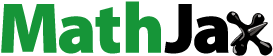
Abstract
This paper investigates interaction processes of the Fe3+, Cu2+, Ni2+, and Co2+ cations with humic acids isolated from brown coals of Ekibastuz basin. It was demonstrated that the sorption of heavy metal ions by humic acids (HA) depends on the concentration and nature of cations and decreases in the order of Fe3+ > Cu2+ > Ni2+ > Co2+; while increase in the concentration of humic acids (HA) results in the higher yield of ions and metals. The occurrence of ion exchange and complexation reactions in metal-humic acid systems is confirmed by the appearance of new bands and the shift of the absorption bands corresponding to stretching and deformation vibrations of carboxylate ions, C = O and OH groups. The occurrence of these processes is also evidenced by spectrophotometric data, as well as a decrease in the pH of the solution due to deprotonation of humic acids during these reactions. Thus, based on the data on the degree of sorption of metal cations by humic acids, the results of infra-red spectroscopy and spectrophotometry, it can be concluded that the Fe3+, Ni2+, Co2+, Cu2+ cations interact with HA by the ionic mechanism and by the complexation mechanism with electron-donating functional groups. Electrochemical studies of the Cu2+ – humic acid system carried out by the method of cyclic voltammetry also indicate the occurrence of the interaction of humic acid with copper cations. Modification of humic acid with copper ions shows the electrochemical activity of copper immobilized on the surface of dispersed humic acid, which is important for the implementation of electrocatalytic reactions on modified glassy carbon-humic electrodes.
Introduction
The presence of electron-donating functional groups in the structure of humic acids (HA), >C = O, -COOH, -C-OH, ArOH, -NH-, -NH2, in various combinations and free orbitals of metal cations contributes to the formation of coordination compounds during their interactions [Citation1–4]. This results in the following mechanisms: ion exchange, complexation with electron donor functional groups; complexation with the formation of a Men+-HA bond due to the presence of free π-electrons on the surface.
The complexing properties of humic acids are influenced by many factors: structural (functional) features; mesomeric and inductive effects inside macromolecules; acid-base characteristics; general physicochemical characteristics of the system, etc.
The applications including the fabrication of different structures of electrochemical sensors have been studied from obtained various carbon compounds [Citation5]. The catalytic properties of carbon materials obtained from natural sources are quite alternative [Citation6]. HA is obtained from a natural resource which has carbohydrate, amino acid, lipids, carboxylic acids and alcohols, aldehyde, ketone and quinone, and ether functional groups [Citation7]. HA has hydrophilic (water loving, polar) and lipophilic (fat-loving) properties, so it forms micelle like structure called pseudo-micelles [Citation8]. HA and metal ion forms complexes which lead to obtaining nano-sized particles that makes them useful in electrocatalytic system [Citation9].
The interaction of copper cations with humic acids of different origin has been studied [Citation1,Citation10]. As a result of the study, it was shown that the amount of sorption cations of copper depends on the functional and structural composition of humic acids and the pH of the medium and that several mechanisms contribute to the sorption process to varying degrees. The interaction of cations Fe(III) with humic substances has shown that carboxyl groups and oxygen-containing alkyl groups in the side chains of substituted aromatic rings are most likely to participate [Citation11]. They are renowned for their ability to bind a large range of metals and metalloids including possibly toxic elements such as REE, Al(III), Pb(II), Ca(II), Mn(II), Mg(II), Fe(III) and smaller amounts of As(V) [Citation12–17], As(III) [Citation12,Citation18–20], Sb(V) [Citation21–23], and Sb(III) [Citation13,Citation21,Citation23,Citation24]. The high binding capacity of HS is usually attributed to the large surface density of oxygen-containing functional groups (carboxylic, phenolic, carbonyl) and, to a lesser extent, nitrogen or sulfur-containing functional groups [Citation25].
Many researchers state that the complexity of the structure of humic substances causes certain difficulties to study their properties and it is the reason for their ambiguous interpretation. Thus, the researchers make cautious assumptions about the fact of the complex formation of Cu2+ with humic substances and emphasize that several mechanisms contribute to varying degrees in the process of sorption of copper cations with humic acids [Citation1].
The aim of the work is to study the interaction of heavy metal ions (Fe3+, Cu2+, Ni2+, Co2+) with humic acids by methods of IR spectroscopy, spectrophotometry, and cyclic voltammetry for isolated from brown coals of the Ekibastuz coal basin (Kazakhstan), and the establishment of interaction mechanisms.
2. Experimental part
2.1. Reagents
FeCl3, CuSO4, Ni SO4, CoSO4, Na2SO4, NaOH, HCI and H2SO4 were purchased from the chemical reagents trade company of Lapborpharma (Almaty, Kazakhstan). Nafion solution (5%) was purchased from Sigma-Aldrich; Merck KGaA (Darmstadt, Germany). Double distilled water was used in all of the experiments. All reagents and chemicals were of analytical grade.
The stock solution of metal salts (0.1 mol L−1) were prepared by dissolving the appropriate amount of salts in double-distilled water. Working electrolyte solutions’ pH fixed with sulfuric acid.
2.2. Synthesis of humic acid
Humates obtained from brown coal mined in the Ekibastuz coal basin (‘Maykuben’ and ‘Sarykol’ deposits) were used. To obtain humic acids and study their physicochemical properties, we used samples of sodium humate from “Asia Compogus Resource” company. Humic acids were isolated according to the method in which the sample is treated with sodium hydroxide and precipitated with hydrochloric acid [Citation3]. The initial samples were carefully restrained in a porcelain mortar, washed with hot distilled water, placed in centrifuge glasses. Then, a 0.1 M HCI solution was added to them at the rate of 50 ml per 5 g of sample. The suspensions were stirred for 30 min with a magnetic stirrer and centrifuged for 20 min. In this case, salts and carbohydrates pass into solutions. After centrifugation, the precipitates were placed in thin section flasks and dissolved in a 0.1 M NaOH solution. Under stirring, nitrogen gas was passed through the solution, the flasks were closed and left for 24 h to extract fulvic acids and humic acids.
After the extraction, the suspensions were centrifuged for 20 min, and the solutions were placed in flasks with a thin section and 6 M HCI was gradually added, adjusting the pH to 1.5 to separate the fulvic acids from humic acids, and nitrogen was passed and left for 20 h. After the specified time, the resulting precipitation of humic acids was separated from the liquid by centrifugation for 20 min. Humic acid precipitates were washed with a diluted solution and dried over P2O5 in a vacuum desiccator or in an oven at a temperature of 60–80 °C to a constant weight.
2.3. The adsorption, IR and spectrophotomer study
The experiments of the adsorption studies were conducted at room temperature (25 °C) in a 250 mL screw-cap conical flask. For each run, from 1 to 12 ml of the 12 adsorbent dosage was taken from 5% of HA soltution and placed in the flask containing 100 mL solution of salts (FeCl3, CuSO4, Ni SO4, CoSO4) of a 10−3 M concentration. The suspension was shaken by lab orbital shaker for 300 min and left 24 h. After agitation, the suspensions were filtered using Whatman no. 1 filter paper. The concentration of the filtrate was determined by using a UV-visible spectrophotometer and complexometric titration. The quantitative content of metals in the initial solution and in the solution that passed through the adsorption process with humic acids was determined by complexometric titration and spectrophotometer. The adsorption efficiency of metal ions was calculated by the difference between their initial and residual concentrations.
The filtered suspension was dried in an oven at a temperature of 60–80 °C to a constant weight. After dried which was send to IR spectroscopy for obtain IR spectra.
5% humic acid solution were prepared by NaOH solution, and pH was fixed with HCl to 2.1. After humic acids solutions with metal ions taken in the ratio metal: HA by volume 1:1; 2:1; 5:1 and 10:1. After obtained those solutions were filtered with filter paper. Filtrate were studied with spectrophotometer.
2.4. Apparatus and procedure
The properties of HAs were studied by IR spectroscopy, spectrophotometry, X-ray fluorescence analysis, and potentiometric titration. Infrared spectra were recorded on a Spectrum 65 Fourier transform IR spectrometer in tablets with KBr, in the range 450–4000 cm−1. Spectrophotometric analysis was carried out on an Agilent Technologies spectrophotometer in the range of wavelengths of 200–900 nm.
Voltammetric measurements were performed on an AUTOLAB potentio-galvanostat series PGSTAT101/M101. A classic three electrode cell system was used for the measurements. A glassy carbon electrode (GCE) with a composite system of HA and Nafion, a reference electrode of Silver/Silver Chloride (Ag/AgCl E0 = +0.222 V) and a counter electrode of glassy carbon were employed for the electrochemical studies. Nitrogen (N2) was bubbled into the solution for 10–25 min to remove dissolved oxygen before each measurement to ensure consistent data acquisition. All studies were carried out at a temperature of 25 °C. The potentials were scanned from +0.7 or +0.5 V to −0.5 V at a scan rate of 50 mV s−1.
2.5. Preparation of the modified electrode
The GCE was sequentially polished with 1.0, 0.3 and 0.05 µm alumina slurry on velvet. The residual alumina slurry was rinsed off with distilled water, and the GCE was placed in anhydrous ethanol and distilled water with ultrasonication (60 kHz, 55 °C) for 10 min. The cleaned GCE was dried with an infrared lamp. The humic acid (10 mg) and Nafion solution (10 µl) were dispersed in 10 ml anhydrous ethanol with ultrasonication (60 kHz, 55 °C) for 30 min to obtain a homogenous suspension. The humic acid (500 mg) and metal solutions (0.1 M, 10 mL) were mixed with ultrasonication (60 kHz, 55 °C) for 10 min and left for 24 h. Humic acid-metal precipitates were washed with a double distilled water and dried over P2O5 in a vacuum desiccator or in an oven at a temperature of 60–80 °C to a constant weight.
The humic acid-Nafion (HN) and humic acid–metal–Nafion (HMN) modified electrode was prepared by casting 5 µl suspension onto the pretreated GCE, followed by drying with an infrared lamp.
3. Results and discussion
3.1. Study of the interactions between HA and metal ions by complexometric titration, IR spectroscopy and spectrophotometry
To establish the nature of the sorption of transition metal ions with humic acids, the interaction of Fe3+, Cu2+, Ni2+, Co2+ with HAs extracted from brown coals of the Ekibastuz basin was studied. For the study, 10−3 M diluted model solutions were used from stock solution of 0.1 M of FeCl3, CuSO4, Ni SO4, CoSO4.
The quantitative content of metals in the initial solution and in the solution of that suspensions were filtered using Whatman no. 1 filter with humic acids was determined by complexometric titration and photocolorimetry. The absorption efficiency of metal ions was calculated by the difference between their initial and residual concentrations.
shows the dependence of the degree of absorption of metal ions on the consumption of HA. The figure shows that with an increase in the concentration of humic acid, the degree of absorption of metal ions increases. The highest degree of recovery (−90%) is observed for iron (III), weaker for copper (II), even weaker for nickel (II) and cobalt (II).
Such a sequence in the ability of metal ions to complex with humic acids can be explained from the standpoint of the theory of hard and soft acids and Pearson bases. Hard acids are electron acceptors in which the atoms of acceptors are small in size, have a large positive charge, sufficiently high electronegativity and low polarizability. The lowest free molecular (MO) orbital (through which chemical bonding occurs) has low energy. An example of a hard acid can serve as the ion Fe(III).
Soft acids are acceptor particles with large size with a small positive charge, low electronegativity and high polarizability. The lowest free molecular orbital is high in energy. Soft acids contain easily polarizable vacant orbitals. The positive charge of the atom-the electron acceptor is small due to the delocalization or no ion.
Hard base-the donor particles having a high electronegativity and low polarizability, the highest occupied molecular orbital, which is involved in the binding with the acceptor orbital has the lowest energy (closest to the nucleus). Example – an atom of oxygen.
Hard acid requires a hard base to form a bond, that is, complexation proceeds according to the principle: hard acid forms a coordination bond with a hard base and soft acid with a soft base. In this regard, it is clear that the ion Fe3+ is the best interacts with humic acid which is more stringent acid (charge +3) among the investigated cations. Sorption of copper ions is higher than that of ions of nickel and cobalt, it is difficult to explain from the perspective of the theory of hard and soft acids, and ions of nickel and copper have the same charge and similar radii-to 0.069 and 0,073 nm, respectively. It all lies in the degree of covalence of the Me-O bond, which is higher for a copper (2.3 nm) ion than for nickel and cobalt ions (1.92 and 1.83 nm).
The presence of active carboxyl groups in the studied humic acids determines the ion exchange process, which is confirmed by an increase in the acidity of the solution upon addition of metal salts compared to the initial one, and a decrease in the pH of solutions by an average of two units. In this case, the protons of the carboxyl groups are exchanged for the cations of metals following the equation:
The appearance in the IR spectra of the samples containing cations sorbing metal strip in the area of 1380–1400 cm−1 (symmetric vibrations of the carboxylate ion) also confirms the occurrence of ion exchange processes, the reactions of complex formation indicates the shift of absorption bands and intensity changes in the region 1700–1720 cm−1 (valent C = O vibrations), 1230–1260 cm−1 (deformation vibrations O-H). These two bands are strongly pH-dependent. Upon the addition of Fe(III), the relative intensities of the bands related to the carboxylate group increase while the one related to the carboxylic group decreases. This is due to the complexation of carboxylate by Fe(III) and to the displacement of the equilibrium between carboxylic and carboxylate groups. Additionally, communication absorption band Me-O is enhanced ().
A spectrophotometric study of these systems also confirms the interaction of humic acids with cations Fe3+, Ni2+, Co2+, Cu2+ ( and ). show the spectra in the UV and visible regions for humic acids (pH = 2.1) and their solutions with metal ions taken in the ratio metal: HA 1:1; 2:1; 5:1 and 10:1. After added salts’ solution pH was changed 2.39, 2.45, 2.4, 2.67 respectively Fe3+, Ni2+, Co2+, Cu2+. It follows from the figures that, with an increase in the concentration of cations, the peak intensity of humic acid at 240 nm decreases. This indicates the interaction of humic acids with metal cations, leading to a decrease in the concentration of free HA.
Peaks that were present in solutions of metal salts (for Ni2+ at 400 nm, for Cu2+ at 300 nm) are not reflected in respective solutions with humic acids. This implies interaction of the metal salts with HA: as the concentration of cations decreases, the intensities of these peaks. As we noted, of the studied metal cations, Fe3+ cations interact most strongly with HAs. On the spectrum of the Fe3+ -HA system, in contrast to the spectra of other systems, a new peak with a maximum at 230 nm appears, which probably corresponds to the complex of iron (III) with humic acid.
Thus, based on the data on the degree of sorption of metal cations by humic acids, the results of IR spectroscopy and spectrophotometry, we can conclude that the Fe3+, Ni2+, Co2+, Cu2+ cations interact with HA by the ionic mechanism and by the complexation mechanism with electron-donating functional groups.
3.2. Electrochemical studies
To confirm the interaction of metal ions with HA and to reveal the features of the electrochemistry of the metal-HA system, electrochemical studies of the Cu-humic acid system were carried out.
shows the results of CVA in integral form, which were obtained in 0.1 M Na2SO4 at pH = 3.5 on a glassy carbon electrode (GCE) and its surface-modified humic acid with Nafion analogues. Moreover, the visible surface used in the research of the GCE is S = 3.14•10−2cm2. The linear sweep cyclic voltammograms were scanned repetitively from anodic (+0.5 V) to cathodic (−0.5 V/SCE) potentials and back, scan rate 50 mV/s. The stationary potential of the working electrode Еstart = −0.18 V.
Figure 6. Linear sweep cyclic voltammetry of Cu (II) (10−4 mol L−1) 0.1 mol L−1 Na2SO4 (pH 3.5) solution at glassy carbon electrodes modified with: (1) CuHA; (2) HA; (3) nafion; (4) GCE. Scan rate 50 mV s−1.
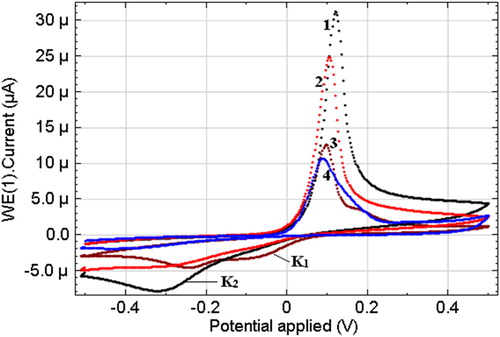
Linear scanning was carried out in the range from Estart to −0.5 V in the cathode direction. Then, at E = −0.5 V there was a time delay. Further scanning was in the anode direction to E = 0.5 V. In this case, copper oxidation proceeds at E = 0.12 V (). The behaviour of copper in an aqueous medium is known from the literature, where the wave of copper oxidation is attributed to the electrochemical transition Cu0 to Cu +1, which can then be oxidized from Cu +1 to Cu+2. In the reverse course of CVA from E = 0.5 V to E = −0.5 V in the cathode branch, two waves of copper recovery K1, K2 are observed. It can be assumed that the K1 wave is the reduction of copper from Cu2+ to Cu+1, and the K2 wave is the reduction of copper from Cu+2 to Cu0.
Based on the experimental data, it is possible to determine the electrochemical activity of the GEM modified with humic acid in the presence of copper ions in the electrolyte. In addition, the high sensitivity of the modified humic acid electrode surface is shown.
It is known that redox potentials and rates of electrochemical reactions depend on the acidity of the medium and the properties of the electrode (electrical conductivity, specific surface area).
shows the results of electrochemical studies obtained on the surface of the GCE, modified with HA with Nafion in 0.1 M Na2SO4, in the presence of СCu+2 = 10−4 M at various pH environments (pH = 3.5; 4.5; 5.5; 6.5). The shape of the obtained integral CVA curves shows a significant difference in the data depending on the pH medium.
Figure 7. Linear sweep cyclic voltammetry of Cu (II) (10−4 mol L−1) solution at the CuHA-GCE. Scan rate 50 mV s−1. Electrolyte: 0.1 mol L−1 Na2SO4 solution at (1) pH 3.0, (2) pH 4.5, (3) pH 5.5 and (4) рН 6.5.
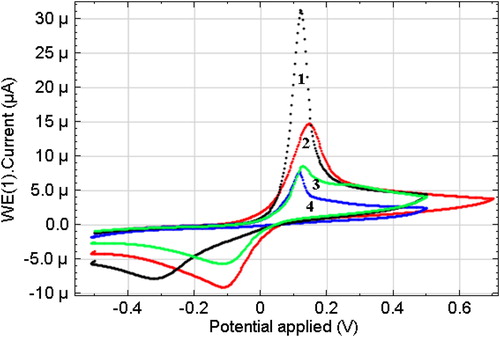
The presence of peaks of copper oxidation in these systems indicates the formation of copper cations, which are known to interact with humic acids [Citation11,Citation26–29].
The work performed on the modification of humic acid with copper ions (Cu+2) showed the electrochemical activity of copper immobilized from a volume of dispersed humic acid on the surface of the powder. Moreover, these particles are in a stable, ordered state, which is important for the implementation of electrocatalytic reactions on modified GC electrodes.
4. Conclusions
The most important processes in humic acid systems with heavy metal ions are sorption and complexation. It was shown that with an increase in the concentration of humic acid, the sorption of metal ions increases. The highest degree of extraction (∼90%) is observed for iron (III), weaker for copper (II), even weaker for nickel (II) and cobalt (II). The results of IR spectroscopy and spectrophotometry, along with data on the degree of sorption of metal cations by humic acids, allow us to conclude that they interact both by the ionic mechanism and by the complexation mechanism with electron-donating functional groups –COOH, –C — OH,> C = O. The CVA method showed the electrochemical activity of copper immobilized from a volume onto the surface of humic acid, which can be used to carry out electrocatalytic reactions on modified electrodes.
Disclosure statement
No potential conflict of interest was reported by the author(s).
Additional information
Funding
References
- Zherebtsov SI, Malyshenko NV, Bryukhovetskaya LV, et al. Sorption of copper cations from aqueous solutions by brown coal and humic acids. Solid Fuel Chem. 2015;49(5):294–303. Russian.
- Dinu MI. Interaction of metals ions in waters with humic substances of gley-podzolic soils. Geochemistry. 2015;2015:276–288. Russian.
- Dinu MI. Comparison of the complexing abilities of fulvic acids and humic acids in an aquatic environment with iron and zinc ions. Water Resour. 2010;37(1):65–69. Russian.
- Savelyeva AV, IvanovA.A YNV. Effect of structural characteristics of humic acids on the effectiveness of interaction with polyvalent metal cations. Chem Plant Raw Mater. 2015;4:77–83. Russian.
- Murugan N, Prakash M, Jayakumar M, et al. Green synthesis of fluorescent carbon quantum dots from Eleusine coracana and their application as a fluorescence ‘turn-off’ sensor probe for selective detection of Cu 2+. Appl. Surf. Sci. 2019;476:468–480..
- Murugan N, Sundramoorthy AK. Green synthesis of fluorescent carbon dots from Borassus flabellifer flowers for label-free highly selective and sensitive detection of Fe3+ ions. New J Chem. 2018;42(16):13297–13307.
- Shulga YM, Baskakov SA, Baskakova YV, et al. Hybrid porous carbon materials derived from composite of humic acid and graphene oxide. Microporous Mesoporous Mater. 2017;245:24–30. http://dx.doi.org/10.1016/j.micromeso.2017.02.061.
- De Melo BAG, Motta FL, Santana MHA. Humic acids: structural properties and multiple functionalities for novel technological developments. Mater Sci Eng C Mater Biol Appl. 2016;62:967–974. http://dx.doi.org/10.1016/j.msec.2015.12.001.
- Zhou S, Chen S, Yuan Y, et al. Influence of humic acid complexation with metal ions on extracellular electron transfer activity. Sci Rep. 2015;5:17067–17069. http://dx.doi.org/10.1038/srep17067.
- Lopes WT, Thobie-Gautier C, Rezende MOO, et al. Electrochemical behavior of Cu (II) on carbon paste electrode modified by humic acid. Cyclic Voltammetry Study. 2002;14(1):71–77.
- Fuentes M, Olaetxea M, Baigorri R, et al. Main binding sites involved in Fe(III) and Cu(II) complexation in humic-based structures. J. Geochemical Explor. 2013;129:14–17.
- Redman AD, Macalady DL, Ahmann D. Natural organic matter affects Arsenic speciation and sorption onto hematite. Environ Sci Technol. 2002;36(13):2889–2896.
- Buschmann J, Sigg L. Antimony(III) binding to humic substances: influence of pH and type of humic acid. Environ Sci Technol. 2004;38(17):4535–4541.
- Lin HT, Wang MC, Li GC. Complexation of arsenate with humic substance in water extract of compost. Chemosphere. 2004;56(11):1105–1112.
- Bauer M, Blodau C. Mobilization of arsenic by dissolved organic matter from iron oxides, soils and sediments. Sci Total Environ. 2006;354(2-3):179–190.
- Ritter K, Aiken GR, Ranville JF, et al. Evidence for the aquatic binding of arsenate by natural organic matter-suspended Fe(III). Environ Sci Technol. 2006;40(17):5380–5387.
- Kar S, Maity JP, Jean JS, et al. Role of organic matter and humic substances in the binding and mobility of arsenic in a Gangetic aquifer. J Environ Sci Heal Part A Toxic/Hazard Subst Environ Eng. 2011;46(11):1231–1238.
- Warwick P, Inam E, Evans N. Arsenic’s interaction with humic acid. Environ Chem. 2005;2(2):119–124.
- Buschmann J, Kappeler A, Lindauer U, et al. Arsenite and arsenate binding to dissolved humic acids: influence of pH, type of humic acid, and aluminum. Environ Sci Technol. 2006;40(19):6015–6020.
- Liu G, Cai Y. Complexation of arsenite with dissolved organic matter: conditional distribution coefficients and apparent stability constants. Chemosphere. 2010;81(7):890–896.
- Pilarski J, Waller P, Pickering W. Sorption of antimony species by humic acid. Water Air Soil Pollut. 1995;84(1-2):51–59.
- Tighe M, Lockwood P, Wilson S. Adsorption of antimony(v) by floodplain soils, amorphous iron(III) hydroxide and humic acid. J Environ Monit. 2005;7(12):1177–1185.
- Filella M. Antimony interactions with heterogeneous complexants in waters, sediments and soils: a review of data obtained in bulk samples. Earth-Science Rev. 2011;107(3-4):325–341.
- Tella M, Pokrovski GS. Antimony(III) complexing with O-bearing organic ligands in aqueous solution: an X-ray absorption fine structure spectroscopy and solubility study. Geochim Cosmochim Acta. 2009;73(2):268–290.
- Evangelou VP, Marsi M. Composition and metal ion complexation behavour of humic fractions derived from corn tissue. Plant Soil. 2001;229(1):13–24.
- Lishtvan II, Kaputsky FN, Yanuta Yu G, et al. The interaction of humic acids with metal ions and the structure of metal humic complexes. Bull Belarusian State Univ Ser. 2012;2:12–16. Russian. http://elib.bsu.by/bitstream/123456789/45291/1/humicacids.pdf.
- Dmitrieva ED, Syundyukova K, Leontiev MM, et al. Influence of the ph of the media on the binding of ions of heavy metals by humic substances and hymatomelanic acids of peat. Acad Rec Kazan Univ Ser Nat Sci. 2017;159:575–588. Russian.
- Sokolova CA, Tsyplakov WE, Kotov V, et al. Determination of concentration stability constants of complexes of heavy metal ions with humic acids. Sorption Chromatogr Process. 2013;13:162–172. Russian.
- Zhernakova ZM. The interaction of metal ions with humic substances in natural environments. Water Chem Ecol. 2011;6:76–81. Russian.