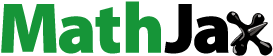
ABSTRACT
In this work, a detailed study on the ethanosolv lignin fractionation from endocarps, aiming at understanding the role played by the ball milling (BM) pretreatment, is presented. Walnut and pistachio shells were chosen as a case study, since they exhibit excellent mechanical properties and their tissues have been extensively studied in terms of structure, composition, and lignin distribution. Here, it was demonstrated that the BM pretreatment is not particularly suited for these biomasses, since it is not able to promote the lignin fractionation from the whole tissue, and additionally, it triggers the oxidation of the substrate. Although in the case of walnut shell this oxidation can be prevented thanks to the remarkable radical scavenger activity (RSA) of its extractives, in the case of pistachio shell the BM is responsible for a partial lignin condensation. Pistachio shells resulted a particularly attractive feedstock since they can be easily delignified using mild ethanosolv processes, leading to highly regular lignin fractions, rich in S units (86%) and β-O-4 linking motif (>60% C9 units), so particularly suited for the chemical upgrading to added-value aromatics.
GRAPHICAL ABSTRACT
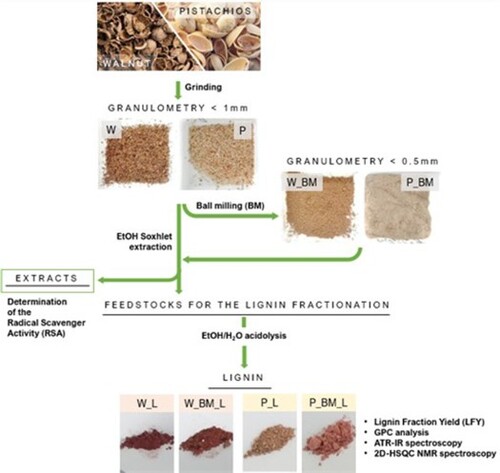
Introduction
Lignocellulosic biomasses (LCBMs) are the most abundant source of renewable carbon on the Earth. The development of an efficient and versatile process able to convert the three main constituents of LCBMs, i.e. cellulose, hemicellulose and lignin, into economically viable products is the keystone for guaranteeing a more sustainable future for the chemical industry.[Citation1] Biorefinery, i.e. the facility that aims to generate a wide range of different products (chemicals, fuels and materials) starting from biomass feedstocks,[Citation2] represent a mature technology especially in the field of biofuel production,[Citation3] since LCBMs are currently exploited mainly for the production of cellulosic ethanol.[Citation4] If we move our interest in the development of added-value chemicals and materials, fundamental is the role of the lignin as the primary natural source of aromatics.[Citation5] Since the first steps of biorefining are generally focused on the extraction of cellulose, due to the recalcitrance of LCBMs to chemical or biological treatments, drastic reaction conditions are usually employed for the complete delignification of the biomasses, leading to highly degraded lignin fractions (technical lignin) which are prevalently burned in industrial boilers for energy recovery.[Citation6] Albeit several approaches have been proposed for the chemical valorization of the lignin, such as redox-active material for energy storage applications,[Citation7–9] adhesive formulation,[Citation10–12] thermoplastic resins,[Citation13] UV-filter,[Citation14] the economic sustainability of these processes is still elusive.[Citation15,Citation16] In this context, particularly interesting are the so-called ‘lignin-first’ approaches, which consist in the lignin acidolysis in organic solvents (organosolv) using mild conditions, followed by the reductive stabilization of the reactive intermediates.[Citation17,Citation18] Therefore, it is possible to separate the lignin from the cellulosic pulp without strongly altering the native structure of the aromatic biopolymer, giving a substrate more suitable for further valorizations. In fact, organosolv lignins result highly pure (no sugar or ash contaminations), with a good retention of the β-O-4 motif (the main linking motif in the native lignin) and with a low level of condensation (formation of new unreactive C–C bonds), therefore they are more valuable compared to technical lignin derived as a byproduct from pulping manufacturing.[Citation19] In the organolsolv panorama, the ethanosolv fractionation is probably one of the most promising strategies since lignin acidolysis and solubilization are performed in hydroalcoholic mixtures.[Citation20–24] Ethanol is a green non-toxic solvent that can be directly obtained by the fermentation of the remaining cellulosic pulp in a completely self-sustainable perspective,[Citation20,Citation25] while the use of hydroalcoholic mixtures allows to considerably reduce the process costs.[Citation26] Furthermore, alcohols prevent the lignin condensation induced by strong acid conditions through α-alkoxylation reactions, which contribute to improve the solubility of the biopolymer in organic solvents.[Citation20,Citation21,Citation27,Citation28] Hence, if we want to move one step forward in the design of low-cost and efficient ethanosolv protocols, every step of the process must be carefully optimized starting from the identification of the best feedstocks. The lignin fractionation from endocarp shells, and especially from dried nutshells, is a cost-effective solution since these LCBMs are classified as an industrial waste with high lignin content,[Citation29] and are produced as a byproduct of the shelling operations. Crushed dried nutshells are easy to handle and do not require a drastic mechanical reduction in size to be chemically processed. Usually, the mechanical size reduction of LCBMs is performed with several milling techniques aiming to improve their handling and the bio-accessibility of their constituents, afforded by the increment of the total surface area of the starting feedstock.[Citation30] This step should be fast, with low capital and operational cost, without determining the degradation of sugars or lignin.[Citation1] Specifically, the effects induced on the lignin structure by the milling pre-treatment are not yet fully clear and well evaluated.[Citation31] Several studies on milled wood lignin (MWL), which is considered the most similar to native lignin among those extracted, led to contradictory results regarding its structure,[Citation32,Citation33] suggesting an active role of the BM process in altering the native structure of the aromatic biopolymer.[Citation31] In fact, the BM promotes the formation of radical species through the breaking of the β-O-4 bounds which causes the further degradation of the biomass.[Citation34] Furthermore, the cracks indued into the feedstock tissue can facilitate the extraction of lignin from different regions of the biomass, increasing the heterogeneity of the obtained fractions.[Citation35] Is the BM really useful for increasing the lignin fractionation yield (LFY), especially in the case of LCBMs characterized by excellent mechanical properties such as dried nutshells?[Citation36] Does the BM induce structural alterations in the lignin structure, and are these alterations effective to improve the final properties of the aromatic biopolymer? This work aims at answering to these questions by assessing the effects induced by a mild BM pretreatment on the ethanolsolv fractionation of lignin from walnut and pistachio shells (). These two LCBMs were selected as starting feedstocks for this study since their tissues were extensively characterized in terms of composition, structure, and lignin distribution,[Citation36–38] allowing to easily determine the influence of the BM pretreatment on the fractionation process. To investigate the structural changes induced by the BM pretreatment, all the lignin samples were characterized by gel permeation chromatography (GPC), Attenuated Total Reflection-Fourier Transform Infrared (ATR-FTIR) spectroscopy and 2D heteronuclear single quantum coherence (HSQC) nuclear magnetic resonance (NMR) spectroscopy. This multi-technique characterization allowed us to demonstrate that the BM pretreatment is not effective in promoting the delignification of the endocarp shells, while can strongly affect the lignin structure in terms of molecular weight, condensation, S/G unit ratio and solvent incorporation via ethoxylation reactions. These effects were prevalently attributed to the oxidative stress induced by the BM pretreatment, as demonstrated by quantifying the radical scavenger activity (RSA) of the ethanolic extracts obtained from the two shells before and after the BM pretreatment (). In fact, it is reported that extracts from endocarp shells display a remarkable antioxidant activity,[Citation39,Citation40] hence they can play a key role in preventing the biomass oxidation leading to lignin fractions considered of superior quality.
Materials and methods
Biomass pretreatment
Dried walnut shells (cultivated in Apulia, Italy) were crushed into small fragments using a hammer and sieved with 1 mm sieve (sample W in ). The same approach was tested on dried pistachio shells (Pistacia vera L. Var. Bronte), but in this case, a large fraction of the starting feedstock maintained a granulometry higher than 1 mm. Hence, pistachio shells were broken using an immersing blender. After the blade milling, a large part of the raw material already showed a powdery aspect, thus the biomass was sieved to guarantee a granulometry lower than 1 mm but higher than 0.5 mm (sample S in ). In this way, it was possible to obtain a sample with a similar granulometry compared to W sample. Both W and S were subjected to a further size reduction using a ball milling apparatus (Fritsch pulverisette 7) equipped with two stainless steel reactors of 12 mL. Each reactor was loaded with approx. 2 g of biomass, together with 10 stainless steel spheres of 5 mm diameter, and 3 stainless steel spheres of 7 mm diameter. In this way, two-thirds of the reactor volume resulted occupied. The BM pretreatment consisted of 8 cycles of 5 min at 450 rpm, interspersed by 10 min of pause to avoid the excessive heating of the LCBM. After the BM pretreatment, the biomasses were sieved to guarantee a granulometry lower than 0.5 mm (samples W_BM and P_BM in ).
Extractives removal and determination of their radical scavenging activity (RSA)
Each sample (W, W_BM, P, P_BM) was subjected to an extraction with ethanol (Panreact, 99.8%) for 12 hours using a Soxhlet apparatus to remove the extractive fraction (). The extracts (W_E, W_BM_E, P_E, P_BM_E) were dried under reduced pressure, stored in air and at room temperature. The extraction yield, expressed as % w/w, was calculated as the average value of three extraction processes. The radical scavenger activity (RSA) of each extract was determined via 2,2-Diphenyl-1-picrylhydrazyl (DPPH) (Sigma-Aldrich) assay (three replicates) using a modified version of a protocol reported in literature.[Citation41–43] For each extract, the inhibitor concentration (IC50) was calculated in comparison with the gallic acid one (Sigma Aldrich, ACS reagent, ≥ 98.0%). A stock solution of DPPH (0.20 mM) in ethanol (Panreact, 99.8%) was prepared and stored at 4°C in the dark. Stock solutions of the extracts (ranging from 0.125 to 25 μg / mL in ethanol) were prepared and stored at 4°C in the dark. The working solutions for the DPPH assay were prepared as follows: 50 μL of extract solution and 100 μL of DPPH solution were added to 850 μL of absolute ethanol to obtain a final volume of 1 mL in a plastic cuvette having an optical path of 1 cm.[Citation43] The absorbance of the working solution between 400 and 600 nm compared to the reference solution (100 μL of DPPH solution in 900 μL of absolute ethanol) was measured using a UV / Visible double beam spectrophotometer (Shimadzu UV- 1601 PC, Japan) after 20 min of incubation at 25°C in the dark.
Lignin fractionation
The lignin fractionation was conducted using a protocol based on a procedure reported in the literature.[Citation21] In a single neck round-bottom flask of 100 mL equipped with a magnetic stirrer and a refrigerator, 4 g of the starting feedstock were weighted. 40 mL of ethanol (Panreact 99.8%) and MilliQ water in the ratio 4:1 were added. Concentrated HCl (Sigma Aldrich, 37% w/w) was added to a final concentration of 0.24 M. The mixture was heated at 85°C for five hours, cooled at room temperature and the extract was collected by filtration and concentrated at reduced pressure. 40 mL of MilliQ water were added to the obtained solid to solubilize the degraded hemicellulose and disperse the water-insoluble lignin. After 15 minutes of sonication, the lignin suspension was centrifuged at 5000 rpm for 10 min, the supernatant was removed, and the centrifugation was repeated until the pH was neutralized. The obtained lignin fractions (W_L, W_BM_L, P_L, P_BM_L in ) were dried at 60°C overnight and stored in air in the dark.
Lignin characterization
Gel permeation chromatography
The ponderal molecular weight (MWW) of each sample was determined using a Knauer Gel permeation chromatography (GPC) system (Model S 2520 HPLC, Berlin, Germany) equipped with an ultraviolet detector (wavelength: 254 nm), using a column TOSOH Corporation (Tokyo, Japan) TSKgel G2000HHR, column size 7.8 mm I.D. × 30 cm, particle size 5 µm with exclusion limit (Polystyrene) equal to 1 × 104. The Column was held at room temperature with a 1 ml/min flow rate. Tetrahydrofuran (THF) (Sigma-Aldrich, ≥ 99.9%, inhibitor-free) was used as eluent.
ATR-FTIR spectroscopy
Attenuated Total Reflection-Fourier Transform Infrared (ATR-FTIR) spectra were recorded on a spectrophotometer (Spectrum Two Perkin Elmer) with a DTGS detector in the region of 4500–500 cm−1 at a resolution of 4 cm−1, using a scan time of 2 min with 15 scanning transients.
HSQC-NMR spectroscopy
For the lignin analysis, ca. 20 mg of lignin were dissolved in 0.7 mL of d6-acetone (Sigma-Aldrich). A few drops of D2O (Sigma-Aldrich) were added to ensure that lignin did fully dissolve. The sample P_BM_L required more D2O to be completely dissolved compared with other samples. NMR analysis was performed on a Agileny500-vnmrs500 (500 MHz) using the following parameters: (F2 = 14 to −1 ppm), (F1 = 160 to −10 ppm), nt = 32, ni = 128, d1 = 1 and pulse sequence gHSQC. Analysis was performed with MestReNova using a protocol reported in the literature.[Citation27]
Results and discussion
At a first analysis, the effect of the BM pretreatment in terms of lignin fractionation yield (LFY) from the two LCBMs (expressed as % w/w of the starting feedstock) was evaluated. For the development of ethanosolv fractionation conditions, HCl (0.24 M) was preferred to other acids such as H2SO4, HBr or organic acids (formic acid, acetic acid) since it is cheaper and it leads to higher delignification yields and lower molecular weight of resulting lignin fractions.[Citation20–22] The EtOH/H2O ratio was fixed at 4:1 because it was observed that the best delignification yields were obtained using an EtOH percentage between 75% and 85% vol.[Citation25] Finally, a temperature of 85°C was chosen to limit the lignin degradation ensuring an efficient delignification.[Citation10] LFYs are reported in (a). The untreated biomasses showed a completely different behavior compared to those subjected to the BM pretreatment: while in the case of walnut shell, the BM pretreatment promoted the lignin extraction (W_BM_L = 4.99% vs. W_L = 4.74%), in the case of pistachio shell the trend was opposite (P_L = 4.68% vs. P_BM_L = 4.28%). The enhancement of the LFY observed in the case of W_BM_L was however modest, and it was not such as to justify the cost of a BM pretreatment in terms of time and energy consumption. This is an important result since the elimination of an expensive step, such as the BM, can considerably increase the economic sustainability of the lignin valorization. Surprisingly, despite a huge difference in the lignin content between the two biomasses, the resulting LFYs were almost comparable, indicating a higher level of delignification for pistachio shells. In fact, walnut shells are considered a sort of benchmark in the field of lignin fractionation due to the elevated lignin content that reaches values around the 40% w/w[Citation21,Citation22,Citation44]; while pistachios shells are generally ignored for these studies due to lower lignin content (ca. 17% w/w)[Citation44,Citation45] compared to other endocarps, being most of the efforts focused on the valorization of its sugar fractions.[Citation46–50] A possible explanation at the base of the different recalcitrance exhibited by the two starting biomasses to the ethanosolv delignification, can be provided by the analysis of the tissue structure of the two biomasses. In the case of the walnut shell, the lignin is almost equally distributed between the cell walls (CWs) and the middle lamella compound (MLC) which surrounds each cell,[Citation38] while for the pistachio shell the lignin is more concentrated in the latter. Considering also the higher superficial density of the pistachio shell cell (the CWs show a more polylobate surface),[Citation38] the availability of lignin in the MLC for these two biomasses is almost comparable. Taking into account the low severity factor of our process,[Citation21] it is reasonable to assume that the MLC represented the main source of lignin while the biopolymer contained in the CWs resulted in less availability, even introducing a BM pretreatment. This result is extremely important because demonstrates that: (i) the mere lignin content of the LCBM is not a suitable parameter to be considered in the choice of the starting feedstocks for lignin fractionation studies using mild conditions, while a detailed characterization of the tissue structure should be considered, (ii) the BM pretreatment is not effective in promoting the lignin extraction from the whole tissue in the case of LCBMs having excellent mechanical properties. Moving to the structural properties of the different samples, the lignin ponderal molecular weight (MWW) was determined through GPC analysis, using polystyrene as a standard and tetrahydrofuran as a solvent ((b)). For all samples, the MWW was around 4 kDa, which is slightly higher compared with literature data collected in similar conditions.[Citation20–22,Citation27,Citation51] In particular, a good correlation between the MWW and the LFY was noticeable, being higher values of LFY associated to lower MWW. Thus, the decrement of LFY observed for the sample P_BM_L can be correlated to a partial lignin condensation induced by the BM: the BM behaves as an oxidant process that promotes the formation of reactive C• and O• radical species through the breaking of ether bonds in lignin and sugars.[Citation34] For this reason, the oxidative stress exerted by the BM on the two biomasses was determined by analyzing the antioxidant properties of their extractives obtained before and after the BM pretreatment. In fact, it is reported in the literature that nutshells are a precious source of bioactive molecules with a remarkable antioxidant and radical scavenger activity (RSA).[Citation39,Citation52,Citation53] The extraction of these low molecular weight compounds contained in the shells including fatty acids, phenols, polyphenols, flavonoids, simple sugars, etc., is typically carried out before the lignin fractionation to avoid lignin contaminations. Monitoring the RSA of walnut and pistachio shell alcoholic extracts, obtained before and after the BM step, allows the estimation of the oxidative stress induced by the mechanical size reduction of the starting biomasses. The extraction yields for extractives from the four different samples (W_E, W_BM_E, P_E, PBM_E) obtained via Soxhlet extraction for 12 hours using absolute ethanol as a solvent are reported in (c).
Figure 2. (a) Lignin fractionation yield (LFY) obtained from walnut and pistachio shells subjected or not to a BM pretreatment. (b) Ponderal molecular weight (MWw) distribution of the lignin fractions determined by GPC analysis (THF). (c) Soxhlet extraction yield (12 hours with ethanol) of walnut and pistachio shells before (W_E, P_E) and after (W_BM_E, P_BM_E) the BM pretreatment.
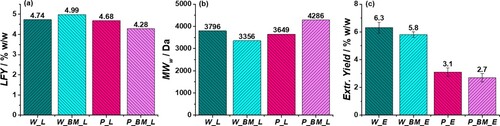
The extraction resulted less effective on substrates where the mechanical size reduction was performed using the BM, i.e. W_BM_E and P_BM_E. In particular, the extraction yield for samples with higher granulometry (W_E and P_E) was the 10% higher, despite the lower superficial density of the starting feedstock. This outcome can be attributed to two possible effects: (i) a partial degradation of the extractives during the BM; (ii) a lower permeability of the solvent to the biomass as a result of the high packing originated by the lower granulometry (note that during the Soxhlet extraction the biomass is not stirred). The former hypothesis is supported by the determination of the RSA of each extract. The %RSA was evaluated via DPPH colorimetric assay using the following equation:
(1)
(1) where Abscontrol represents the value of absorbance of the control solution (0.02 mM of DPPH in ethanol), Absblank is the absorbance of the ethanol solution containing the extract at a given concentration without DPPH, and Abssample is the absorbance of the DPPH solution added with the given extract concentration. All the absorbance values were registered at 517 nm wavelength. From the linear fitting of the %RSA at different extract concentrations, it can be calculated the inhibitor concentration (IC50) of each sample, i.e. the concentration of extract needed to decrease the free radical concentration by the 50%. These values are reported in alongside the IC50 of the gallic acid, typically used as the target antioxidant species.
Table 1. Inhibitor concentration (IC50) of the ethanolic extracts from walnut and pistachio shells before (E) and after the BM pretreatment (BM_E).
The sample W_E exhibited the lowest IC50 value (the highest RSA) among all the samples and was comparable with the one of the gallic acid. The BM strongly affected the RSA of the extractive phases in the case of walnut shells, in fact the sample W_BM_E showed an IC50 four orders higher than the one of W_E, indicating the effective formation of radical species during the BM process that were scavenged by the extractives contained in the biomass. Hence in the case of walnut shells, the abundance of extractives (6.3% w/w) together with their remarkable RSA, afforded protection to the lignin during the BM, avoiding the condensation of the aromatic biopolymer and facilitating its extraction during the ethanosolv fractionation. On the contrary, in the case of the pistachio shells, the biomass contained a lower amount of extractives (3.1% w/w) with a RSA that resulted in three orders lower than the one of walnut shells. Hence, in this case, the RSA of the extract was not sufficient to prevent the structural damages to the lignin induced by the radical species generated during the BM.
To further characterize the molecular structure of the lignin fractions, the ATR-FTIR characterization was performed. The ATR-IR spectra of W_L, W_BM_L and P_L, P_BM_L, respectively are reported in (a,b).
In both cases, the BM pretreatment is not responsible for drastic variations in the band shape of the spectra. This observation highlights that the BM does not significantly change the core of the lignin structure. For walnut shell lignin it can be seen a slight variation in the relative intensity of the signals at 1590, and 1505 cm−1 due to aromatic skeleton vibrations,[Citation51] suggesting a different S/G/H ratio, while in the sample P_BM_L the signal at 1270 cm−1, attributed to the stretching C–O of the guaiacyl ring,[Citation54–56] has a higher intensity compared to P_L. Hence, for both biomasses, it seems that the BM affects prevalently the relative ratio between the three monolignols constituting the biopolymer. Additionally, from the direct comparison between the lignin extracted from the two sources, it is possible to identify two main differences: (i) in the W_L and W_BM_L samples the signal at 1270 cm−1 of the G unit is more intense compared to the pistachio shell lignin, in which the signal at 1120 cm−1 of the syringyl ring breathing[Citation51] is particularly intense; (ii) a higher signal associated to the stretching of -OH can be detected in the case of walnut shell lignin. Finally, in all samples can be found the signals at 1665 cm−1 and 1730cm−1 attributed to the stretching of conjugated carbonyl groups and aliphatic acetate, respectively.[Citation51] More detailed structural information was obtained from 2D-HSQC NMR spectroscopy. In (a) the HSQC spectra of the four lignin samples in the aromatic region (δC = 100–130 ppm, δH = 6.0–7.8 ppm) are reported. Signals were assigned based on previous reports[Citation21,Citation22,Citation57–60] while the quantitative analysis was calculated by integrating the contour signals.[Citation21,Citation27] In the case of walnut shells, the BM does not affect the condensation of the biopolymer, indeed in both W_L and W_BM_L samples it is not possible to recognize the signal of the Scond units ((a)). For W_BM_L, in accordance with the ATR-FTIR analysis, the mechanical pretreatment increased the relative abundance of S units decreasing the G and H ones. In general, the walnut shell lignin exhibits a S/G ratio of about 1, which makes it comparable to softwood lignin.[Citation61] P_L and P_BM_L completely differ from the walnut shell samples. Also in this case, HSQC confirmed the ATR-IR analysis since the pistachio shell lignin is mainly constituted by S units, with a low content of G units and no H ones, as in the case of hardwood lignins.[Citation62] For the sample P_L, S units represent the 86% of the total. This is one of the highest values reported in literature. This value decreases to 79% in the case of P_BM_L. Interestingly, in both pistachio shell samples the signal of Scond units can be found even though it is twice more intense for P_BM_L. This evidence confirms the oxidative behavior of the BM pretreatment on the lignin and the inability of pistachio extracts to neutralize it. Moreover, the abundance of S units (electron-rich rings) makes pistachio shell lignin more prone to aromatic electrophilic substitutions (i.e. condensation). From the data analysis in (b), it can be seen how the mild ethanolsolv fractionation can preserve the β-O-4 motif since in all samples the total amount of this linkage is around the 60% C9 units. P_BM_L was the sample in which the α-ethoxylation reaction resulted less effective. For this reason, a higher amount of deuterated water was needed to completely dissolve the sample for the NMR characterization. This is an important aspect because poor solubility in organic solvents can hinder the development of an effective valorization strategy for the lignin fractions. The extremely low amount of G units in P_L and P_BM_L led to a more regular structure,[Citation61] without the β−5 motif, but with a higher content of β-β linkages. The signal at δC/δH = 84.8/5.26 ppm was attributed to the presence of the α-carbonyl group in the β-O-4 motif (β’’-O-4 in (b)), in fact the content of S’ units resulted higher in P_L ((a)). The β−5 motif can be found in the walnut shell lignin because of the higher concentration of G units. In fact, the sample W_L (the richer in G units) showed the highest content of β−5 linkers.
Figure 4. (a) 2D-HSQC spectra in d6-acetone of the lignin in the aromatic region (δC = 100–130 ppm, δH = 6.0–7.8 ppm) and quantification of S, G and H units in the four samples. (b) 2D-HSQC spectra in d6-acetone of the lignin in the aliphatic region (δC = 50–95 ppm, δH = 3.0–5.7 ppm) and quantification of linking motifs in the four samples.
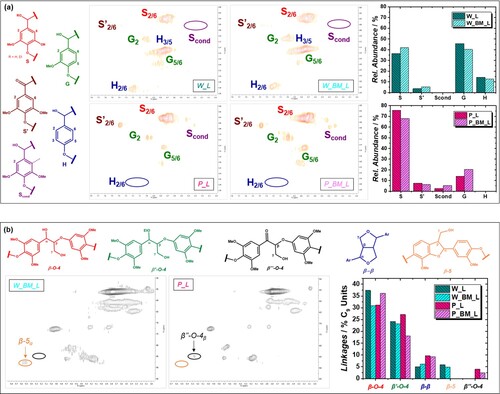
Results obtained from the structural characterization of the lignin samples are reported in , and can be summarized as follows: (i) pistachio shells are a better feedstock compared with walnut ones since they can be efficiently delignified using mild ethanosolv fractionation processes without needing a drastic mechanical size reduction of the granulometry; (ii) the BM has a slight positive impact on the ethanolsolv fractionation of walnut shell, inducing a reduction of the lignin molecular weight which positively affects the lignin extraction; (iii) the high content of extractives with a remarkable RSA activity which are contained into the walnut shells, together with the low content of S units, prevent the lignin condensation induced by the oxidative stress exerted by the BM pretreatment, even though the sample W_BM_L shows a lower retention of the β-O-4 motif and an increased S/G ratio; (iv) the total amount of extractives in pistachio shells, and their RSA, are not sufficient to prevent the oxidative degradation of lignin during the BM, hence the pretreatment results detrimental for this biomass.
Conclusions
In conclusion, the effects induced by a mild BM pretreatment on the ethanosolv fractionation process from walnut and pistachio dried shells are described. These two starting biomasses possess a unique tissue structure that is responsible for their exceptional mechanical properties. In the case of the walnut shell, the BM slightly improved the LFY while for the pistachio shells it resulted detrimental, due to the condensation of the aromatic biopolymer. Based on the extractive fractions and lignin characterization, this behavior was attributed to two main reasons: the extractives contained in the pistachio shells are at a low concentration and exhibit a poor RSA, unable to protect the biomass from the oxidative stress associated to the BM pretreatment; the pistachio shell lignin is mainly constituted by S units, that are more prone to give condensation reactions. In addition, the higher condensation of P_BM_L determined a lower yield of α-ethoxylation reaction, with consequent lower solubility in organic solvents. Interestingly, contrarily to walnut shells, pistachio shells can be efficiently delignified using mild ethanolsolv processes without the necessity for a drastic mechanical size reduction of the biomass. This behavior was explained considered the different distribution of lignin in the two tissues. Hence, with a low-cost approach, it was possible to separate the lignin fraction from the cellulosic pulp, opening the way for the development of an economic-sustainable way for the valorization of the whole biomass. In fact, it was demonstrated for the first time that pistachio shell lignin shows a highly regular structure, like in the case of hardwood lignins, caused by the extremely high content of S units (86%). This aspect, together with the high retention of β-O-4 motif (> 60% C9 units), make this lignin particularly suited for the production of high-value monomers via reductive convergent depolymerization and for the design of new materials.[Citation63] This work demonstrated how the opportune choice of the starting feedstocks for lignin-first studies, can avoid the implementation of useless and expensive pretreatment, leading to lignin fractions with superior properties and increasing the economic sustainability of the biomass valorization process.
Acknowledgement
DB gratefully acknowledges the REFIN (Return for Future Innovation) action for funding, an initiative co-funded by European Union through the POR Puglia 2014–2020 (ID grant 2455F798). DM gratefully acknowledges the PON-RI Industrial Chemical and Molecular Sciences PhD XXXVII cycle action for funding, an initiative co-founded by European Union through the PON 2022–2025 for Green and Innovation topics (ID grant 634338).
Disclosure statement
No potential conflict of interest was reported by the author(s).
Additional information
Funding
References
- Agbor, V.B.; Cicek, N.; Sparling, R.; Berlin, A.; Levin, D.B. Biotechnol. Adv. 2011, 29, 675–685.
- Manzanares, P. Acta Innov. 2020, 1, 47–56.
- Wenger, J.; Stern, T. Biofuels, Bioprod. Biorefin. 2019, 13, 1347–1364.
- Lynd, L.R. Nat. Biotechnol. 2017, 35, 912–915.
- Ponnusamy, V.K.; Nguyen, D.D.; Dharmaraja, J.; Shobana, S.; Banu, J.R.; Saratale, R.G.; Chang, S.W.; Kumar, G. Bioresour. Technol. 2019, 271, 462–472.
- Gillet, S.; Aguedo, M.; Petitjean, L.; Morais, A.R.C.; da Costa Lopes, A.M.; Łukasik, R.M.; Anastas, P.T. Green Chem. 2017, 19, 4200–4233.
- Liu, L.; Solin, N.; Inganäs, O. Adv. Energy Mater. 2021, 11, 1–12.
- Wu, X.; Jiang, J.; Wang, C.; Liu, J.; Pu, Y.; Ragauskas, A.; Li, S.; Yang, B. Biofuels, Bioprod. Biorefin. 2020, 14, 650–672.
- Chaleawlert-umpon, S.; Berthold, T.; Wang, X.; Antonietti, M.; Liedel, C. Adv. Mater. Interfaces 2017, 4, 1–7.
- Gan, D.; Xing, W.; Jiang, L.; Fang, J.; Zhao, C.; Ren, F.; Fang, L.; Wang, K.; Lu, X. Nat. Commun. 2019, 10, 1–10.
- Dababi, I.; Gimello, O.; Elaloui, E.; Quignard, F.; Brosse, N. Polymers (Basel) 2016, 8, 340.
- Sivasankarapillai, G.; Eslami, E.; Laborie, M.P. ACS Sustain. Chem. Eng. 2019, 7, 12817–12824.
- Parit, M.; Jiang, Z. Int. J. Biol. Macromol. 2020, 165, 3180–3197.
- Tran, M.H.; Phan, D.-P.; Lee, E.Y. Green Chem. 2021, 23, 4633–4646.
- Delmas, M. Chem. Eng. Technol. 2008, 31, 792–797.
- Huang, K.; Fasahati, P.; Maravelias, C.T. iScience 2020, 23, 100751.
- Feghali, E.; Carrot, G.; Thuéry, P.; Genre, C.; Cantat, T. Energy Environ. Sci. 2015, 8, 2734–2743.
- Korányi, T.I.; Fridrich, B.; Pineda, A.; Barta, K. Molecules 2020, 25, 2815.
- Chio, C.; Sain, M.; Qin, W. Renew. Sustain. Energy Rev 2019, 107, 232–249.
- Lancefield, C.S.; Panovic, I.; Deuss, P.J.; Barta, K.; Westwood, N.J. Green Chem. 2017, 19, 202–214.
- Zijlstra, D.S.; Lahive, C.W.; Analbers, C.A.; Figueirêdo, M.B.; Wang, Z.; Lancefield, C.S.; Deuss, P.J. ACS. Sustain. Chem. Eng. 2020, 8, 5119–5131.
- Nishide, R.N.; Truong, J.H.; Abu-Omar, M.M. ACS Omega 2021, 6, 8142–8150.
- Cederholm, L.; Xu, Y.; Tagami, A.; Sevastyanova, O.; Odelius, K.; Hakkarainen, M. Ind. Crops Prod. 2020, 145, 112152.
- Florian, T.D.M.; Villani, N.; Aguedo, M.; Jacquet, N.; Thomas, H.G.; Gerin, P.; Magali, D.; Richel, A. Ind. Crops Prod. 2019, 132, 269–274.
- Bauer, S.; Sorek, H.; Mitchell, V.D.; Ibáñez, A.B.; Wemmer, D.E. J. Agric. Food Chem. 2012, 60, 8203–8212.
- Hernández-Beltrán, J.U.; Hernández-De Lira, I.O.; Cruz-Santos, M.M.; Saucedo-Luevanos, A.; Hernández-Terán, F.; Balagurusamy, N. Appl. Sci.
- Zijlstra, D.S.; De Santi, A.; Oldenburger, B.; De Vries, J.; Barta, K.; Deuss, P.J. J. Vis. Exp. 2019,, 2019, 1–12.
- Lan, W.; Amiri, M.T.; Hunston, C.M.; Luterbacher, J.S. Angew. Chemie 2018, 130, 1370–1374.
- Li, W.; Amos, K.; Li, M.; Pu, Y.; DeBolt, S.; Ragauskas, A.J.; Shi, J. Biotechnol. Biofuels 2018, 11, 1–14.
- Barakat, A.; Mayer-Laigle, C.; Solhy, A.; Arancon, R.A.D.; De Vries, H.; Luque, R. RSC Adv. 2014, 4, 48109–48127.
- Sapouna, I.; Lawoko, M. Green Chem. 2021, 23, 3348–3364.
- Crestini, C.; Melone, F.; Sette, M.; Saladino, R. Biomacromolecules 2011, 12, 3928–3935.
- Balakshin, M.; Capanema, E.A.; Zhu, X.; Sulaeva, I.; Potthast, A.; Rosenau, T.; Rojas, O.J. Green Chem. 2020, 22, 3985–4001.
- Fujimoto, A.; Matsumoto, Y.; Chang, H.M.; Meshitsuka, G. Journal of Wood Science 2005, 51, 89–91.
- Karinkanta, P.; Ämmälä, A.; Illikainen, M.; Niinimäki, J. Biomass Bioenergy 2018, 113, 31–44.
- Antreich, S.J.; Xiao, N.; Huss, J.C.; Horbelt, N.; Eder, M.; Weinkamer, R.; Gierlinger, N. Adv. Sci. 2019, 6, 1–6.
- Huss, J.C.; Antreich, S.J.; Bachmayr, J.; Xiao, N.; Eder, M.; Konnerth, J.; Gierlinger, N. Adv. Mater. 2020, 32, 1–7.
- Xiao, N.; Felhofer, M.; Antreich, S.J.; Huss, J.C.; Mayer, K.; Singh, A.; Bock, P.; Gierlinger, N. R. Soc. Open Sci. 2021, 8, 210399.
- Rodríguez-Padrón, D.; Zhao, D.; Garín Ortega, R.N.; Len, C.; Balu, A.M.; García, A.; Luque, R. ACS Sustain. Chem. Eng. 2020, 8, 1513–1519.
- Sagar, N.A.; Pareek, S.; Sharma, S.; Yahia, E.M.; Lobo, M.G. Compr. Rev. Food Sci. Food Saf. 2018, 17, 512–531.
- Amendola, D.; De Faveri, D.M.; Spigno, G. J. Food Eng. 2010, 97, 384–392.
- García, A.; González Alriols, M.; Spigno, G.; Labidi, J. Biochem. Eng. J. 2012, 67, 173–185.
- Aresta, A.; Calvano, C.D.; Trapani, A.; Cellamare, S.; Zambonin, C.G.; De Giglio, E. J. Nanopart. Res. 2013, 15, 662–673.
- Yeganeh, M.M.; Kaghazchi, T.; Soleimani, M. Chem. Eng. Technol. 2006, 29, 1247–1251.
- Hesam, F.; Tarzi, B.G.; Honarvar, M.; Jahadi, M. Journal of Food Measurement and Characterization 2021, 15, 33–45.
- Movva, M.; Kommineni, R. Mater. Res. Express 2017, 4, 045014.
- Özbek, H.N.; Fockink, D.H.; Yanık, D.K.; Göğüş, F.; Lukasik, R. J. Cleaner Prod. 2018, 196, 842–851.
- Kasiri, N.; Fathi, M. Int. J. Biol. Macromol. 2018, 106, 1023–1031.
- Göncü, B.; Gülşen, H. Energy Sources, Part A Recover. Util. Environ. Eff. 2021, 43, 1444–1455.
- Göncü, B.; Gülşen, H.; Hoşgün, E.Z. Environ. Technol. 2021, 42, 2438–2446.
- El Hage, R.; Brosse, N.; Chrusciel, L.; Sanchez, C.; Sannigrahi, P.; Ragauskas, A. Polym. Degrad. Stab. 2009, 94, 1632–1638.
- Queirós, C.S.G.P.; Cardoso, S.; Lourenço, A.; Ferreira, J.; Miranda, I.; Lourenço, M.J.V.; Pereira, H. Biomass Convers. Biorefinery 2020, 10, 175–188.
- Gentile, C.; Tesoriere, L.; Butera, D.; Fazzari, M.; Monastero, M.; Allegra, M.; Livrea, M.A. J. Agric. Food Chem. 2007, 55, 643–648.
- Zhou, S.; Liu, L.; Wang, B.; Xu, F.; Sun, R. Process Biochem. 2012, 47, 1799–1806.
- Lu, Y.; Lu, Y.C.; Hu, H.Q.; Xie, F.J.; Wei, X.Y.; Fan, X. J. Spectrosc. 2017.
- Stark, N.M.; Yelle, D.J.; Agarwal, U.P. Lignin in Polymer Composites 2016, 49–66.
- Su, S.; Wang, S.; Song, G. Green Chem. 2021, 23, 7235–7242.
- Wang, X.; Guo, Y.; Zhou, J.; Sun, G. RSC Adv. 2017, 7, 8314–8322.
- Kim, H.; Li, Q.; Karlen, S.D.; Smith, R.A.; Shi, R.; Liu, J.; Yang, C.; Tunlaya-Anukit, S.; Wang, J.P.; Chang, H.M.; Sederoff, R.R.; Ralph, J.; Chiang, V.L. ACS Sustain. Chem. Eng. 2020, 8, 3644–3654.
- Lancefield, C.S.; Rashid, G.M.M.; Bouxin, F.; Wasak, A.; Tu, W.C.; Hallett, J.; Zein, S.; Rodríguez, J.; Jackson, S.D.; Westwood, N.J.; Bugg, T.D.H. ACS Sustain. Chem. Eng. 2016, 4, 6921–6930.
- Nordström, Y.; Norberg, I.; Sjöholm, E.; Drougge, R. J. Appl. Polym. Sci. 2013, 129, 1274–1279.
- Akiyama, T.; Goto, H.; Nawawi, D.S.; Syafii, W.; Matsumoto, Y.; Meshitsuka, G. Holzforschung 2005, 59, 276–281.
- Jardim, J.M.; Hart, P.W.; Lucia, L.; Jameel, H. Polymers (Basel) 2020, 12, 1795.