ABSTRACT
Plant genotype and agroecology influence the composition and functionality of seed endophytic bacterial communities. Taxonomic analysis of 123 wheat seeds endophytic bacteria classified these into 23 genera predominantly under Firmicutes followed by Actinobacteria and Proteobacteria. Genus Bacillus was most abundant (30.7%) followed by Streptomyces (18.4%) with other representative genera such as Stenotrophomonas, Paenibacillus, Mixta, Enterobacter, Micrococcus, Pantoea, Alkalihalobacillus, Cortiobacterium, and more. Across agroecologies, the core microbiota of seeds consists of Bacillus, Streptomyces, Paenibacillus, and Stenotrophomonas, with maximum diversity and abundance observed in seeds of the North Western Plain Zone of India. Seed endophytic bacteria had PGP traits; nitrogen fixation (n = 101), production of IAA (n = 65), siderophores (n = 43), ammonia (n = 82) and solubilization of phosphate (n = 47), potassium (n = 37), and zinc (n = 8). The isolates produced HCN and hydrolytic enzymes and displayed antagonism against fungal pathogens. Overall, the information generated on wheat seeds’ endophytic bacterial taxonomy and beneficial traits may pave the path for the development of novel bioinoculants.
Key message
Wheat seeds from various agro-ecologies of India harbor diverse endophytic bacteria.
Firmicutes were dominant followed by Actinobacteria and Proteobacteria.
Genus Bacillus, Stenotrophomonas, Streptomyces, and Paenibacillus were core endophytic bacteria in different agro-ecologies.
The endophytic bacterial strains were displaying diverse functional traits.
Introduction
Wheat is one of the most economic important crops that played a major role in global food security along with rice and maize. It supplies almost a fifth of global food calories and protein as a balanced food to millions of people each day. It is a significant and the second-largest cereal crop grown for grains worldwide. The domestication of wheat occurrs on diverse continents and exhibits enormous genetic diversity within it. The growing world population and climate change are two major factors, which dictate the need to develop methods to increase crop production. It is grown in India on about 30 million hectares with a production of about 113 million tons, and the cultivation area is divided into six agro-climatic zones viz. northern hill zone (NHZ), northern western plain zone (NWPZ), northern eastern plain zone (NEPZ), central zone (CZ), peninsular zone (PZ), and southern hill zone (SHZ). Majorly three wheat species are cultivated under these zones including Triticum aestivum (Bread wheat), T. durum, and T. dicoccum. T. aestivum is grown throughout the zones, while T. durum is mainly grown in CZ and PZ and T. dicoccum is limited to PZ. According to data availability, around 294 wheat genotypes have been cultivated under different agro-climatic and cultural conditions (Gupta et al. Citation2018).
Plant harbors diverse microbial population colonized in its different niches such as endosphere, phyllosphere, and rhizosphere. These were observed on or within the host plant’s vegetative and reproductive organs (Turner et al. Citation2013; Truyens et al. Citation2015). In the last two decades, endophytes were more emphasized because of their nature as these endophytic microbes reside within the host tissues without causing any visible adverse impact on the host (Zhang et al. Citation2006; Reinhold-Hurek and Hurek Citation2011). Previous studies displayed diverse bacterial taxa using culture-dependent and independent analysis in different host plants, which have originated through vertical transfer from seeds (maternally transmitted) or from the soil via a horizontal transmission (Bright and Bulgheresi Citation2010; Suman et al. Citation2021). The hologenome theory of evolution displayed that the host and its associated beneficial microbiome have coevolved for mutual interactions (Zilber-Rosenberg and Rosenberg Citation2008). Bacterial endophytes play a role in nutrition acquisition, growth regulation, and defense through mineral solubilization (P, K, Zn), nitrogen fixation, siderophore production, and hormones such as auxin, cytokinin, and gibberellic acid, enzymes such as cellulase, amylase, pectinase, chitinases, and protease and antimicrobial compounds to inhibit pathogens. Such kind of interaction of exchange is possible through bacterial colonization and community formation within or on the plant host surface (Lugtenberg et al. Citation2002).
Microbes associated with seeds contribute significantly to the plant life cycle in addition to having possible effects on their host plant. They can assist with seed health and germination preparation initially (Chee-Sanford et al. Citation2006). The second possibility is that these microorganisms can serve as the first members of the bacterial communities that would eventually form the newly formed plant and benefit their hosts. Most importantly, plants can use seeds as microbial vectors for the multigenerational preservation of these advantageous relationships (Truyens et al. Citation2015). Moreover, it has been noticed that the seed microbiota functions as the new seedling microbiome's community assembly's starting point and finishing point (Shade et al. Citation2017; Shahzad et al. Citation2018). As a result, the bacteria linked with seeds were called seed-borne or seed-transmitted microorganisms (Truyens et al. Citation2014; Shade et al. Citation2017). Robinson et al. (Citation2016) and Shade et al. (Citation2017) reported that the structure of the plant microbiome and, eventually, plant production, are significantly influenced by microbial transfer through seeds. The previous studies displayed that the microorganism resides in the different parts of seeds; Fusarium and Epichloe from wheat embryos (Robinson et al. Citation2016), Clavibacter and Verticillium from tomato endosperm (Tancos et al. Citation2013) although grape vine seed coat harbors Bacillus and Alternaria (Compant et al. Citation2011). In recent years, the seed endophytic microorganisms of rice and maize have been explored (Liu et al. Citation2013; Rijavec et al. Citation2007; Liu et al. Citation2017; Shahzad et al. Citation2017; Verma et al. Citation2017). Kuźniar et al. (Citation2020) reported the existence of Stenotrophomonas, Acinetobacter, Pseudomonas, Pantoea, Paenibacillus, Paracoccus, and Flavobacterium in Triticum aestivum and T. spelta seeds. Herrera et al. (Citation2016) recovered Bacillus, Paenibacillus, and Pantoea from endosperms of wheat. Similarly, Robinson et al. (Citation2016) recovered Paenibacillus and Erwinia from T. aestivum. Although sporadic information about wheat seed endophytes is available no systematic study is available to show the impact of agro-ecology on the wheat seed endophytic population, their type, and role.
The current study objective was to determine the most important, beneficial seed endophytic bacteria that might be used to create a generic formulation that would work in all agro-ecologies. The researchers focused on the diversity and functionality of the culturable microbiome of wheat seeds of different genotypes growing in six agro-climatic zones of India.
Materials and methods
Agro-ecological zones, wheat genotypes, and seed sampling
Seeds of 12 popular wheat genotypes, two from each of six major agro-ecological zones representing three species of wheat viz. T. aestivum, T. dicoccum, and T. durum were collected. The agro-climatic zones are the northern hill zone (NHZ), northern western plain zone (NWPZ), northern eastern plain zone (NEPZ), central zone (CZ), peninsular zone (PZ), and southern hill zone (SHZ). From each zone, seed samples were collected from five locations to make a composite sample (). The seed samples were collected and kept at 4°C until use in sterile polybags.
Table 1. Summary of wheat seeds used as a source for isolation of endophytic bacteria from different sampling sites with physio-chemical characteristics.
Surface sterilization and isolation of bacterial endophytes from wheat seeds
The seed sterilization and bacterial endophyte isolation method described in the previous study were used (Sharma et al. Citation2022), in which, seeds were surface sterilized by sequential disinfection with ethanol (70%), sodium hypochlorite (1%), again with ethanol, and finally rinsed with sterile distilled water for three times. The effectiveness of the sterilization method was determined by inoculating the third rinse of sterile water (1 ml) in nutrient broth and observed for a week at 30 ± 2°C.
After seed sterilization, seeds were ground into powder using a sterile pestle and mortar and serially diluted in the 0.8% (w/v) saline solution and for isolation of bacterial endophytes, 1 ml pour plated on different isolating media, including nutrient agar (NA), tryptic soy agar (TSA), starch casein agar (SCA), soybean casein digest agar, and wheat seed medium agar (WSM) (supplementary Table 1), and incubated at 30 ± 2°C for 7 days. The plates were examined regularly for bacterial growth and were picked up based on their morphological appearance. Furthermore, the selected isolates were maintained on nutrient agar and preserved at −20°C in 30% (v/v) glycerol.
Genotypic and phylogenetic analysis of bacterial endophytes
The method defined in the previous study was used for the isolation of genomic DNA and amplification of the 16S rRNA gene of selected seed bacterial endophytes (Sharma et al. Citation2022). The amplified PCR product was purified and sequenced by Agrigenome Pvt. Ltd., India. The obtained raw sequences were processed for making contigs using BioEdit software and checked for chimera using the online software DECIPHER 3.0. The phylogenetic analysis was performed with a neighbor-joining method in MEGA X software. The Kimura 2-parameter model was used to create the tree's topography, which was then analyzed using a bootstrap analysis by resampling 1000 times (Kimura Citation1980; Felsenstein Citation1985). The 16S rRNA gene sequences of the isolates have been submitted by GenBank of the National Centre for Biotechnological Information (http://www.ncbi.nlm.nih.gov/genebank/) (NCBI).
Functional characterization of wheat seed endophytic bacteria
Wheat seed bacterial endophytes were assessed for various plant growth promoting (PGP) traits viz. nutrients (phosphorus, zinc, and potassium) solubilization, nitrogen fixation, growth hormone (IAA) production, extracellular hydrolytic enzyme production (amylase, cellulase, protease, xylanase, and lipase), and antagonistic activity against wheat major pathogens (Fusarium garminearum, Bipolaris sorkiniana, Alternaria sp., and Tilletia indica). Tricalcium phosphate (TCP) was used as an insoluble phosphorous substrate, and the solubilization of phosphate was qualitatively evaluated by spotting on Pikovskaya agar medium plates. After seven days of incubation at 30 ± 2°C, the development of a halo zone surrounding the colonies was seen as positive for phosphate solubilization. Similarly, a modified Aleksandrov’s agar medium (pH 7.2 ± 0.2) with 0.2% (w/v) potassium aluminosilicate as a potash source was used for qualitative evaluation of K mobilization. The isolates were spotted and incubated for 96 h at 30 ± 2°C. The appearance of a clear zone around the bacterial colony was considered positive K solubilizer. The zinc solubilizing activity of isolates was assessed on Tris-HCl minimal salt medium supplemented with 14 mM zinc oxide (insoluble) as a zinc substrate. The fresh isolates were spotted and incubated at 30 ± 2°C for 5 days. The clear zone surrounding spots displays zinc-solubilizing capability. The modified plate assay method defined by Schwyn and Neilands (Citation1987) was used to assess the isolates for siderophore production. It dissolved 60.5 mg of chrome azurol S dye in 50 ml of distilled water and was combined with the 10 mL of Fe(III) solution (1 mM FeCl3 in 10 mM HCl). With constant stirring, this solution was added to 40 mL of hexa-decyl-trimethyl ammonium bromide (72.9 mg) before being autoclaved for 15 min at 121°C. 900 mL of autoclaved nutritional agar (pH 6.8) was gently incorporated into the final solution before being put into sterile petri plates. Freshly grown bacterial inoculum (10 µL) was spotted and incubated for 7 days at 30 ± 2°C. A yellow-orange halo zone that appears surrounding the colony was considered positive for siderophore formation.
The plate screening method of Latt et al. (Citation2018) was used to check the ability of isolates for nitrogen fixation on glucose nitrogen-free mineral medium. The fresh isolates were spotted and incubated at 30 ± 2°C for 7 days. The blue color of the growth medium around the spot was recorded as a positive result for nitrogen fixation. Patten and Glick (Citation2002) described the colorimetric method used in the study for the detection of IAA using the Salkowski reagent. The isolates were cultured in nutrient broth in an incubator shaker at 30 ± 2°C for 7 days. Salkowski reagent drops (3–5) were added to the cultured medium and color changes of medium to pink was recorded as a positive result.
Extracellular hydrolytic enzyme production was screened using appropriate substrates. The isolates were spotted onto nutrient agar plates supplemented with 1% soluble starch and cultured for 5 days at 30 ± 2°C to determine their amylase activity. After incubation, lugol's iodine solution was poured over each plate. It was determined how far the isolates were from the stained area. CMC agar with carboxy methyl cellulose (1%) was used for the detection of cellulase activity. Cultures were spotted and incubated at 30 ± 2°C for 5 days. The plates were flooded with Gram’s iodine and a clear zone around the spot was measured and considered positive. The isolate’s protease activity was tested on nutrient agar plates with skimmed milk (1.5%, w/v) and incubated at 30 ± 2°C for 5 days. The clear zone surrounding the colonies was recorded. Sharma et al. (Citation2013) method was used to check the xylanase activity of isolates using birchwood xylan. The lipase activity of isolates was examined on media (peptone, 10.0; NaCl, 5.0; CaCl2, 0.10; Tween-20, 10 ml; agar, 16.0; pH, 7.2 ± 0.2) and incubated for 5 days at 30 ± 2°C and white precipitation around colony was observed and considered positive enzyme activity.
Four fungal pathogens (F. graminearum, Alternaria sp., B. sorokiniana, and T. indica) were used to evaluate the antagonistic activity of the isolates. The dual-culture agar plate method described by Semwal et al. (Citation2018) was used, in which, an 8 mm fungal pathogen plug was placed at the center and the isolates were spotted 4 cm away. Plates were incubated for 7 days at 30 ± 2°C and a zone of inhibition was recorded.
Statistical analysis
Diversity indices of cultured bacterial seed endophytes were calculated to observe the richness and evenness of culturable microbial load present in variable genotypes of particular zone. Diversity indices were estimated by using the mean value from OTU tables rarefied to even depth. The PAST software tool, version 3.0, was used to carry out this diversity analysis (Hammer et al. Citation2001). A rarefaction curve and Hill’s series of alpha diversity were compared for the relative diversity in agri-ecological zones. Similarly, the relationship between the isolates at the genera level was understood by Principal coordinate analysis (PCoA) and Pearson correlation coefficient (r), performed using PAST software. Upset plots were generated to understand the distribution and shared isolates at the genus level across the various zones, performed using the UpSetR Shiny app (Lex et al. Citation2014).
Results
Isolation of wheat seed endophytic bacteria
Culturing different wheat seed samples from six different agro-ecological zones of India yielded innumerable colony morphological distinct bacterial morphotypes. For the enumeration of culturable bacteria, five media were employed to recover maximum bacterial diversity. These media include nutrient agar (a nutrient-rich medium for the cultivation of non-fastidious microorganisms), tryptic soya agar (medium with glucose, dipotassium hydrogen phosphate, and sodium chloride is suitable for fastidious and non-fastidious microorganisms), starch casein agar (a nutrient-rich medium for the isolation of saccharolytic bacteria), a wheat matrix medium (a specific medium designed to isolate non-fastidious microorganism) and soybean casein digest agar with bromo-cresol purple (a medium for a variety of microorganisms). The obtained bacteria displayed different colony morphologies with variability in their color (white, off-white, yellow, and orange gray), shape (circular, irregular, filamentous, and oval), size (pinpoint, small, medium, and large), margin (entire, irregular, wavy irregular, and filiform), and elevation (flat, raised, umbonate, and crateriform). The bacterial counts in the Northern Hill zone ranged from 35.3 ± 2.4 to 305.7 ± 24.6 CFU/g of seed. Similarly, 21 ± 1.1-271.7 ± 24.7 CFU/g, 16.3 ± 0.9–507.7 ± 21.1 CFU/g, 17 ± 1.0 −271.3 ± 7.7 CFU/g, 37.3 ± 5.4–835.7 ± 28.9 CFU/g, and 28.3 ± 4.1–671.3 ± 30.0 CFU/g range of bacterial population were obtained from NWPZ, NEPZ, CZ, PZ, and SHZ respectively (). The maximum bacterial population was recovered on nutrient agar and wheat matrix medium (). A total of 193 bacterial endophytes were purified based on of their morphological appearance on different isolating mediums, of which 123 morphotypes were selected (Supplementary Figure 1) and preserved in glycerol stock for further study.
Table 2. Enumeration and selection of seed endophytic bacteria (CFU/g) from wheat genotypes on different growth media.
Taxonomic diversity of wheat seeds’ endophytic bacteria
On screening further for phenotypic duplication among 193 isolates on nutrient agar, 123 isolates were selected and identified by 16S rRNA gene sequencing. These sequences were submitted to NCBI GenBank with the following accession numbers: OP658890-OP658911 (NHZ), OP648194-OP648224 (NWPZ), OP648226-OP648244 (NEPZ), OP630927-OP630946 (CZ), OP658875-OP658889 (PZ), and OP630954-OP630969 (SHZ). The bacterial isolates were identified as 23 genera with 66 different species having 98-100% similarity with the nearest type strain (Supplementary Table 2). Bacterial isolates were distributed within phyla Firmicutes, Actinobacteria, and Proteobacteria, with 7 genera in Firmicutes viz. Bacillus, Paenibacillus, Priestia, Alkalihalobacillus, Brevibacillus, Staphylococcus, and Cytobacillus. There are 7 genera of Actinobacteria including Streptomyces, Nocardiopsis, Micrococcus, Kocuria, Nocardia, Pseudarthrobacter, and Cellulosimicrobium. There are nine genera in phylum Proteobacteria, including Stenotrophomonas, Enterobacter, Mixta, Pseudomonas, Acinetobacter, Pantoea, Yokenella, Klebsiella, and Tianweitania (). Four isolates WSB14, WSB69, WSB70, and WSB119, displaying a similarity of 97.79%, 97.91%, 96.77%, and 97.75% with the nearest strain Paenibacillus silagei, Priestia aryabhattai, Streptomyces malachitofuscus, and Priestia aryabhattai, respectively established as a unique sub-clade demonstrating their status as potential new species (Supplementary Figure 2). Three genera Bacillus, Streptomyces, and Stenotrophomonas were common among seeds of all wheat varieties.
Wheat seed endophytic bacterial community
On screening further for phenotypic duplication among 193 isolates on nutrient agar, 123 isolates were selected and identified by 16S rRNA gene sequencing. These sequences were submitted to NCBI GenBank with the following accession numbers: OP658890-OP658911 (NHZ), OP648194-OP648224 (NWPZ), OP648226-OP648244 (NEPZ), OP630927-OP630946 (CZ), OP658875-OP658889 (PZ), and OP630954-OP630969 (SHZ). The bacterial isolates were identified as 23 genera with 66 different species having 98-100% similarity with the nearest type strain (Supplementary Table 2), The bacterial community profile of wheat seeds was be variable among different cultivation zones. Overall, the maximum bacterial endophytes belonged to Firmicutes (46.34%) followed by Actinobacteria (29.27%) and Proteobacteria (24.39%) (). Among Firmicutes, Bacillus (28.46%) was dominant followed by Paenibacillus (8.94%), Priestia (4.88%), Alkalihalobacillus (1.63%), Brevibacillus (0.81%), Staphylococcus (0.81%), and Cytobacillus (0.81%). Similarly, among Actinobacteria, Streptomyces (17.07%) was dominant followed by Nocardiopsis (5.69%), Micrococcus (2.44%), Kocuria (1.63%), Nocardia (0.81%), Pseudarthrobacter (0.81%), and Cellulosimicrobium (0.81%). While in Proteobacteria, Stenotrophomonas (7.32%) was observed as the dominant genera followed by Enterobacter (5.69%), Mixta (4.88%), Pseudomonas (1.63%), Acinetobacter (1.63%), Pantoea, Yokenella, Klebsiella, and Tianweitania showing 0.81% ().
Figure 2. Taxonomic diversity of the obtained representative bacterial endophytic isolates (a) Composition percentage of the isolate at phylum level (b) Abundance heat map of bacterial isolates at genus level present in varied locations.
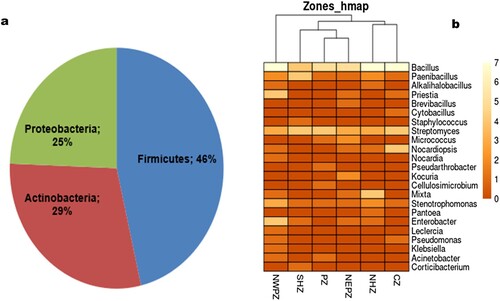
Table 3. Diversity of seed endophytic bacteria across wheat genotypes from different agro-ecological zones.
The composition of wheat seed endophytic bacteria was affected both by agro-ecological conditions and plant genotypes (Supplementary Figure 4). Across six agro-ecologies the seed bacterial community was grouped and the diversity profile of bacterial communities was plotted using Hill’s series ((b)). The plot displayed the global comparison of diversity indices, including Shannon and Simpson indices, richness and evenness, which are described as α = 1, α = 2, α = 0a and α = ∞, respectively. The alpha diversity indices of the bacterial communities vary among six zone samples ( and ). The NWPZ had the greatest Shannon's diversity index (2.35), Simpson's (0.88), and species’ richness values, whereas SHZ had the lowest (1.72). other parameters such as the Brillouin index, Chao-1, Evenness, and individual rarefaction curves confirm it ( and (a)). While the distribution of Actinobacterial and Firmicutes populations was shared in PZ and NEPZ. The relative abundance of the culturable bacterial community at the class and genus level is described in Supplementary Figure 3. The relative abundance of the bacterial community was found higher in seeds cultivated in NWPZ compared to other cultivation zones. Fourteen different bacterial genotypes were obtained from NWPZ followed by NEPZ (n = 11). While 9, 8, 8, and 7 different genera were obtained from NHZ, CZ, PZ, and CZ, respectively. Four genera, Bacillus, Paenibacillus, Streptomyces, and Stenotrophomonas, were found common in all the zones. At the genus level, Bacillus (n = 35) was the most abundant followed by Streptomyces (n = 23). Genus Enterobacter was observed in NHZ, NWPZ, SHZ, and NEPZ seed samples. Similarly, the genus Nocardiopsis was isolated from NHZ, NWPZ, CZ, and NEPZ. Alkalohalobacillus was obtained from NHZ and NWPZ. While the genus Mixta was obtained from seeds of Northern zone varieties. Acinetobacter was shared in NWPZ, PZ, and CZ, while Pseudomonas was shared in NWPZ and CZ.
Figure 3. A diversity profile of endophytic bacteria isolated from wheat seeds of different zones (a) Rarefaction and (b) Hill’s series.
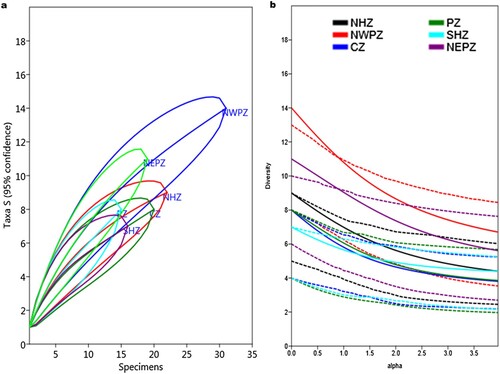
Figure 4. Bacterial alpha diversity indices among different zones. Indices calculated using PAST software after percentile type bootstrapping; Error bars indicate lower and upper values obtained after bootstrapping (N = 9999) (a) Shannon’s index (b) Simpson’s index (c) Evenness and (d) Chao1 index.
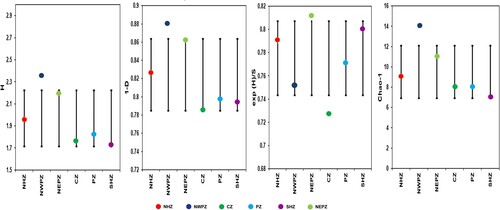
Table 4. Diversity indices of bacterial endophytes associated with seed across genotypes from diverse agro-ecological zones.
In NWPZ, seeds of two genotypes HD-2967 and HD-3117 yielded 19 and 12 bacterial isolates, respectively. Bacterial isolates Bacillus, Priestia, Streptomyces, and Stenotrophomonas genera were shared by both genotypes. While Paenibacillus, Nocardiopsis, Nocardia, Leclercia, Enterobacter, Pseudomonas, Klebsilla, and Acinetobacter were obtained in HD-2967 and Alkalihalobacillus and Mixta were from HD-3117. Similarly in another case from CZ, seeds of HI-1620 (T. durum) and HI-8713 (T. aestivum) were used and 9 and 11 isolates were recovered, respectively. It was found that only Bacillus and Streptomyces were shared, while Cytobacillus, Pseudomonas, and Stentrophomanas were recovered from HI-1620. Panibacillus, Priestia, and Nocardopsis were from HI-8713. This also displayed that Proteobacteria were found more dominant in the T. durum genotype (HI-1620) compared to T. aestivem (HI-8713), which were grown in the central zone.
Functional diversity of wheat seeds endophytic bacteria
All the isolates were studied in-vitro for beneficial traits for facilitating plant nutrient acquisition (phosphorus, potassium, nitrogen, zinc, and iron), growth promotion (auxin production) along with traits associated with antagonistic activity (cellulase, amylase, proteases, and antifungal activity against fungal pathogens). The most predominant functional traits of all the isolates were nitrogen fixation (89.43%), followed by ammonia production, cellulase activity, IAA production, protease activity, amylase activity, HCN production, phosphate solubilization, siderophore production, potassium solubilization, and xylanase activity, displaying 72.36%, 64.23%, 60.16%, 50.41%, 47.97%, 47.15%, 45.53%, 42.28%, 34.15%, and 19.51%, respectively. The rarest traits of the isolates were zinc solubilization (12.20%) and lipase production (1.63%). The maximum isolates were representing antagonistic activity against F. graminearum (61.79%) and the least against B. sorokiniana (24.39%). Of the strains that contribute various functional traits to their hosts, the genus Bacillus was dominating followed by Streptomyces. 32 of the strains from Bacillus and 21 from Streptomyces were those that contributed N-fixation potential to their hosts. Out of 65 strains that produced IAA, 14 belonged to the genus Streptomyces and 13 to the species Bacillus. Proteobacteria, such as those in the genera Stenotrophomonas, Mixta, Enterobacter, Pantoea, Pseudomonas, and Acinetobacter, were also helping their host by producing growth-regulating traits like the solubilization of phosphate and potassium, siderophore production, IAA, ammonia production, and nitrogen fixation ().
Table 5. Wheat seed endophytic bacterial genera-specific distribution of in vitro functional traits.
Agroecology-specific functional activities of wheat seed bacteria
All isolates recovered from NEPZ displayed nitrogen fixation trait followed by the isolates of NWPZ (96.77%), CZ (90.0%), SHZ (87.5%), PZ (80.0%), and NHZ (77.27%). Similarly for nutrition acquisition traits, maximum P, K, and Zn solubilizers were obtained from PZ (66.67%), NWPZ (48.39%), and NWPZ (19.35), respectively. Similarly, maximum siderophore (67.14%) and ammonia (84.21%) producers were obtained from NWPZ and NEPZ, respectively. Maximum growth hormone (IAA)-producing isolates (67.74%) were observed from NWPZ and more than 60% of bacterial isolates from NHZ, NEPZ, and SHZ displayed IAA production. A minimum IAA producer was observed from PZ. In the case of extracellular enzyme production highest amylase producers were obtained from CZ, protease producers from NEPZ, cellulase producers from SHZ and xylanase producer from CZ. While lipase producers were obtained from SHZ. Maximum isolates obtained from CZ were displaying antifungal activity against all fungal pathogens including F. garminearum, B. sorkiniana, Alternaria sp., and T. indica ( and Supplementary Figure 5).
Table 6. Agro-ecological zones-specific distribution of functional traits across wheat seed endophytic bacteria.
Discussion
The selection of certain crop qualitative traits during domestication has caused significant genetic, phonological, and physiological changes in plants. Plant as holobiont has innate microbial communities as plant microbiome that are affected by external elements such as soil types, nutrient availability, water, growing environment, etc. leaving a significant impact on plant characteristics (Hacquard Citation2016). Seeds, an important component of the plant life cycle, harbor microbial communities transmitted to it vertically through the plant itself and/or horizontally from the external environment. Microbial transmission in the seed may occur by the internal vascular system, stigma-mediated foliar transmission, and direct interaction with microbial populations present in surrounding reproductive organs, such as fruits and flowers (Barret et al. Citation2015; Nelson et al. Citation2018). The functionality of seed colonizers and their diversity is influenced by physiological changes that occur during seed development. After germination, the seed-associated endophytes may establish as the seedling microbiome and perform advantageous tasks for the host like protection from bacterial and fungal phytopathogens, nutrient mobilization, and growth-development activities (Truyens et al. Citation2015).Wheat, the most important staple cereal crop, has diverse genotypes and grown worldwide under different agro-ecologies. Much information is available about wheat genotypes in terms of their genetics, physiology, and agronomy, but with limited information related to crop-associated microbiomes. There are research gaps related to the persistence, assembly, and functionality of the seed microbiome, especially when grown across different ecologies. It is hypothesized that the most important wheat bacterial endophytes would be spread through seeds and preserved throughout the family, displaying a long evolutionary origin that preceded the diversity of species. In this study, 193 seed-associated bacterial endophytes were recovered from surface sterilized whole seeds of 12 wheat genotypes from six agro-climatic ecologies of India, representing 22 genera from three phylum, four classes and 13 families. Our results were consistent with the similar study performed by Khalaf and Raizada (Citation2016) and Aswini et al. (Citation2023), in which cucurbit and wheat seeds harbor more diverse bacterial endophytes. The study displayed that phylum Firmcutes was dominant and among which genus Bacillus was predominant.
The PCoA analysis was performed using normalized data of seed endophytic bacteria as affected both by agro-ecologies and genotypes. The scatter plot indicating 33.1 and 18.6% variance across two coordinates of PCoA of agro-ecolgies () indicated the close association of seven genera of which the genera Stentrophomonas, Bacillus, Paenibacillus, and Streptomyces were found in all ecologies, whereas Kocuria, Staphylococcus, and Brevibacterium were specific to particular zones. Genotype-based scatter plot (Supplementary Figure 6) indicated the close association between isolates Stentrophomonas, Bacillus, and Streptomyces. Furthermore, correlation analysis (Pearson’s) revealed a significant (p < 0.05) connection between the agro-ecological zones. The correlation coefficient (r) of 0.93, 0.89, 0.86, 0.80, 0.79, and 0.77 was observed for NWPZ, NEPZ, NHZ, CZ, SHZ, and PZ, respectively with a p-value of 0.00. In a similar study, Sahu et al. (Citation2022) displayed that rice varieties belonging to the same agro-climatic zone showed convergent phyllomicrobiome patterns, according to principal coordinate analysis. It is interesting to note that the rice genotype grown in the different zones showed different phyllomicrobiomes that may have been influenced by the environment.
Figure 5. Principal coordinate analysis based on Bray-Curtis dissimilarity matrix for the seed endophytic bacterial community plotted using PAST software.
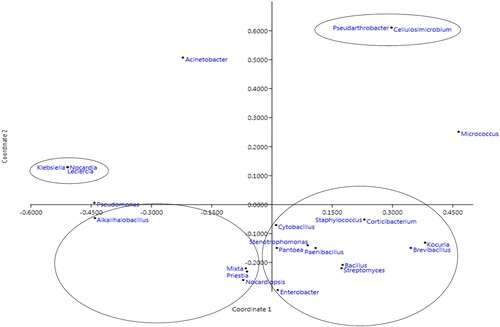
In spite of this diversity, seeds of different wheat genotypes have a core culturable, seed-associated microorganism, among which Bacillus was dominating (). In a similar study, Proteobacteria was dominant at early stages (after 10 days of flowering) such as Sphingomonas sp. and Methylobacterium sp. While on the later stage of flowering (after 20 days) Bacillus sp. and Curtobacterium sp. were dominating in rice seed. Furthermore, there is evidence that bacterial endophytic communities are more likely to accumulate during seed maturity due to the increase of starch and the loss of water (Mano et al. Citation2006; Shahzad et al. Citation2018).
Reports on metagenome analysis of seed endophytes of different plants indicate that phylum Proteobacteria is predominant, among which Gamma-proteobacteria was dominant followed by Actinobacteria and Firmicutes (Kuźniar et al. Citation2020; Graziano et al. Citation2022). Our results were consistent with the seed metagenomic analysis ((a)). Kuźniar et al. (Citation2020) reported seven bacterial genera cultured as dominant endophytes represented as Streptomyces, Paenibacillus, Serratia, Methanobacterium, Lactobacillus, Pantoea, and Masillain the internal compartment of seed (embryo and endosperm) of the two wheat varieties. They also observed that the two genera, Pantoea and Paenibacillus, dominantly colonized the endosperm of the seed portion.
In this study, the genus Bacillus was found to be the predominant core seed bacterial endophyte across all tested wheat genotypes. Streptomyces was the second dominant genus followed by Paenibacillus and Stenotrophomonas ((b)). These four genera accounted for more than 65% of the total endophytic isolates and are expected to be specially recruited by the host that might have a role in various microbial-induced host functional attributes. Earlier it was reported that the genera, Bacillus, Streptomyces, and Paenibacillus are ubiquitous bacterial genera in an agroecosystem (Gardener Citation2004; Sharma et al. Citation2022). Their cosmopolitan existence has been confirmed by culture-dependent and unculture-dependent techniques (Aswini et al. Citation2023). However, they are known for specific attributes that favor them as crop inoculants. They produce secondary metabolites, enzymes, hormones, nutrient mobilization, and spore-forming ability, which make them dominant in the agroecosystem. The spore-forming ability of these seed endophytic bacteria was an important characteristic, which helps bacteria to protect within seeds under adverse conditions such as desiccation, storage, seed germination, and maturation (Truyens et al. Citation2015; Rana et al. Citation2020). Because of this property genus, Bacillus has more importance and is commonly used in plant growth promotion activity for variable crops as biofertilizers (Borriss Citation2011). While Stenotrophomonas were also dominant and recovered from agroecosystem (Suman et al. Citation2016), it has gene exchange capability, displays resistance under adverse conditions, produces biofilm, and valueable compounds such as organic acid, antagonistic compound, etc (An and Berg Citation2018). Herrera et al. (Citation2016) reported that Bacillus and Paenibacillus were dominant in the endosperm of the wheat seed grain of T.aestivum. Similarly, Paenibacillus, Acinetobacter, Stenotrophomonas, Pantoea, Bacillus, Paracoccus, Pseudomonas, and Flavobacterium were predominant genera in the wheat seeds of two varieties, T. aestivum and T. spelta (Kuźniar et al. Citation2020). Furthermore, Bacillus and Paenibacillus were isolated and reported as seed endophytes from rice (Zhang et al. Citation2021a), maize (Bodhankar et al. Citation2017), cucurbit (Khalaf and Raizada Citation2016), Eucalyptus (Ferreira et al. Citation2008), and tomato (Xu et al. Citation2014).
Some rare unique bacterial genera were observed specific to different agro-ecologies. Nocardia, Leclercia, and Klebsiella were found to be specific to NWPZ; Brevibacillus and Kocuria to NEPZ; Corticibacterium and Staphylococcus to SHZ; Pseudarthrobacter and Cellulosimicrobium to PZ; Pantoea and Cytobacillus to NHZ and CZ, respectively ().
Figure 6. UpSet plot showing the distribution of seed colonizing bacterial genotypes in different zones: In a bar graph, each bar graph displays the sharing genus while intersections display genus in each zone.
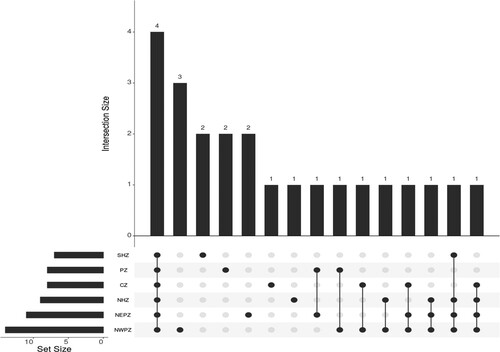
Seed-associated bacterial population displayed several potential functional traits including nitrogen fixation, phosphate and potassium solubilization, production of siderophore, HCN, ammonia hormones (IAA, GA, and cytokinin), and antagonistic activities against plant pathogens. Microorganisms displayed symbiotic association with the host plant to assist in biological nitrogen fixation. It was observed that 89.43% of seed bacterial endophytes could grow on N-free media, displaying either biological nitrogen-fixing or N-scavenging activity. Previous studies suggest that diverse bacterial genera including Bacillus, Paenibacillus, Stenotrophomonas, Streptomyces, Pantoea etc., can fix nitrogen and are used commercially as nitrogen fixers (Maheshwari Citation2011; Suman et al. Citation2016; Muresu et al. Citation2022). Nitrogen, phosphorous, and potassium are the most limiting mineral nutrients for plant growth and development. Despite their abundance in the soil, these are not directly available to plants because of low solubility (Weyens et al. Citation2009). Many microbes in the rhizosphere can solubilize these mineral nutrients through the production of organic acids or enzymes (Bulgarelli et al. Citation2015; Marag and Suman Citation2018). In this study, 45.53 and 34.15% of the isolates displayed P and K solubilization activity, respectively, of which Bacillus isolates were predominant followed by Stenotrophomonas, Mixta, Enterobacter, Streptomyces, Priestia, and Acinitobacter. Our study is consistent with previous reports (Johnston-Monje and Raizada Citation2011; Devi et al. Citation2022) and these isolates may have the potential for future sustainable crop improvement.
Zinc is required as a micronutrient for plant growth and the availability of zinc to plants is critical, its deficiency displayed adverse effects on soil, plant, and grains (Hafeez et al. Citation2013). The zinc content in soil is present in lesser amounts and not directly available to plants. Reports indicate that various species of the genus Enterobacter, Pseudomonas, Bacillus, etc. could solubilize Zn and making it available to plants by lowering the pH due to the production of organic acids (Abaid-Ullah et al. Citation2015; Upadhayay et al. Citation2022). Our results are also consistent with the above research and observed that Bacillus, Priestia, Stenotrophomonas, Enterobacter, and Acinetobacter successfully solubilized the zinc oxide.
Iron is also an important micronutrient and is primarily present in unavailable form for plants and microbes. Apart from two strategies followed by plants using reductases and/or phyto siderophores, microbes are reported to play a third strategy for improving the availability of Fe by producing (Abiraami et al. Citation2021). In this study 42.28% of isolates produced siderophores, predominant species of Bacillus, Paenibacillus, Streptomyces, Mixta, Stenotrophomonas, Enterobacter, Acenitobacter, Klebsiella, Pseudomonas, and Pantoea. These results agree with previous studies on Pseudomonas, Enterobacter, Bacillus, and Pantoea to produce iron-chelating siderophores of different chemotypes such as catecholate, carboxylate, and hydroxymate (Duijff et al. Citation1993; Ahmed and Holmström Citation2014; Abiraami et al. Citation2021).
Plant growth hormone indole acetic acid (IAA) stimulates the root system by cell elongation, division, and differentiation and also promotes microbial association (Duca et al. Citation2014; Gilbert et al. Citation2018). Microbes in the rhizosphere produce IAA and benefit plant growth. Several bacteria belonging to genera Bacillus, Micrococcus, Streptomyces, Pseudomonas, Klebsiella, Pantoea, Enterobacter, Acinetobacter, and Paenibacillus have been reported (Lal and Tabacchioni Citation2009; Maheshwari Citation2011; Xu et al. Citation2014; Shahzad et al. Citation2017). In this study, 60.16% of seed endophytic bacteria produced IAA, of which 14 out of 21 Streptomyces isolates displayed maximum IAA production followed by Bacillus (n = 13), Stenotrophomonas, and Paenibacillus (n = 9), Enterobacter and Priestia (n = 5), Mixta (n = 3), Micrococcus (n = 2), Nocardiopsis, Acinetobacter, Pseudomonas Cytobacillus, and Brevibacillus (n = 1). The results displayed divergences sharply with seeds of wild rice in which 45 out of 47 isolates were IAA producers (Zhang et al. Citation2021b).
Extracellular enzymes, including amylase, protease, cellulase, xylanase, pectinase, and lipase, assist bacterial interaction, colonization, seedling growth, and disease defense in host plants (Hardoim et al. Citation2008; Sai Prasad et al. Citation2020). Flemming and Wingender (Citation2010) displayed that the bacterial extracellular cellulases and proteases play a role in biofilm formation rich in polysaccharides and peptides, which allows the bacterial community to interact with the host plant. Additionally, it was noted that cellulase and pectinase help bacteria to colonize plant roots by degrading root cell walls (Truyens et al. Citation2015). Studies also point to the importance of Bacillus proteases in the cuticle degradation of nematodes (Lian et al. Citation2007). Palaniyandi et al. (Citation2013) displayed the role of bacterial enzymes in the degradation of the cell walls of plant pathogenic fungi and bacteria. In this study, it was observed that different extracellular enzymes were produced by bacterial isolates, among which Bacillus produced major hydrolytic enzymes, followed by Streptomyces and Stenotrophomonas.
Endophytic bacteria play a role in the inhibition of the fungal pathogens. Various researchers used Bacillus and Pseudomonas as biocontrol against different fungal pathogens (Shi et al. Citation2014; Palazzini et al. Citation2016; Chen et al. Citation2018). Whitaker and Bakker (Citation2019) used bacterial endophytes Bacillus Paenibacillus, Terribacillus, Pseudomonas, Xanthomonas, Pantoea, Acinetobacter, Stenotrophomonas, Kosakonia, and Serratia to inhibit the F.graminearum in wheat. Our study is also consistent with the previous studies, Bacillus, Streptomyces, Stenotrophomas, Enterobacter, Nocardiopsis, and Paenibacillus displayed antifungal activity against all selected fungal pathogens. In this study, bacterial isolates obtained different agro-ecologies showing variable functional properties. For instance, maximum growth-promoting traits were displayed by the isolates recovered from NWPZ. While in the case of antifungal traits, isolates of CZ produced more xylanase enzyme. Similarly, antifungal activity against B. sorokiniana was displayed by the isolates of CZ (). IThe isolates showing beneficial traits can be used alone or clubbed to make a consortium for the improvement of seed germination, growth, and development of the plant.
Conclusion
The study has explored the culture-dependent seed-associated endophytic bacteria of varied wheat genotypes cultivated in different agro-ecological regions. Crop seeds undergo several stages of harvesting, transportation, and storage facing different environmental conditions before going to initiate the next cycle of plant growth. Seed-vectored endophytic microbes get activated on germination and develop as seedling nascent microbiome and some exude out in the rhizosphere also. Overall this study has indicated a high and diverse bacterial endophytes in wheat seeds from different agro-ecologies. We demonstrated for the first time that twenty-three bacteria genera could be classified as obligatory bacteria that inhabit the seeds of the different Triticum species. Bacterial abundance was higher in northern western plain zone seed samples. It is revealed that irrespective of wheat seeds from T. aestivum, T. durum or T. dicoccum, the core bacterial community associated with the seeds, shows the genus Bacillus as dominant and abundant, followed by other genera Paenibacillus, Stenotrophomonas, and Streptomyces. But at the species level, only two bacterial isolates, i.e. Stenotrophomonas maltophilia and Bacillus aerius could be defined as putative core seed bacterial endophytes. Defining the specificity of endophytes in different plant host species needs further exploration. These bacterial genera showed potential plant growth-promoting activities for nutrient acquisition, plant growth promotion, and protection from fungal pathogens, thus benefitting the host plant in general fitness and survival of the seedlings. Seed endophytes have been preferentially stored by the plant, hence potential endophytes with multiple traits for plant growth promotion and variable growth characteristics may be used for developing novel formulations as bio-inoculants for sustainable agriculture.
Supplemental Material
Download MS Word (4.8 MB)Acknowledgements
Division of Microbiology and ICAR-IARI centers are duly acknowledged for providing necessary facilities and wheat seed material.
Disclosure statement
No potential conflict of interest was reported by the author(s).
Additional information
Funding
Notes on contributors
Pushpendra Sharma
Dr Pushpendra Sharma, Senior Research Fellow.
Archna Suman
Dr Archna Suman, Principal Scientist.
Krishnan Aswini
Ms Krishnan Aswini, PhD scholar.
Jogdande SaiPrasad
Jogdande Sai Prasad, PhD Scholar.
Shrikant Gond
Mr Shrikant Gond, Technical Assistant, Division of Microbiology, ICAR-Indian Agricultural Research Institute (ICAR-IARI), New Delhi, India, 110012.
References
- Abaid-Ullah M, Nadeem M, Hassan M, Ganter J, Muhammad B, Nawaz K, Hafeez FY. 2015. Plant growth promoting rhizobacteria: an alternate way to improve yield and quality of wheat (Triticum aestivum). Int J Agri Biol. 17(1):51–60.
- Abiraami TV, Suman A, Singh B, Aswini K, Annapurna K. 2021. Radiochemical evidence for the contribution of chemo typed siderophore producing bacteria towards plant iron nutrition. Curr Microbiol. 78(12):4072–4083. doi:10.1007/s00284-021-02658-8
- Ahmed E, Holmström SJ. 2014. Siderophores in environmental research: roles and applications. Microb Biotechnol. 7(3):196–208. doi:10.1111/1751-7915.12117
- An S-Q, Berg G. 2018. Stenotrophomonas maltophilia. Trends Microbiol. 26(7):637–638. doi:10.1016/j.tim.2018.04.006
- Aswini K, Suman A, Sharma P, Singh PK, Gond S, Pathak D. 2023. Seed endophytic bacterial profiling from wheat varieties of contrasting heat sensitivity. Front Plant Sci. 14:1101818. doi:10.3389/fpls.2023.1101818
- Barret M, Briand M, Bonneau S, Préveaux A, Valière S, Bouchez O, Jacques MA. 2015. Emergence shapes the structure of the seed microbiota. Appl Environ Microbiol. 81(4):1257–1266. doi:10.1128/AEM.03722-14
- Bodhankar S, Grover M, Hemanth S, Reddy G, Rasul S, Yadav SK, Srinivasarao C. 2017. Maize seed endophytic bacteria: dominance of antagonistic, lytic enzyme-producing Bacillus spp. 3 Biotech. 7(4):1–13. doi:10.1007/s13205-017-0860-0
- Borriss R. 2011. Use of plant-associated Bacillus strains as biofertilizers and biocontrol agents in agriculture. In: DK Maheshwari, editor. Bacteria in agrobiology: plant growth responses. Berlin: Springer; p. 41–76.
- Bright M, Bulgheresi S. 2010. A complex journey: transmission of microbial symbionts. Nat Rev Microbiol. 8(3):218–230. doi:10.1038/nrmicro2262
- Bulgarelli D, Garrido-Oter R, Münch PC, Weiman A, Dröge J, Pan Y, Schulze-Lefert P. 2015. Structure and function of the bacterial root microbiota in wild and domesticated barley. Cell Host Microbe. 17(3):392–403. doi:10.1016/j.chom.2015.01.011
- Chee-Sanford JC, Williams MM, Davis AS, Sims GK. 2006. Do microorganisms influence seed-bank dynamics? Weed Sci. 54(3):575–587. doi:10.1614/WS-05-055R.1
- Chen L, Heng J, Qin S, Bian K. 2018. A comprehensive understanding of the biocontrol potential of Bacillus velezensis LM2303 against fusarium head blight. PLoS One. 13(6):e0198560. doi:10.1371/journal.pone.0198560
- Compant S, Mitter B, Colli-Mull JG, Gangl H, Sessitsch A. 2011. Endophytes of grapevine flowers, berries, and seeds: identification of cultivable bacteria, comparison with other plant parts, and visualization of niches of colonization. Microb Ecol. 62(1):188–197. doi:10.1007/s00248-011-9883-y
- Devi R, Kaur T, Kour D, Yadav AN, Suman A. 2022. Potential applications of mineral solubilizing rhizospheric and nitrogen fixing endophytic bacteria as microbial consortium for the growth promotion of chilli (Capsicum annum L.). Biologia. 77:1–11. doi:10.1007/s11756-022-01127-2.
- Duca D, Lorv J, Patten CL, Rose D, Glick BR. 2014. Indole-3-acetic acid in plant–microbe interactions. Antonie Van Leeuwenhoek. 106(1):85–125. doi:10.1007/s10482-013-0095-y
- Duijff BJ, Meijer JW, Bakker PA, Schippers B. 1993. Siderophore-mediated competition for iron and induced resistance in the suppression of Fusarium wilt of carnation by fluorescent Pseudomonas spp. Neth J Plant Path. 99(5):277–289. doi:10.1007/BF01974309
- Felsenstein J. 1985. Phylogenies and the comparative method. Am Nat. 125(1):1–15. doi:10.1086/284325
- Ferreira A, Quecine MC, Lacava PT, Oda S, Azevedo JL, Araújo WL. 2008. Diversity of endophytic bacteria from Eucalyptus species seeds and colonization of seedlings by Pantoeaagglomerans. FEMS Microbiol Lett. 287(1):8–14. doi:10.1111/j.1574-6968.2008.01258.x
- Flemming HC, Wingender J. 2010. The biofilm matrix. Nat Rev Microbiol. 8(9):623–633. doi:10.1038/nrmicro2415
- Gardener BBM. 2004. Ecology of Bacillus and Paenibacillus spp. in Agricultural Systems. Phytopathology. 94(11):1252–1258. doi:10.1094/PHYTO.2004.94.11.1252
- Gilbert S, Xu J, Acosta K, Poulev A, Lebeis S, Lam E. 2018. Bacterial production of indole related compounds reveals their role in association between duckweeds and endophytes. Front Chem. 6:265. doi:10.3389/fchem.2018.00265
- Graziano S, Caldara M, Gullì M, Bevivino A, Maestri E, Marmiroli N. 2022. A metagenomic and gene expression analysis in wheat (T. durum) and maize (Z. mays) biofertilized with PGPM and biochar. Int J Mol Sci. 23(18):10376. doi:10.3390/ijms231810376
- Gupta A, Singh C, Kumar V, Tyagi B, Tiwari V, Chatrath R, Singh G. 2018. Wheat varieties notified in India since 1965. ICAR-Indian Institute of Wheat & Barley Research, Karnal, 101.
- Hacquard S. 2016. Disentangling the factors shaping microbiota composition across the plant holobiont. New Phytol. 209(2):454–457. doi:10.1111/nph.13760
- Hafeez BMKY, Khanif YM, Saleem M. 2013. Role of zinc in plant nutrition – a review. Am J Exp Agri. 3(2):374. doi:10.9734/AJEA/2013/2746
- Hammer Ø, Harper DA, Ryan PD. 2001. PAST: paleontological statistics software package for education and data analysis. Palaeontologia Electronica. 4(1):9.
- Hardoim PR, van Overbeek LS, van Elsas JD. 2008. Properties of bacterial endophytes and their proposed role in plant growth. Trends Microbiol. 16(10):463–471. doi:10.1016/j.tim.2008.07.008
- Herrera SD, Grossi C, Zawoznik M, Groppa MD. 2016. Wheat seeds harbour bacterial endophytes with potential as plant growth promoters and biocontrol agents of Fusarium graminearum. Microbiol Res. 186:37–43. doi:10.1016/j.micres.2016.03.002
- Johnston-Monje D, Raizada MN. 2011. Conservation and diversity of seed associated endophytes in Zea across boundaries of evolution, ethnography and ecology. Plos one. 6(6):e20396. doi:10.1371/journal.pone.0020396
- Khalaf EM, Raizada MN. 2016. Taxonomic and functional diversity of cultured seed associated microbes of the cucurbit family. BMC Microbiol. 16(1):1–16. doi:10.1186/s12866-015-0617-z
- Kimura M. 1980. A simple method for estimating evolutionary rates of base substitutions through comparative studies of nucleotide sequences. J Mol Evol. 16(2):111–120. doi:10.1007/BF01731581
- Kuźniar A, Włodarczyk K, Grządziel J, Goraj W, Gałązka A, Wolińska A. 2020. Culture-independent analysis of an endophytic core microbiome in two species of wheat: Triticum aestivum L.(cv.‘Hondia’) and the first report of microbiota in Triticum spelta L.(cv.‘Rokosz’). Syst Appl Microbiol. 43(1):126025. doi:10.1016/j.syapm.2019.126025
- Lal S, Tabacchioni S. 2009. Ecology and biotechnological potential of paenibacilluspolymyxa: a minireview. Indian J Microbiol. 49(1):2–10. doi:10.1007/s12088-009-0008-y
- Latt ZW, Yu SS, Kyaw EP, Lynn TM, New MT, Mon WW, Aye KN. 2018. Using cellulolytic nitrogen fixing bacterium, azomonasagilisfor effective degradation of agricultural residues. Open Microbiol J. 12:154–162. doi:10.2174/1874285801812010154
- Lex A, Gehlenborg N, Strobelt H, Vuillemot R, Pfister H. 2014. Upset: visualization of intersecting sets. IEEE Trans Vis Comput Graph (InfoVis). 20(12):1983–1992. doi:10.1109/TVCG.2014.2346248
- Lian LH, Tian BY, Xiong R, Zhu MZ, Xu J, Zhang KQ. 2007. Proteases from Bacillus: a new insight into the mechanism of action for rhizobacterial suppression of nematode populations. Lett Appl Microbiol. 45(3):262–269. doi:10.1111/j.1472-765X.2007.02184.x
- Liu J, Nagabhyru P, Schardl CL. 2017. Epichloëfestucae endophytic growth in florets, seeds, and seedlings of perennial ryegrass (Lolium perenne). Mycologia. 109(5):691–700. doi:10.1080/00275514.2017.1400305
- Liu Y, Zuo S, Zou Y, Wang J, Song W. 2013. Investigation on diversity and population succession dynamics of endophytic bacteria from seeds of maize (Zea mays L., Nongda108) at different growth stages. Ann Microbiol. 63(1):71–79. doi:10.1007/s13213-012-0446-3
- Lugtenberg BJJ, Chin-A-Woeng TFC, Bloemberg GV. 2002. Microbe-plant interactions: principles and mechanisms. Antonie Van Leeuwenhoek. 81:373–383. doi:10.1023/A:1020596903142
- Maheshwari DK, editor. 2011. Bacteria in agrobiology: plant growth responses. Berlin, Heidelberg: Springer Science & Business Media.
- Mano H, Tanaka F, Watanabe A, Kaga H, Okunishi S, Morisaki H. 2006. Culturable surface and endophytic bacterial flora of the maturing seeds of rice plants (Oryza sativa) cultivated in a paddy field. Microb Environ. 21(2):86–100. doi:10.1264/jsme2.21.86
- Marag PS, Suman A. 2018. Growth stage and tissue specific colonization of endophytic bacteria having plant growth promoting traits in hybrid and composite maize (Zea mays L.). Microbiol Res. 214:101–113. doi:10.1016/j.micres.2018.05.016
- Muresu R, Porceddu A, Concheri G, Stevanato P, Squartini A. 2022. Legumes of the Sardinia Island: knowledge on symbiotic and endophytic bacteria and interactive software tool for plant species determination. Plants. 11(11):1521. doi:10.3390/plants11111521
- Nelson EB, Simoneau P, Barret M, Mitter B, Compant S. 2018. Editorial special issue: the soil, the seed, the microbes and the plant. Plant Soil. 422(1):1–5. doi:10.1007/s11104-018-3576-y
- Palaniyandi SA, Yang SH, Zhang L, Suh JW. 2013. Effects of actinobacteria on plant disease suppression and growth promotion. Appl Microbiol Biotechnol. 97(22):9621–9636. doi:10.1007/s00253-013-5206-1
- Palazzini JM, Dunlap CA, Bowman MJ, Chulze SN. 2016. Bacillus velezensis RC 218 as a biocontrol agent to reduce Fusarium head blight and deoxynivalenol accumulation: genome sequencing and secondary metabolite cluster profiles. Microbiol Res. 192:30–36. doi:10.1016/j.micres.2016.06.002
- Patten CL, Glick R. 2022. Role of Pseudomonas putida indole acetic acid in development of the host plant root system. Appl Environ Microbiol. 68(8):3795–3801. doi:10.1128/AEM.68.8.3795-3801.2002
- Rana KL, Kour D, Kaur T, Devi R, Yadav AN, Yadav N, Saxena AK. 2020. Endophytic microbes: biodiversity, plant growth-promoting mechanisms and potential applications for agricultural sustainability. Antonie Van Leeuwenhoek. 113(8):1075–1107. doi:10.1007/s10482-020-01429-y
- Reinhold-Hurek B, Hurek T. 2011. Living inside plants: bacterial endophytes. Curr Opin Plant Biol. 14(4):435–443. doi:10.1016/j.pbi.2011.04.004
- Rijavec T, Lapanje A, Dermastia M, Rupnik M. 2007. Isolation of bacterial endophytes from germinated maize kernels. Can J Microbiol. 53(6):802–808. doi:10.1139/W07-048
- Robinson RJ, Fraaije BA, Clark IM, Jackson RW, Hirsch PR, Mauchline TH. 2016. Wheat seed embryo excision enables the creation of axenic seedlings and Koch’s postulates testing of putative bacterial endophytes. Sci Rep. 6(1):1–9. doi:10.1038/s41598-016-0001-8
- Sahu KP, Kumar A, Sakthivel K, Reddy B, Kumar M, Patel A, Sheoran N, Gopalakrishnan S, Prakash G, Rathour R, Gautam RK. 2022. Deciphering core phyllomicrobiome assemblage on rice genotypes grown in contrasting agroclimatic zones: implications for phyllomicrobiome engineering against blast disease. Environ Microbiome. 17(1):28. doi:10.1186/s40793-022-00421-5
- Sai Prasad J, Aswini K, Sharma P, Gond S, Suman A. 2020. A novel wheat matrix medium (WMM) for rapid isolation of hydrolytic enzyme producing bacterial seed endophytes. Int J CurrMicrobiol Appl Sci. 9(12):2181–2197. doi:10.20546/ijcmas.2020.912.258
- Schwyn B, Neilands JB. 1987. Universal chemical assay for the detection and determination of siderophores. Anal Biochem. 160(1):47–56. doi:10.1016/0003-2697(87)90612-9
- Semwal P, Rawat R, Sharma P, Baunthiyal M. 2018. Actinobacteria from cow feces: isolation, identification and screening for industrially important secondary metabolites. Microbiol Biotechnol Lett. 46(1):68–76. doi:10.4014/mbl.1709.09004
- Shade A, Jacques MA, Barret M. 2017. Ecological patterns of seed microbiome diversity, transmission, and assembly. Curr Opin Microbiol. 37:15–22. doi:10.1016/j.mib.2017.03.010
- Shahzad R, Khan AL, Bilal S, Asaf S, Lee IJ. 2018. What is there in seeds? Vertically transmitted endophytic resources for sustainable improvement in plant growth. Front Plant Sci. 9:24. doi:10.3389/fpls.2018.00024
- Shahzad R, Waqas M, Khan AL, Al-Hosni K, Kang SM, Seo CW, Lee IJ. 2017. Indole acetic acid production and plant growth promoting potential of bacterial endophytes isolated from rice (Oryza sativa L.) seeds. Acta BiologicaHungarica. 68(2):175–186. doi:10.3389/fpls.2018.00024
- Sharma P, Aswini K, Sai Prasad J, Kumar N, Pathak D, Gond S, Suman A. 2022. Characterization of actinobacteria from wheat seeds for plant growth promoting traits and protection against fungal pathogens. J Basic Microbiol. 62:1–15. doi:10.1002/jobm.202270001
- Sharma P, Kumar V, Naik B, Bisht GRS. 2013. Screening of xylanase activity of Streptomyces albidoflavousPSM-3n isolated from Uttarakhand. EurAsian J Biosci. 7(4):30–40. doi:10.5053/ejobios.2013.7.0.4
- Shi C, Yan P, Li J, Wu H, Li Q, Guan S. 2014. Biocontrol of Fusarium graminearum growth and deoxynivalenol production in wheat kernels with bacterial antagonists. Int J Environ Res Public Health. 11(1):1094–1105. doi:10.3390/ijerph110101094
- Suman A, Govindasamy V, Ramakrishnan B, Aswini K, SaiPrasad J, Sharma P, Pathak D, Annapurna K. 2021. Microbial community and function-based synthetic bioinoculants: a perspective for sustainable agriculture. Front Microbiol. 12:1–19. doi:10.3389/fmicb.2021.644708
- Suman A, Yadav AN, Verma P. 2016. Endophytic microbes in crops: diversity and beneficial impact for sustainable agriculture. In: D Singh, H Singh, R Prabha, editors. Microbial inoculants in sustainable agricultural productivity. New Delhi: Springer; p. 117–143. doi:10.1007/978-81-322-2647-5_7
- Tancos MA, Chalupowicz L, Barash I, Manulis-Sasson S, Smart CD. 2013. Tomato fruit and seed colonization by Clavibactermichiganensis subsp. michiganensis through external and internal routes. Appl Environ Microbiol. 79(22):6948–6957. doi:10.1128/AEM.02495-13
- Truyens S, Jambon I, Croes S, Janssen J, Weyens N, Mench M, Vangronsveld J. 2014. The effect of long-term Cd and Ni exposure on seed endophytes of Agrostis capillaris and their potential application in phytoremediation of metal-contaminated soils. Int J Phytoremediation. 16(7-8):643–659. doi:10.1080/15226514.2013.837027
- Truyens S, Weyens N, Cuypers A, Vangronsveld J. 2015. Bacterial seed endophytes: genera, vertical transmission and interaction with plants. Environ Microbiol Rep. 7(1):40–50. doi:10.1111/1758-2229.12181
- Turner TR, James EK, Poole PS. 2013. The plant microbiome. Genome Biol. 14(6):1–10. doi:10.1186/gb-2013-14-6-209
- Upadhayay VK, Singh AV, Khan A, Sharma A. 2022. Contemplating the role of zinc-solubilizing bacteria in crop biofortification: an approach for sustainable bioeconomy. Frontiers in Agronomy. 72:1–13. doi:10.3389/fagro.2022.903321
- Verma SK, Kingsley K, Irizarry I, Bergen M, Kharwar RN, White Jr JF. 2017. Seed-vectored endophytic bacteria modulate development of rice seedlings. J Appl Microbiol. 122(6):1680–1691. doi:10.1111/jam.13463.
- Weyens N, van der Lelie D, Taghavi S, Vangronsveld J. 2009. Phytoremediation: plant–endophyte partnerships take the challenge. Curr Opin Biotechnol. 20(2):248–254. doi:10.1016/j.copbio.2009.02.012.
- Whitaker BK, Bakker MG. 2019. Bacterial endophyte antagonism toward a fungal pathogen in vitro does not predict protection in live plant tissue. FEMS Microbiol Ecol. 95(2):fiy237. doi:10.1093/femsec/fiy237.
- Xu M, Sheng J, Chen L, Men Y, Gan L, Guo S, Shen L. 2014. Bacterial community compositions of tomato (Lycopersicum esculentum Mill.) seeds and plant growth promoting activity of ACC deaminase producing Bacillus subtilis (HYT-12-1) on tomato seedlings. World J Microbiol Biotechnol. 30(3):835–845. doi:10.1007/s11274-013-1486-y.
- Zhang HW, Song YC, Tan RX. 2006. Biology and chemistry of endophytes. Nat Prod Rep. 23(5):753–771. doi:10.1039/b609472b.
- Zhang X, Zhang Y, Xu C, Liu K, Bi H, Ai X. 2021b. H2o2 functions as a downstream signal of IAA to mediate H2S-induced chilling tolerance in cucumber. Int J Mol Sci. 22(23):12910. doi:10.3390/ijms222312910.
- Zhang Z, Liu T, Zhang X, Xie J, Wang Y, Yan R, Zhu D. 2021a. Cultivable endophytic bacteria in seeds of Dongxiang wild rice and their role in plant-growth promotion. Diversity (Basel). 13(12):665. doi:10.3390/d13120665.
- Zilber-Rosenberg I, Rosenberg E. 2008. Role of microorganisms in the evolution of animals and plants: the hologenome theory of evolution. FEMS Microbiol Rev. 32(5):723–735. doi:10.1111/j.1574-6976.2008.00123.x.