Abstract
The physical and chemical integrity of a biopharmaceutical must be maintained not only during long-term storage but also during administration. Specifically for the intravenous (i.v.) delivery of a protein drug, loss of stability can occur when the protein formulation is compounded with i.v. bag diluents, thus modifying the original composition of the drug product. Here we present the challenges associated with the delivery of a low-dose, highly potent monoclonal antibody (mAb) via the i.v. route. Through parallel in-use stability studies and conventional formulation development, a drug product was developed in which adsorptive losses and critical oxidative degradation pathways were effectively controlled. This development approach enabled the i.v. administration of clinical doses in the range of 0.1 to 0.5 mg total protein, while ensuring liquid drug product storage stability under refrigerated conditions.
Abbreviations
i.v. | = | intravenous |
mAb | = | monoclonal antibody |
CD3 | = | cluster of differentiation 3 |
PO | = | polyolefin |
PVC | = | polyvinylchloride |
PES | = | polyethersulfone |
PS80 | = | polysorbate 80 |
Met | = | methionine |
Cys | = | cysteine |
Glut | = | glutathione |
MTG | = | monothioglycerol |
EDTA | = | edetate disodium |
M | = | methionine residues |
MS | = | mass spectrometry |
SDS-PAGE | = | sodium dodecyl sulfate polyacrylamide gel electrophoresis |
HPLC | = | high performance liquid chromatography |
cIEF | = | capillary isoelectric focusing |
DSC | = | differential scanning calorimetry |
CMC | = | critical micelle concentration |
Introduction
While some biopharmaceuticals are formulated and provided ready for clinical administration without further manipulation, many such products require varying degrees of handling by healthcare professionals.Citation1 During handling and administration, the physical and chemical stability of the protein drug must be maintained. Loss of stability can occur when the protein formulation is diluted to low concentrations with intravenous (i.v.) solutions, which lowers the excipient concentrations and modifies the composition and properties of the original drug product formulation. When delivering a biopharmaceutical product via the i.v. route, several factors such as protein properties, formulation composition, concentration of the active pharmaceutical ingredient, choice of diluent, product contact surfaces, and infusion time and rate must be considered.Citation1,2 The final critical factors assessed are environmental effects (e.g., area lighting and temperatures). The contact surface is of particular interest because proteins tend to adsorb at interfaces due to their amphiphilic nature.Citation3 With the widespread use of a variety of plastic polymers in syringes and i.v. infusion containers and lines, the risk of protein loss by adsorption is substantial, especially at low concentrations (<0.5 mg/mL). For example, previous reports have indicated that, due to adsorption onto the product contact materials, protein recovery could decrease by up to 40% at concentrations as low as 0.01 mg/mL when diluted in either isotonic 0.9% sodium chloride (normal saline) or isotonic 5% dextrose in i.v. containers.Citation4-6 Significant adsorption was also observed during administration of undiluted protein drug products.Citation7,8 Current pharmaceutical regulations require that i.v.-delivered medications be consistently within 10% of the nominal concentration.Citation9-11 Thus, protein loss due to adsorption onto containers, filters, and tubing used for administration must be investigated and addressed in parallel with the formulation development efforts, particularly for low-dose products.
There is a complex array of devices and components to deliver medicinal product to the patient during clinical trials. Before such trials can be initiated, the drug product development group typically conducts in-use stability studies mimicking the dosing methodology and using the type of components that are intended for drug administration. In-use stability studies for clinical trials, also referred to as compatibility, practical stability, or post-dilution studies, are critical studies conducted as a means to confirm maintenance of protein quality and quantity, thus ensuring that handling results in a diluted product that is both safe and efficacious.Citation12-17 Of particular importance to the clinic is confirmation that the subjects consistently receive the desired dose (i.e., no underdose or variable dose).
One of the major classes of therapeutic proteins is monoclonal antibodies (mAbs), which are commonly used to treat cancer and immunological disorders.Citation18,19 As of early 2015, nearly 50 mAb products were approved or were under regulatory review in either the US or EU, and others are being developed for various indications.Citation20 Although the typical pharmacological doses of mAbs comprise tens or hundreds of mg, some therapeutic agents require substantially lower doses.Citation21,22 Intravenous administration of low-dose mAbs may involve compounding of very dilute solutions of active pharmaceutical ingredient in normal saline or other diluents prior to infusion.Citation23 For example, low doses (0.04 mg/mL) of oregovomab were administered by infusion in Phase 3 trials.Citation21 As discussed above, the diluted low concentration mAbs are susceptible to losses via adsorption onto various contact surfaces, and the adsorption risk needs to be evaluated via in-use stability studies.
Otelixizumab is a humanized mAb (IgG1) directed against human CD3. Its N-linked glycosylation site in the Fc domain was eliminated via amino acid mutation.Citation24,25 To facilitate the accurate preparation and administration of the clinical doses (0.1 to 0.5 mg per dose), the drug product was developed at a concentration of 0.2 mg/mL. The dose administration required dilution of the mAb in an i.v. bag containing normal saline and delivery of the entire bag contents via infusion pump. This dosing methodology led to protein concentrations in the i.v. bag as low as 0.002 mg/mL. As a direct consequence, the risk of significant protein loss on the administration set components was very high. For this reason, in-use stability and compatibility studies with commercially relevant i.v. giving sets were performed to assess the feasibility of administering the product, as well as to develop an optimized formulation that would support the proposed dilution and administration design intent. Here we present the challenges associated with the safe administration of the entire low-dose, potent therapeutic mAb and describe the formulation design to stabilize otelixizumab against both physical degradation during i.v. administration and chemical degradation during long-term storage of the clinical product.
Results
Assessment of otelixizumab loss during in-use stability studies
An initial stability study (in-use stability study 1) was conducted to assess the feasibility of administering the 0.2 mg/mL early phase formulation (histidine-based buffer containing no excipients) using commercially available i.v. giving sets. These studies were performed by testing the proposed clinical trial administration procedure, as described in the Materials and Methods section. A drug product volume of 0.5 mL (equivalent to the target dose of 0.1 mg) was added to an i.v. bag containing normal saline, and the stability of the diluted product was assessed. Volumes of either 25 or 50 mL (rather than ≥100 mL) were selected to maximize protein concentration in the bag and minimize contact surface area, thus minimizing potential protein losses due to adsorption. The resulting active concentrations in the i.v. bags were 0.004 and 0.002 mg/mL, respectively. In addition, i.v. bags constructed of 2 different types of materials (polyolefin (PO) and polyvinylchloride (PVC)) were evaluated. First, the diluted samples were held in the i.v. infusion bag for a period of time not less than that specified in the clinical trial procedure and samples were collected directly from the infusion bag. Second, the diluted drug was passed through an infusion set with an in-line filter (low PVC content i.v. set with 0.2 μm polyethersulfone (PES) filter) and samples were collected at the end of the infusion line. There was good agreement between measurements conducted on replicate bags prepared by different scientists. Percent (%) average recovery results, as measured via HPLC analysis, are shown in . The in-use recovery criterion for the measured dose was ± 10% of the nominal dose. The recovery at 0.004 mg/mL mAb concentration in the bag was better than at 0.002 mg/mL. The mAb recoveries from all PO bags (range of 23% to 88%) trended lower than those from PVC bags (range of 41% to 98%). Overall, the losses in all test systems after room temperature storage for 2 hours were well above 10%. Furthermore, greater than 50% of the protein was lost when the product was infused through an i.v. line and filter, regardless of bag material. Although not shown here, it is important to note that other critical quality attributes were assessed using various qualified and stability-indicating analytical methods, and the results indicated no other significant changes in quality of the diluted product.
Optimization of surfactant concentration in i.v. bags and vials
Due to unacceptable protein loss upon administration of the mAb formulated in histidine buffer alone, a second study (in-use stability study 2) was designed to determine whether incorporating the surfactant polysorbate 80 (PS80) into the formulation could enable the delivery of ≥90% of the dose. The preparation and infusion of 0.1 mg protein were tested using a PO bag containing 50 mL saline (0.002 mg/mL mAb) in combination with an i.v. line with an in-line filter. Only the PO bag was studied as the initial results showed lower recoveries in PO bags compared to those in PVC bags; thus, the PO bag represented the more difficult hurdle to achieving acceptable protein recovery. Samples were prepared such that a broad range (0.0002 to 0.003%) of PS80 concentrations was achieved in the i.v. bag following product dilution. There was good agreement between measurements conducted on replicate bags prepared by different scientists. The average percentage mAb recoveries, as determined via HPLC analysis, are shown in .
Figure 2. Plot representations of otelixizumab recovery data obtained from the in-use stability study 2 in the presence of various concentrations of PS80 (the i.v. bag data was best fitted with a linear function; for the i.v. infusion, results the best fit was obtained when an exponential function was used).
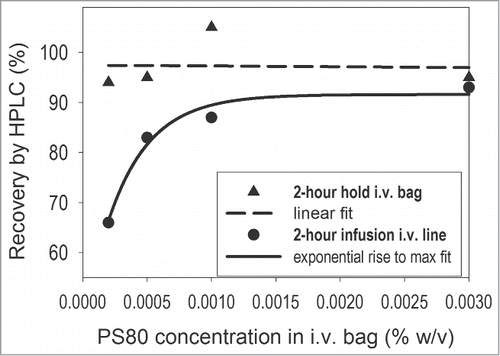
Depending on the test condition, the measured recovery values ranged from 66% to 105%. Approximately 95% protein recoveries at all test PS80 concentrations were observed in the i.v. bags during the 2-hour hold. Thus, PS80 concentrations as low as 0.0002% in the bag (equivalent to 0.02% in the drug product formulation) were sufficient to inhibit adsorption-mediated mAb loss during the first step of clinical product handling. However, there was an additional, significant loss to the i.v. line and filter. The dependency of total protein recovery on surfactant concentration appeared to be non-linear, with close to 90% recovery attained at PS80 concentrations at or higher than 0.001% in a 50 mL i.v. bag. The observed non-linearity of the protein recovery may be associated with the critical micelle concentration (CMC) of PS80 in the diluted system since it is close to the reported CMC of PS80 in water (0.0017% w/v). Although the actual CMC was not measured in the diluted system, these data suggest that the recovery of the mAb from this particular clinical giving set can be maximized by selecting a PS80 concentration in the product formulation that is close to the CMC upon final dilution in the i.v. bag. As a result, 0.1% PS80 was the surfactant concentration selected for the product formulation.
Surfactant effect on otelixizumab structure via DSC
Differential scanning calorimetry (DSC) can be used to measure the thermodynamic parameters of protein unfolding, including the midpoint unfolding temperature (Tm). Tm is commonly used to compare protein stability in different formulations.Citation26 Tm values for otelixizumab in the presence and absence of 0.1% PS80 were determined from DSC thermograms (). There was a high level of similarity between DSC scans with and without PS80, with a consistent unfolding profile; this was indicative of no changes in tertiary fold or thermodynamic populations as a function of surfactant. At least 2 species/domains, corresponding to 2 unfolding transitions, could be clearly identified. The integrated DSC results for the mAb thermal stability in the absence and presence of PS80 showed no significant differences between both Tm1 (63.1 versus 62.9°C) and Tm2 (80.6 vs. 80.7°C). In addition, PS80 had no effect on the thermal stability of the mAb, as indicated by a comparison of other relevant DSC-derived thermodynamic parameters such as T½ (width of the transition at half height of the peak, with values of 5.3°C in the absence and presence of PS80) and total enthalpy of unfolding (759 kcal/mol for the mAb in buffer alone versus 802 kcal/mol for the sample containing 0.1% PS80).
Effect of oxidation on otelixizumab structure via DSC
Peroxides can be present in significant quantities in raw materials such as polysorbates.Citation27 The effect of residual peroxides needs to be evaluated because they can cause oxidative damage to mAb that could have biological consequences (e.g., different pharmacokinetics, efficacy, and immunogenicity).Citation28 To assess the risk of whether oxidation is a significant degradation pathway for the studied mAb, otelixizumab in histidine buffer alone (i.e., containing no PS80) was oxidized by treatment with hydrogen peroxide, and the oxidation-induced structural changes were evaluated by DSC and compared to a non-oxidized control. The scans overlay is shown in . Tm analysis showed an oxidation-induced destabilization of the first unfolding transition, typically assigned to the CH2 domain of the mAb.Citation29,30 There was a significant decrease (by 8.2°C, from 63.7°C to 55.5°C) in Tm1 (the low-temperature transition) concomitant with peak broadening. The second transition (i.e., Tm2) was not affected by oxidation. Mass spectrometry (MS) analysis correlated with DSC findings and confirmed that one exposed methionine residue in the CH2 domain was 90% oxidized. These results demonstrate that the major oxidation site is the methionine residue in the CH2 domain and that the oxidation event correlates with thermodynamic destabilization of this domain.
Figure 4. DSC detects significant oxidation-induced structural changes in otelixizumab. The PS80-free mAb sample was oxidized with hydrogen peroxide at 10,000:1 molar ratio at room temperature for 1 hour. The peroxide was subsequently removed using a desalting column. DSC scans were collected on both the peroxide-treated sample and peroxide-free control and the Tm values for the 2 unfolding transitions determined.
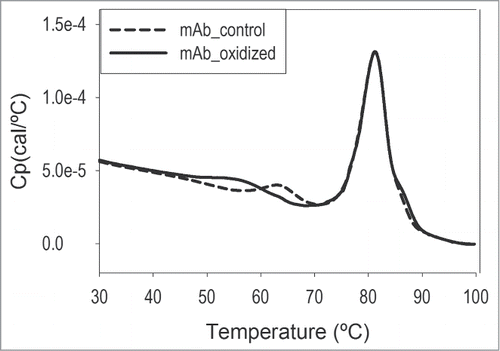
Effect of PS80 on otelixizumab stability in vials and selection of antioxidants
Protein stability under accelerated storage conditions has conventionally been assessed during biopharmaceutical product development to aid in identifying an optimum formulation for long-term storage and to enable a prediction of product shelf life under proposed storage conditions.Citation31 Here, a short-term (2-week) accelerated stability study was conducted at 40°C to evaluate the stability of the 0.1% PS80-containing formulation, specifically to assess the risk that PS80 (or impurities and degradation products) may adversely affect the stability of the mAb via oxidative degradation during long-term storage.
Oxidation of the methionine residues leads to the formation of methionine sulfoxide and a mass shift of 16 Da, which can be detected using MS. Thus, MS was used to compare relative amounts of oxidized and unoxidized peptides. A MS peptide map analysis of various samples (35 samples, one preparation per sample) displayed a mean baseline level of oxidation at one methionine residue of 2.9% with a standard deviation of 0.5%, thus the method variability was estimated at ±1.5% (3 standard deviations). For the mAb control sample (in buffer with no excipients), the measured percentage oxidation at the tested methionine residues was as high as 11% (). When the surfactant was present in the formulation, a significantly increased level of mAb oxidation (up to 30%) at the 2 most exposed methionine residues (M254 and M430) was observed. This increase is predictive of decreased long-term stability, as oxidation can trigger further degradation events such as aggregation.Citation28,32 Thus, MS emerged as a critical stability-indicating assay for the evaluation of oxidative degradation pathways.
Table 1. Stability of 0.2 mg/mL otelixizumab formulated with 0.1% PS80 and various antioxidants: results of MS and cIEF analysis following storage in vials for 2 weeks at 2–8°C and 40°C
Two other non-ionic surfactants, polysorbate 20 (PS20) and poloxamer 188 (PX188), were investigated as potential alternative surfactants for formulation development. These results showed that the level of mAb oxidation in the presence of PS20 was significantly higher than in the presence of PS80 and PX188. In addition, samples containing PS20 showed significant chemical instability of the mAb, as confirmed by capillary isoelectric focusing (cIEF), sodium dodecyl sulfate polyacrylamide gel electrophoresis (SDS-PAGE), and MS analysis (data not shown). Based on these results, and given the acceptability of PS80 for use in commercialized mAb parenteral products (e.g., Humira at 0.1% PS80, and MabThera at 0.07% PS80), 0.1% PS80 was selected for drug product formulation.
Additional work was conducted to screen excipients that improve the chemical stability of the mAb in the proposed formulation containing 0.1% PS80. Five different antioxidants, including free radical scavengers and a metal chelator, were tested at a concentration of either 1 mM (metal chelator) or 5 mM (all other). The screened antioxidants were methionine (Met), cysteine (Cys), glutathione (Glut), monothioglycerol (MTG), and edetate disodium (EDTA). All antioxidants were able to attenuate or entirely control the surfactant-related oxidative effects (). The MS data show the lowest extent of oxidation in the formulation containing Met. Although EDTA was less effective than Met at controlling oxidation, in these studies it significantly reduced the extent of PS80-dependent oxidation by almost 4-fold.
The results of charge analysis via cIEF analysis are shown in . The largest values for % Main peak product were observed for the control formulation (buffer only) and for the PS80-containing formulations that included either Met or EDTA. The cIEF results indicated that the least stable PS80-containing formulations included Cys, Glut, or MTG. SDS-PAGE was performed to help explain the discrepancies observed between the MS and cIEF results (i.e., by MS, all antioxidants controlled oxidation, but, by cIEF, antioxidant formulations presented different stability profiles regardless of storage temperatures). The SDS-PAGE results for the formulations containing Cys, Glut, or MTG showed a significant change in molecular weight of product with an associated shift of bands in the non-reduced gel for the samples stored at 40°C for 2 weeks (), indicative of chemical degradation via disulfide shuffling. A similar SDS-PAGE profile was observed for samples stored at 2–8°C for 2 weeks (data not shown), in line with the corresponding cIEF results for this storage condition. The disulfide instability of the mAb in the presence of Cys, Glut, or MTG, as detected by SDS-PAGE, correlated with the increase in the acidic peaks by cIEF (). Hence, while these antioxidants were able to protect against PS80-related oxidation, they activated disulfide shuffling mechanisms regardless of storage temperature, which rendered them unsuitable for use in combination with this mAb. Met and EDTA were the only antioxidants tested that imparted no disruption of the disulfide bond pattern in the product and were confirmed to have optimum antioxidant activity. The overall product stability in formulations containing antioxidants can be ranked as Met >EDTA>>Cys, Glut, MTG. Based on the results both Met and EDTA were incorporated in the PS80-containing product formulation.
Long-term stability of the reformulated product and identification of functional antioxidant levels
A development stability study was performed to determine the optimal solution composition and to assess the long-term and accelerated stability of the 0.2 mg/mL mAb drug product formulation containing PS80, Met, and EDTA. The EDTA level was selected based on previous mAb formulation experience. The stability of 8 formulations () was assessed. Formulations A – F incorporated Met at 0, 5, 10, 15, 20, and 40 mM with a fixed level of EDTA (0.05 mM) and PS80 (0.1%). Formulations G and H served as PS80 (only) and buffer alone (no excipients) controls, respectively. Extensive bioanalytical and biophysical testing was performed; however, only oxidation-relevant results are included and discussed here.
Table 2. Experimental setup of formulation development stability study for otelixizumab. The formulation composition pertaining to each formula code is detailed
The oxidization levels of methionine residues for initial and 6-month stability samples containing different levels of surfactant, antioxidant, and metal chelator are presented in . Representative oxidation trends for samples C (PS80, Met, and EDTA), G (PS80 only), and H (no excipients) stored at 2–8°C and 25°C for 6 months are shown in . Oxidation at the most exposed methionine residue, M254, was initially observed in all samples. Interestingly, differences between samples were present at initial analysis. Samples G and H showed ∼3% oxidation at the second most exposed methionine residue M430; for samples A through F, which contained at least one antioxidant (Met), the oxidation levels were below the level of quantification. Thus, these data may not only be representative of stability upon storage, but also may be reflective of the degradation during sample preparation and analysis in the absence of antioxidants.
Figure 6. Oxidation trends for otelixizumab stability samples C (containing a surfactant and a combination of 2 antioxidants), G (containing a surfactant only), and H (no excipient control), stored at 2–8°C and 25°C for up to 6 months. For illustration purposes, the data were fitted to a linear regression except for sample G at 25°C. The formulation composition pertaining to each formula code is described in detail in .
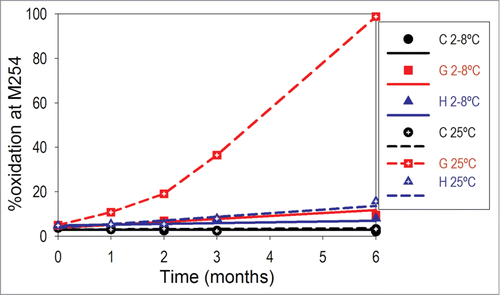
Table 3. Results of peptide mapping by MS analysis reported for various methionine residues in otelixizumab stability samples A-H, containing different levels of surfactant, antioxidant, and metal chelator, and following storage for 6 months at various storage temperatures. The formulation composition pertaining to each formula code is described in detail in
MS analysis of sample A (containing EDTA without Met) assisted in differentiating various oxidation mechanisms. While oxidation levels in sample A (PS80 and EDTA) remained slightly lower than in the control sample H (buffer only) and much lower than in the control sample G (PS80), they were higher than those measured in samples B – F (containing PS80, Met and EDTA). In other words, the oxidation levels detected in the formulation H (buffer only) were significantly higher than in the samples containing the antioxidant combination (A – F). This comparison was of critical importance as it confirmed 3 previous stability risks: 1 - oxidation is a degradation pathway even in the absence of PS80; 2 - oxidation occurs at refrigerated storage and it is significantly accelerated at increased storage temperatures; and 3 - addition of PS80 accelerates the oxidative degradation pathway (through both of the peroxide- and metal- dependent pathways).
Data showed that oxidation reached the highest levels (>97%) in the PS80 control formulation G at both M254 and M430 when the product is stored at 25°C for 6 months. The protective effect of the combined addition of Met and EDTA was evident in formulations B – F containing both antioxidants. Specifically, over a 6-month period at 2–8°C and 25°C, oxidation at M254 remained low (≤4.1%), while little or no oxidation was observed at M430. The results indicate that a combination of 2 antioxidants, Met (a free radical scavenger) and EDTA (a metal chelator) prevents oxidation, and that the quenching mechanism is not temperature dependent. These data were compelling and pointed not only to the robustness of these formulations, but also to the effectiveness of the antioxidant combination.
Discussion
The current understanding of adsorption is not sufficiently comprehensive to allow for predictions of the amount of protein that may be adsorbed to clinical giving sets nor the potential effect on efficacy and safety.Citation2 For this reason, in-use stability studies must be conducted prior to administration of drug in a clinical setting to assess the stability of the diluted product, the compatibility of the product or dosing solution to clinically-relevant materials of construction, and the recovery and quality of active compound when prepared using the intended method for dose preparation and administration.Citation13-17
Otelixizumab drug product was formulated at a concentration of 0.2 mg/mL in a histidine-based buffer. Based on in-house experience and literature reports, an apparent administration risk, i.e., underdosing, was identified. In-use stability studies were conducted to assess the feasibility of i.v. administration of the drug product diluted to 0.002 mg/mL with normal saline. Overall, losses exceeded the maximum allowable 10%, likely due to active binding to the surfaces of the i.v. bag, tubing and in-line filter.
When adsorption is identified during in-use stability studies, the formulation scientist can resort to a number of stabilization approaches. Previous strategies reported to reduce protein loss via adsorption include the development of biomaterials with low adsorption properties.Citation33 The use of excipients such as human serum albumin, glycerol, trehalose or surfactants, as well as polymer carriers in protein product formulations has also been employed.Citation34-37 The excipients used to prevent or reduce adsorption are either more surface-active than the protein, thus preferentially adsorbing at interfaces, or act as competitive inhibitors of adsorption.Citation2 For example, the conventional surfactants used in protein formulations are typically small non-ionic surfactants that either occupy the interface by direct competition with the protein, form surfactant-protein complexes that are less surface-interactive and thus remain in solution, or a combination of both.Citation38
Non-ionic surfactants from the polysorbate class, such as PS80 (also known as Tween® 80), are common excipients used in formulations of therapeutic proteins. Typically, surfactants have been added to protect against mechanical stresses, namely freeze-thaw and shake.Citation39-41 In addition, surfactants have been successfully used to minimize adsorption to surfaces via competitive binding.Citation42,43 A review of the current landscape of mAb products marketed in the United States reveals that the majority contain polysorbates. Some specific examples of mAbs formulated with PS80 include adalimumab (Humira®), alemtuzumab (Campath®/Lemtrada™), rituximab (Rituxan®), and tocilizumab (Actemra®). Within mAb commercial preparations, the polysorbate concentrations range between 0.001% (w/v) PS80 (2 mg/mL abciximab, ReoPro®) and 0.22% (w/v) PS80 (10 mg/mL eculizumab, Soliris®), with most formulations containing between 0.005 to 0.02% polysorbate.
In-use stability studies indicated that the 0.2 mg/mL otelixizumab product formulated in histidine alone could not be administered via an i.v. infusion method because of unacceptable loss of mAb content after dilution to low concentration. To ensure that the entire dose could be administered to the patient, product development efforts were directed toward the identification of an otelixizumab formulation that would protect from adsorption losses at doses as low as 0.1 mg. A surfactant strategy was implemented for adsorption prevention studies. The data indicated that a formulation buffer incorporating PS80 at 0.1% would support the desired preparation and i.v. administration.
One issue previously identified in polysorbate-containing formulations is the potential for an adverse effect on protein stability during long-term storage.Citation44 Polysorbates can undergo various degradation reactions which can lead to not only loss of their stabilizing properties but also chemical modifications of proteins, such as oxidation.Citation45 Specifically, polysorbates undergo autooxidation that results in hydroperoxide formation, side-chain cleavage, and eventually formation of short chain acids such as formic acid, all of which having the potential to alter the stability of a biopharmaceutical product.Citation46 In addition, PS80 was previously found to contain significant concentrations of hydrogen peroxides with considerable lot-to-lot and manufacturer-to-manufacturer variabilities.Citation27 Awareness of and control against the effects of residual peroxides in surfactants are particularly important as these peroxides can potentially affect the chemical stability of oxidation-sensitive proteins, including mAbs, as previously shown.Citation44,45,47
Multiple degradation pathways exist for mAbs.Citation48 Many mAbs display undesirable biophysical properties such as the propensity to aggregate.Citation49 Chemical degradation occurring through various pathways, including deamidation, oxidation, and fragmentation, is also frequently observed. The methionine residue is one of the amino acids most susceptible to oxidation due to its thiol group reactivity and solvent exposure.Citation50 Methionine residues can be readily oxidized to sulfoxide derivatives, but under extreme conditions the sulfone can also be generated.Citation51 Oxidation at methionine residues has been extensively studied and was found to result in detectable changes in the secondary and tertiary structure of the mAb, which correlated with decreased thermodynamic stability and increased aggregation rates.Citation52 Of special interest are the methionine residues in the Fc region that are located on the protein surface, as their exposure to the solvent makes them particularly prone to oxidative degradation. In the 3-dimensional structure of a recombinant mAb, 2 methionine residues close to the CH2‒CH3 interface were found to be easily oxidized.Citation51,53 Changes in the oxidation status of a mAb can affect its biological function.Citation32 Previous studies have shown that peroxide-induced oxidation at methionine residues can significantly decrease the binding affinity of the human IgG1 to Fc receptors.Citation52 Similarly, the metal-catalyzed oxidation of IgG can alter its ability to bind to the Fc receptor.Citation54
Otelixizumab was the first aglycosylated mAb to enter clinical trials.Citation24 The molecule consists of a humanized heavy chain that has been modified to eliminate its single glycosylation site in the CH2 domain. The overall stability, and in particular the oxidation propensity of this mAb, is complicated by the lack of glycosylation. It was previously shown that the removal of oligosaccharides alters solubility and stability because the carbohydrates contribute to the dynamics and stability of the Fc domain.Citation32,55-57 In addition, oligosaccharide removal could cause structural changes that in turn accelerate the oxidative degradation of the antibody.Citation58 Otelixizumab contains 10 methionine residues, 4 on each heavy chain and one on each light chain. Of the 4 methionine residues located on the heavy chain, M254 and M430 are close to the CH2‒CH3 interface. In a typical glycosylated mAb, the oligosaccharides are in close proximity and shield the CH2 domain, providing some degree of stabilization to the mAb. Since glycosylation was missing in otelixizumab, the risk of oxidation during its manufacturing and storage was found to be high, and consequently carefully evaluated.
In agreement with previous reports in the literature, our DSC analysis confirmed that oxidation decreases the thermodynamic stability of otelixizumab, specifically the stability of the CH2 domain. In addition, formulation development studies with otelixizumab confirmed the known risk that PS80 can adversely affect the long-term stability of the mAb by accelerating the oxidative degradation pathways. Thus, it became critical to evaluate, monitor and control PS80-induced oxidation during manufacturing and storage of this mAb when formulated with PS80.
Oxidation is dependent on both protein and formulation properties. There are a number of different mechanisms by which oxidation can occur in proteins. Oxidation can be mediated by stresses such as light, or impurities such as trace metals and peroxides.Citation50 Protecting the product from light is a relatively straightforward and common strategy for photooxidation control. However, the detection and control of impurities are more difficult. Trace impurities such as heavy metals are especially problematic because they can be introduced during the manufacturing process by leaching or can be present at sufficient levels in compendia-grade raw materials to promote significant oxidation. Protection against metal-induced oxidation can be achieved by adding a chelating agent to the formulation. One example is EDTA, which binds to metal ions responsible for catalysis of oxidative degradation. However, non-ionic surfactants such as polysorbates are a class of excipients where the main impurities (peroxides) are more problematic and especially difficult to control. Although ultrapure, low-peroxide grade polysorbates are now available, their degradation may still require stringent control. To overcome the oxidation induced by the presence of polysorbate impurities, proteins can be formulated with sufficient amounts of antioxidants. Several antioxidants are currently used in pharmaceutical products, including chelating agents, reducing agents, oxygen scavengers, and chain terminators.Citation59 The most common antioxidants are the sacrificial reductants, or compounds that oxidize more readily than the protein, thereby reducing the concentration of oxidants in the protein solutions and protecting the protein from the oxidative degradation. Met is a sulfur-containing amino acid and a sacrificial antioxidant. It was previously observed that protein oxidation can be effectively inhibited by antioxidants like Met.Citation60-62
Since the stability of some proteins has been shown to be affected when formulated with certain antioxidants, the antioxidant should be carefully selected for each protein. We conducted additional work to screen excipients that would improve the chemical stability of the otelixizumab product formulated with PS80. Several different types of antioxidants (Met, Cys, Glut, MTG, and EDTA) were selected and tested based on the presence in marketed protein formulations and their mechanism of action.Citation60-65 Results from our stability studies demonstrate that incorporation of Met and EDTA as antioxidants in the PS80 formulation substantially improved the stability of this mAb and provided protection from oxidation without altering the protein structure and solution properties. These data also suggested that oxidation occurs not only through a peroxide-mediated pathway, but also through a metal-catalyzed reaction and highlighted the value of a combination approach toward ensuring a stable formulation.
The typical sequence of drug product development starts with formulation development and continues with long-term stability assessment of the selected formulation. Studies to verify that the clinical dosing methodology can safely administer the drug to the patient without loss of therapeutic properties are usually performed after the formulation has been selected. However, because of the significant risk of adsorption to surfaces during administration, the scope of product development work for low concentration biopharmaceuticals needs to be reevaluated to simultaneously address both the stability during storage and the clinical administration scheme. In other words, in-use stability studies need to be performed much earlier in the development program because the results can shape the composition of the final drug product. This approach is exemplified in the case study presented here. Preliminary i.v. infusions of the lowest dose of otelixizumab revealed that the 0.2 mg/mL mAb solution could not be administered as initially formulated, and a new formulation buffer was required to control the unacceptable protein loss via binding to the surfaces of the i.v. administration set. Parallel formulation development and in-use stability studies showed that the low concentration mAb was stabilized by a non-ionic surfactant (necessary to increase recovery from the tested i.v. bag, line, and filter, to levels greater than 90%, thus ensuring that the target dose could be administered to the patient), as well as antioxidants (needed to prevent free radical and metal-dependant oxidation and substantially improve the stability of the mAb).
We conclude that the administration of low concentration therapeutic mAbs comes with special challenges, a critical one being the order in which formulation development and in-use stability studies are performed. For high-risk products, such as the mAb discussed here, we recommend that the clinical dosing and handling methodology be evaluated early in development to guide and complement the selection of the formulation.
Materials and Methods
Monoclonal antibody
Otelixizumab is a humanized monoclonal antibody (IgG1) specific for human CD3. The molecule consists of a humanized heavy chain that has been modified to eliminate its single glycosylation site in the CH2 domain of the gamma (γ) heavy chain and a chimeric lambda (λ) light chain containing a rat variable domain linked to a human constant λ domain. The heavy chain constant region was reengineered by mutating asparagine 297 to alanine, thereby eliminating the only N-linked carbohydrate attachment site on the heavy chain. The otelixizumab drug substance is an aqueous solution of purified antibody containing 5 mg of antibody per mL in a histidine buffer with no additional excipients. The mAb was produced at AbbVie (Worcester, MA) by expression in Chinese hamster ovary cells followed by conventional downstream processing operations for purification.
Drug product vial container closure
Excipient screening and formulation stability studies were performed in 3 mL clear borosilicate type I glass vial (West Pharmaceutical Services) fitted with a gray-coated rubber stopper (Omniflex plus, FM259, Datwyler) and sealed using an aluminum overseal (West Pharmaceutical Services).
In-use stability materials
The following i.v. bags were tested in the in-use studies: PVC bag (Baxter, Viaflex container, 2B1301) and PO bag (B. Braun, PAB container, S8004-5384). The following i.v. infusion sets were assessed: B. Braun Horizon Pump Filtered i.v set (low PVC content, 0.2 μm PES filter, 2 injection sites, 3.1 m, V7415F) and Baxter Continu-Flo Solution Set with filter (PVC lines, 0.2 μm PES filter, 2 injection sites, 2.7 m, 2C6571s). Gravity-driven intravenous infusions were performed in all studies; the infusion rate was adjusted manually via manipulations of the roller clamp.
In-use stability study 1
The volume of normal saline in the i.v. bag was manually adjusted to match the target volumes of 25 mL and 50 mL, respectively. The otelixizumab drug substance was diluted to 0.2 mg/mL using histidine buffer (histidine J.T.Baker 2080, and histidine monohydrochloride monohydrate J.T.Baker 2081). All dilutions were made fresh and used immediately. To prepare the diluted i.v. dose, a volume needed to provide 0.1 mg (0.5 mL of 0.2 mg/mL mAb solution) was added to the i.v. normal saline bag. Following dilutions, the final concentrations in the i.v. bags were 0.004 mg/mL (for the 25 mL i.v. bags) and 0.002 mg/mL (for the 50 mL i.v. bags). The bags were placed on the laboratory bench and exposed to ambient temperature and lighting conditions. Three sets of bags were prepared for each test configuration: bag 1 served as a test of the initial condition, bag 2 reflected a 2-hour hold, and bag 3 simulated the clinical preparation and infusion of the protein solution through an i.v. line with in-line filter (B. Braun Horizon Pump Filtered i.v set). Replicate bags were prepared by different scientists, and samples were tested in duplicates. Samples were collected and tested immediately after the i.v. bag preparation (0 hours hold in the i.v. bag) and following a 2-hour hold in the i.v. bag. Additional samples were collected after a 2-hour infusion through the i.v. line. All samples were collected into polypropylene tubes (BD 352070). PS80 (polysorbate 80, J.T.Baker 4117) was added to all analytical retains at a final concentration of 0.001% to prevent loss via adsorption within the collection tubes. Percent (%) average recovery results were measured via HPLC analysis. Recoveries were calculated based on target concentrations in the bag, and the reported values were the average of 2 different preparations.
In-use stability study 2
A similar experimental design was used in the second study, as follows: mAb stock solutions were prepared fresh at 0.2 mg/mL concentration and containing various PS80 concentrations (0.02, 0.05, 0.1, and 0.3% w/v) by diluting the drug substance with 1% PS80 stock solution and histidine buffer. Infusion bags (PO only) were then prepared as previously described. The final mAb concentration in the i.v. bag was 0.002 mg/mL, and the final surfactant concentrations in the normal saline bags were 0.0002, 0.0005, 0.001, and 0.003%. The i.v. line tested was a Baxter Continu-Flo Solution Set with filter. Additional PS80 to a final concentration of at least 0.001% was added to all analytical samples. The mAb concentration was measured at initial and following either a 2-hour hold in the i.v. bag or infusion through the selected i.v. line. The recovery results were determined via HPLC analysis. Recoveries were calculated based on target concentrations in the bag, and the reported values were the average of 2 different preparations.
Stability of PS80-containing product and antioxidant screening study
The target formulations were prepared by diluting the drug substance with various excipient stock solutions prepared in histidine buffer as follows: 1% PS80; 250 mM Met (methionine, J.T.Baker 2085); 250 mM Cys (cysteine, Sigma, C6852); 250 mM Glut (glutathione, Calbiochem 3541); 250 mM MTG (monothioglycerol, MP Biomedicals 155723); 200 mM EDTA (edetate disodium, Mallinkckrodt Chemicals 4931). The antioxidant concentrations in the product vial were: 5 mM Met, 5 mM Cys, 5 mM Glut, 5 mM MTG, and 1 mM EDTA. The final mAb concentration in each vial was 0.2 mg/mL. Each test condition was aliquoted into 3 mL glass vials, 2.5 mL per vial, in a clean laminar flow hood. Four vials were prepared per timepoint per condition. Vials were stored at 2–8°C and 40°C for 2 weeks. The analytical testing included SDS-PAGE, cIEF, and MS analyses. Initial testing was not performed.
Drug product stability study
The mAb concentration in each vial was 0.2 mg/mL. The formulations incorporated Met at 0, 5, 10, 15, 20, and 40 mM levels with a fixed level of EDTA (0.05 mM) at a PS80 concentration of 0.1%. Additional formulations served as PS80 (no antioxidants) and buffer (no excipients) controls, respectively. Each formulation was aliquoted into glass vials at a fill volume of 2.5 mL per vial in a clean laminar flow hood. Vials were stored inverted at refrigerated (2–8°C, primary) and 25°C (accelerated) conditions. Analytical pulls were planned for 1, 2, 3, and 6 months, and oxidation levels on stability were measured using MS.
mAb concentration by Poros A – high performance liquid chromatography
Protein concentration measurements were carried out on an Agilent HP1100 system. Protein affinity HPLC analysis was developed for the quantification of the mAb content using a perfusion-type protein A-HPLC column (Poros A Immuno Detection Sensor Cartridge, 2.1 mm id × 30 mm L, 20 μm particle size). The chromatographic conditions were as follows: the mobile phases were 50 mM sodium phosphate, 500 mM sodium chloride, pH 7.0 (A) and 50 mM sodium phosphate, 500 mM sodium chloride, pH 2.0 (B), flow rate was kept constant at 1 mL/min, and column temperature was 25°C. The 6-minute method had the following gradient: the column was initially held at 100% solvent A; solvent A was then reduced to 0% at 0.4 min, returned to the starting level at 3.6 min, and held constant until the end of the assay. For mAb concentrations larger than 0.1 mg/mL, protein standards at either 5 mg/mL or 10 mg/mL were injected at varying volumes to create a calibration curve of protein loads at 10 μg, 20 μg, 30 μg, and 40 μg. The mAb was detected using UV light at 280 nm. The assay quantitation limit is 0.06 mg/mL. For mAb concentrations smaller than 0.1 mg/mL, a similar method was used which utilized the same Protein A column but detection was performed by fluorescence detection to increase sensitivity. The excitation wavelength was 280 nm and the emission wavelength 330 nm. The protein standard at 20 μg/mL was injected at varying volumes to create a calibration curve of protein loads at 60 ng, 100 ng, 200 ng, and 300 ng.
Stability assessment by sodium dodecyl sulfate polyacrylamide gel electrophoresis
The purity and stability of otelixizumab were analyzed by non-reducing SDS-PAGE. The protein solutions were diluted with 4X non-reducing sample buffer (Laemmli's Tris-HCl SDS, Boston BioProducts) to a final concentration of 0.15 mg/mL. The samples were heated for 10 minutes at 70°C and then centrifuged for 10 seconds at 10,000 rpm. From each sample prepared, 33 μL (containing 5 μg of antibody) was loaded per lane on a 10-well polyacrylamide gradient Tris-Glycine pre-cast gel (4–20% acrylamide, 1.5 mm, 10 × 10 cm, Tris-Glycine gel; Invitrogen - Novex) and focused at 125 V for 2 hours and 5 minutes. The running buffer was Tris/Glycine/SDS buffer (electrophoresis purity reagent, Bio-Rad). In order to determine the molecular weight of the detected bands, a marker (Mark 12 Molecular Weight standards, Invitrogen - Novex) was used. Protein bands were detected after staining the gels with Colloidal Blue Stain (Invitrogen) and de-staining with Milli Q water. Gel analysis was performed using a densitometric scanner (Molecular Dynamics), and integration of the resultant peaks was conducted via software analysis using Image Quant TL (GE Healthcare Lifesciences). Non-reduced gels were compared visually to determine if they are comparable to the reference standard.
Stability assessment by capillary isoelectric focusing
cIEF analysis was carried out using iCE280 electrophoresis system (Convergent) with a cartridge FC-coated capillary (Convergent 101701) and a 280 nm UV detector. Data were collected and analyzed using iCE280 CFR software 2.3. The capillary was first preconditioned according to the manufacturer's recommendations. The Focus Period 1 (desalting step) was set at 1500 V for 1 min. For Focus Period 2, 10 min at 3000 V was selected. The wash duration was 90 s. The injection volume was 35 μL. Two pI markers (pI 7.55 and 9.22) were present in the electropherogram. Phosphoric acid (90 mM H3PO4 with 0.1% methylcellulose aqueous solution) and sodium hydroxide (100 mM NaOH, with 0.1% methylcellulose in water) were used as anolyte and catholyte solutions, respectively. The samples were prepared by performing a 25-fold dilution with sample buffer solution containing 180 μL of water, 600 μL of 1% methylcellulose (Convergent Bioscience 101876), 200 μL of ampholytes (Pharmalyte 3–10, Sigma P/N P1522), and 20 μL of marker mix (1:1 v/v mix of pI 7.05 and pI 9.22 standards purchased from Convergent Biosciences). The total run time was ∼15 minutes for each run. All steps were conducted at 20°C, and the autosampler temperature was held constant at 5°C.
Determination of mAb oxidation by mass spectrometry
To assess the extent of methionine residue oxidation, peptide mapping was performed on the mAb samples following denaturation, reduction, alkylation, and tryptic digestion. Denaturation of the protein using guanidine hydrochloride as a chaotrope preceded reduction with dithiothreitol to convert all disulfide linkages to cysteine residues. To block the disulfide linkages from reforming, the cysteine residues were alkylated using iodoacetate to yield S-carboxymethyl cysteine. The S-carboxymethylated protein was proteolytically digested with trypsin, which cleaves the peptide bonds at the carboxyl terminal side of lysine and arginine. The resultant peptides were separated by reverse phase HPLC using a narrow bore C18 column and a gradient of water and acetonitrile containing 0.1% trifluroacetic acid. The column effluent was monitored by UV detection at 215, 254, and 280 nm, and by MS at m/z 100–2200.
Thermodynamic stability by differential scanning calorimetry
A VP Capillary-DSC instrument (MicroCal) was used to scan the mAb solutions from 25°C to 100°C at a scan rate of 70°C per hour with 10 minutes equilibration before each scan. All solutions were formulated at 1 mg/mL mAb in the histidine buffer containing 0% or 0.1% PS80. Tm (melting temperature, midpoint of unfolding transition) values were estimated from the thermograms using Origin 7.0 for DSC software.
Disclosure of Potential Conflict of Interest
All authors are current or former employees of GlaxoSmithKline. No potential conflicts of interest were disclosed.
Acknowledgments
The authors would like to thank Narendra Bam and Jon Dermott for reviewing the manuscript.
References
- Geigert J. Overview of the stability and handling of recombinant protein drugs. J Parenter Sci Technol 1989; 43:220-224; PMID:2809930
- Pinholt C, Hartvig RA, Medlicott NJ, Jorgensen L. The importance of interfaces in protein drug delivery - Why is protein adsorption of interest in pharmaceutical formulations? Expert Opin Drug Deliv 2011; 8:949-964; PMID:21557707; http://dx.doi.org/10.1517/17425247.2011.577062
- Burke CJ, Steadman BL, Volkin DB, Tsai PK, Bruner MW, Middaugh CR. The adsorption of proteins to pharmaceutical container surfaces. Int J Pharm 1992; 86:89-93; http://dx.doi.org/10.1016/0378-5173(92)90034-Y
- Patel JP, Tran LT, Sinai WJ, Carr LJ. Activity of urokinase diluted in 0.9% sodium chloride injection or 5% dextrose injection and stored in glass or plastic syringes. Am J Hosp Pharm 1991; 48:1511-1514; PMID:1882883
- Semba CP, Weck S, Patapoff T. Alteplase: Stability and bioactivity after dilution in normal saline solution. J Vasc Interv Radiol 2003; 14:99-102; PMID:12525593; http://dx.doi.org/10.1097/01.RVI.0000052297.26939.05
- Semba CP, Weck S, Razavi MK, Tuomi L, Patapoff T. Tenecteplase: Stability and bioactivity of thawed or diluted solutions used in peripheral thrombolysis. J Vasc Interv Radiol 2003; 14:475-479; PMID:12682204; http://dx.doi.org/10.1097/01.RVI.0000064843.87207.F7
- Tzannis ST, Hrushesky WJM, Wood PA, Przybycien TM. Adsorption of a formulated protein on a drug delivery device surface. J Colloid and Interface Sci 1997; 189:216-228; http://dx.doi.org/10.1006/jcis.1997.4841
- Zahid N, Taylor KMG, Gill H, Maguire F, Shulman R. Adsorption of insulin onto infusion sets used in adult intensive care unit and neonatal care settings. Diabetes Res Clin Practice 2008; 80:11-13; PMID:18395926; http://dx.doi.org/10.1016/j.diabres.2008.02.013
- Parshuram CS, Ng GY, Ho TK, Klein J, Moore AM, Bohn D, Koren G. Discrepancies between ordered and delivered concentrations of opiate infusions in critical care. Crit Care Med 2003; 31:2483-2487; PMID:14530755; http://dx.doi.org/10.1097/01.CCM.0000089638.83803.B2
- Parshuram CS, To T, Seto W, Trope A, Koren G, Laupacis A. Systematic evaluation of errors occurring during the preparation of intravenous medication. CMAJ 2008; 178:42-48; PMID:18166730; http://dx.doi.org/10.1503/cmaj.061743
- The United States Pharmacopeial Convention 1999. Chapter <797> Pharmaceutical Compounding—Sterile Preparations. United States Pharmacopeia, Rockville, MD.
- Bardin C, Astier A, Vulto A, Sewell G, Vigneron J, Trittler R, Daouphars M, Paul M, Trojniak M, Pinguet F. Guidelines for the practical stability studies of anticancer drugs: A european consensus conference. Euro J Hosp Pharm Sci Pra 2012; 19:278-285
- Beer PM, Wong SJ, Schartman JP, Kulas KE, Hartman CL, Giganti M, Falk NS. Infliximab stability after reconstitution, dilution, and storage under refrigeration. Retina 2010; 30:81-84; PMID:20010324; http://dx.doi.org/10.1097/IAE.0b013e3181b48fb4
- Kumru OS, Liu J, Ji JA, Cheng W, Wang J, Wang T, Joshi SB, Middaugh CR, Volkin DB. Compatibility, physical stability, and characterization of an IgG4 monoclonal antibody after dilution into different intravenous administration bags. J Pharm Sci 2012; 101:3636-3650; PMID:22733600; http://dx.doi.org/10.1002/jps.23224
- Kwong Glover ZW, Gennaro L, Yadav S, Demeule B, Wong PY, Sreedhara A. Compatibility and stability of pertuzumab and trastuzumab admixtures in i.v. infusion bags for coadministration. J Pharm Sci 2012; 102:794-812; PMID:23225178; http://dx.doi.org/10.1002/jps.23403
- Paul M, Vieillard V, Jaccoulet E, Astier A. Long-term stability of diluted solutions of the monoclonal antibody rituximab. Int J Pharm 2012; 436:282-290; PMID:22789912; http://dx.doi.org/10.1016/j.ijpharm.2012.06.063
- Sreedhara A, Glover ZK, Piros N, Xiao N, Patel A, Kebakoff B. Stability of IgG1 monoclonal antibodies in intravenous infusion bags under clinical in-use conditions. J Pharm Sci 2012; 101:21-30; PMID:21905032; http://dx.doi.org/10.1002/jps.22739
- Pandey M, Mahadevan D. Monoclonal antibodies as therapeutics in human malignancies. Future Oncol 2014; 10:609-636; PMID:24754592; http://dx.doi.org/10.2217/fon.13.197
- Buss NAPS, Henderson SJ, McFarlane M, Shenton JM, De Haan L. Monoclonal antibody therapeutics: History and future. Curr Opin Pharmacol 2012; 12:615-622; PMID:22920732; http://dx.doi.org/10.1016/j.coph.2012.08.001
- Reichert JM. Antibodies to watch in 2015. mAbs 2015; 7:1-8; PMID:25484055; http://dx.doi.org/10.4161/19420862.2015.988944
- Berek JS. Immunotherapy of ovarian cancer with antibodies: A focus on oregovomab. Expert Opin Biol Ther 2004; 4:1159-1165; PMID:15268682; http://dx.doi.org/10.1517/14712598.4.7.1159
- Daugherty AL, Mrsny RJ. Formulation and delivery issues for monoclonal antibody therapeutics. Adv Drug Deliv Rev 2006; 58:686-706; PMID:16839640; http://dx.doi.org/10.1016/j.addr.2006.03.011
- Ikesue H, Vermeulen LC, Hoke R, Kolesar JM. Stability of cetuximab and panitumumab in glass vials and polyvinyl chloride bags. Am J Health Syst Pharm 2010; 67:223-226; PMID:20101065; http://dx.doi.org/10.2146/ajhp090031
- Jung ST, Kang TH, Kelton W, Georgiou G. Bypassing glycosylation: engineering aglycosylated full-length IgG antibodies for human therapy. Curr Opin Biotechnol 2011; 22:858-867; PMID:21420850; http://dx.doi.org/10.1016/j.copbio.2011.03.002
- Wiczling P, Rosenzweig M, Vaickus L, Jusko WJ. Pharmacokinetics and pharmacodynamics of a chimeric/humanized anti-CD3 monoclonal antibody, otelixizumab (TRX4), in subjects with psoriasis and with type 1 diabetes mellitus. J Clin Pharmacol 2010; 50:494-506; PMID:19934031; http://dx.doi.org/10.1177/0091270009349376
- Burton L, Gandhi R, Duke G, Paborji M. Use of microcalorimetry and its correlation with size exclusion chromatography for rapid screening of the physical stability of large pharmaceutical proteins in solution. Pharm Dev Technol 2007; 12:265-273; PMID:17613890; http://dx.doi.org/10.1080/10837450701212610
- Wasylaschuk WR, Harmon PA, Wagner G, Harman AB, Templeton AC, Xu H, Reed RA. Evaluation of hydroperoxides in common pharmaceutical excipients. J Pharm Sci 2007; 96:106-116; PMID:16917844; http://dx.doi.org/10.1002/jps.20726
- Torosantucci R, Schoneich C, Jiskoot W. Oxidation of therapeutic proteins and peptides: Structural and biological consequences. Pharm Res 2014; 31:541-553; PMID:24065593; http://dx.doi.org/10.1007/s11095-013-1199-9
- Garber E, Demarest SJ. A broad range of Fab stabilities within a host of therapeutic IgGs. Biochem Biophys Res Commun 2007; 355:751-757; PMID:17321501; http://dx.doi.org/10.1016/j.bbrc.2007.02.042
- Liu H, Bulseco GG, Sun J. Effect of posttranslational modifications on the thermal stability of a recombinant monoclonal antibody. Immunol Lett 2006; 106:144-153; PMID:16831470; http://dx.doi.org/10.1016/j.imlet.2006.05.011
- Waterman KC, Adami RC. Accelerated aging: prediction of chemical stability of pharmaceuticals. Int J Pharm 2005; 293:101-125; PMID:15778049; http://dx.doi.org/10.1016/j.ijpharm.2004.12.013
- Liu D, Ren D, Huang H, Dankberg J, Rosenfeld R, Cocco MJ, Li L, Brems DN, Remmele RL, Jr. Structure and stability changes of human IgG1 Fc as a consequence of methionine oxidation. Biochemistry 2008; 47:5088-5100; PMID:18407665; http://dx.doi.org/10.1021/bi702238b
- Rabinow BE, Ding YS, Qin C, McHalsky ML, Schneider JH, Ashline KA, Shelbourn TL, Albrecht RM. Biomaterials with permanent hydrophilic surfaces and low protein adsorption properties. J Biomater Sci Polym Ed 1994; 6:91-109; PMID:7947476; http://dx.doi.org/10.1163/156856295X00788
- Kraegen EW, Lazarus L, Meler H. Carrier solutions for low level intravenous insulin infusion. Br Med J 1975; 3:464-466; PMID:1156820; http://dx.doi.org/10.1136/bmj.3.5981.464
- Page C, Dawson P, Woollacott D, Thorpe R, Mire-Sluis A. Development of a lyophilization formulation that preserves the biological activity of the platelet-inducing cytokine interleukin-11 at low concentrations. J Pharm Pharmacol 2000; 52:19-26; PMID:10716599; http://dx.doi.org/10.1211/0022357001773643
- Suelter CH, DeLuca M. How to prevent losses of protein by adsorption to glass and plastic. Anal Biochem 1983; 135:112-119; PMID:6670734; http://dx.doi.org/10.1016/0003-2697(83)90738-8
- Wasiewski W, Fasco MJ, Martin BM. Thrombin adsorption to surfaces and prevention with polyethylene glycol 6,000. Thrombosis Res 1976; 8:881-886; PMID:960059; http://dx.doi.org/10.1016/0049-3848(76)90017-7
- Lee HJ, McAuley A, Schilke KF, McGuire J. Molecular origins of surfactant-mediated stabilization of protein drugs. Advanced Drug Delivery Reviews 2011; 63:1160-1171; PMID:21763375; http://dx.doi.org/10.1016/j.addr.2011.06.015
- Kiese S, Papppenberger A, Friess W, Mahler HC. Shaken, not stirred: mechanical stress testing of an IgG1 antibody. J Pharm Sci 2008; 97:4347-4366; PMID:18240293; http://dx.doi.org/10.1002/jps.21328
- Mahler HC, Muller R, Friess W, Delille A, Matheus S. Induction and analysis of aggregates in a liquid IgG1-antibody formulation. Eur J Pharm Biopharm 2005; 59:407-417; PMID:15760721; http://dx.doi.org/10.1016/j.ejpb.2004.12.004
- Vidanovic D, Milic AJ, Stankovic M, Poprzen V. Effects of nonionic surfactants on the physical stability of immunoglobulin G in aqueous solution during mechanical agitation. Pharmazie 2003; 58:399-404; PMID:12857003
- Duncan MR, Lee JM, Warchol MP. Influence of surfactants upon protein/peptide adsorption to glass and polypropylene. Int J Pharm 1995; 120:179-188; http://dx.doi.org/10.1016/0378-5173(94)00402-Q
- Johnston TP. Adsorption of recombinant human granulocyte colony stimulating factor (rhG-CSF) to polyvinyl chloride, polypropylene, and glass: effect of solvent additives. PDA J Pharm Sci Technol 1996; 50:238-245; PMID:8810839
- Ha E, Wang W, Wang YJ. Peroxide formation in polysorbate 80 and protein stability. J Pharm Sci 2002; 91:2252-2264; PMID:12226852; http://dx.doi.org/10.1002/jps.10216
- Wang W, Wang YJ, Wang DQ. Dual effects of Tween 80 on protein stability. Int J Pharm 2008; 347:31-38; PMID:17692480; http://dx.doi.org/10.1016/j.ijpharm.2007.06.042
- Kerwin BA. Polysorbates 20 and 80 used in the formulation of protein biotherapeutics: structure and degradation pathways. J Pharm Sci 2008; 97:2924-2935; PMID:17973307; http://dx.doi.org/10.1002/jps.21190
- Usami A, Ohtsu A, Takahama S, Fujii T. The effect of pH, hydrogen peroxide and temperature on the stability of human monoclonal antibody. J Pharm Biomed Anal 1996; 14:1133-1140; PMID:8818025; http://dx.doi.org/10.1016/S0731-7085(96)01721-9
- Lowe D, Dudgeon K, Rouet R, Schofield P, Jermutus L, Christ D. Aggregation, stability, and formulation of human antibody therapeutics. Adv Protein Chem Struct Biol 2011; 84:41-61; PMID:21846562; http://dx.doi.org/10.1016/B978-0-12-386483-3.00004-5
- Lahlou A, Blanchet B, Carvalho M, Paul M, Astier A. Mechanically-induced aggregation of the monoclonal antibody cetuximab. Ann Pharm Fr 2009; 67:340-352; PMID:19695370; http://dx.doi.org/10.1016/j.pharma.2009.05.008
- Ji JA, Zhang B, Cheng W, Wang YJ. Methionine, tryptophan, and histidine oxidation in a model protein, PTH: mechanisms and stabilization. J Pharm Sci 2009; 98:4485-4500; PMID:19455640; http://dx.doi.org/10.1002/jps.21746
- Chumsae C, Gaza-Bulseco G, Sun J, Liu H. Comparison of methionine oxidation in thermal stability and chemically stressed samples of a fully human monoclonal antibody. J Chromatogr B Analyt Technol Biomed Life Sci 2007; 850:285-294; PMID:17182291; http://dx.doi.org/10.1016/j.jchromb.2006.11.050
- Bertolotti-Ciarlet A, Wang W, Lownes R, Pristatsky P, Fang Y, McKelvey T, Li Y, Li Y, Drummond J, Prueksaritanont T, Vlasak J. Impact of methionine oxidation on the binding of human IgG1 to Fc Rn and Fc gamma receptors. Mol Immunol 2009; 46:1878-1882; PMID:19269032; http://dx.doi.org/10.1016/j.molimm.2009.02.002
- Liu H, Gaza-Bulseco G, Zhou L. Mass spectrometry analysis of photo-induced methionine oxidation of a recombinant human monoclonal antibody. J Am Soc Mass Spectrom 2009; 20:525-528; PMID:19103498; http://dx.doi.org/10.1016/j.jasms.2008.11.011
- Margiloff L, Chaplia L, Chow A, Singhal PC, Mattana J. Metal-catalyzed oxidation of immunoglobulin G impairs Fc receptor-mediated binding to macrophages. Free Radic Biol Med 1998; 25:780-785; PMID:9823543; http://dx.doi.org/10.1016/S0891-5849(98)00130-0
- Kayser V, Chennamsetty N, Voynov V, Forrer K, Helk B, Trout BL. Glycosylation influences on the aggregation propensity of therapeutic monoclonal antibodies. Biotechnol J 2011; 6:38-44; PMID:20949542; http://dx.doi.org/10.1002/biot.201000091
- Wen J, Jiang Y, Nahri L. Effect of carbohydrate on thermal stability of antibodies. Am Pharm Rev 2008; 11:98-104; http://dx.doi.org/10.1002/jps.3080110209
- Zheng K, Bantog C, Bayer R. The impact of glycosylation on monoclonal antibody conformation and stability. MAbs 2011; 3:568-576; PMID:22123061; http://dx.doi.org/10.4161/mabs.3.6.17922
- Liu H, Gaza-Bulseco G, Xiang T, Chumsae C. Structural effect of deglycosylation and methionine oxidation on a recombinant monoclonal antibody. Mol Immunol 2008; 45:701-708; PMID:17719636; http://dx.doi.org/10.1016/j.molimm.2007.07.012
- Waterman KC, Adami RC, Alsante KM, Hong J, Landis MS, Lombardo F, Roberts CJ. Stabilization of pharmaceuticals to oxidative degradation. Pharm Dev Technol 2002; 7:1-32; PMID:11852692; http://dx.doi.org/10.1081/PDT-120002237
- Lam XM, Yang JY, Cleland JL. Antioxidants for prevention of methionine oxidation in recombinant monoclonal antibody HER2. J Pharm Sci 1997; 86:1250-1255; PMID:9383735; http://dx.doi.org/10.1021/js970143s
- Reyes N, Ruiz L, Aroche K, Geronimo H, Brito O, Hardy E. Stability of Ala 125 recombinant human interleukin-2 in solution. J Pharm Pharmacol 2005; 57:31-37; PMID:15638990; http://dx.doi.org/10.1211/0022357055182
- Yin J, Chu JW, Ricci MS, Brems DN, Wang DI, Trout BL. Effects of antioxidants on the hydrogen peroxide-mediated oxidation of methionine residues in granulocyte colony-stimulating factor and human parathyroid hormone fragment 13-34. Pharm Res 2004; 21:2377-2383; PMID:15648272; http://dx.doi.org/10.1007/s11095-004-7692-4
- Knepp VM, Whatley JL, Muchnik A, Calderwood TS. Identification of antioxidants for prevention of peroxide-mediated oxidation of recombinant human ciliary neurotrophic factor and recombinant human nerve growth factor. PDA J Pharm Sci Technol 1996; 50:163-171; PMID:8696780
- Sosabowski JK, Lee M, Dekker BA, Simmons BP, Singh S, Beresford H, Hagan SA, McKenzie AJ, Mather SJ, Watson SA. Formulation development and manufacturing of a gastrin/CCK-2 receptor targeting peptide as an intermediate drug product for a clinical imaging study. Eur J Pharm Sci 2007; 31:102-111; PMID:17387005; http://dx.doi.org/10.1016/j.ejps.2007.02.007
- Yin J, Chu JW, Ricci MS, Brems DN, Wang DI, Trout BL. Effects of excipients on the hydrogen peroxide-induced oxidation of methionine residues in granulocyte colony-stimulating factor. Pharm Res 2005; 22:141-147; PMID:15771240; http://dx.doi.org/10.1007/s11095-004-9019-x