Abstract
Agents that block the anti-phagocytic signal CD47 can synergize with pro-phagocytic anti-tumor antigen antibodies to potently eliminate tumors. While CD47 is overexpressed on cancer cells, its expression in many normal tissues may create an ‘antigen sink’ that could minimize the therapeutic efficacy of CD47 blocking agents. Here, we report development of bispecific antibodies (BsAbs) that co-target CD47 and CD20, a therapeutic target for non-Hodgkin lymphoma (NHL), that have reduced affinity for CD47 relative to the parental antibody, but retain strong binding to CD20. These characteristics facilitate selective binding of BsAbs to tumor cells, leading to phagocytosis. Treatment of human NHL-engrafted mice with BsAbs reduced lymphoma burden and extended survival while recapitulating the synergistic efficacy of anti-CD47 and anti-CD20 combination therapy. These findings serve as proof of principle for BsAb targeting of CD47 with tumor-associated antigens as a viable strategy to induce selective phagocytosis of tumor cells and recapitulate the synergy of combination antibody therapy. This approach may be broadly applied to cancer to add a CD47 blocking component to existing antibody therapies.
Abbreviations
SPR | = | surface plasmon resonance |
IgG | = | immunoglobulin G |
VH | = | immunoglobulin heavy chain variable region |
VL | = | immunoglobulin light chain variable region |
Introduction
Monoclonal antibodies hold enormous promise as anti-cancer therapeutics due to their ability to harness the immune system for attack of a highly specific target cell population. Identification of tumor-specific antigens has revolutionized cancer therapy, with ∼40 antibodies currently approved and over 300 antibodies undergoing clinical development.Citation1-2 However, while therapeutic antibodies have proved efficacious as molecularly targeted cancer therapies, they are generally administered in combination with chemotherapy due to limited clinical efficacy as monotherapy.Citation3
Immune effector cells are critical to the efficacy of anti-cancer antibodies through a number of mechanisms including antibody-dependent cell-mediated cytotoxicity (ADCC), antibody-dependent cellular phagocytosis (ADCP), and priming of antigen-specific T cells through cross-presentation of tumor antigens.Citation1,4 Phagocytosis is partially regulated by SIRPα, a protein expressed on the surface of phagocytic cells, including macrophages and dendritic cells.Citation5 The interaction of SIRPα with its ligand, CD47, a widely expressed transmembrane protein, transmits a “don't eat me” signal by initiating signaling cascades that ultimately inhibit phagocytosis.Citation6-9 Increased expression of CD47 has been detected on acute myeloid leukemia stem cells (AML LSC), multiple subtypes of B cell non-Hodgkin lymphoma (NHL), and many human solid tumor cells.Citation10-13 CD47 plays an important role in cancer pathogenesis, as increased expression on tumor cells permits evasion of phagocytosis.Citation14 This mechanism can be therapeutically modulated with monoclonal antibodies targeting CD47 or recombinant SIRPα proteins that disrupt the CD47-SIRPα interaction.Citation10,15,16
Therapeutic agents that antagonize the CD47-SIRPα interaction present a unique opportunity to enhance the efficacy of cancer-targeting therapeutic antibodies. Since CD47 blocking antibodies enable phagocytosis by blocking an inhibitory signal, therapeutic synergy can be achieved by combining CD47 blockade with a pro-phagocytic signal elicited by an Fc receptor (FcR)-activating antibody.Citation17 Such synergy was demonstrated through combination of blocking anti-CD47 antibody with rituximab, an approved anti-CD20 antibody known to engage FcRs.Citation11,18 Further evidence of synergy was provided by the demonstration of enhanced trastuzumab-mediated killing of breast cancer cells upon blockade of the CD47-SIRPα interaction with antibodies directed against CD47 or SIRPα.Citation19 Most recently, synergistic induction of phagocytosis was observed between high affinity SIRPα monomers that antagonize CD47 and tumor-specific monoclonal antibodies including trastuzumab, rituximab, and cetuximab.Citation15 Collectively, these studies highlight the potential for synergistic elimination of cancer cells by adding a CD47-SIRPα blocking component to existing antibody therapies.
Bispecific antibodies (BsAbs) are an emerging class of antibody therapeutics that exhibit specific binding to 2 different antigens.Citation20-21 Many formats of BsAbs have been engineered using recombinant approaches, including IgG-like BsAbs that contain an Fc domain. These BsAbs are desired for many clinical applications because the intact Fc region supports effector functions and confers a long serum half-life. A unique feature of BsAbs is the potential for bispecificity to afford stronger binding to dual antigen-expressing cells relative to single-antigen cells as a result of multivalency leading to higher avidity interactions. This potential has been extensively proposed, but there are few successful examples.Citation22-25 Bispecific fragments directed against different epitopes within the same antigen exhibit increased binding, but avidity in these cases is likely dependent upon conformational changes of the antigen.Citation26-28 Improved tumor localization was observed with a BsAb targeting ErbB2 and carcinoembryonic antigen, but avid BsAb binding to single antigen-expressing cells indicated a lack of selectivity for dual antigen-expressing cells.Citation29 Similarly, improved tumor targeting was reported with a BsAb targeting ErbB2 and ErbB3.Citation30 Notably, both of these BsAbs exhibiting some selectivity for dual antigen-expressing cells were small recombinant BsAb fragments that did not contain an Fc domain. Thus far, IgG-like BsAbs capable of selectively binding dual antigen-expressing cells have not been described.
Attempts to induce phagocytosis through blockade of CD47-SIRPα signaling with either blocking antibodies or recombinant SIRPα proteins have shown promise, but these strategies fail to address the issue of selective targeting of tumor cells. Expression of CD47 on normal tissues may create an ‘antigen sink’ that prevents anti-CD47 therapeutic antibodies from reaching intended tumor cell targets in vivo. One strategy to circumvent this issue is to employ BsAbs with reduced affinity for CD47 that retain the ability to block the CD47-SIRPα interaction, but require binding to a second tumor antigen for high affinity binding. We hypothesized that such BsAbs targeting CD47 and CD20 would direct CD47-SIRPα blockade specifically to cells that co-express CD20, an established therapeutic target in NHL.Citation31 Moreover, we hypothesized that such BsAbs would recapitulate the therapeutic synergy observed with anti-CD47 and anti-CD20 combination therapy. Here, we tested these hypotheses by generating BsAbs specific for CD47, with reduced affinity, and CD20, and investigated their binding specificity and functional effects against human NHL.
Results
Bispecific antibodies targeting CD47 and CD20 bind to each antigen alone
BsAbs were generated using the dual-variable-domain immunoglobulin (DVD-Ig) format that introduces the variable domains from the heavy and light chains of one antibody onto the amino termini of the respective chains of a second antibody through an amino acid linker (; Fig. S1).Citation32 Variable domains in the internal position of DVD-Ig molecules often exhibit reduced affinity for their target antigens compared to the parental antibodies.Citation33 We sought to take advantage of this reduced affinity by engineering the anti-CD47 variable domains into the internal position. Two variants of CD20-CD47 DVD-Igs were generated with either a short (SL) or long (LL) linker sequence between the variable domains. Co-transfection of the heavy and light chains of each DVD-Ig led to the production of a single IgG–like species, with expected molecular weights for each chain (). The increased size of each chain relative to the parental antibodies is reflective of the additional variable domain on each chain, and is consistent with previously reported molecular weights for DVD-Ig chains.Citation32 Size exclusion chromatography confirmed that the CD20-CD47 DVD-Ig molecule was produced as a single species with no detectable aggregate formation (Fig. S2)
Figure 1. Bispecific antibodies targeting CD47 and CD20 bind to each antigen alone: (A) Schematic of a CD20-CD47 DVD-Ig molecule with each variable domain of anti-CD20 (red) connected to each variable domain of anti-CD47 (blue) through a short or long amino acid linker. Constant regions are human Constant Light (kappa) and CH1, CH2, CH3 from human IgG1. (B) SDS-PAGE analysis of purified antibodies under non-reducing (left) and reducing (right) conditions. Sizes of heavy chain (HC) and light chain (LC) for each antibody type are indicated. (C) Antibodies were used to stain rat YB2/0 cells engineered to express human CD20, but not human CD47 (left) or YB2/0 cells engineered to express human CD47, but not human CD20 (right) prior to detection with PE-conjugated anti-human secondary antibody by flow cytometry. The experiment was performed 3 times with similar results. (D) Kinetic association and dissociation parameters, along with calculated affinity (KD) were measured by surface plasmon resonance using Biacore for anti-CD47 (B6H12-hIgG4), CD20-CD47 SL, or CD20-CD47 LL for human CD47. (E) CD20+CD47- YB2/0 cells were incubated with the indicated antibodies over a range of concentrations prior to staining with 10 μg/ml DyLight 488 (DL488) anti-CD20. Mean fluorescence intensity (MFI) of the DL488 signal was measured by flow cytometry. Data are representative of 3 independent experiments performed in triplicate. (F) CD20-CD47+ YB2/0 cells were incubated with the indicated antibodies over a range of concentrations prior to staining with 10 μg/ml Alexa Fluor 647 (AF647) anti-CD47. Mean fluorescence intensity (MFI) of the AF647 signal was measured by flow cytometry. Data are representative of 3 independent experiments performed in triplicate.
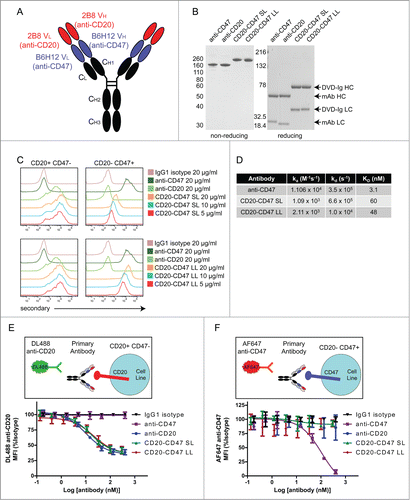
BsAbs were evaluated for their ability to bind to antigen-expressing cells using a rat cell line engineered to express human CD20 or, separately, human CD47. Binding of each BsAb to CD20 was comparable to the binding of parental anti-CD20 antibody () Both BsAbs also bound to CD47, but with compromised affinity relative to the parental anti-CD47 antibody () Notably, CD20-CD47 LL exhibited stronger binding to CD47 than CD20-CD47 SL, presumably due to improved accessibility to antigen.
To quantitatively determine the affinity of each antibody for monomeric CD47, binding affinities were determined using surface plasmon resonance (SPR) with Biacore (; Fig. S3) The affinity of CD20-CD47 LL was reduced ∼ 15-fold, while the affinity of CD20-CD47 SL was reduced 20-fold relative to anti-CD47 .() The increased affinity observed with the CD20-CD47 LL relative to CD20-CD47 SL is indicative of the greater flexibility and accessibility to antigen allowed by the longer linker, and is consistent with reported data for the DVD-Ig format.Citation33
The epitope recognized by the 2B8 anti-CD20 clone is dependent upon the conformation of a loop structure that is created between transmembrane domains, and is therefore not possible to mimic with a recombinant fusion protein for use in SPR studies.Citation34-35 To assess the strength of BsAb binding to the CD20 antigen on the cell surface, rat cells engineered to express human CD20 were stained with test antibodies prior to staining with a fluorescently conjugated anti-CD20 antibody generated from the same 2B8 clone (). Both BsAbs blocked the fluorescent anti-CD20 signal with an identical pattern as observed with the anti-CD20 parental antibody, indicating a comparable affinity. The reciprocal experiment was conducted using cells engineered to express human CD47 and fluorescently conjugated anti-CD47 antibody (), and only the parental anti-CD47 blocked binding of labeled anti-CD47 antibody. Given the ability of the BsAbs to bind these cells in the absence of competition with anti-CD47 antibody (), the lack of blocking with the BsAbs is indicative of a weaker interaction with CD47 that is outcompeted by labeled anti-CD47.
Simultaneous binding to CD47 and CD20 contributes to BsAb binding to cells
We next wanted to address whether a single BsAb molecule could simultaneously bind to both antigens. Cells expressing CD20 but not CD47 were coincubated with test antibodies and complexes containing the recombinant biotinylated CD47 antigen bound to neutravidin-fluorescent conjugates. Antibody binding to cells was then detected by flow cytometry with a secondary antibody, and double positive live cell events indicated simultaneous binding to CD20 and CD47 (). CD20-CD47 SL and CD20-CD47 LL were capable of simultaneous binding to both antigens, with CD20-CD47 LL demonstrating more double-positive events, consistent with its greater affinity for CD47 (; Fig. S4). Having demonstrated that both BsAbs are capable of binding simultaneously to each antigen in trans, we next sought to determine if simultaneous binding occurred in the context of antigens expressed on the cell surface. This is particularly important as the BsAbs retain a high affinity to CD20 () that could potentially drive BsAb binding to dual-antigen cells independent of CD47 binding. Raji cells, a human lymphoma cell line that expresses both CD20 and CD47, were stained with test antibodies prior to staining with fluorescently conjugated parental anti-CD47 (). Anti-CD47 and both BsAbs completely blocked the subsequent binding of fluorescent anti-CD47, indicating that these antibodies bound to CD47 (). The reciprocal experiment demonstrated binding of BsAbs to CD20 on dual antigen-expressing Raji cells (). Collectively, these data demonstrate that CD20 and CD47 each contribute to BsAb binding to dual antigen-expressing cells.
Figure 2. Simultaneous binding to CD47 and CD20 contributes to BsAb binding to cells: (A) Schematic of experimental design to detect simultaneous binding to CD20 and CD47. Biotinylated CD47 antigen was linked to a fluorescent (DyLight 650) neutravidin tetramer, which was co-incubated with unlabeled test antibody and CD20+CD47- YB2/0 cells. Test antibody binding was detected by staining with PE-conjugated anti-human secondary antibody. PE+DyLight 650+ live cells indicate simultaneous binding of antibody to both antigens. (B) Double positive events were detected by flow cytometry and represent simultaneous binding to CD47 and CD20. ****p < 0.0001, t test, (n = 6), error bars ± SEM. (C) CD20+CD47+ Raji cells were incubated with the indicated primary antibodies at 10 μg/ml prior to staining with 10 μg/ml AF647 anti-CD47. MFI of the AF647 signal was determined by flow cytometry and all test antibodies were compared to isotype control. ***p < 0.001; ns, not significant, t test, (n = 4), error bars ± SEM. (D) CD20+CD47+ Raji cells were incubated with the indicated primary antibodies at 10 μg/ml prior to staining with 10 μg/ml DyLight 488 anti-CD20. MFI of the DyLight 488 signal was determined by flow cytometry and all test antibodies were compared to isotype control. ***p < 0.001; ns, not significant, t test, (n = 4), error bars ± SEM.
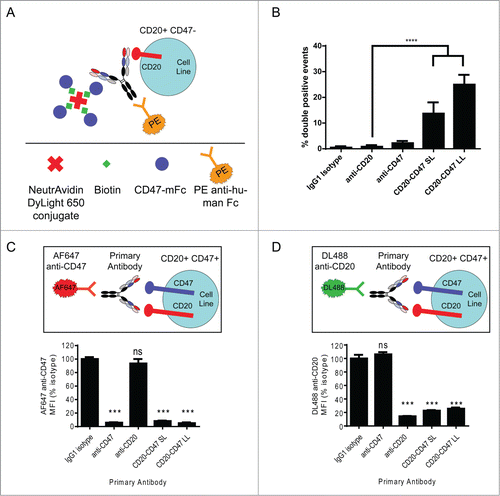
CD20-CD47 SL selectively binds to dual antigen-expressing cells in the presence of an ‘antigen sink’
A major reason for generating BsAbs targeting CD20 and CD47 is to achieve the therapeutic benefit of blocking the CD47-SIRPα interaction on tumor cells while avoiding a potential ‘antigen sink’ created by normal cells expressing CD47. Red blood cells (RBCs) are a likely candidate for an antigen sink as they are abundant, accessible to antibody in the bloodstream, and highly express CD47, which is involved in RBC clearance.Citation36 To determine whether BsAbs selectively bind dual antigen-expressing cells in the presence of excess CD47-only expressing cells, CD20+CD47+ tumor cells were labeled with CFSE and mixed with a 20-fold excess of unlabeled RBCs prior to incubation with antibody (). Cells from 2 patients with B cell chronic lymphocytic leukemia (B-CLL) were used as dual antigen cells in this assay because high expression of CD47 has been observed in this tumor type ().Citation11 To determine the relative strength with which each antibody bound to each cell type, events were gated by cell type and antibody binding was assessed. BsAbs, as well as the control anti-CD47 and anti-CD20, bound to tumor cells, whereas only anti-CD47 and CD20-CD47 LL bound appreciably to RBCs (). Importantly, CD20-CD47 SL did not bind strongly to RBCs, indicating that reduced affinity for CD47 successfully reduced binding to RBCs. To determine whether each antibody preferentially bound to tumor cells or RBCs, cells within the PE-positive gate were discriminated as either CLL (CFSE+) or RBC (CFSE-). The majority of cells bound by anti-CD47 and CD20-CD47 LL were RBC (). In contrast, anti-CD20 and CD20-CD47 SL bound preferentially to tumor cells (). To confirm that BsAbs were engaging CD47 on tumor cells, the ability of the antibody to block subsequent binding of labeled anti-CD47 was determined as in (). Both BsAbs blocked staining with this antibody, indicating that the BsAbs were binding to the CD47 antigen on tumor cells (). Similar findings were observed with Raji cells (Fig. S5). Notably, at a lower concentration, both BsAbs blocked the labeled anti-CD47 more completely than anti-CD47 (Fig. S5), suggesting that antibodies capable of dual binding to both antigens exhibit higher avidity binding to Raji cells than antibody that bound to CD47 alone.
Figure 3. CD20-CD47 SL selectively binds to dual antigen-expressing cells in the presence of an antigen sink: (A) Schematic of the experimental design to assay for selectivity in binding to dual antigen-expressing cells in the presence of an excess of CD47-only expressing cells. CLL cells were labeled with CFSE and mixed with a 20-fold excess of CD20-CD47+ human red blood cells (RBCs). Cell mixtures were incubated with primary antibody prior to staining with PE anti-human Fc secondary and AF647 anti-CD47, and analysis by flow cytometry. (B) Two primary CLL samples were stained for CD47 and CD20 expression to identify the percentage of dual antigen-expressing cells by flow cytometry. (C) CLL cells were distinguished from RBCs on the basis of CFSE uptake. Primary antibody was used at 10 μg/ml and binding to cells was detected with PE secondary antibody staining. (D) Percentages of CLL cells (CFSE+) and RBC (CFSE-) within the antibody bound (PE+) population. A single replicate from each donor was averaged together (n = 2). (E) Binding of AF647 anti-CD47 to CLL cells from panel C is reported as MFI normalized to isotype control. A single replicate from each donor was averaged together (n = 2).
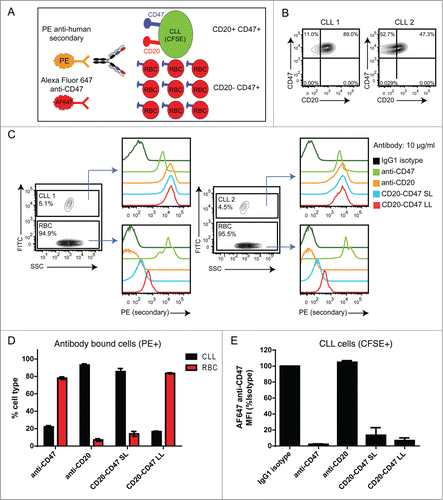
BsAbs induce phagocytosis of target cells in an FcR-dependent manner and recapitulate the synergy of antibody combinations
We hypothesized that CD20-CD47 BsAbs would enable phagocytosis of tumor cells as a result of blocking the CD47-SIRPα interaction and engaging FcRs on macrophages. To test this, CD20+CD47+ CFSE-labeled cell lines were co-incubated with unlabeled human macrophages in the presence of different antibodies, and phagocytosis of target cells was assessed by flow cytometry. Antibodies directed against CD47 or CD20 induced significant phagocytosis relative to the baseline level observed with isotype control antibody (). Both BsAbs recapitulated the synergy of anti-CD47 and rituximab combination treatment and increased phagocytosis relative to treatment with anti-CD47 alone in most cell lines tested.
Figure 4. BsAbs induce phagocytosis of target cells in an FcR-dependent manner and recapitulate the synergy of antibody combinations: (A) Phagocytosis of CD20+CD47+CFSE+ cell lines by donor-derived human macrophages was assessed by flow cytometry. All antibodies were used at a final concentration of 10 μg/ml. Anti-CD47 is B6H12-mIgG1. The assay was performed with macrophages derived from 3 independent donors. The percentage of CFSE+ macrophages was normalized to the maximal response for each donor. ****p < 0.0001; ***p < 0.001; **p < 0.01; ns, not significant versus anti-CD47 by one-way ANOVA, (n = 9), error bars ± SEM. (B) J774 mouse macrophage cells were pre-incubated with anti-CD16/anti-CD32 to block Fc receptors. Macrophages were co-incubated with CD20+CD47+GFP+ Raji cells and the indicated antibodies at 1 μg/ml. Anti-CD47 is B6H12-mIgG1. The percentage of GFP+ macrophages was determined by flow cytometry and was normalized to the maximal response in each of 2 independent experiments. ns, not significant; ****p < 0.0001, t test, (n = 6), error bars ± SEM.
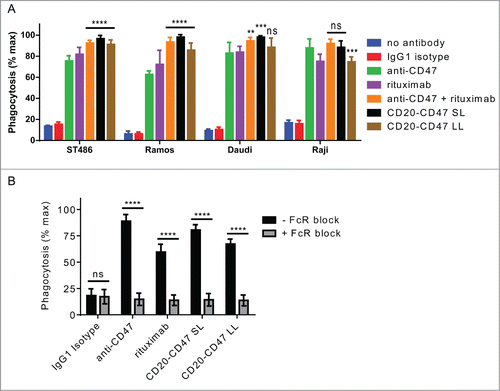
To explore whether FcR interactions contributed to the pro-phagocytic effect of the BsAbs, phagocytosis was measured in the presence of inhibitors of FcR binding. All antibody-mediated phagocytosis was potently inhibited by pre-incubation with a cocktail of FcR-blocking anti-CD16 and anti-CD32 antibodies, indicating a requirement of FcR interaction for induction of phagocytosis by the BsAbs ().
CD20-CD47 SL reduces lymphoma burden and extends survival in vivo
We opted to pursue CD20-CD47 SL as our lead candidate for in vivo studies because its reduced affinity for CD47 successfully reduces binding to the CD47-expressing antigen sink (). To test the in vivo therapeutic potential of CD20-CD47 SL, we utilized localized and disseminated human NHL models that we previously described.Citation11 To model localized disease, NSG mice, which lack T, B, and NK cells but retain phagocytic cells,Citation37 were transplanted subcutaneously with luciferase-expressing Raji cells. Upon establishment of engraftment, mice were administered daily antibody treatments. Anti-CD47 antibody and rituximab alone each decreased lymphoma burden () and marginally extended survival relative to control mice (). As we previously reported, the combination of anti-CD47 and rituximab eliminated detectable lymphoma and led to long-term disease-free survival ().Citation11 Strikingly, CD20-CD47 SL recapitulated the effect of combination therapy and significantly prolonged survival relative to mice treated with either anti-CD47 or rituximab alone (). Similar findings were observed in a disseminated lymphoma model, in which 3 different doses were tested in order to identify conditions with DVD-Ig serum levels comparable to rituximab (Fig. S6). This was best achieved with 1 mg of DVD-Ig and 200 micrograms of rituximab (Fig. S6). Notably, the improved outcome with CD20-CD47 SL relative to anti-CD47 or rituximab in both models occurs despite lower serum levels of CD20-CD47 SL ()
Figure 5. CD20-CD47 SL reduces lymphoma burden and extends survival in vivo: (A) NSG mice transplanted subcutaneously with Raji-luciferase cells were treated with daily injections of 200 μg mouse IgG control, anti-CD47 antibody, rituximab, or 200 μg anti-CD47 antibody + 200 μg rituximab. CD20-CD47 SL was administered at 1 mg to account for the increased molecular weight (n = 10 per treatment group). Luciferase imaging of representative mice from pre-treatment and after 14 doses are shown. (B) Bioluminescence values were normalized to pre-treatment values for each mouse. p values were derived by one-way ANOVA with Holm-Sidak's correction. *p < 0.05, **p < 0.01, ****p < 0.0001, line denotes mean. (C) Survival of engrafted mice. Arrows indicate the start (day 7) and stop (day 21) of treatment. Statistical analysis was performed by Mantel-Cox test. (D) Serum concentration pharmacokinetic profiles of each antibody throughout the course of treatment. Each line represents an individual mouse. Arrows indicate start (day 7) and stop (day 21) of treatment. Immobilized antibody directed against the human or mouse Fc region was used to capture the treatment antibodies from mouse serum prior to detection with HRP-conjugated antibody against the human or mouse kappa light chain of the treatment antibodies. Standard curves using known concentrations of antibodies were used to derive the serum levels. Antibody concentration is represented as μM of anti-CD20 F(ab’)2 (left) or as μM of anti-CD47 F(ab’)2 (right).
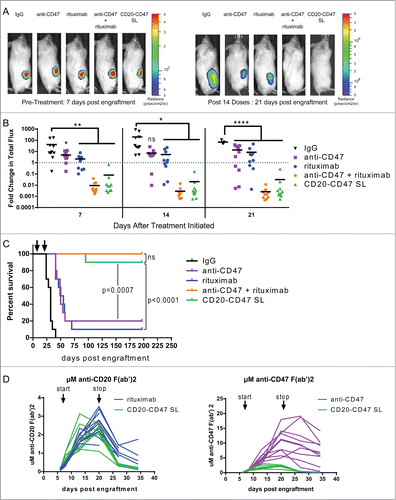
Discussion
We report here the generation of BsAbs that target CD47 along with CD20 and recapitulate the benefits of combination antibody treatment within a single molecule. Specifically, we established a BsAb format that exhibited reduced affinity for CD47 relative to the parental anti-CD47 antibody, permitting selective binding to dual antigen-expressing cells in the presence of a large antigen sink. Moreover, our CD20-CD47 BsAb was able to simultaneously bind to both antigens and retain the therapeutic benefit of tumor cell phagocytosis. Importantly, the CD20-CD47 SL BsAb was able to recapitulate the in vivo therapeutic synergy obtained through combination of anti-CD47 and rituximab. Together, these results establish BsAb targeting of CD47 along with a tumor antigen as a viable strategy for directing the synergistic benefits of combination therapy specifically toward tumor cells.
CD47-mediated activation of SIRPα signaling results in inhibition of phagocytosis, and reagents that antagonize this interaction are potential therapeutics that act by enabling phagocytosis.Citation17,38 Importantly, CD47-targeting agents have the potential to augment existing antibody therapies by adding the benefit of blocking the CD47-mediated inhibitory signal to the established therapeutic effect of pro-phagocytic anti-cancer antibodies. We originally tested this hypothesis using rituximab, an FcR-activating pro-phagocytic antibody, in combination with a blocking anti-CD47 antibody, and observed synergistic phagocytosis and elimination of human NHL in vivo.Citation11 This synergy mechanism was further demonstrated with several approaches to disrupt CD47-SIRPα interactions resulting in increased antibody-mediated elimination of melanoma cells in vivo and enhanced trastuzumab-mediated ADCC in vitro.Citation19 Notably, interference of the CD47-SIRPα interaction with F(ab’)2 fragments of anti-CD47 synergized with trastuzumab, but had no effect alone, suggesting a requirement in this setting for an Fc domain in addition to CD47-SIRPα blockade for cancer cell elimination. In agreement with these findings, a third study showed that high affinity SIRPα variants increased phagocytosis when presented as Fc fusion proteins, but failed to effectively induce phagocytosis in monomeric form or when expressed as dimers lacking Fc domains.Citation15 Interestingly, this property allowed SIRPα monomers to function as adjuvants to increase the efficacy of the tumor-specific monoclonal antibodies trastuzumab, rituximab, and cetuximab.Citation15
While our in vivo experiments demonstrate potent anti-tumor efficacy with CD20-CD47 SL that is comparable to combination therapy, they do not address the issue of selectivity in the presence of an antigen sink as CD20-CD47 SL does not cross-react with mouse CD47. One approach to test this hypothesis would be to create transgenic mice that express human CD47 in all cells, but such a strain is not currently available. Alternatively, immunocompromised mice could be engrafted with human hematopoietic cells prior to engraftment with tumor cells and antibody treatment. However, this approach does not lead to engraftment with RBCs that are the primary physiological antigen sink, and mice do not maintain human RBCs after transfusion (data not shown).Citation39 In light of these limitations, the most feasible approach to test this hypothesis would be to create a CD20-CD47 SL DVD-Ig using VH and VL from an anti-CD47 antibody that cross-reacts with mouse CD47. While this reagent may provide insight in proof-of-concept studies, prior observations with DVD-Ig molecules using different antibodies targeting the same antigen demonstrate that binding properties are empiric to each DVD-Ig.Citation33 Thus, findings from such a molecule would not necessarily extrapolate to the CD20-CD47 SL that we have developed. Given these limitations, the in vitro systems that we have used represent the best available strategy to test the hypothesis that CD20-CD47 SL avoids an antigen sink presented by human blood cells that would be prohibitive to combination therapy. Importantly, these experiments demonstrate that CD20-CD47 SL, by nature of its reduced affinity for CD47, binds poorly to RBC () and this property suggests a potential advantage over combination therapy.
The CD20-CD47 SL BsAb developed here is a potential therapeutic for NHL, where targeting of CD20 via rituximab is an established therapy and co-expression of CD20 and CD47 has been reported.Citation11 Our demonstration of the use of a BsAb in the DVD-Ig format to achieve highly selective tumor targeting while retaining CD47 binding is proof of principle for future CD47 targeted therapies that incorporate other tumor antigens. For example, we have demonstrated that BsAbs with similar reduced affinity for CD47 relative to parental antibody can be generated in the DVD-Ig format targeting CD47 and CD33, an antigen expressed on AML cells, thus illustrating the adaptability of this approach to other antigen pairs. (Fig. S1) In addition to its high expression on NHL and AML, CD47 expression has been observed on most human solid tumor types evaluated, including ovarian, bladder, colon, brain, breast, and hepatocellular carcinoma.Citation10,12 There are clinically approved therapeutic antibodies for many of these cancer types, in addition to many other antibodies in clinical development that could be used to construct DVD-Igs.Citation40 We feel it is likely that the approach described here can be used to enhance the specificity and efficacy of most anti-tumor antigen antibodies that engage Fc receptors including trastuzumab, cetuximab, and others. Ultimately, we anticipate that BsAb targeting of CD47 along with tumor-associated antigens will be a viable therapeutic strategy for preferentially inducing the phagocytosis of tumor cells that can be broadly applied to cancer.
Materials and Methods
Bispecific antibody construction and production
The VH and VL of 2B8, huM195, or B6H12.2 were synthesized using custom gene synthesis (MCLAB).Citation41-43 Each VH and VL was subcloned into the pCEP4 mammalian expression vector (Invitrogen) containing the human CH1 or CK genes, respectively, to create the heavy and light chain for expression of B6H12-hIgG1 (anti-CD47), 2B8-hIgG1 (anti-CD20), or huM195-hIgG1 (anti-CD33). To create BsAbs in the DVD-Ig format, the amino terminus of each B6H12 variable domain was fused to the carboxyl terminus of each 2B8 (or huM195) variable domain by overlapping PCR. A linker separating each variable domain was introduced into the PCR primers in this process. The short linker (SL) and long linker (LL) on the light chain are TVAAP and TVAAPSVFIFPP, respectively. On the heavy chain, the SL was ASTKGP and the LL was ASTKGPSVFPLAP. These linkers are derived from the amino termini of human CH1 and human Ck chains. Plasmids containing each chain were co-transfected into Freestyle 293 cells using 293 Fectin (Invitrogen). Antibody was purified from expression media on Protein A Sepharose (GE Healthcare Lifesciences), dialyzed against PBS, and analyzed by 10% SDS-PAGE (Invitrogen) under reducing and non-reducing conditions followed by Coomassie Brilliant Blue staining. Purified antibody was quantified by A280.
Therapeutic antibodies
Rituximab (anti-CD20, human IgG1) was obtained from the Stanford University Medical Center, mouse IgG was purchased from Innovate Research, and B6H12.2 (anti-CD47, mouse IgG1) was described previously.Citation10
Cell lines
The YB2/0, Raji, Daudi, Ramos, ST486, and J774 cell lines were obtained from American Type Culture Collection. Raji cells expressing modified firefly luciferase and eGFP were described previously.Citation11 YB2/0 cells were engineered to stably express human CD20 cDNA (Genecopoeia) using an engineered transposable element.Citation44 YB2/0 cells were engineered to stably express human CD47 by lentiviral transduction with virus generated from human CD47 cDNA (Open Biosystems) in the pCDH backbone (System Biosciences).
Biacore
SPR-based measurements were performed by Biosensor Tools, LLC with a Biacore 2000 instrument. A CM5 sensor chip was coated with an anti-human capturing agent prior to capture of anti-CD47, CD20-CD47 SL, or CD20-CD47 LL. The CD47 antigen was a His-tagged monomer containing the epitope for B6H12 to allow for affinity measurements in the absence of avidity contributions. The antigen was injected over reaction matrices in a 3-fold dilution series. Each sample was injected across the antibody surface for 460 seconds. The association and dissociation rate constant, ka (M−1s−1) and kd (s−1), respectively, were monitored and a KD value was determined.
Xenograft models
Mice were maintained in a barrier facility under the care of the Stanford Veterinary Services Center and handled according to protocols approved by the Stanford University Administrative Panel on Laboratory Animal Care (APLAC protocol number 22264). 1 × 106 luciferase-labeled Raji cells were injected intravenously into the tail vein or subcutaneously into the hind flank of 6 to 10 week old NOD.Cg-PrkdcscidII2rgtm1Wjl/SzJ (NSG) mice. Mice were monitored for engraftment via bioluminescent imaging and daily intraperitoneal injections of 200 μg mouse IgG control, anti-CD47, rituximab, or anti-CD47 antibody + rituximab (200 μg each) were administered for 3 weeks (intravenous model) or 2 weeks (subcutaneous model). Mice treated with CD20-CD47 SL were administered 300, 500, or 1000 μg antibody to account for the increased molecular weight and reduced serum levels of the DVD-Ig molecule relative to conventional antibodies. Mice were monitored with weekly bioluminescent imaging analysis and were followed for overall survival. Luciferase imaging analysis was performed as described previously.Citation11
Human samples
Normal human peripheral blood and salvage red blood cell product were obtained from the Stanford Blood Center. Mononuclear cells were prepared from leukocyte reduction system (LRS) chambers using Ficoll-Paque Plus (GE Healthcare). Human CLL samples () were obtained from patients at the Stanford Medical Center with informed consent, under Stanford IRB # 6453.
Disclosure of Potential Conflicts of Interest
RM is a co-inventor of U.S. Patent No. 8,562,997 entitled “Methods of Treating Acute Myeloid Leukemia by Blocking CD47” and U.S. Patent No. 8,758,750 entitled “Synergistic Anti-CD47 Therapy for Hematologic Cancers.” RM and JL have filed U.S. Patent Application Serial No. 13/675,274 entitled “Humanized and Chimeric Monoclonal Antibodies to CD47.”
Authorship Contributions
ECP, JL, ST, and RM designed the experiments. ECP and RM wrote the manuscript. ECP, SJ, ST, CER, CN, LW, KW, and RM performed experiments and analyzed data.
Supplemental_Material.pdf
Download PDF (1.7 MB)Acknowledgments
The authors acknowledge Adriel Cha, Stephen Willingham, Aaron Ring, and Ryan Corces-Zimmerman for reagents, Feifei Zhao and Melissa Stafford for lab management, and Irv Weissman for discussion.
Funding
ECP is supported by an NIH T32 Ruth L. Kirschstein National Research Service Award (AI07290). RM holds a Career Award for Medical Scientists from the Burroughs Wellcome Fund and is a New York Stem Cell Foundation - Robertson Investigator. This research was supported by a Translational Research Program Award from the Leukemia and Lymphoma Society (RM), funding from the J. Benjamin Eckenhoff Memorial Foundation, and funding from the Virginia and D. K. Ludwig Fund for Cancer Research.
Supplemental Material
Supplemental data for this article can be accessed on the publisher's website.
References
- Weiner LM, Surana R, Wang S. Monoclonal antibodies: versatile platforms for cancer immunotherapy. Nat Rev Immunol 2010; 10:317-27; PMID:20414205; http://dx.doi.org/10.1038/nri2744
- Reichert JM. Which are the antibodies to watch in 2013? MAbs 2013; 5:1-4; PMID:23254906; http://dx.doi.org/10.4161/mabs.22976
- Adams GP, Weiner LM. Monoclonal antibody therapy of cancer. Nat Biotechnol 2005; 23:1147-57; PMID:16151408; http://dx.doi.org/10.1038/nbt1137
- Cheson BD, Leonard JP. Monoclonal antibody therapy for B-cell non-Hodgkin's lymphoma. N Engl J Med 2008; 359:613-26; PMID:18687642; http://dx.doi.org/10.1056/NEJMra0708875
- Brown EJ, Frazier WA. Integrin-associated protein (CD47) and its ligands. Trends Cell Biol 2001; 11:130-5; PMID:11306274; http://dx.doi.org/10.1016/S0962-8924(00)01906-1
- Jiang P, Lagenaur CF, Narayanan V. Integrin-associated protein is a ligand for the P84 neural adhesion molecule. J Biol Chem 1999; 274:559-62; PMID:9872987; http://dx.doi.org/10.1074/jbc.274.2.559
- Seiffert M, Brossart P, Cant C, Cella M, Colonna M, Brugger W, Kanz L, Ullrich A, Buhring HJ. Signal-regulatory protein alpha (SIRPalpha) but not SIRPbeta is involved in T-cell activation, binds to CD47 with high affinity, and is expressed on immature CD34(+)CD38(-) hematopoietic cells. Blood 2001; 97:2741-9; PMID:11313266; http://dx.doi.org/10.1182/blood.V97.9.2741
- Barclay AN, Brown MH. The SIRP family of receptors and immune regulation. Nat Rev Immunol 2006; 6:457-64; PMID:16691243; http://dx.doi.org/10.1038/nri1859
- Tsai RK, Discher DE. Inhibition of “self” engulfment through deactivation of myosin-II at the phagocytic synapse between human cells. J Cell Biol 2008; 180:989-1003; PMID:18332220; http://dx.doi.org/10.1083/jcb.200708043
- Majeti R, Chao MP, Alizadeh AA, Pang WW, Jaiswal S, Gibbs KD, Jr., van Rooijen N, Weissman IL. CD47 is an adverse prognostic factor and therapeutic antibody target on human acute myeloid leukemia stem cells. Cell 2009; 138:286-99; PMID:19632179; http://dx.doi.org/10.1016/j.cell.2009.05.045
- Chao MP, Alizadeh AA, Tang C, Myklebust JH, Varghese B, Gill S, Jan M, Cha AC, Chan CK, Tan BT, et al. Anti-CD47 antibody synergizes with rituximab to promote phagocytosis and eradicate non-Hodgkin lymphoma. Cell 2010; 142:699-713; PMID:20813259; http://dx.doi.org/10.1016/j.cell.2010.07.044
- Willingham SB, Volkmer JP, Gentles AJ, Sahoo D, Dalerba P, Mitra SS, Wang J, Contreras-Trujillo H, Martin R, Cohen JD, et al. The CD47-signal regulatory protein alpha (SIRPa) interaction is a therapeutic target for human solid tumors. Proc Natl Acad Sci U S A 2012; 109:6662-7; PMID:22451913; http://dx.doi.org/10.1073/pnas.1121623109
- Chao MP, Alizadeh AA, Tang C, Jan M, Weissman-Tsukamoto R, Zhao F, Park CY, Weissman IL, Majeti R. Therapeutic antibody targeting of CD47 eliminates human acute lymphoblastic leukemia. Cancer Res 2011; 71:1374-84; PMID:21177380; http://dx.doi.org/10.1158/0008-5472.CAN-10-2238
- Jaiswal S, Jamieson CH, Pang WW, Park CY, Chao MP, Majeti R, Traver D, van Rooijen N, Weissman IL. CD47 is upregulated on circulating hematopoietic stem cells and leukemia cells to avoid phagocytosis. Cell 2009; 138:271-85; PMID:19632178; http://dx.doi.org/10.1016/j.cell.2009.05.046
- Weiskopf K, Ring AM, Ho CC, Volkmer JP, Levin AM, Volkmer AK, Ozkan E, Fernhoff NB, van de Rijn M, Weissman IL, et al. Engineered SIRPalpha Variants as Immunotherapeutic Adjuvants to Anticancer Antibodies. Science 2013. 341:88-91 ; PMID:23722425; http://dx.doi.org/10.1126/science.1238856
- Theocharides AP, Jin L, Cheng PY, Prasolava TK, Malko AV, Ho JM, Poeppl AG, van Rooijen N, Minden MD, Danska JS, et al. Disruption of SIRPalpha signaling in macrophages eliminates human acute myeloid leukemia stem cells in xenografts. J Exp Med 2012; 209:1883-99; PMID:22945919; http://dx.doi.org/10.1084/jem.20120502
- Chao MP, Weissman IL, Majeti R. The CD47-SIRPalpha pathway in cancer immune evasion and potential therapeutic implications. Curr Opin Immunol 2012; 24:225-32; PMID:22310103; http://dx.doi.org/10.1016/j.coi.2012.01.010
- Winiarska M, Glodkowska-Mrowka E, Bil J, Golab J. Molecular mechanisms of the antitumor effects of anti-CD20 antibodies. Front Biosci 2011; 16:277-306; PMID:21196171; http://dx.doi.org/10.2741/3688
- Zhao XW, van Beek EM, Schornagel K, Van der Maaden H, Van Houdt M, Otten MA, Finetti P, Van Egmond M, Matozaki T, Kraal G, et al. CD47-signal regulatory protein-alpha (SIRPalpha) interactions form a barrier for antibody-mediated tumor cell destruction. Proc Natl Acad Sci U S A 2011; 108:18342-7; PMID:22042861; http://dx.doi.org/10.1073/pnas.1106550108
- Muller D, Kontermann RE. Bispecific antibodies for cancer immunotherapy: Current perspectives. BioDrugs 2010; 24:89-98; PMID:20199124; http://dx.doi.org/10.2165/11530960-000000000-00000
- May C, Sapra P, Gerber HP. Advances in bispecific biotherapeutics for the treatment of cancer. Biochem Pharmacol 2012; 84:1105-12; PMID:22858161; http://dx.doi.org/10.1016/j.bcp.2012.07.011
- Fischer N, Leger O. Bispecific antibodies: molecules that enable novel therapeutic strategies. Pathobiology 2007; 74:3-14; PMID:17496428; http://dx.doi.org/10.1159/000101046
- Marvin JS, Zhu Z. Recombinant approaches to IgG-like bispecific antibodies. Acta Pharmacol Sin 2005; 26:649-58; PMID:15916729; http://dx.doi.org/10.1111/j.1745-7254.2005.00119.x
- Holliger P, Prospero T, Winter G. Diabodies: small bivalent and bispecific antibody fragments. Proc Natl Acad Sci U S A 1993; 90:6444-8; PMID:8341653; http://dx.doi.org/10.1073/pnas.90.14.6444
- Deyev SM, Lebedenko EN. Multivalency: the hallmark of antibodies used for optimization of tumor targeting by design. Bioessays 2008; 30:904-18; PMID:18693269; http://dx.doi.org/10.1002/bies.20805
- Cheong HS, Chang JS, Park JM, Byun SM. Affinity enhancement of bispecific antibody against two different epitopes in the same antigen. Biochem Biophys Res Commun 1990; 173:795-800; PMID:1702631; http://dx.doi.org/10.1016/S0006-291X(05)80857-5
- Neri D, Momo M, Prospero T, Winter G. High-affinity antigen binding by chelating recombinant antibodies (CRAbs). J Mol Biol 1995; 246:367-73; PMID:7533215; http://dx.doi.org/10.1006/jmbi.1994.0091
- Lu D, Kotanides H, Jimenez X, Zhou Q, Persaud K, Bohlen P, Witte L, Zhu Z. Acquired antagonistic activity of a bispecific diabody directed against two different epitopes on vascular endothelial growth factor receptor 2. J Immunol Methods 1999; 230:159-71; PMID:10594363; http://dx.doi.org/10.1016/S0022-1759(99)00135-0
- Dorvillius M, Garambois V, Pourquier D, Gutowski M, Rouanet P, Mani JC, Pugniere M, Hynes NE, Pelegrin A. Targeting of human breast cancer by a bispecific antibody directed against two tumour-associated antigens: ErbB-2 and carcinoembryonic antigen. Tumour Biol 2002; 23:337-47; PMID:12677091; http://dx.doi.org/10.1159/000069793
- Robinson MK, Hodge KM, Horak E, Sundberg AL, Russeva M, Shaller CC, von Mehren M, Shchaveleva I, Simmons HH, Marks JD, et al. Targeting ErbB2 and ErbB3 with a bispecific single-chain Fv enhances targeting selectivity and induces a therapeutic effect in vitro. Br J Cancer 2008; 99:1415-25; PMID:18841159; http://dx.doi.org/10.1038/sj.bjc.6604700
- Beers SA, Chan CH, French RR, Cragg MS, Glennie MJ. CD20 as a target for therapeutic type I and II monoclonal antibodies. Semin Hematol 2010; 47:107-14; PMID:20350657; http://dx.doi.org/10.1053/j.seminhematol.2010.01.001
- Wu C, Ying H, Grinnell C, Bryant S, Miller R, Clabbers A, Bose S, McCarthy D, Zhu RR, Santora L, et al. Simultaneous targeting of multiple disease mediators by a dual-variable-domain immunoglobulin. Nat Biotechnol 2007; 25:1290-7; PMID:17934452; http://dx.doi.org/10.1038/nbt1345
- Wu C, Ying H, Bose S, Miller R, Medina L, Santora L, Ghayur T. Molecular construction and optimization of anti-human IL-1alpha/beta dual variable domain immunoglobulin (DVD-Ig) molecules. MAbs 2009; 1:339-47; PMID:20068402; http://dx.doi.org/10.4161/mabs.1.4.8755
- Ernst JA, Li H, Kim HS, Nakamura GR, Yansura DG, Vandlen RL. Isolation and characterization of the B-cell marker CD20. Biochemistry 2005; 44:15150-8; PMID:16285718; http://dx.doi.org/10.1021/bi0511078
- Binder M, Otto F, Mertelsmann R, Veelken H, Trepel M. The epitope recognized by rituximab. Blood 2006; 108:1975-8; PMID:16705086; http://dx.doi.org/10.1182/blood-2006-04-014639
- Khandelwal S, van Rooijen N, Saxena RK. Reduced expression of CD47 during murine red blood cell (RBC) senescence and its role in RBC clearance from the circulation. Transfusion 2007; 47:1725-32; PMID:17725740; http://dx.doi.org/10.1111/j.1537-2995.2007.01348.x
- Shultz LD, Lyons BL, Burzenski LM, Gott B, Chen X, Chaleff S, Kotb M, Gillies SD, King M, Mangada J, et al. Human lymphoid and myeloid cell development in NOD/LtSz-scid IL2R gamma null mice engrafted with mobilized human hemopoietic stem cells. J Immunol 2005; 174:6477-89; PMID:15879151; http://dx.doi.org/10.4049/jimmunol.174.10.6477
- Jaiswal S, Chao MP, Majeti R, Weissman IL. Macrophages as mediators of tumor immunosurveillance. Trends Immunol 2010; 31:212-9; PMID:20452821; http://dx.doi.org/10.1016/j.it.2010.04.001
- Doulatov S, Notta F, Laurenti E, Dick JE. Hematopoiesis: a human perspective. Cell Stem Cell 2012; 10:120-36; PMID:22305562; http://dx.doi.org/10.1016/j.stem.2012.01.006
- Reichert JM. Antibodies to watch in 2013: Mid-year update. MAbs 2013; 5:513-7; PMID:23727858; http://dx.doi.org/10.4161/mabs.24990
- Reff ME, Carner K, Chambers KS, Chinn PC, Leonard JE, Raab R, Newman RA, Hanna N, Anderson DR. Depletion of B cells in vivo by a chimeric mouse human monoclonal antibody to CD20. Blood 1994; 83:435-45; PMID:7506951
- Gresham HD, Goodwin JL, Allen PM, Anderson DC, Brown EJ. A novel member of the integrin receptor family mediates Arg-Gly-Asp-stimulated neutrophil phagocytosis. J Cell Biol 1989; 108:1935-43; PMID:2785522; http://dx.doi.org/10.1083/jcb.108.5.1935
- Caron PC, Co MS, Bull MK, Avdalovic NM, Queen C, Scheinberg DA. Biological and immunological features of humanized M195 (anti-CD33) monoclonal antibodies. Cancer Res 1992; 52:6761-7; PMID:1458463
- Huang X, Wilber AC, Bao L, Tuong D, Tolar J, Orchard PJ, Levine BL, June CH, McIvor RS, Blazar BR, et al. Stable gene transfer and expression in human primary T cells by the Sleeping Beauty transposon system. Blood 2006; 107:483-91; PMID:16189271; http://dx.doi.org/10.1182/blood-2005-05-2133