physiol. 26:192–195.
- Gupta P, Kumar V, Usmani Z, Rani R, Chandra A, Gupta VK. 2019. A comparative evaluation towards the potential of Klebsiella sp. and Enterobacter sp. in plant growth promotion, oxidative stress tolerance and chromium uptake in Helianthus annuus (L.). J Hazard Mater. 377:391–398.
- Gupta P, Kumar V, Usmani Z, Rani R, Chandra A, Gupta VK. 2020. Implications of plant growth promoting Klebsiella sp. CPSB4 and Enterobacter sp. CPSB49 in luxuriant growth of tomato plants under chromium stress. Chemosphere. 240:124944.
- Hita DD, Fuentes M, Zamarreo AM, Ruiz Y, Garcia-Mina JM. 2020. Culturable bacterial endophytes from sedimentary humic acid-treated plants. Front Plant Sci. 11:837.
- Hwang SY, VanToai TT. 1991. Abscisic acid induces anaerobiosis tolerance in corn. Plant Physiol. 97:593–597.
- Jia LC, Zhai H, Shao-Zhen HE, Yang YF, Liu QC. 2017. Analysis of drought tolerance and genetic and epigenetic variations in a somatic hybrid between Ipomoea batatas (L. Lam. and I. Triloba L.). J Integr Agr. 16:36–46.
- Joe MM, Devaraj S, Benson A, Sa T. 2016. Isolation of phosphate solubilizing endophytic bacteria from Phyllanthus amarus Schum & Thonn: evaluation of plant growth promotion and antioxidant activity under salt stress. J Appl Res Med Aroma. 3:71–77.
- Kaushal M, Wani SP. 2016. Rhizobacterial-plant interactions: strategies ensuring plant growth promotion under drought and salinity stress. Agr Ecosyst Environ. 231:68–78.
- Ke Q, Park SC, Ji CY, Kim HS, Wang Z, Wang S, Li H, Xu B, Deng X, Kwak SS. 2018. Stress-induced expression of the sweetpotato gene IbLEA14 in poplar confers enhanced tolerance to multiple abiotic stresses. Environ Exp Bot. 156:261–270.
- Khan Z, Doty SL. 2009. Characterization of bacterial endophytes of sweet potato plants. Plant Soil. 322:197–207.
- Kim SH, Ahn YO, Ahn MJ, Jeong JC, Lee HS, Kwak SS. 2013. Cloning and characterization of an Orange gene that increases carotenoid accumulation and salt stress tolerance in transgenic sweetpotato cultures. Plant Physiol Biochem. 70:445–454.
- Kitayama M, Samphumphuang T, Tisarum R, Theerawitaya C, Cha-um K, Takagaki M, Cha-um S. 2020. Calcium and soluble sugar enrichments and physiological adaptation to mild NaCl salt stress in sweet potato (Ipomoea batatas) genotypes. J Hortic Sci Biotech. 95:782–793.
- Komaresofla BR, Alikhani HA, Etesami H, Khoshkholgh-Sima NA. 2019. Improved growth and salinity tolerance of the halophyte salicornia sp. by co–inoculation with endophytic and rhizosphere bacteria. Appl Soil Ecol. 138:160–170.
- Ksouri R, Megdiche W, Debez A, Falleh H, Grignon C, Abdelly C. 2007. Salinity effects on polyphenol content and antioxidant activities in leaves of the halophyte Cakile maritima. Plant Physiol Biochem. 45 (3-4):244–249.
- Koźmińska A, Wiszniewska A, Hanus-Fajerska E, Boscaiu M, Hassan MA, Halecki W, Vicente O. 2019. Identification of salt and drought biochemical stress markers in several Silene vulgaris populations. Sustainability. 11:800.
- Lai Y. 2008. Breeding of the new sweet potato variety, Tainung no. 73. J Taiwan Agric Res. 57:279–294.
- Liu D, He S, Zhai H, Wang L, Zhao Y, Wang B, Li R, Liu Q. 2014. Overexpression of IbP5CR enhances salt tolerance in transgenic sweetpotato. Plant Cell Tiss Org. 117 (1):1–16.
- Liu Y, Wang L, Xing X, Sun L, Pan J, Kong X, Zhang M, Li D. 2013. ZmLEA3, a multifunctional group 3 LEA protein from maize (Zea mays L.), is involved in biotic and abiotic stresses. Plant Cell Physiol. 54 (6):944–959.
- Marasco R, Rolli E, Ettoumi B, Vigani G, Mapelli F, Borin S, Abou-Hadid AF, El-Behairy UA, Sorlini C, Cherif A. 2012. A drought resistance-promoting microbiome is selected by root system under desert farming. PLoS One. 7 (10):e48479.
- Marques JM, da Silva TF, Vollu RE, de Lacerda JRM, Blank AF, Smalla K, Seldin L. 2015. Bacterial endophytes of sweet potato tuberous roots affected by the plant genotype and growth stage. Appl Soil Ecol. 96:273–281.
- Mevy JP, Guibal F, Lecareux C, Miglietta F. 2020. The decline of Fraxinus angustifolia Vahl in a Mediterranean salt meadow: chlorophyll fluorescence measurements in long-term field experiment. Estuar Coast Shelf S. 247:107068.
- Mukherjee T, Banik A, Mukhopadhyay SK. 2020. Plant growth-promoting traits of a thermophilic strain of the Klebsiella group with its effect on rice plant growth. Curr Microbiol. 77 (10):2613–2622.
- Nakano Y, Asada K. 1981. Hydrogen peroxide is scavenged by ascorbate-specific peroxidase in spinach chloroplasts. Plant Cell Physiol. 22:867–880.
- Nguyen HC, Chen CC, Lin KH, Chao PY, Huang MY. 2021. Bioactive compounds, antioxidants, and health benefits of sweet potato leaves. Molecules. 26 (7):1820.
- Otieno N, Lally RD, Kiwanuka S, Lloyd A, Ryan D, Germaine KJ, Dowling DN. 2015. Plant growth promotion induced by phosphate solubilizing endophytic pseudomonas isolates. Front Microbiol. 6:745.
- Park SC, Kim YH, Jeong JC, Kim CY, Lee HS, Bang JW, Kwak SS. 2011. Sweetpotato late embryogenesis abundant 14 (IbLEA14) gene influences lignification and increases osmotic- and salt stress-tolerance of transgenic calli. Planta. 233 (3):621–634.
- Pramanik K, Mitra S, Sarkar A, Soren T, Maiti TK. 2017. Characterization of cadmium-resistant Klebsiella pneumoniae MCC 3091 promoted rice seedling growth by alleviating phytotoxicity of cadmium. Environ Sci Pollut R. 24:24419–24437.
- Puri RR, Dangi SR, Dhungana SA, Itoh K. 2018. Diversity and plant growth promoting ability of culturable endophytic bacteria in Nepalese sweet potato. Adv Microbiol. 08 (09):734–761.
- Ruan L, Chen L, Chen Y, He J, Zhang W. 2012. Expression of Arabidopsis HOMEODOMAIN GLABROUS 11 enhances tolerance to drought stress in transgenic sweet potato plants. J Plant Biol. 55 (2):151–158.
- Saitou N, Nei M. 1987. The neighbor-joining method: a new method for reconstructing phylogenetic trees. Mol Biol Evol. 4:406–425.
- Singh RP, Jha P, Jha PN. 2015. The plant-growth-promoting bacterium Klebsiella sp. SBP-8 confers induced systemic tolerance in wheat (Triticum aestivum) under salt stress. J Plant Physiol. 184:57–67.
- Sumanta N, Haque CI, Nishika J, Suprakash R. 2014. Spectrophotometric analysis of chlorophylls and carotenoids from commonly grown fern species by using various extracting solvents. Res J Chem Sci Res J Chem Sci. 2231:606X.
- Takahashi K, Rahman NA, Katayama T, Kasan A, Yamada Y. 2020. Taxon-and growth phase-specific antioxidant production by chlorophyte, bacillariophyte, and haptophyte strains isolated from tropical waters. Front Bioeng Biotech. 8:581628.
- Tamura K, Dudley J, Nei M, Kumar S. 2007. MEGA4: molecular evolutionary genetics analysis (MEGA) software version 4.0. Mol Biol Evol. 24 (8):1596–1599.
- Ullah A, Nisar M, Ali H, Hazrat A, Yang X. 2019. Drought tolerance improvement in plants: an endophytic bacterial approach. Appl Microbiol Biot. 103:7385–7397.
- Wang B, Zhai H, He S, Zhang H, Ren Z, Zhang D, Liu Q. 2016. A vacuolar Na+/H+ antiporter gene, IbNHX2, enhances salt and drought tolerance in transgenic sweetpotato. Sci Hortic. 201:153–166.
- Wang D, Liu H, Wang H, Zhang P, Shi C. 2020. A novel sucrose transporter gene IbSUT4 involves in plant growth and response to abiotic stress through the ABF-dependent ABA signaling pathway in sweetpotato. BMC Plant Biol. 20:157.
- Weatherley PE. 1950. Studies in the water relation of cotton plant. I. The field measurement of water deficits in leaves. New Phytol. 49 (1):81–97.
- Wintermans J, De Mots A. 1965. Spectrophotometric characteristics of chlorophylls a and b and their phenophytins in ethanol. Biophys Acta. 109:448–453.
- Wu Z, Kong Z, Lu S, Huang C, Huang S, He Y, Wu L. 2018. Isolation, characterization and the effect of indigenous heavy metal-resistant plant growth-promoting bacteria on sorghum grown in acid mine drainage polluted soils. J Gen Appl Microbiol. 65 (5):254–226.
- Yan H, Li Q, Park SC, Wang X, Liu YJ, Zhang YG, Tang W, Kou M, Ma DF. 2016. Overexpression of CuZnSOD and APX enhance salt stress tolerance in sweet potato. Plant Physiol Biochem. 109:20–27.
- Yooyongwech S, Theerawitaya C, Samphumphuang T, Cha-Um S. 2013. Water-deficit tolerant identification in sweet potato genotypes (Ipomoea batatas (L.) Lam.) in vegetative developmental stage using multivariate physiological indices. Sci Hortic. 162:242–251.
- Zhai H, Wang F, Si Z, Huo J, Xing L, An Y, He S, Liu Q. 2016. A myo-inositol-1-phosphate synthase gene, IbMIPS1, enhances salt and drought tolerance and stem nematode resistance in transgenic sweet potato. Plant Biotech J. 14 (2):592–602.
- Zhang H, Gao X, Zhi Y, Li X, Zhang W, Niu J, Wang J, Zhai H, Zhao N, Li J, et al. 2019a. A non-tandem CCCH-type zincfinger protein, IbC3H18, functions as a nuclear transcriptional activator and enhances abiotic stress tolerance in sweet potato. New Phytol. 223 (4):1918–1936.
- Zhang Y, Deng G, Fan W, Yuan L, Zhang P. 2019b. NHX1 and eIF4A1-stacked transgenic sweetpotato shows enhanced tolerance to drought stress. Plant Cell Rep. 38 (11):1427–1438.