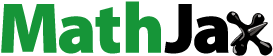
Abstract
Fragmentation in the hinge region of an IgG1 monoclonal antibody (mAb) can affect product stability, potentially causing changes in potency and efficacy. Metals ions, such as Cu2+, can bind to the mAb and undergo hydrolysis or oxidation, which can lead to cleavage of the molecule. To better understand the mechanism of Cu2+-mediated mAb fragmentation, hinge region cleavage products and their rates of formation were studied as a function of pH with and without Cu2+. More detailed analysis of the chemical changes was investigated using model linear and cyclic peptides (with the sequence of SCDKTHTC) derived from the upper hinge region of the mAb. Cu2+ mediated fragmentation was determined to be predominantly via a hydrolytic pathway in solution. The sites and products of hydrolytic cleavage are pH and strain dependent. In more acidic environments, rates of Cu2+ induced hinge fragmentation are significantly slower than at higher pH. Although the degradation reaction rates between the linear and cyclic peptides are not significantly different, the products of degradation vary. mAb fragmentation can be reduced by modifying His, which is a potential metal binding site and a known ligand in other metalloproteins. These results suggest that a charge may contribute to stabilization of a specific molecular structure involved in hydrolysis, leading to the possible formation of a copper binding pocket that causes increased susceptibility of the hinge region to degradation.
Abbreviations
ATCUN | = | amino terminal copper and nickel |
BSA | = | bovine serum albumin |
ACM | = | acetaminomethyl |
mAbs | = | monoclonal antibodies |
Introduction
Monoclonal antibodies (mAbs) are protein therapeutic molecules widely used for the treatment of a range of life-threatening diseases, including oncology (cetuximab, trastuzumab), inflammatory diseases (adalimumab, rituximab), and rare “orphan” disease indications (eculizumab for paroxysmal nocturnal hemoglobinuria).Citation1 IgG1 mAbs contain heterogeneity in size and charge generated during cell culture, purification, and processing and can accrue a variety of degradation products over storage.Citation2 During product development, characterization and monitoring of molecular attributes are necessary to demonstrate manufacturing consistency and shelf-life prediction to ensure a potent and safe drug product.Citation3
mAb fragmentation, or cleavage of the peptide backbone typically by hydrolysis, is a degradation process that occurs in a liquid drug product formulation. In particular, the IgG1 mAb hinge region of the heavy chain is prone to cleavage due to limited structural constraints and high solvent accessibility.Citation4 This highly conserved hinge consists of 3 regions: upper, core, and lower.Citation5 Fragmentation has been shown under solution storage to be typically confined to the upper hinge sequence,Citation6,7 i.e., SC220DKTHTC (Eu numberingCitation8), which is linked to the light and inter-heavy chains through disulfide bonds at Cys220 and Cys226, respectively.
Treatment of a mAb with enzymes such as papain and trypsin cleaves within the hinge region between the His-Thr bondCitation9 and Lys-Thr or Lys-Asp,Citation10 respectively, with the latter reaction influenced by nearby Asp residues.Citation11 Non-enzymatic fragmentation, on the other hand, can be observed by direct hydrolysis,Citation6 β-elimination,Citation7 and free-radical catalysis of peptide bond cleavage in mAbs,Citation12 and metal-mediated oxidative cleavage in peptides.Citation13 Fragmentation kinetics by non-enzymatic methods has been shown to vary with pH; pH 6 has the lowest rate of cleavage and rates increase in both more acidic and basic conditions,Citation14 and storage temperature.Citation15 Importantly, amino acids in the hinge such as His can facilitate fragmentation.Citation16
In general, the peptide bond is inherently resistant to hydrolysis, with a half-life of up to 267 y at 37°C and 350 y at 25°C, as determined by constructing pH-rate profiles with Gly-Val and Gly-Gly peptides studies, respectively, in uncatalyzed reactions at pH 7.Citation17,18 Metal ions can enhance the rate of peptide cleavage and catalyze reactions when structurally positioned in the proper conformation for specific stereochemistry to occur.Citation19-23 A binding pocket, or active site, mediated by specific atoms within the amide bond and side chain moieties of residues can facilitate high-affinity binding and metal-dependent chemical reactivity.Citation24
Cu2+ is known to form strong complexes with organic molecules due to their relatively high electron affinity,Citation25 especially with His and amide nitrogens.Citation26 In effect, Cu2+ can bind and enhance the degradation of proteins such as mAbs and hydrolyze small peptides and BSA.Citation27 Despite potential degradation reactions, Cu2+ is intentionally added as a component during mAb manufacture in the cell culture process to help maintain cell viability and to improve mAb titers.Citation28 Although most large-scale purification processes are able to remove most metals, trace amounts of Cu2+ (e.g., 15 ppb) may be sufficient to enable site-specific, metal-mediated mAb degradation.Citation29 Unintentionally, Cu2+ is introduced as an impurity often found in buffer components such as sodium chloride.Citation29 Previous work showed evidence of Cu2+-mediated hinge cleavage of the mAb Campath 1H (alemtuzumab) in slightly alkaline pH.Citation30 The rate of cleavage was reduced in slightly acidic conditions (pH < 6) and was accelerated by increasing concentrations of cupric ion and higher temperatures.Citation30 With the addition of EDTA, the reaction was completely inhibited, which confirmed the involvement of metal ions in the cleavage. Allen et al. studied several peptides with the hinge sequence DKTHT at various pH values. They observed that the rate of the reaction was slow below pH 5 and increased gradually from pH 7 to 9.5. Hydrolysis primarily occurred at the Lys-Thr peptide bond,Citation31 which is consistent with the trypsin cleavage site. Allen et al. deliberately eliminated cysteine residues from the peptide to avoid non-native reactivity with the free cysteine sulfhydryl groups in the presence of Cu2+.
In this paper the dependence of a mAb to undergo fragmentation in the hinge region in the presence of Cu2+ was demonstrated and the mechanism of cleavage was further explored using model peptides derived from the hinge sequence. Since His is known to bind to metal ions, including Cu2+, peptides with a His to Ser mutant and an IgG1 with H224S mutant in the hinge region were made and stability studies were conducted in the presence of Cu2+. Typically, mutational substitutions are made with small hydrophobic residues (e.g., Ala) to remove the effects of the side chain.Citation32 In our work, however, Ser was substituted for His to better understand the effect of hinge fragmentation mediated by another metal binding amino acid that possibly influences the Cu2+ coordination geometry.
Results
IgG1 mAb hinge region fragmentation in the presence of Cu2+ was studied in detail in the pH range of 5–7 using either peptide models or a full length IgG1. Hinge model peptides enabled the examination of site-specific interactions of hinge residues with Cu2+ without the complexities of a full-length mAb. The initial rates and pH dependence of degradation for the peptide were determined in the absence and presence of Cu2+. A linear peptide (SCDKTHTC) with protected Cys () that mimics the upper hinge region was used. To determine the effect of conformational rigidity on Cu2+-mediated hinge fragmentation, degradation of a cyclized peptide, in which the 2 Cys participate in an intramolecular disulfide linkage, also was examined (). While a linear peptide closely resembles the primary sequence of the upper hinge, the embedded Cys in disulfide bonds can limit flexibility in the region. Evaluation of a structurally rigid model, such as a cyclic peptide, offers the opportunity to help understand the role of strain on fragmentation. Because Cu2+ is a redox active metal capable of oxidative reactions, Cu2+-mediated fragmentation of the hinge peptide was considered with respect to both a hydrolytic and oxidative pathway. Based on the site and pH specific fragmentation presented herein, a Cu2+ binding site within the IgG1 hinge region and a hydrolytic cleavage mechanism is proposed.
Figure 1. Model hinge peptides. (A) Linear peptide with N-terminal acetylation, C-terminal amidation, and *Cys blocked with acetaminomethyl (ACM) groups. (B) Cys deproptected and cyclized peptide.
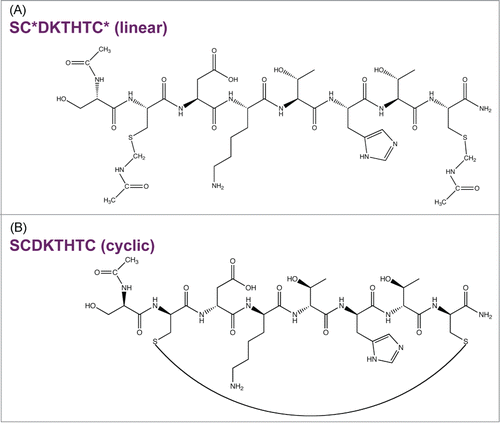
mAb 1 degradation in the presence of Cu2+
mAb 1 was treated with Cu2+ in sodium acetate or histidine acetate buffer at pH 5.3, stored at 40°C for up to 5 weeks, and monitored by SEC to detect fragmentation in the hinge region. The SEC chromatographic profile of mAb 1 in sodium acetate buffer containing various Cu2+ concentrations is shown in . The identity of these SEC peaks for mAb 1 were previously determined using mass spectrometry.Citation33 Addition of Cu2+ increased the rate of mAb 1 fragmentation as shown in , resulting in generation of the Fab arm and the single-arm antibody (Fab + Fc) from the parent mAb 1. Increased fragmentation with the addition of Cu2+ to mAb 1 in sodium acetate buffer was clearly observed and was not detected when formulated with histidine acetate buffer (). The increase in total fragmentation (sum of peaks C and D) was tracked as a function of time to quantify the rate of degradation by linear regression (). In the presence of Cu2+, mAb 1 in sodium acetate buffer exhibited significantly more fragmentation than in histidine acetate. In sodium acetate buffer, the increase in molar ratio of Cu2+: mAb 1 to 1 : 9, 1 : 4, or 1 : 2 resulted in an approximate 5-, 6-, or 8-fold increase in fragmentation, respectively, as compared to the control (no Cu2+, mAb 1 only). In contrast, mAb 1 in histidine buffer plus 0.038 or 0.15 mM Cu2+ showed an approximate 2 to 3 fold increase in fragmentation as compared to the control ().
Figure 2. SEC chromatographic profile of IgG1 mAb 1 fragments in sodium acetate pH 5.3 in various copper concentrations after 1 wk at 40°C as detected by absorbance at 280 nm. (A) High molecular weight species, (B) monomer, (C) fragment missing Fab arm (Fc + Fab), (D) Fab arm.
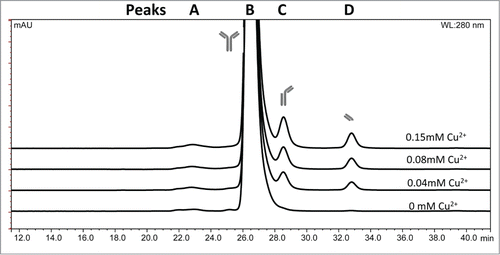
Figure 3. SEC analysis of total fragment formation for mAb ± Cu2+ during 40 °C storage at pH 5.3 in (A) sodium acetate or (B) histidine acetate.
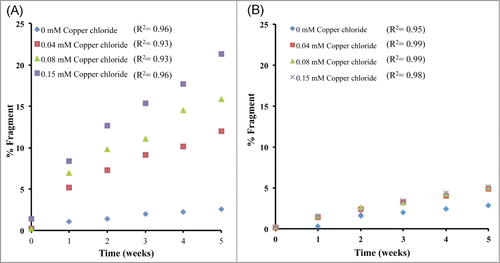
Table 1. Linear regression fit of the initial fragmentation rates for mAb 1 ± Cu2+ at 40°C up to 5 weeks determined using SEC (*Total fragments = Peaks C + D as shown in )
Peptide degradation rates in the presence of Cu2+
To better understand hinge fragmentation of the mAb in the presence of Cu2+, a linear octapeptide derived from the upper hinge region, SC*DKTHTC* (; *acetaminomethyl (ACM) protecting group on Cys to block thiol reactivity) was synthesized and studied at pH 5, 6, and 7. Loss of the parent (intact) linear peptide incubated in the presence of Cu2+ at 50°C was monitored using RP-HPLC to determine the initial rate of intact peptide loss by linear regression. The loss of the linear and cyclic intact peptide resulted in a heterogeneous mixture of degradation products. The linear peptide showed the lowest rate of peptide loss at pH 5 (). As the pH increased, the rate loss of the intact peptide also increased. The initial rates of intact peptide loss for 0.15 mM Cu2+ to 0.22 mM peptide at pH 5, 6, and 7 were approximately 3, 53, and 47-fold higher respectively than the corresponding control (no Cu2+) reaction.
Table 2. Initial peptide degradation rates at 50°C. Results for no Cu2+ and + Cu2+
To identify the degradation products of the linear peptide in sodium acetate at pH 5, RP-HPLC and RP-HPLC- MS were performed. At pH 5, species with masses of 948-, 702-, and 1076-Da are detected beneath peak 1, 2, and 3, respectively ( and ). The 948-Da species suggests a +32 oxidation product of the intact peptide with the formation of a succinimide ring at the Asp residue, while the 702-Da species is the cleavage fragment DKTHTC, and the 1076-Da species is an Asp isomer of the intact peptide (Fig. S1). A minor amount of dehydroalanine (MW = 900 Da) was also observed beneath peak 1 (MS data not shown). The +32 products of the intact peptide were observed when the Cys blocking groups were disassociated from the peptide and the Cys thiol groups get oxidized. Cys oxidation reaction commonly occurs in proteins as reported by Giles et al.Citation34 The decoupling reaction between the blocking group and Cys are not well understood and will need further investigation. Detection of the DKTHTC peptide fragment (MW = 702 Da) confirms that cleavage occurs on the N-terminal side of Asp, suggesting a hydrolytic reaction is responsible for cleavage of the Cys-Asp bond. A more hydrophilic peak was observed at 10.0 minutes (peak 3), which corresponds to a 1076-Da species (Fig. S1). This is the same mass as the intact peptide and is expected to be SC*isoDKTHTC*, which is a result of isomerization at the Asp residue. As expected, the change in the intensity of this peak was dependent on temperature and pH, but independent of the presence of Cu2+ (data not shown).
Figure 4. RP-HPLC chromatographic profiles of hinge peptides showing Cu2+-induced degradation at 50°C, as detected by absorbance at 214 nm, in either pH 5 sodium acetate buffer or pH 7 HEPES buffer. (A) Linear pH 5, 240 h, (B) linear pH 7, 96 h, (C) cyclic pH 5, 216 h, and (D) cyclic pH 7, 100 h. Time points were selected to show adequate formation of degradation peaks because the rate and extent of degradation are pH specific. Similar chromatographic profiles are observed at pH 6 as compared to pH 7 for the linear peptide under similar conditions. The number of each labeled peak corresponds to that identified in .
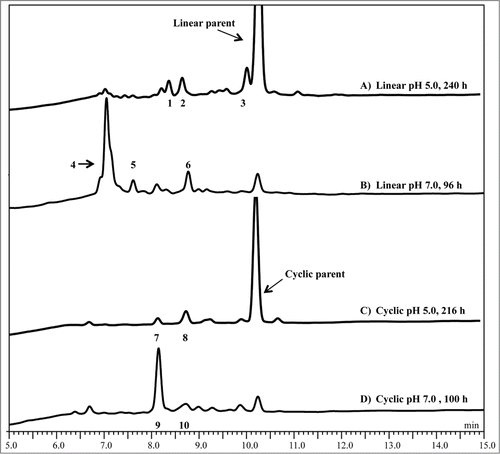
Table 3. Identification by RP-HPLC-MS of the linear and cyclic peptides treated with Cu2+
Formation of the degradation products is Cu2+ dependent at pH 7 in HEPES buffer when incubated at 50°C and the results are summarized in . At pH 7, the major degradation products were the THTC* (530 Da) and SC*DK (564 Da) fragments detected beneath peaks 4 and 5, respectively ( and S2). The hydrolytic cleavage site between Lys and Thr was consistent with previous Cu2+-induced hinge region degradation studies at slightly alkaline pH.Citation30 Peak 6 contains a 773-Da species, which is most likely the C-terminal fragment DKTHTC* or DKTHTC modified with a pyruvoyl group (pDKTHTC, Fig. S2). Furthermore, a small amount of a 900-Da species also eluted with peak 6, suggesting the formation of a dehydroalanine derivative (i.e., SdADKTHTC, data not shown). The observation of these species is consistent with a single chemistry; both species are intermediate products of beta elimination at either of the Cys residues.Citation7 Similar products were observed at pH 6 in MES (data not shown).
A deprotected, cyclized version of the hinge peptide, linked by an intramolecular disulfide bridge, was also prepared to assess the impact of molecular conformation, in the form of a more rigid and less flexible model, on the stability in similar conditions as the linear peptide (). In presence of 0.15 mM Cu2+, initial rates for loss of the intact cyclic peptide are slightly lower compared to the linear peptide at pH 5 and 7 (). The degradation products of the linear and the cyclic peptides, however, are distinctly different and summarized in . At pH 5, two new peaks 7 and 8 were observed when the cyclic peptide was incubated in presence of 0.15 mM Cu2+ (). In the absence of Cu2+ the rate of intact peptide loss was extremely low (data not shown). Peak 7 contains a +18 Da product of the intact peptide (950 Da), while the peak 8 contains a species with the same mass as the cyclic intact peptide (932 Da, Fig. S3A). Similarly, at pH 7 two peaks were observed that correspond to a 950-Da (peak 9) and a 932-Da (peak 10) species (, mass spectrum not shown). These peaks were determined by MS to be the same products as at pH 5, such that the peaks 7 and 8 were analogous to the peaks at 9 and 10 at pH 7. Interestingly, the Asp related N-terminal hydrolysis and isomerization products generated when the linear peptide was incubated in presence of Cu2+ under similar conditions were not observed with the cyclic peptide. The +18-Da species was further analyzed using LC-MS/MS to confirm that Cu2+ induced a hydrolytic degradation between Lys and Thr at both pH 5 and pH 7, in contrast to what was observed in the reactions of Cu2+ with the linear peptide. To confirm the cleavage was indeed between Lys-Thr, a tryptic digest of the cyclic peptide was performed followed by LC-MS/MS. The results indicate the formation of the same product (950-Da species), which further validates the Cu2+ reaction leads to cleavage between the Lys-Thr (Fig. S3B).
The influence of the histidine-224 on hinge region stability
To investigate the importance of the His224 in the hinge for binding Cu2+, a peptide variant (His to Ser) was synthesized and stability studies were conducted. Slower degradation of the main peak with the variant peptide compared to the wild-type peptide was observed (data not shown). To confirm whether these linear peptide results translate to the full-length antibody, an IgG1 mAb (mAb 2 wt) with the hinge region His mutated to Ser (mAb 2 H224S) was also studied for hinge fragmentation. mAb 2 wt and mAb 2 H224S were subjected to 40°C storage up to 2 weeks with and without Cu2+ in sodium acetate buffer at pH 5.3. The relative rates of degradation for mAb 2 wt and mAb 2 H224S are shown in . In the presence of Cu2+, mAb 2 H224S showed a 3-fold decrease in the rate of fragmentation compared to mAb 2 wt. mAb 2 H224S with and without Cu2+ and mAb 2 wt without Cu2+ showed virtually no change up to 7 d. However an increase in fragmentation was noticed thereafter in these samples.
Figure 5. Plot of the total fragment data for mAb 2 and mAb 2 H224S, as determined by SEC. Samples were incubated in 20 mM sodium acetate, pH 5.3, in the absence or presence of 0.04 mM Cu2+ and stored at 40°C. Aliquots were examined at various time points for up to 2 weeks. The R2 values for the mAb 2 wt and mAb 2 H224S incubated with Cu2+ are 0.97 and 0.84 and without Cu2+ are 0.78 and 0.80, respectively.
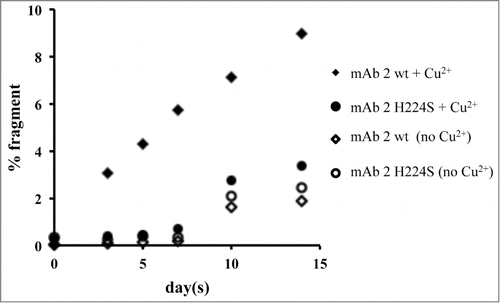
Cu2+ binding pocket in IgG1 mAbs
Given the site-specific hinge fragmentation observed, a binding pocket that presents additional ligands to accomplish metal coordination in an appropriate geometry for Cu2+ binding is expected. Examination of the crystal structure of an IgG1 (PDB: 1HZH)Citation35 revealed that the upper hinge region sequence containing the peptide sequence studied herein adopts the appropriate geometry and distances to accommodate binding Cu2+ in a square planar organization and is shown in .
Figure 6. Model of Cu2+ bound to the DKTH sequence of the hinge region in IgG1. Square planar geometry of the Cu2+ ion (purple circle) is expected based on other copper-binding peptides and the crystal structure of IgG1 (PDB: 1HZH)Citation35, which shows these residues are poised to accommodate square planar coordination via the backbone amide nitrogens from Lys, Thr and His and the imidazole N from the His. Image created using PyMOL.Citation45
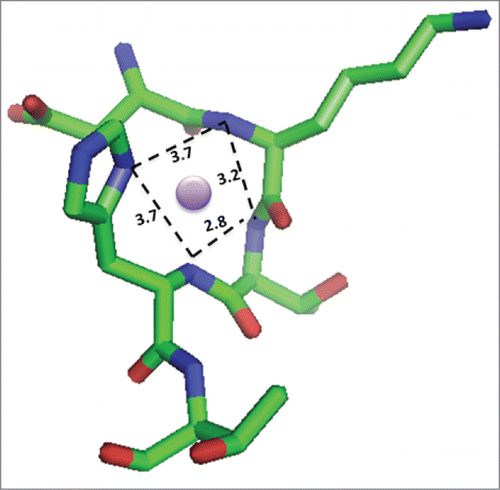
The impact of reactive oxidative species on the hinge region
To test whether a free radical is responsible for cleavage of the hinge region, the Cu2+-treated linear peptide was incubated with and without the hydroxyl radical scavenger mannitol in sodium acetate buffer at pH 5.3. The RP-HPLC results show a similar degradation pattern for both samples (Fig. S4). To demonstrate that the reaction was not attributed to single electron transfer to Cu2+, a bathocuproine binding assay was also performed.Citation36 Incubation of the peptide in the presence 0.15 mM Cu2+ at T0 and 60°C for 24 hours resulted in 1 and 22 µM Cu1+, respectively, indicating no appreciable amount of Cu2+ reduced to Cu1+. Subsequently, the Amplex Red assay showed very little formation of H2O2 (2.9 and 1.0 µM H2O2for the peptide at T0 and after 24 hours treated with 150 µM Cu2+, respectively), indicating that the reduction of Cu2+ to Cu1+ and subsequent formation of H2O2 is not a predominant pathway during the degradation of the hinge peptide.
Discussion
Allen et al have previously shown that the hinge peptide lacking a Cys residue undergoes Cu2+ mediated fragmentation at pH 7 and higher. We were interested in understanding the fragmentation patterns in the pH range of 5–7 because most of the mAbs in clinical development are formulated in this pH range. We also investigated the role of Cys and the role of strain in this fragmentation chemistry. Since Cu2+ is a redox active metal ion, we wanted to test the possibility of both hydrolytic and oxidative fragmentation chemistry. As the Cu2+-mediated fragmentation was site specific, we propose a metal binding site within the hinge region of the IgG1 mAb.
A species corresponding to a DKTHTC fragment is formed due to a direct hydrolysis of the Cys-Asp bond when the linear peptide is incubated in presence of Cu2+ at pH 5. The Asp residue in peptides is known to be more susceptible to hydrolysis than other amino acids, especially under mildly acidic conditions.Citation37 Direct hydrolysis at the N-terminal side of Asp likely proceeds by a nucleophilic attack on the carbonyl of the n−1 amino acid residue (i.e., Cys), resulting in formation of a 6-membered anhydride ring intermediate, followed by hydrolytic cleavage of the bond between Cys-Asp, as shown in . Hydrolysis at the N-terminal side of Asp would then form the DKTHTC fragment. Since no pyruvoyl was detected at pH 5, the degradation pathway that formed the DKTHTC fragment most likely did not proceed via beta elimination through formation of dehydroalanine followed by further hydrolysis to form a pyruvoyl group. The reaction of Cys to dehydroalanine is typically driven at higher pH, such as pH 8.Citation7 However, trace amounts of a dehydroalanine species were observed even at pH 6 in our studies, indicating the presence of Cu2+ has an influence on the formation of this degradation product even at lower pH. The generation of dehydroalanine and pyruvoyl groups in the presence of Cu2+ at pH greater than 6 indicated that the Cys residues play an important role in the degradation of the linear peptide.
Scheme 1. Proposed mechanisms for fragmentation of the linear peptide at pH 5 showing direct hydrolysis facilitated by the Asp side chain.
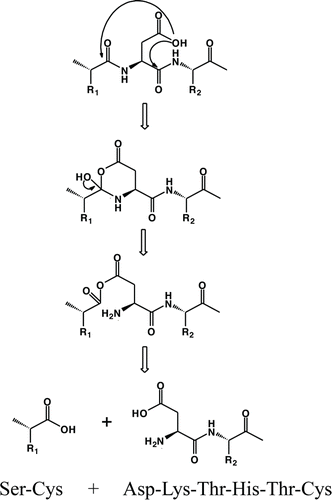
Iso-Asp formation is observed at pH 5 in the presence of Cu2+. At pH 6 and 7, no isomer peak was observed in the presence of Cu2+, and instead degradation of the linear peptide yielded SCDK and a substantial THTC fragment. The cleavage sites were consistent with previous studies and confirmed by MS results in an IgG1Citation30,38 and other peptides.Citation31 This cleavage could occur via 2 different pathways as shown in . Cu2+ may coordinate to the peptide via the histidyl nitrogen and 2 peptide amide nitrogens to facilitate cleavage on the N-terminal side of Thr in an intramolecular hydroxyl catalyzed reaction as shown in Pathway A. Here, an O-acyl intermediate would be formed between the Thr and the n−1 carbonyl carbon followed by hydrolysis of the ester. Alternatively, in Pathway B, general acid catalysis by the Cu2+-bound water can occur without the involvement of the Thr hydroxyl group. Mutant studies by Allen et al. showed with the parent sequence FDKTHY that substitution of the Thr with Ala or Gly lowered the rate of cleavage compared to the parent, suggesting that the Thr-mediated intramolecular hydroxyl-catalyzed pathway was more likely than a general acid-catalyzed reaction to be responsible for hinge hydrolysis.Citation31 Thr or Ser can polarize the peptide bond via coordination and intramolecular attack of the hydroxyl group on the carbonyl carbon to form an ester intermediate in this N to O acyl rearrangement reaction.Citation19 Studies with Cu2+ in myoglobin showed highly selective cleavage in the sequence QSHAT, where only the peptide bonds on the N-terminal side of the Ser and Thr residues were hydrolyzed.Citation39 Overall from our linear peptide studies, it is clear that at the slightly acidic pH (i.e., pH 5), the major cleavage site was the Cys-Asp bond (), whereas at pH 6 and 7, the Lys-Thr bond is cleaved.
Previously, Bogdanowich-Knipp et al. demonstrated the impact of peptide cyclization and its effect in solution stability.Citation40 The authors conducted studies using a cyclic RGD peptide at 50°C that showed a marked increase in solution stability over the range of pH 3–7 as compared to the linear counterpart, with up to a 30-fold improvement at pH 7. The lack of flexibility in the cyclized peptide prevents Asp-mediated fragmentation via the carboxylic acid side chain to cleave the peptide backbone. In the cyclic hinge peptide, a single product with mass of +18 Da was observed at pH 5 and 7 in the presence of Cu2+ in our studies. This differs from the linear peptide where pH altered the degradation products and suggests that conformation rather than the pH in this range has a larger influence on the cleavage reaction. The +18-Da products were characterized as a hydrolysis reaction between Lys-Thr in the cyclic peptide and confirmed using trypsin digest. Cyclization of the peptide increased the structural rigidity and interestingly prevented the attack by the Asp residue to mediate peptide backbone cleavage. As such, the susceptibility of the Lys-Thr site becomes dominant. After the Cu2+-mediated fragmentation of the cyclic peptide results in SCDK and THTC fragments, and the resultant release of conformational strain, a succinimide ring product (−18 Da) was observed at both pH 5 and pH 7. Such a succinimide ring was not observed when control experiments without Cu2+ were carried out for extended periods of time in similar buffer systems (data not shown). Data generated using the cyclic peptide indicates that conformation plays a significant role in the Cu2+-mediated fragmentation, mostly via a hydrolytic pathway.
Cu2+ is a redox active metal ion that has been implicated in both hydrolytic as well as oxidative cleavage of peptide bonds. The one-electron reduction of Cu2+ to Cu1+ in the presence of a reducing agent and molecular oxygen can lead to the generation of various reactive oxygen species (ROS; equations Equation1–4).Citation41 The generation of Cu1+ can be detected using the bathocuproine assay, while the generation of H2O2 can be detected using the Amplex assay.(Eq.\, 1)
(Eq.\, 1)
(Eq.\, 2)
(Eq.\, 2)
(Eq.\, 3)
(Eq.\, 3)
(Eq.\, 4)
(Eq.\, 4)
Additionally, Cu2+ in presence of hydrogen peroxide can generate hydroxyl radicals that can cause additional damage to the peptide backbone. Hydroxyl radicals generated in the presence of Cu2+ sulfate have been implicated in the cleavage of the IgG1 hinge region.Citation12 Our data using mannitol showed that there was no impact on fragmentation in the presence of this hydroxyl radical scavenger. Nonetheless, it has been shown that a hydroxyl radical generated in close proximity to the site of oxidation may be consumed before it can diffuse, which would render mannitol unable to quench the reaction.Citation42 Data from the Amplex red assay indicates that very little to no hydrogen peroxide was generated when the peptide was incubated with Cu2+. The data from the bathocuproine assay showed only approximately 15% reduction of Cu2+ to Cu1+, adding further support that single electron transfer and further generation of various ROS is not the major pathway in Cu2+-mediated degradation of the hinge peptide. Taken together these data indicate the mechanism of hinge peptide fragmentation by Cu2+ is predominantly hydrolytic in nature, though the specificity of this site could only be explained via a binding pocket hypothesis.
The variant and mutation analyses emphasize the high degree of specificity that Cu2+ has for His in the hinge region. To achieve site-specific cleavage, a binding pocket that presents additional specific ligands to accomplish metal coordination in an appropriate geometry for Cu2+ binding would be expected. Crystal structures of Cu2+ peptides, such as the Cu2+-GGH-methyl-amide complex,Citation24 confirm Cu2+ complexes often adopt square planar geometry. Amino terminal copper and nickel (ATCUN) motifs occur in nature and are known to bind Cu2+ and Ni2+ specifically. The GGH or ATCUN motif utilizes the amino-terminal nitrogen, the following 2 peptide backbone nitrogens, and the imidazole nitrogen of the His with particularly high binding affinity (i.e., Kd = 2.07 × 10−17).Citation24,43 While 4 nitrogen atoms are involved in metal ion binding in a square planar configuration, only 1.9% of His screened in as many as 1949 polypeptide chains were found capable of forming ATCUN like motifs,Citation43 substantiating that Cu2+ binding to IgG1 may not be a random event. IgG1 hinge sequence does not contain an ATCUN motif, but examination of the crystal structure of an IgG1 (PDB: 1HZH)Citation35 revealed that there is a potential metal binding site that could easily accommodate a square planar geometry as preferred by Cu2+. Mutating the His to a Ser most likely changes the geometry and does not allow for efficient Cu2+ binding geometry and hence less efficient fragmentation. Combined with the optimal pH, buffer, and storage conditions, specific coordination of Cu2+ in this binding pocket would significantly enhance the rate of hydrolysis of the hinge region.
mAbs in a liquid formulation are susceptible to binding and hydrolytic cleavage mediated by redox active transition metals such as Cu2+. Accelerated degradation of the mAb mediated by Cu2+ was predominantly in the hinge region. Based on the published crystal structure of a full length IgG1 motif (1HZH),35 the linear SCDKTHTC sequence has the potential to form a square planar coordination structure that potentially facilitates Cu2+ binding. Substitution of the His to other amino acids that do not contain an imidazole moiety but still bind metal ions prevents degradation, while incubating the hinge peptide in His buffer also prevented fragmentation. Ouellette et al. observed atypically high amounts of fragmentation of an IgG1 mAb containing a lambda light chain in histidine buffer at pH 6 incubated with Fe2+, and they showed that a mAb containing kappa light chain when treated with Fe2+ in a similar buffer showed no increase in fragments.Citation44 These results were consistent with our observations that histidine buffer offered protective effects on a kappa light chain molecule in the presence of Cu2+. Collectively, our studies show that the amount of degradation products can be limited by a mAb formulation such as in histidine buffer at pH 5 or by mutating out key moieties located within in the proposed metal binding site of the mAb. Such mutations may lead to a more stable molecule that can resist the detrimental effects caused by trace amounts of metals that are unavoidable during the mAb manufacturing process. On-going studies will include examination of the effects of other site-specific mutations on the hinge, the effects of various amino acid side chain residues, the role of redox inactive metal ions (e.g., Zn2+) on mAb stability and whether the proposed Cu2+ binding site in the hinge region binds other metal ions as well.
Materials and Methods
The recombinant humanized monoclonal IgG1 antibodies mAb 1, mAb 2, and mAb 2 (H224S) mutant were produced using Chinese hamster ovarian (CHO) cells and purified at Genentech (South San Francisco, CA). The amino acid substitutions were generated by site directed mutagenesis. The hinge region linear and cyclic octapeptide SC220DKTHTC was synthesized at Genentech (South San Francisco, CA) with the N-terminus acetylated, C-terminus amidated, and the cysteines blocked with acetaminomethyl (ACM) to prevent dimer formation for only the linear peptide. The peptide was stored as a lyophilized powder at 5°C and reconstituted for use with sterile water to make a 20 mg/mL stock solution. The reconstituted peptide was stored at −70°C. Purity was greater than 95% as determined by RP-HPLC. All chemical reagents used were analytical grade or higher.
Sample preparation
mAb 1 at 30 mg/mL (0.2 mM), mAb 2 wildtype (wt) at 1 mg/mL (0.007 mM), and mAb 2 (H226S) at 1 mg/mL were formulated in 20 mM sodium acetate or 20 mM histidine acetate at pH 5.3. mAb stocks were stored in liquid solutions at −70°C. Test samples were incubated at 40°C for up to 5 weeks with 0.04, 0.08, or 0.15 mM copper chloride (CuCl2) (Sigma Aldrich, St. Louis, MO).
For all studies, the hinge peptide was diluted to 0.2 mg/mL (0.22mM) in either 10 mM sodium acetate, pH 5.0 (Sigma Aldrich, St. Louis, MO); 10 mM MES, pH 6.0 (Sigma Aldrich, St. Louis, MO); or 10 mM sodium HEPES, pH 7.0 (Sigma Aldrich, St. Louis, MO) buffer. The samples were stored for varying lengths of time at 50 and 60°C with or without 0.15 mM CuCl2.
For the oxidation study, the hinge peptide was diluted to 0.2 mg/mL in 10 mM sodium acetate, pH 5.3. Samples were prepared with either 1) the peptide and 1.5 mM hydrogen peroxide (H2O2) (Sigma Aldrich, St. Louis, MO); 2) peptide, 1.5 mM H2O2, and 0.15 mM CuCl2; 3) peptide only; and 4) peptide, mannitol, and CuCl2 and incubated at 40°C for varying lengths of time (24–192 hrs). The reaction was quenched by cooling briefly on wet ice followed by storage at −70°C thereafter.
Size exclusion chromatography (SEC)
Size variant distributions of the mAbs were determined by size exclusion chromatography (SEC) using a TosoHaas Bioscience column G3000 SWXL (South San Francisco, CA) with an autosampler set at 5°C on an Agilent 1200 HPLC (Santa Clara, CA). All samples were injected at 50-μg load onto the column and eluted over 45 minutes with 0.2 M potassium phosphate, 0.25 M potassium chloride (pH 6.2) mobile phase at a flow rate of 0.3 mL/min. Protein elution was monitored at 280 nm. Results are reported as relative percent, which is the area of an individual peak divided by the total area under the curve. Data analysis performed on all chromatograms and integration was done using Thermo Scientific Chromeleon software (Sunnyvale, CA).
Reverse phase high performance liquid chromatography (RP- HPLC) and mass spectrometry (MS)
RP-HPLC was used to analyze the peptide on either an Agilent 1100 or Agilent 1200 HPLC (Santa Clara, CA) using a Vydac 218TP C-18 analytical column (Grace Alltech, Columbia, MD) equilibrated at 82% and 18% of 0.1% TFA/ purified water and 0.1% TFA/ acetonitrile, respectively, then eluted with a gradient at 30°C over 15 minutes at 1.0 mL/ min. The samples were injected onto the column at 2-µg load and monitored with UV absorption at 214 nm. Results are reported as relative percent peak area. Data analysis was done using Thermo Scientific Chromeleon software (Sunnyvale, CA). The samples were also injected onto the column at 10-µg load and analyzed by electrospray ionization MS using either an QStar Elite (AB Sciex, Framingham, MA) or a Orbitrap Elite™ Hybrid Ion Trap (Thermo Fisher Scientific, Waltham, MA) mass spectrometer. During the Orbitrap Elite mass spectrometer sample analysis, a splitter was used to lower the flow rate into the mass spectrometer to approximately 0.2 mL/min prior to coupling. Eluting products were ionized by positive mode electrospray ionization. MS/MS data was collected for the top 5 abundant peaks from every full MS scan. MS data analysis was performed using BioAnalyst (AB Sciex) or Xcalibur (Thermo Fisher) software. The identities of individual products were assigned by accurate mass measurement and confirmed by MS/MS data analysis.
Bathocuprione assay
The generation of Cu1+ from the reduction of Cu2+ in the samples was monitored at room temperature by measuring the absorbance at 483 nm in the presence of bathocuproine-disulfonic acid (BC, Nacalai Tesque), which specifically chelates Cu1+. The reaction was carried out in 96-well plates containing 0.2 mg/mL peptide or buffer, 150 µM Cu2+, 300 µM glycine, 20 mM sodium acetate at pH 5.3 and incubated at 60°C. The standard curve was generated using 200 mM ascorbic acid and Cu2+ ranging from 0 to 100 µM. The sample mixture was incubated for 10 minutes at 37°C in a 96-well plate and covered in foil prior to absorbance measurements on a plate reader Spectra Max.
Amplex red assay
Generation of hydrogen peroxide in the samples was measured using the Amplex Ultra Red Assay (Invitrogen, Carlsbad, CA) per the manufacturer's recommended procedure. The dye, horseradish peroxidase, reacts in a 1 : 1 ratio with H2O2, when added to the sample and results in the production of the fluorescent oxidation product resorufin. The reaction was carried out in a 96-well plate containing 0.2 mg/mL peptide, 150 µM Cu2+, 300 µM glycine, 20 mM sodium acetate at pH 5.3 after incubation at 60°C for 24 hours and quantified using a Spectra Max M2Microplate Reader (Molecular Devices, Sunnyvale, CA) with excitation and emission set at 560 and 590 nm, respectively. Standard curve ranging from 0 to 20 µm H2O2 was generated to determine the final H2O2 concentrations.
Disclosure of Potential Conflicts of Interest
No potential conflicts of interest were disclosed.
Supplemental_Material.zip
Download Zip (280.8 KB)Acknowledgments
We thank Amy Shen from Cell Culture for the mAb2 H224S mutant and Clifford Quan and Jeffrey Tom from the Peptide Synthesis Lab for the preparation of the synthetic peptides at Genentech. The authors would like to thank Aditya Wakankar, John Wang, and Danielle Lieske for helpful discussions on the cyclic peptide.
Supplemental Material
Supplemental data for this article can be accessed on the publisher's website.
References
- Beck A, Wurch T, Bailly C, Corvaia N. Strategies and challenges for the next generation of therapeutic antibodies. Nat Rev Immunol 2010; 10:345-52; PMID:20414207; http://dx.doi.org/10.1038/nri2747
- Liu H, Gaza-Bulseco G, Chumsae C, Newby-Kew A. Characterization of lower molecular weight artifact bands of recombinant monoclonal IgG1 antibodies on non-reducing SDS-PAGE. Biotechnol Lett 2007; 29:1611-22; PMID:17609855; http://dx.doi.org/10.1007/s10529-007-9449-8
- Martin-Moe S, Lim FJ, Wong RL, Sreedhara A, Sundaram J, Sane SU. A new roadmap for biopharmaceutical drug product development: Integrating development, validation, and quality by design. J Pharm Sci 2011; 100:3031-43; PMID:21425164; http://dx.doi.org/10.1002/jps.22545
- Vlasak J, Ionescu R. Fragmentation of monoclonal antibodies. mAbs 2011; 3:253-63; PMID:21487244; http://dx.doi.org/10.4161/mabs.3.3.15608
- Padlan EA. Anatomy of the antibody molecule. Mol Immunol 1994; 31:169-217; PMID:8114766; http://dx.doi.org/10.1016/0161-5890(94)90001-9
- Cordoba AJ, Shyong BJ, Breen D, Harris RJ. Non-enzymatic hinge region fragmentation of antibodies in solution. J Chromatogr B Anal Technol Biomed Life Sci 2005; 818:115-21; http://dx.doi.org/10.1016/j.jchromb.2004.12.033
- Cohen SL, Price C, Vlasak J. Beta-elimination and peptide bond hydrolysis: two distinct mechanisms of human IgG1 hinge fragmentation upon storage. J Am Chem Soc 2007; 129:6976-7; PMID:17500521; http://dx.doi.org/10.1021/ja0705994
- Edelman GM, Cunningham BA, Gall WE, Gottlieb PD, Rutishauser U, Waxdal MJ. The covalent structure of an entire gammaG immunoglobulin molecule. Proc Natl Acad Sci U S A 1969; 63:78-85; PMID:5257969; http://dx.doi.org/10.1073/pnas.63.1.78
- Wang AC, Wang IY. Cleavage sites of human IgG1 immunoglobulin by papain. Immunochemistry 1977; 14:197-200; PMID:863464; http://dx.doi.org/10.1016/0019-2791(77)90194-X
- Fan X, Brezski RJ, Fa M, Deng H, Oberholtzer A, Gonzalez A, Dubinsky WP, Strohl WR, Jordan RE, Zhang N, et al. A single proteolytic cleavage within the lower hinge of trastuzumab reduces immune effector function and in vivo efficacy. Breast Cancer Res 2012; 14:R116; PMID:22873525; http://dx.doi.org/10.1186/bcr3240
- Siepen JA, Keevil EJ, Knight D, Hubbard SJ. Prediction of missed cleavage sites in tryptic peptides aids protein identification in proteomics. J Proteome Res 2007; 6:399-408; PMID:17203985; http://dx.doi.org/10.1021/pr060507u
- Yan B, Boyd D. Breaking the light and heavy chain linkage of human immunoglobulin G1 (IgG1) by radical reactions. J Biol Chem 2011; 286:24674-84; PMID:21606498; http://dx.doi.org/10.1074/jbc.M111.255026
- Kowalik-Jankowska T, Ruta M, Wisniewska K, Lankiewicz L, Dyba M. Products of Cu(II)-catalyzed oxidation in the presence of hydrogen peroxide of the 1-10, 1-16 fragments of human and mouse beta-amyloid peptide. J Inorg Biochem 2004; 98:940-50; PMID:15149800; http://dx.doi.org/10.1016/j.jinorgbio.2004.03.001
- Gaza-Bulseco G, Liu H. Fragmentation of a recombinant monoclonal antibody at various pH. Pharm Res 2008; 25:1881-90; PMID:18473123; http://dx.doi.org/10.1007/s11095-008-9606-3
- Xiang T, Lundell E, Sun Z, Liu H. Structural effect of a recombinant monoclonal antibody on hinge region peptide bond hydrolysis. J Chromatogr B: Anal Technol Biomed Life Sci 2007; 858:254-62; http://dx.doi.org/10.1016/j.jchromb.2007.08.043
- Yates Z, Gunasekaran K, Zhou H, Hu Z, Liu Z, Ketchem RR, Yan B. Histidine residue mediates radical-induced hinge cleavage of human IgG1. J Biol Chem 2010; 285:18662-71; PMID:20304919; http://dx.doi.org/10.1074/jbc.M110.108597
- Smith RM, Hansen DE. The pH-rate profile for the hydrolysis of a peptide bond. J Am Chem Soc 1998; 120:8910-3; http://dx.doi.org/10.1021/ja9804565
- Radzicka A, Wolfenden R. Rates of uncatalyzed peptide bond hydrolysis in neutral solution and the transition state affinities of proteases. J Am Chem Soc 1996; 118:6105-9; http://dx.doi.org/10.1021/ja954077c
- Yashiro M, Sonobe Y, Yamamura A, Takarada T, Komiyama M, Fujii Y. Metal-ion-assisted hydrolysis of dipeptides involving a serine residue in a neutral aqueous solution. Org Biomol Chem 2003; 1:629-32; PMID:12929447; http://dx.doi.org/10.1039/b209565c
- Belczyk-Ciesielska A, Zawisza IA, Mital M, Bonna A, Bal W. Sequence-Specific Cu(II)-Dependent Peptide Bond Hydrolysis: Similarities and Differences with the Ni(II)-Dependent Reaction. Inorg Chem 2014; 53:4639-46; PMID:24735221; http://dx.doi.org/10.1021/ic5003176
- Krezel A, Mylonas M, Kopera E, Bal W. Sequence-specific Ni(II)-dependent peptide bond hydrolysis in a peptide containing threonine and histidine residues. Acta Biochim Pol 2006; 53:721-7; PMID:17117212
- Protas AM, Bonna A, Kopera E, Bal W. Selective peptide bond hydrolysis of cysteine peptides in the presence of Ni(II) ions. J Inorg Biochem 2011; 105:10-6; PMID:21134597; http://dx.doi.org/10.1016/j.jinorgbio.2010.09.003
- Kaminskaia NV, Kostic NM. New selectivity in peptide hydrolysis by metal complexes. platinum(II) complexes promote cleavage of peptides next to the tryptophan residue. Inorg Chem 2001; 40:2368-77; PMID:11327915; http://dx.doi.org/10.1021/ic0014033
- Regan L. The Design of Metal-Binding Sites in Proteins. Annu Rev Biophys Biomol Struct 1993; 22:257-81; PMID:8347991; http://dx.doi.org/10.1146/annurev.bb.22.060193.001353
- Koch KA, Pena MM, Thiele DJ. Copper-binding motifs in catalysis, transport, detoxification and signaling. Chem Biol 1997; 4:549-60; PMID:9281528; http://dx.doi.org/10.1016/S1074-5521(97)90241-6
- Katz AK, Shimoni-Livny L, Navon O, Navon N, Bock CW, Glusker JP. Copper-binding motifs: Structural and theoretical aspects. Helv Chim Acta 2003; 86:1320-38; http://dx.doi.org/10.1002/hlca.200390120
- Hegg EL, Burstyn JN. Hydrolysis of Unactivated Peptide-Bonds by a Macrocyclic Copper(Ii) Complex – Cu([9]Anen(3))Cl−2 Hydrolyzes Both Dipeptides and Proteins. J Am Chem Soc 1995; 117:7015-6; http://dx.doi.org/10.1021/ja00131a030
- Chaderjian WB, Chin ET, Harris RJ, Etcheverry TM. Effect of copper sulfate on performance of a serum-free CHO cell culture process and the level of free thiol in the recombinant antibody expressed. Biotechnol Prog 2005; 21:550-3; PMID:15801797; http://dx.doi.org/10.1021/bp0497029
- Salinas BA, Sathish HA, Shah AU, Carpenter JF, Randolph TW. Buffer-dependent fragmentation of a humanized full-length monoclonal antibody. J Pharm Sci 2010; 99:2962-74; PMID:20091831; http://dx.doi.org/10.1002/jps.21797
- Smith MA, Easton M, Everett P, Lewis G, Payne M, Riveros-Moreno V, Allen G. Specific cleavage of immunoglobulin G by copper ions. Int J Pept Protein Res 1996; 48:48-55; http://dx.doi.org/10.1111/j.1399-3011.1996.tb01105.x
- Allen G, Campbell RO. Specific cleavage of histidine-containing peptides by copper(II). Int J Pept Protein Res 1996; 48:265-73; PMID:8897094; http://dx.doi.org/10.1111/j.1399-3011.1996.tb00840.x
- Cunningham BC, Wells JA. High-resolution epitope mapping of hGH-receptor interactions by alanine-scanning mutagenesis. Science 1989; 244:1081-5; PMID:2471267; http://dx.doi.org/10.1126/science.2471267
- Kamerzell TJ, Li M, Arora S, Ji JA, Wang YJ. The relative rate of immunoglobulin gamma 1 fragmentation. J Pharm Sci 2011; 100:1341-9; PMID:24081469; http://dx.doi.org/10.1002/jps.22389
- Giles NM, Watts AB, Giles GI, Fry FH, Littlechild JA, Jacob C. Metal and redox modulation of cysteine protein function. Chem Biol 2003; 10:677-93; PMID:12954327; http://dx.doi.org/10.1016/S1074-5521(03)00174-1
- Saphire EO, Parren PW, Pantophlet R, Zwick MB, Morris GM, Rudd PM, Dwek RA, Stanfield RL, Burton DR, Wilson IA. Crystal structure of neutralizing humam IGG against HIV-1: a template for vaccine design. Science 2001; 293(5532): 1155-9.
- Huang X, Cuajungco MP, Atwood CS, Hartshorn MA, Tyndall JD, Hanson GR, Stokes KC, Leopold M, Multhaup G, Goldstein LE, et al. Cu(II) potentiation of alzheimer abeta neurotoxicity. Correlation with cell-free hydrogen peroxide production and metal reduction. J Biol Chem 1999; 274:37111-6; PMID:10601271; http://dx.doi.org/10.1074/jbc.274.52.37111
- Schultz J, Allison H, Grice M. Specificity of the cleavage of proteins by dilute acid. I. Release of aspartic acid from insulin, ribonuclease, and glucagon. Biochemistry 1962; 1:694-8; PMID:13909510; http://dx.doi.org/10.1021/bi00910a024
- Rustandi RR, Wang Y. Use of CE-SDS gel for characterization of monoclonal antibody hinge region clipping due to copper and high pH stress. Electrophoresis 2011; 32:3078-84; PMID:22145164; http://dx.doi.org/10.1002/elps.201100186
- Zhang L, Mei Y, Zhang Y, Li S, Sun X, Zhu L. Regioselective cleavage of myoglobin with copper(II) compounds at neutral pH. Inorg Chem 2003; 42:492-8; PMID:12693231; http://dx.doi.org/10.1021/ic025619b
- Bogdanowich-Knipp SJ, Chakrabarti S, Williams TD, Dillman RK, Siahaan TJ. Solution stability of linear vs. cyclic RGD peptides. J Pept Res 1999; 53:530-41; PMID:10424348; http://dx.doi.org/10.1034/j.1399-3011.1999.00052.x
- Khossravi M, Borchardt RT. Chemical pathways of peptide degradation: IX. Metal-catalyzed oxidation of histidine in model peptides. Pharm Res 1998; 15:1096-102; PMID:9688066; http://dx.doi.org/10.1023/A:1011946631197
- Sreedhara A, Lau K, Li C, Hosken B, Macchi F, Zhan D, Shen A, Steinmann D, Schoneich C, Lentz Y. Role of surface exposed tryptophan as substrate generators for the antibody catalyzed water oxidation pathway. Mol Pharmaceutics 2013; 10:278-88; http://dx.doi.org/10.1021/mp300418r
- Sankararamakrishnan R, Verma S, Kumar S. ATCUN-like metal-binding motifs in proteins: identification and characterization by crystal structure and sequence analysis. Proteins 2005; 58:211-21; PMID:15508143; http://dx.doi.org/10.1002/prot.20265
- Ouellette D, Alessandri L, Piparia R, Aikhoje A, Chin A, Radziejewski C, Correia I. Elevated cleavage of human immunoglobulin gamma molecules containing a lambda light chain mediated by iron and histidine. Anal Biochem 2009; 389:107-17; PMID:19318085; http://dx.doi.org/10.1016/j.ab.2009.03.027
- The PyMOL Molecular Graphics System, Version 1.5.0.5. New York, NY: Schrodinger, LLC. Available at https://www.pymol.org/