Abstract
Green synthesis of nanoparticles is regarded as a safe and non-toxic process whereas conventional synthesis using chemical methods produces toxic substance. This study provides a novel insight for enzymatic synthesis method of silver nanoparticles using purple acid phosphatase, isolated from Limonia acidissima (wood apple) as a new source and used in the synthesis of silver nanoparticles. Stable silver nanoparticles were produced by sonochemical method using apoenzyme as a stabilising and capping agent and were characterised by various physicochemical techniques like UV–Visible spectroscopy, Fourier-transform infrared, X-ray diffraction and transmission electron microscopy. X-ray study shows that nanoparticles are composed of silver and silver oxide. The synthesised nanoparticles exhibited excellent antimicrobial activity against Escherichia coli, Pseudomonas aeruginosa and Staphylococcus aureus.
1. Introduction
In the recent years nanotechnology has gained prime importance in many technological innovations.[Citation1] Nanostructured materials are technically significant objects that possess optical and electrical properties that mainly depend upon the size and shape of nanoparticles. They also exhibit many other applications such as nanosensors, catalyst, in semiconductors and energy devices and potent applications in the medicinal field. Metals have been used for centuries as a bactericidal agents especially silver, gold, copper zinc and titanium have attracted particular attention, each having various properties and spectra of activity.[Citation2] Silver nanoparticles (AgNPs) owing to their well proven antimicrobial activities have vast applicability in the field of medicine, biology and cosmetics along with their usage in biosensing, engineering, electronics and textile industry.[Citation3–5] To expand the applicability of AgNPs, it is crucial to develop cost effective, eco-friendly, simple and consistent methods for fabrication of AgNPs. Several physical and chemical methods have been reported for the synthesis of AgNPs [Citation6,Citation7] but most of these methods suffered from environmental and biological hazards associated with them.[Citation8]
Considering the wide range of applications of AgNPs it is necessary to find new sources to produce stable AgNPs and the quest to overcome unwanted side effects associated with chemical synthesis procedures leads to a new environmental friendly approach called ‘green synthesis’ where microbes or plant extracts are utilised for synthesis purpose. This greener approach also provides advancements over chemical methods as (1) it is cost effective, and can be easily scaled up for large-scale synthesis, (2) fabrication procedure is simple and eco-friendly due to utilisation of non-toxic chemicals and solvents and (3) easy bioavailability of starting materials as a reducing and capping agents (plants, microbes, etc.). Earlier researchers have reported the bio reduction methods of synthesis using microorganisms, fungus and enzymes.[Citation9–12] Similar to microbes-assisted method, several rapid plant mediated synthesis using crude plant extracts like Triticum aestivum,[Citation13] Sorbus aucuparia [Citation14] and Chenopodium album [Citation15] have also been reported. The synthesis of nanoparticles by using plant extracts are more advantageous over other biological processes because it eliminates the elaborate process of maintaining cell cultures and can be suitably scaled up for large-scale production under no aseptic environments.[Citation16]
In this study, we have proposed green synthesis of AgNPs using purple acid phosphatase (PAP) as a reducing and stabilising agent. PAPs are involved in a multitude of biological functions [Citation17] while Limonia acidissima (LA) has got high medicinal value with each part of the fruit possess medicinal property. LA is a deciduous, slow-growing, erect tree belonging to monotypic genus Limonia and is native to India, Pakistan, Sri Lanka and Southeast Asia east to Java. It possesses a wide range of biological activities like antidiabetic, hepatoprotective activity and antifungal activities [Citation18–20] and is commonly known as wood apple or elephant apple.
Literature survey shows that multiple PAP-like isoforms have been identified in the genomes of sweet potato [Citation21,Citation22] tomato [Citation23] and potato [Citation24], but to the best of our knowledge this is the first report on the application of LA as a novel source for PAP isolation and a simple green methodology to synthesise stable AgNPs efficiently at room temperature in a single step.
2. Experimental details
2.1. Materials
PAP was extracted and isolated from LA (wood apple). The samples were chosen from local region of Pune, Maharashtra. Silver nitrate (AgNO3) was purchased from Merck, Germany. HCl, KOH, ammonium sulphate and dialysis bag (Dialysis membrane-150, diameter – 25.4 mm, flat width – 42.44 mm) were purchased from Himedia.
2.2. Isolation and identification of purple acid phosphatase
Sample were collected and processed by random sampling method in and around Pune city. The PAP isolation and purification was performed by modified Averill process.[Citation25] Fruit pulp of LA was used for extraction and isolation of enzyme. Briefly fruit pulp was soaked in 0.145 M NaCl saline solution and then crushed and homogenised in a saline solution before keeping it at 4 °C for 24 hrs. The resultant solution was centrifuged for 10 min at 2800 rpm and supernatant was chosen as the crude extract. Extract is then subjected to 45% and 85% ammonium sulphate fractionation for the isolation of enzyme. A summery of PAP purification is given in .
The protein estimation was done using Lowry's method using bovine serum albumin (BSA) as standard [Citation26] while carbohydrate content was determined via phenol sulphuric acid method.[Citation27] PAP activity was determined by measuring the released p-nitro phenol by the enzyme from p-nitro phenyl phosphate as a substrate at 405 nm. The molar extinction coefficient of p-nitro phenol is 1.77 × 104 cm−1 and specific activity is expressed in µg/mg of protein.
After the step of 85% ammonium sulphate fractionation followed by acid treatment for pH 4 and pH 6 supernatant was dialysed against phosphate buffer pH 7.9. After this step gel filtration was carried out on Sephadex G-100 and activity rich fractions were pulled and applied on Con A seralose (affinity chromatography column) equilibrated with saline and recycled three times. The column was washed with saline to remove unbound protein and high protein and high activity fractions were dialysed against saline to remove α-methyl–D-glucopyranoside. These fractions were applied on Sephadex G-100 column again. Fractions of high protein and high activity of PAP from this step were lyophilised and stored at −20 °C till further characterisation.
Optimisation of pH was done using acetate, citrate and phosphate buffer range. Optimisation of temperature was done using temperature range 0–100 °C with a constant substrate concentration of 2 mM. Adventitious metal ions were removed from buffer by passage through a chelex 100 column. Metal estimation of PAP was done using graphite furnace equipped with atomic absorption spectroscopy (GF-AAS).
Detailed purification procedure is described in electronic supplemental data.
2.3. Apoenzyme preparation and synthesis of silver nanoparticles
Purified fraction of PAP enzyme was brought to dialysis against 1 mole acetic acid for three changes and then dialysed against 0.01 mole ethylenediaminetetraacetic acid (EDTA) for three changes. After the apoenzyme preparation it was brought to check regular activity and metal concentration. This fraction was used for the synthesis of Ag nanoparticles. Ten ml of PAP enzyme was added to 200 ml 1mM AgNO3 in the ratio of 1:4 followed by 10 min sonication. Immediately colour of the solution changes to brown as indicated in . The formation of AgNPs was monitored via UV–Visible (UV–Vis) spectroscopic method.
2.4. Characterisation of AgNPs
Characterisation of AgNPs was done using UV–Vis, Fourier-transform infrared (FTIR) and X-ray diffraction (XRD) studies which confirms the formation of nanoparticles. The crystallinity and phases of the AgNPs were characterised by X-ray diffractometer (XRD-6000, Shimadzu, Japan) with Cukα radiation (λ = 1.5412 Å) in the range 10°–80° with 2°/min scanning rate. The further composition of AgNPs was characterised by FTIR (FTIR, Perkin Elmer, Spectrum BX) spectroscopy in the range 4000–400 cm−1. In addition, the optical property of prepared AgNPs was analysed via UV–Vis (UV–Vis, Perkin Elmer, Lambda 35) absorption double beam spectrophotometer with a deuterium and tungsten iodine lamp in the range from 200 to 1100 nm at room temperature. The transmission electron microscopy (TEM) micrographs were taken for morphological analysis of nanoparticles with a JEOL 3010 field emission electron microscope with an accelerating voltage of 300 kV. The samples were analysed by preparing a dilute solution made in distilled water and drop casted on a carbon coated copper grid, followed by drying the sample at ambient conditions before it was attached to the sample holder on the microscope.
3. Results and discussion
3.1. Isolation of PAP and synthesis of AgNPs
PAP belongs to the family of dinuclear metallozymes [Citation28] and can be differentiated with other acid phosphatases by its purple colour due to Tyr to Iron (III) charge transition.[Citation29] As name suggests PAP catalyses hydrolysis of phosphate esters but apart from this few other functions have also been suggested by many researchers. Tobacco plant PAP is involved in β-glucan deposition while tomato PAP may be involved in phosphate release from extracellular phosphate ester in the condition of phosphate starvation.[Citation30] As reported, it possibly plays a role in reactive oxygen metabolism, iron transport and bone resorption.[Citation31–33] Here, we have explored the catalytic activities of PAP for the synthesis of AgNPs and isolated the enzyme from a novel source LA (wood apple). Especially modification was done by using Con A seralose affinity chromatography and gel filtration column through Sephadex G-100 column. Compared to Averill's method, we have replaced diethylaminoethanol (DEAE) anion exchanger column separation step by gel filtration on Sephadex G-100 and in between gel filtration we have worked out with affinity chromatography for better separation and concentration of glycoprotein PAP. These changes resulted in increase of protein concentration in purified enzyme.
Molecular mass of the purified enzyme was done by gel filtration and calibration curve using standard proteins and the obtained molecular weight is 110 kD. Effect of temperature and pH studies of purified PAP fraction was carried with p-nitro phenyl phosphate as substrate and it indicates 5.6 as optimum pH and the enzyme was active at 65 °C with 82% activity when incubated for 10 min.
Metal estimation indicates that the presence of catalytic di-iron site (1.47 ± 0.2 mole) together with co-catalytic site manganese and zinc (0.60 ± 0.3 mole) has been determined by GF-AAS technique. According to Averill metal constellation of Zn/Mn may be assigned as impurity or co-catalytic site and it may be due to competition of Fe+2 site with divalent metal ions in constellation but Fe+3 tyrosine must remain intact at active site as a chromophoric iron.
Biological activity for phosphor ester hydrolysis was studied with p-nitro phenyl phosphate as a substrate. Apoenzyme of PAP from LA was prepared by dialysing against EDTA and acetic acid and zero activity was done by ‘Burst experiment’ and zero metal ion concentration was confirmed by using GF-AAS technique.
AgNPs were prepared via one-step bioreduction of AgNO3 in PAP extract and as synthesised AgNPs were purified by centrifugation at 13000 rpm for 20 min to remove the impurities, unreacted salt and PAP extract. The purified nanoparticles were dispersed in distilled water and further evaluated for their physicochemical characteristics.
3.2. Characterisation of AgNPs
TEM has been employed to characterise the size, shape and morphology of synthesised AgNPs. The TEM image of AgNPs shows the polydispersed nature of particles which is shown in . From the image, it is evident that the morphology of AgNPs is spherical which is in agreement with the shape of surface plasmon resonance (SPR) band in the UV–Vis spectrum. The minimum particles size measured from the TEM image exhibited size of 25–45 nm which is in good agreement with the particle size calculated from the XRD analysis.
shows the UV–Vis absorption spectrum of the synthesised Ag nanoparticles. AgNPs have free electrons, which give SPR absorption band due to the combined vibration of electrons of AgNPs as well as silver oxide impurity in resonance with light wave. A broad absorption peak was observed at 445 nm, with one more absorption at ∼250 nm which indicates the formation of silver oxide.
Figure 3. UV–Visible spectra of silver/silver oxide nanoparticles. All the experiments were performed at room temperature (∼25 °C).
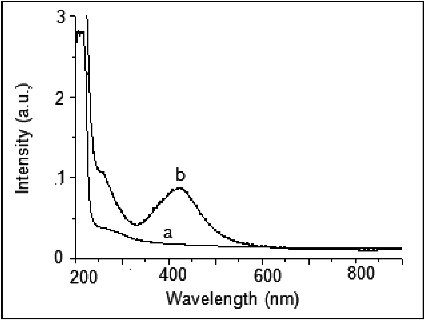
FTIR measurement was carried out to identify the possible biomolecules responsible for capping and reducing agent for the AgNPs synthesised by extract.
Two obvious infrared bands are observed at 3271 and 1637 cm−1. The intense broad band at 3271 cm−1 is due to N—H and O—H stretching mode in the linkage of the proteins. The medium intense band at 1637 cm−1 arises from the C˭O stretching mode in amine group which is commonly found in the protein, indicating the presence of proteins as capping agent for AgNPs which increases the stability of the nanoparticles synthesised. On the other hand, the intense and broad peak is expected 386 cm−1 corresponds to the Ag metal (). The crystallinity and phases of the AgNPs were characterised by X-ray diffractometer (XRD-6000, Shimadzu, Japan) with Cukα radiation (λ = 1.5412 Å) in the range of 10°–80° with 2°/min scanning rate. XRD pattern for synthesised AgNPs shows () four main characteristic diffraction peaks indicating face-centererd cubic Ag crystals (JCPDS no. 00-004-0783). Peaks from any other phase were observed showing that single phase Ag with cubic structure nanoparticles. Also, peaks are observed at 2θ values (54.70° and 31.93°) may correspond to presence of silver oxide nanoparticles.
3.3. Antimicrobial activity of AgNPs
The antimicrobial activity of AgNPs from LA root extract as determined by the antimicrobial assay is given in . The particles showed activity against Pseudomonas aeruginosa (P. aeruginosa) and Staphylococcus aureus (S. aureus). Less activity was obtained against Escherichia coli. This showed that AgNPs in LA root extract show activity against both Grams positive as well as Gram negative organisms. The results are tabulated in .
Table 1. Summary of purification of PAP from 1 kg tubers namely Limonia acidissima.
Table 2. Antimicrobial activity of silver nanoparticles from enzyme against test organisms.
To determine the minimum inhibitory concentration (MIC) of AgNPs, broth dilution method according to the Clinical Laboratory Standards Institute (CLSI) guidelines was performed. The MIC obtained was 2.95 µg/ml against Gram negative bacteria P. aeruginosa and Gram positive bacteria S. aureus. As the compound was turbid, only visually inspecting the tubes for the presence of growth was not possible. Hence, growth from the tubes was streak plated on plates of sterile nutrient agar and the values indicated the minimum bactericidal concentration.
4. Conclusion
A simple, one-step green approach was developed for the synthesis of AgNPs using apoenzyme PAP from LA. Apoenzyme acts as both reducing and stabilising agent. The XRD spectrum of as synthesised sample shows the presence of AgNPs along with minute presence of AgO. The TEM analysis shows the nanostructure nature of the sample with the particle size of 25–45 nm. MIC obtained for Ag/AgO nanoparticles was 2.95 µg/ml against Gram negative bacteria P. aeruginosa and Gram positive bacteria S. aureus.
Supplemental data
Supplemental data for this article can be accessed here.
tjen_Green_synthesis_of_silver_nanoparticles_suppl.data.doc
Download MS Word (189 KB)Acknowledgement
Dr Waghmode gratefully acknowledges support of the ISRO-UoP-STC [project number PU/ISRO-STC/1354]; DST-FEST grant for Department of Chemistry, Abasaheb Garware College, Pune, Maharashtra, India. The author (Dr Preeti Nigam Joshi) would like to thank Department of Science and Technology, Government of India for providing financial support as INSPIRE faculty award.
Disclosure statement
No potential conflict of interest was reported by the authors.
Additional information
Funding
References
- Younes A, Abdeslam EB, Hafid A, Khalid B, Jamal M. Microwave-assisted approach for rapid and green synthesis of silver nanoparticles using aqueous onion (Allium cepa) and their antimicrobial activity. J Nanostruct Chem. 2013;3:84–90.
- Sreeram KJ, Nidhin M, Nair BU. Microwave assisted template synthesis of silver nanoparticles. Bull Mater Sci. 2008;31:937–942.
- Ahamed M, AlSalhi MS, Siddiqui MKJ. Silver nanoparticle applications and human health. Clin Chim Acta. 2010;411:1841–1848.
- Prucek R, Tuček J, Kilianová M, Panáček A, Kvítek L, Filip J. The targeted antibacterial and antifungal properties of magnetic nanocomposite of iron oxide and silver nanoparticles. Biomaterials. 2011;32:4704–4713.
- Samal S, Jeyaraman P, Vishwakarma V. Sonochemical coating of Ag-TiO2 nanoparticles on textile fabrics for stain repellency and self-cleaning- the Indian scenario: a review. J Minerals Mater Characterization Eng. 2010;9:519–525.
- Kim S, Kuk E, Yu K, Kim J, Park S, Lee H. Antimicrobial effects of silver nanoparticles. Nanomedicine. 2007;3:95–101.
- Tan Y, Dai Y, Li Y, Zhua D. Preparation of gold, platinum, palladium and silver nanoparticles by the reduction of their salts with a weak reductant–potassium bitartrate. J Mater Chem. 2003;13:1069–1075.
- Mukunathan K, Elumalai E, Patel T, Murty V. Catharanthus roseus: a natural source for the synthesis of silver nanoparticles. Asian Pac J Trop Biomed. 2011;1:270–274.
- Klaus T, Joerger R, Olsson E, Granqvist C. Silver-based crystalline nanoparticles, microbially fabricated. J Proc Natl Acad Sci USA. 1999;96:13611–13614.
- Konishi Y, Uruga T. Bioreductive deposition of platinum nanoparticles on the bacterium Shewanella algae. J Biotechnol. 2007;128:648–653.
- Nair B, Pradeep T. Coalescence of nanoclusters and formation of submicron crystallites assisted by lactobacillus strains. Cryst Growth Des. 2001;2:293–298.
- Willner I, Baron R, Willner B. Growing metal nanoparticles by enzymes. J Adv Mater. 2006;18:1109–1120.
- Waghmode S, Chavan P, Kalyankar V, Dagade S. Synthesis of silver nanoparticles using Triticum aestivum and its effect on peroxide catalytic activity and toxicology. J Chem. 2013;2013:5–10.
- Dubey S, Lahtinen M, Sarkka H, Sillanpaa M. Bio-prospective of Sorbus aucuparia leaf extract in development of silver and gold nanocollides. Colloids Surfaces B. 2010;80:26–33.
- Dwivedi A, Gopal K. Biosynthesis of silver and gold nanoparticles using Chenopodium album leaf extract. Colloids Surfaces A. 2010;369:27–33.
- Shankar S, Ahmad A, Sastry M. Geranium leaf assisted biosynthesis of silver nanoparticles. Biotechnol Prog. 2003;19:1627–1631.
- Klabunde T, Stahl B, Suerbaum H, Hahner S, Karas M, Hillenkamp F, Krebs B, Witze H. The amino acid sequence of the red kidney bean Fe(III)-Zn(II) purple acid phosphatase. Determination of the amino acid sequence by a combination of matrix-assisted laser desorptiodionization mass spectrometry and automated Edman sequencing. Eur J Biochem. 1994;226:369–375.
- Upadhya S, Shanbhag K, Suneetha G, Naidu B. A study of hypoglycemic and antioxidant activity of Aegle marmelos in alloxan induced diabetic rats. Ind J Physiol Pharmacol. 2004;48:476–480.
- Singanan V, Singanan M, Begum H. The hepatoprotective effect of bael leaves (Aegle marmelos) in alcohol induced liver injury in albino rats. Int J Sci Technol. 2007;2:83–92.
- Patil R, Chaudhary B, Settipalli S. Antifungal and antiaflatoxigenic activity of Aegle marmelos. Linn Pharmacog J. 2009;1:298–301.
- Schenk G, Ge Y, Carringto L, Wynne C, Searle I, Carroll B, Hamilton S, De-Jersey J. Binuclear metal centres in plant purple acid phosphatases: Fe–Mn in sweet potato and Fe–Zn in soybean. Arch Biochem Biophys. 1999;370:183–189.
- Durmus A, Eicken C, Sift B, Kratel A, Kappl R, Huttermann J, Krebs B. The active site of purple acid phosphatase from sweet potatoes (Ipomoea batatas). Eur J Biochem. 1999;260:709–716.
- Bozzo G, Raghotham K, Plaxton W. Purification and characterization of two secreted purple acid phosphatase isozymes from phosphate-starved tomato (Lycopersicon esculentum) cell cultures. Eur J Biochem. 2002;269:6278–6286.
- Zimmermann P, Regierer B, Kossmann J, Frossard E, Amrhein N, Bucher M. Differential expression of three purple acid phosphatase from potato. Plant Biol. 2004;6:519–528.
- Averill B, Merkx M. The activity of oxidized bovine spleen purple acid phosphatase is due to an Fe(III)-Zn(II) ‘impurity’. Biochemistry. 1998;37:11223–11231.
- Lowrey O, Rosebrough N, Farr L, Randall R. Protein measurement with the Folin phenol reagent. J Biochem. 1951;193:265–275.
- Dubois M, Gilles K, Hamilton J, Rebers P, Smith F. Colorimetric method for determination of sugars and related substances. Anal Chem. 1956;28:350–356.
- Klabunde T, Strater N, Frohlich R, Witzel H, Kreb B. Mechanism of Fe(III)–Zn(II) purple acid phosphatase based on crystal structure. J Mol Biol. 1996;259:737–748.
- Antanaitis B, Aisen P, Lilienthal H. Physical characterization of two-iron uteroferrin. J Biol Chem. 1983;258:3166–3172.
- Kaida R, Serada S, Norioka N, Norioka S, Neumetzler L, Pauly M, Sampedro J, Zarra I, Hayashi T, Kaneko T. Potential role for purple acid phosphatase in the dephosphorylation of wall proteins in tobacco cells. Plant Physiol. 2010;153:603–610.
- del Pozo J, Allona I, Rubio V, Leyva A, del la Pena A, Aragoncillo C, Paz-Ares J. A type 5 acid phosphatase gene from Arabidopsis thaliana is induced by phosphate starvation and by some other types of phosphate mobilizing/oxidative stress condition. Plant J. 1999;19:579–589.
- Nuttleman P, Roberts R. Transfer of iron from uteroferrin (purple acid phosphatase) to transferrin related to acid phosphatase activity. J Biol Chem. 1990;265:12192–12199.
- Ek-Rylander B, Flores M, Wendel M, Heinegards D, Andersson G. Dephosphorylation of osteopontin and bone sialoprotein by osteoclastic tartrate-resistant acid phosphatase. J Biol Chem. 1994;269:14853–14856.