ABSTRACT
Human IgG is the main antibody class used in antibody therapies because of its efficacy and longer half-life, which are completely or partly due to FcγR-mediated functions of the molecules. Preclinical testing in mouse models are frequently performed using human IgG, but no detailed information on binding of human IgG to mouse FcγRs is available. The orthologous mouse and human FcγRs share roughly 60–70% identity, suggesting some incompatibility. Here, we report binding affinities of all mouse and human IgG subclasses to mouse FcγR. Human IgGs bound to mouse FcγR with remarkably similar binding strengths as we know from binding to human ortholog receptors, with relative affinities IgG3>IgG1>IgG4>IgG2 and FcγRI>>FcγRIV>FcγRIII>FcγRIIb. This suggests human IgG subclasses to have similar relative FcγR-mediated biological activities in mice.
Introduction
Immunoglobulin G (IgG) is the predominant antibody class present in mouse and human serum. It is also the main class used in development of antibody therapies, especially those for cancer.Citation1 IgG is also used to treat immune deficiencies and autoimmune diseases in the form of intravenous immunoglobulin (IVIg), generated from a plasma pool of thousands of donors.Citation2,3 The working mechanisms, efficacy and safety of immunoglobulin therapies must be investigated in in vivo model systems before they can be studied in human trials. Therefore, a good understanding of the different model systems is required.
Most studies use mouse models to validate and investigate the efficacy of monoclonal antibody therapies.Citation4 Many mouse disease models of different settings are available or can be developed.Citation5,6 Antibody therapies currently in development are usually based on humanized or human antibodies to reduce the possibility of immunogenicity, increase half-life and increase efficacy.Citation1 Cancer therapies often depend not only on the neutralizing capacity of the antibody's antigen binding fragments (Fabs) to eliminate pathogen or malignant cells, but also rely on interaction of the constant domain (Fragment crystallizable, Fc) with components of the immune system. This involves binding to molecules including C1q,Citation7 intracellular Fc receptor TRIM21,Citation8 neonatal Fc receptor FcRnCitation9 and the family of Fc gamma receptors (FcγRs). For antibody-mediated cancer-therapies, the FcγRs (found on myeloid cells, natural killer (NK) cells, and also B cells) are generally considered to mediate the main effector mechanisms, and lead to phagocytosis, antibody-dependent cell-mediated cytotoxicity (ADCC) and other effector functions.Citation10
Human IgG can be divided in 4 subclasses, IgG1, IgG2, IgG3 and IgG4, and mouse in IgG1, IgG2a, IgG2b and IgG3, with IgG2c being the equivalent of IgG2a in some mouse strains such as C47Bl/6 mice.Citation11-13 All subclasses mediate effector functions slightly different due to variable specificity and affinity for the different IgG binding partners mentioned above.Citation14-16 Most of the currently approved therapeutic monoclonal antibodies are IgG1, but also IgG2 and IgG4 are used.Citation17-20 IgG2 and IgG4 are preferred when Fc-mediated effector functions are not needed or not preferred, as these subclasses bind with lower affinity to the FcγR and activate complement less actively.Citation14,15
To evaluate results obtained by testing human antibodies in mice, it is important to consider the differences in Fc-mediated binding to effector molecules. The general properties of the mouse and human FcγR have recently been reviewed by Bruhns and Jönsson.Citation21 The human FcγR locus contains 5 activating FcγR (FcγRIa, FcγRIIa, FcγRIIc, FcγRIIIa and FcγRIIIb) associated with an immunoreceptor tyrosine-based activation motif (ITAM, except FcγRIIIb) in the intracellular domain (FcγRIIa and FcγRIIc) or the associated common FcR γ-chain (FcγRIa and FcγRIIIa).Citation14 Human granulocytes express FcγRIIIb, which is a GPI-linked receptor without direct signaling motif, but may still participate in signaling through association with other molecules in lipid rafts.Citation14,22 Humans also express one inhibitory FcγR (FcγRIIb) within this locus, which expresses an immunoreceptor tyrosine-based inhibitory motif (ITIM) in the intracellular domain.
Similarly, mice have 3 activating receptors containing an ITAM motif (FcγRIa, FcγRIII and FcγRIV) and also one inhibitory FcγR containing an ITIM motif (FcγRIIb).Citation12 These receptors are orthologues to the human FcγRI, FcγRIIa, FcγRIIIa and FcγRIIb, respectively.Citation12 They differ slightly from the human system in expression level and function, although their relative affinities for IgG is generally similar within the 2 species.Citation12,21 This has been well documented for both human,Citation14 and mouse IgG subclasses for their corresponding FcγR within species.Citation12
To date, the affinity of human antibodies to mouse FcγR has not been thoroughly investigated, hampering interpretation of findings using human antibodies in mice. We know that human IgG (hereafter referred to as hIgG) subclasses activate FcγR-bearing mouse cells for phagocytosis and ADCC.Citation23,24 Furthermore, it has been shown that hIgG subclasses compete for binding to mouse FcγR, with hIgG3 generally showing more competitive binding than hIgG1, followed by hIgG2 and then hIgG4.Citation23 In vitro and in vivo functional assays have revealed, however, that hIgG1 effector functions dominate over hIgG3, and hIgG4 shows surprisingly good effector functions, perhaps explained by the higher FcγRI affinity as, for example, IFNγ-activated macrophages bear this receptor.Citation23,24 Attempts have been made to circumvent this gap in knowledge of their exact affinities by using humanized mouse models lacking endogenous FcγR but expressing human FcγR,Citation25 but these also have limitations because the FcγR-expression levels and expression patterns do not completely represent the human situation, still warranting the use of wild-type mice. For those cases, detailed information on the affinity of the hIgG subclasses for mouse FcγR is crucial to fully understand and rationalize the use of hIgG in mouse models. We therefore assessed the binding affinity of hIgG subclasses for mouse FcγR and compared that with endogenous affinity in a surface plasmon resonance (SPR) system.
Results
Binding affinity of mouse and human IgG for the 4 mouse FcγR (FcγRI, FcγRIIb, FcγRIII and FcγRIV) was measured using SPR on the IBIS MX96. The IBIS MX96 is a multiplex biosensor with the ability to measure up to 96 different ligand - analyte interactions simultaneously. All the FcγR, and controls, were spotted at various concentrations using a continuous flow microspotter onto a single sensor array. During measurement in the IBIS MX96, the analytes (IgG) were injected over the sensor in increasing concentration. RU values at 360 s were plotted vs concentration of antibody, and data were fitted to a 1:1 Langmuir binding model, in agreement with previous literature, although the binding characteristics are intrinsically complex.Citation26-28 This is consistent with many other studies of IgG-FcγR binding; for some of those interactions, association/dissociation kinetics fall outside of the range that is accurately quantifiable with SPR. The complexity of the IgG-FcγR-interactions, with IgG having 2 mutually exclusive binding sites for FcγRs and the possible involvement of glycans on both FcγR and IgG, introduces additional heterogeneity. To minimize other sources of heterogeneity we used C-terminally biotin tagged FcγRI, FcγRIIb and FcγRIV and anti-HIS was coupled to a streptavidin-coated sensor at 4 different concentrations. The anti-HIS was used to capture c-terminally HIS tagged FcγRIII onto the chip. All subclasses were V-region matched for either mouse or human IgG subclasses, with anti-Kell or anti-D specificity, respectively. Each subclass was injected in several concentrations (shown for human IgG1 in ), and association and dissociation was monitored (). These data were used to generate affinity plots calculating affinity (KD) for each receptor concentration (). This method was chosen because the association-dissociation profiles did not fit simple 1:1 kinetic binding models, but more heterogeneous binding models. This result is in accordance with findings from other groups,Citation26-29 but hampered accurate estimation of association and dissociation constants. The obtained dissociation constants (KD) were interpolated to calculate the affinity at the receptor concentration giving Rmax = 500 as previously described,Citation30 allowing fair affinity comparison at one bioactive concentration between all the receptors (). As previously observed,Citation30 the apparent KD was independent of the receptor density on the sensor for lower affinity receptors, but did vary slightly for the high affinity FcγRI, underlining the importance of reporting the KD at a fixed receptor density.
Figure 1. Calculation of KD by interpolation of equilibrium fit. Sensorgrams (a) and derived affinity plots (b) of human IgG1 anti-RhD binding to ligands FcγRI, FcγRIIb, FcγRIII and FcγRIV for 4 different ligand (FcγR) concentrations; 30, 10, 3 and 1nM, c) The affinity plot derived KD and Rmax of each ligand concentration are plotted for interpolation of KD to a constant Rmax of 500.
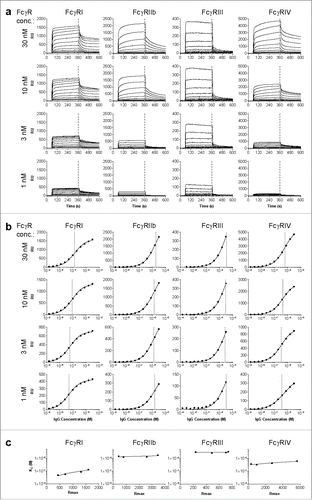
The corresponding data sets were also generated for the other human and mouse IgG subclasses (Fig. S1). The calculated dissociation constants in KD (M) for the mouse IgG subclasses at Rmax = 500 are displayed in . We detected no binding of mIgG1 to FcγRI and FcγRIV, no binding of mIgG2b to FcγRIII and no binding of mIgG3 to any of the mouse FcγR. mIgG1 bound equally well to both FcγRIIb and FcγRIII. mIgG2a bound all receptors with FcγRI > FcγRIV > FcγRIII > FcγRIIb. Similarly, mIgG2b bound all receptors except mouse FcγRI with similar preference.
Figure 2. Binding of mouse IgG subclasses to mouse FcγR. The mouse IgG1, IgG2a, IgG2b and IgG3 with anti-Kell specificity were assessed for their binding affinity in KD (M) to the mouse FcγRI, FcγRIIb, FcγRIII and FcγRIV by SPR. −/− denotes no binding detected. n = 3. The horizontal dashed line represents the maximum concentration of IgG which is used, and the threshold required to accurately calculate the KD.
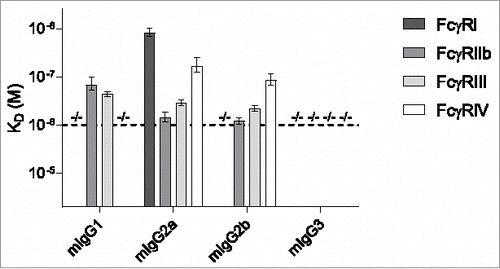
The calculated dissociation constants for human IgG to the mouse FcγRs at Rmax = 500 are displayed in and summarized in . To estimate/calculate the affinity of weak binding interactions (mainly for hIgG2 and hIgG4) more precisely, curve fitting was performed using values of Rmax based on fits for the other subclasses of the respective receptors. Increasing the concentration of these IgG to achieve more accurate results was impractical. We used an average of at least 3 replicate experiments, and this yielded consistent results, with all IgG binding to FcγRI and FcγRIV for example showing similar Rmax. One notable exception was FcγRIII, where fits based on Rmax for hIgG1 were consistently lower than based on Rmax for hIgG3. Therefore, the KD values in for hIgG1, hIgG2 and hIgG4 represent the average and SEM of both calculations (see Materials & Methods section for details). hIgG1 and hIgG3 bound all receptors with affinities that were remarkably similar to those of mouse IgG2a to all the receptors. hIgG4 also bound all mouse receptors, but with significantly lower affinity. Binding of hIgG2 was only observed for FcγRIIb and FcγRIII, with a similar reactivity pattern as mouse IgG1, albeit with a ∼5-60x lower affinity. These affinity ranges were confirmed using another set of human IgG subclasses (Fig. S3). Furthermore, using the same setup in IBIS MX96, the human IgG subclasses bind the human FcγR with affinities comparable to those reported in literatureCitation14 (Fig. S4).
Figure 3. Binding of human IgG subclasses to mouse FcγR. The human IgG1, IgG2, IgG3 and IgG4 with anti-RhD specificity (clone 19A10) were assessed for their binding affinity in KD (M) to the mouse FcγRI, FcγRIIb, FcγRIII and FcγRIV by SPR. −/− means no binding detected; *denotes binding > 2 μM or > 3 μM for hIgG2 or hIgG4, respectively, represented by the horizontal dashed lines, which represent the maximum concentration of IgG that is used, and the threshold required to accurately calculate the KD, 2 μM for IgG2 and 3 μM for IgG1, IgG3 and IgG4; **KDs for FcγRIII binding of hIgG1, hIgG2 and hIgG4 represent the average of 2 alternative estimates (see Material & Methods section for details). n = 3.
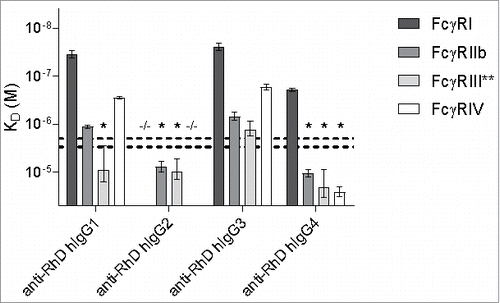
Table 1. Affinity in KD (μM) of human IgG subclasses for mouse FcγRs, SEM in brackets behind KD, −/− no binding detected.
Discussion
Here, we report for the first time the binding affinities of hIgG of all subclasses to all mouse FcγRs and compare this to normal binding of endogenous mouse IgG. We found that human IgG subclasses bind both with similar relative affinity to the mouse FcγR as to ortholog receptors in humans. In addition, we found that human IgG binds mouse FcγR with strikingly similar affinities as mouse IgG, which was highly analogous to that seen for human IgG binding to human FcγR, with only a marginal decrease in affinity. This suggests that preclinical testing of human IgG1 in mouse models may mimic FcγR-mediated effector functions surprisingly well.
We also report the affinities of IgG to Fc-receptors, using a more sophisticated method than previously attempted, by performing a more fair comparison between FcγR using affinity ranking by calculating the KD at a fixed maximal response in the biosensor. This was possible because we simultaneously measured IgG binding to all receptors, the latter being fixed to a biosensor-array through a site-specific c-terminal tag at different concentrations. Injecting the ligand at different concentrations allowed us to calculate affinity for all at ligand densities of the receptors. The final affinity constant was then calculated for an interpolated theoretical Rmax, identical for all the receptors, resulting in a fair comparison between the receptors. This is important, especially for the higher affinity receptors FcγRI and FcγRIV, because the calculated affinities depend on the exact ligand density, which is partly due to interfering effects, including rebinding effects.Citation30
The affinities we obtained for mouse IgG for mouse FcγR and human IgG for human FcγR are in agreement with those previously reported.Citation12,14,16,21,31-34 We have compared the mouse data obtained in this research with that found in literature in a summarizing table (Table S1). Some minor differences were noted, with our data falling well within the range of the affinities found by other groups. The minor discrepancies noted could be due to in the different methods used, as the previous efforts relied on IgG being randomly coupled to the biosensor measuring binding of soluble FcγR, while in our study IgG was titrated and flowed over spotted FcγRs coupled in homogenous orientation through a site-specific c-terminal tag.
We did not observe binding of mIgG2b or mIgG3 to mFcγRI, although some groups have reported positive interactions.Citation21,34,35 One likely explanations are allelic variations of FcγRI within mouse, which increases binding of this receptor to these 2 subclasses.Citation34,35 For this study, we only had access to a single allelic variant of FcγRI. In addition, a recent report has demonstrated that mouse IgG subclasses are also subject to allotypic variations, which may also have affected these interactions.Citation36
The relative affinities of human IgG for the mouse receptors have also been studied by Overdijk et al.Citation23 using competition assays. They observed a higher affinity of hIgG3 to the mouse FcγR that did not translate in higher efficacy in their cell-based and in vivo assays. We also found that hIgG3 generally bound slightly better to mouse FcγR than hIgG1, but these were small differences. Even though we find similar binding affinities and patterns of hIgG1 and hIgG3 to the set of mouse FcγR, several studies showed less efficacy of hIgG3 in mouse cell and in mouse tumor models.Citation23,24 hIgG3 has, however, been found to be effective in mediating complement-dependent cytotoxicity, particularly against tumor targets with low epitope densities, which is probably due to the extraordinary long hinge of hIgG3 that may form a hexameric docking platform for C1q under those conditions.Citation37-39 Similarly, hIgG3 has been found highly efficient in protecting against pneumococcal infections in vivo, also through complement.Citation40,41
hIgG2 has a comparable binding pattern for the mouse Fc receptors as mIgG1, but with an estimated 5- to 50-fold lower affinity than mIgG1. This is in agreement with the results found by Overdijk et al., who showed hIgG2 to compete moderately with mIgG1 binding for mFcγRIIb and mFcγRIV.Citation23
Interestingly, hIgG4 bound most mouse FcγR with relative low affinities, but with medium affinity to mFcγRI. Studies from Overdijk et al. and Steplewski et al. showed high hIgG4 efficacy in mouse xenograft models, which are explained by the higher FcγRI affinity and hIgG4 efficacy in ADCC assays where cells, such as IFNγ activated macrophages, bear this receptor.Citation23,24
For the low affinity interactions (with a KD above ca. 1 × 10−6 M), absolute KD values become less precise, as can be appreciated by comparing results for clones 19A10 (anti-D) and GDob1 ( and Fig. S3), although the overall binding patterns were fairly consistent.
In this study, we have described binding of human IgG to all mouse FcγR in much more detail than previously reported. The relative binding patterns are strikingly similar as we know from the ortholog interaction with the human receptors, with the general relative affinities IgG3>IgG1>IgG4>IgG2. The mouse FcγR also displayed similar preference for human IgG as they do for mouse IgG, with FcγRI>>FcγRIV>FcγRIIb>FcγRIII (hIgG1 and hIgG3 mainly), which is close to what we know for the human ortholog receptors, hFcγRI>>hFcγRIIIa>FcγRIIa>FcγRIIb (where mFcγRIV is ortholog to hFcγRIIIa and mFcγRIII is ortholog to hFcγRIIa.Citation12)
This high conservation of both interspecies affinity and reactivity pattern suggests that using human IgG in mouse models to test FcγR-effector mechanism is feasible, and the use of humanized models of Tg/KO mice, which do not yet completely reflect human expression patterns and levels,Citation25 may not be necessary.
Material and methods
To generate anti human-rhesus D (anti-D) human IgG subclasses, the sequence of anti-human Rhesus D heavy and light chain variable domains of clone 19A1042 were codon optimized and synthesized from Geneart (Life Technologies). IgG constant domains with flanking 3′ NheI and 5′ EcoRI restriction sites were designed, codon optimized and ordered at Mr Gene and cloned as described previously.Citation43 The variable region of the heavy chain (anti-D VH) was subcloned into pEE6.4 (Lonza) expression vector containing either human IgG1, 2, 3 or 4 constant domains. The variable region of the light chain (anti-D VL) was subcloned into pEE14.4 (Lonza) expression vector containing a kappa light chain. Heavy and light chain vectors to express anti Streptococcus pneumoniae serotype 6A/B IgG1, IgG2 and IgG3 named GDob1 (classed switched variants derived from clone Dob144) were described by Saeland et al.Citation41 Heavy and light chain vectors to express anti-BetV1 IgG4 directed against major birch allergen from birch pollen (Betula verrucosa) were previously described.Citation45
To generate anti-Kell mouse IgG subclasses, we used PUMA1 variable domain specific for Kell antigen. The specifics of PUMA1 isolation sequencing and manipulation are reported elsewhere.Citation46 Briefly, wild-type mice were transfused with KEL1 RBCs to induce immunity and a monoclonal anti-KEL1 antibody was isolated by standard myeloma fusion (PUMA1 antibody). VH and VL regions of PUMA1 were isolated by 5′ RACE, codon optimized and synthesized from Geneart (Life Technologies). The variable region of the heavy chain (VH) was subcloned into pFuse expression vectors for mouse IgG1, IgG2a, IgG2b and IgG3 (Invivogen, pfuse-mg1fc1, pfuse-mg2afc1, pfuse-mg2bfc1, pfuse-mg3fc1); the variable region of the kappa light chain (VL) was subcloned into pFuse expression vector for mouse κ-Light chain (Invivogen, pfuse2-mclk).
All IgGs were produced by transient transfection of HEK-freestyle cells (Thermo Fisher Scientific), as previously described by Vink et al.Citation47 and Dekkers et al.Citation48 After 5 days, IgG-containing cell supernatant from these cells was harvested by spinning twice at maximum speed (> 4000 g) and subsequent filtration with 0.45 nm puradisc syringe filter (Whatmann, GE Healthcare, 10462100).
IgG was isolated from cell supernatant with affinity chromatography columns HiTrap Protein A HP (GE Healthcare, 29–0485–76) for human IgG1, IgG2, and IgG4 or HiTrap Protein G HP (GE Healthcare, 29–0485–81) for human IgG3 and mouse IgG1, IgG2a, IgG2b and IgG3 on ÄKTA prime (GE Healthcare) according to standard procedures. Purified antibody fractions were concentrated to concentration >1mg/mL using Protein Concentrators, 9K MWCO (Pierce, Thermo Fisher Scientific, 89884A) and subsequently dialysed against phosphate-buffered saline (PBS) overnight using Slide-A-Lyzer™ Dialysis Cassettes, 10K MWCO (Thermo Fisher Scientific, 66384). Antibody concentration was determined using Nanodrop 2000c UV/VIS spectrophotometer (Thermo Fisher Scientific).
Surface plasmon resonance (SPR) measurements were carried out on a IBIS MX96 (IBIS technologies) as described.Citation49 Biotinylated mouse FcγRI (50086-M27H-B-50), FcγRIIb (50030-M27H-B-50) and FcγRIV (50036-M27H-B-50) were purchased from SinoBiologicals; biotinylated mouse FcγRIII was not available, therefore His-tag conjugated FcγRIII (SinoBiologicals, 50326-M08H-50) was used. All FcγR were spotted using a Continuous Flow Microspotter (Wasatch Microfluidics) onto a single SensEye G-streptavidin sensor (Senss, 1–08–04–008) allowing for binding affinity measurements of each antibody to all FcγR simultaneously on the IBIS MX96 (IBIS technologies) as described.Citation49 The biotinylated FcγR were spotted in duplicate and in 3-fold dilutions, ranging from 30 nM to 1 nM for FcγRI, FcγRIIb and FcγRIV in PBS 0.075% Tween-80 (VWR, M126–100mL), pH 7.4. For the His-tagged FcγRIII, biotinylated anti-His IgG1 (GenScript, A00613) was spotted in duplicate and 3-fold dilution onto the sensor and 100 nM his-FcγRIII (equally diluted in PBS 0.075% Tween-80, pH 7.4) was loaded onto the sensor before each antibody injection.
The IgGs were then injected over the IBIS at dilution series in PBS supplemented with 0.075% Tween-80 ranging from 3.9 nM to 1140 nM for all mouse IgG subclasses, ranging from 1.5 nM to 3000 nM for human IgG1 and IgG3, ranging from 15.6 nM to 2000 nM for human IgG2, or ranging from 23.4 nM to 3000 nM for human IgG4. Regeneration after each sample was carried out with acid buffer (10 mM Gly-HCl, pH 2.2). The system was referenced for slight changes in refractive index due to environmental factors by buffer composition or temperature referencing the signals on spots to signals outside spots. Calculation of the dissociation constant (KD) was performed by fitting a 1:1 Langmuir binding model to the RU360sec values at each antibody concentration. This furthermore resulted in a Rmax value reflecting the functional amount of receptor molecules on the sensor (see ). For consistent reporting, these fits were carried out at each receptor density, and final reported KDs were calculated by interpolating to Rmax = 50030 (see ). In the case of FcγRIII, his-FcγRIII association and dissociation curves on anti-His were subtracted before calculation of IgG-binding affinity using SPRINT 1.9.4.4 software (IBIS technologies). In case of very low affinity (hIgG2 and hIgG4 binding to FcγRIIb and FcγRIII, and IgG4 binding to FcγRIV), fits were performed by fixing Rmax values obtained using IgG1/3 with the same receptor densities, as the theoretical maximal number of available IgG-binding places on a sensor surface is independent of affinity and therefore subclass. For binding of human IgG clone 19A10 (anti-RhD) to FcγRIII, fits based on Rmax obtained for IgG1 binding to FcγRIII were consistently lower than Rmax, obtained for IgG3-binding to the same receptor. The values for this receptor in therefore represent the average and SEM of both calculations. Analysis and calculation of all binding data was carried out with Scrubber software version 2 (Biologic Software).
All statistical analyses were performed using Graphpad Prism software. Error bars represent one standard deviation and significance was determined by a p-value <0.05.
Disclosure of potential conflicts of interest
No potential conflicts of interest were disclosed.
Author contributions
G.D, A.E.H.B, H.L.H, T.S. and S.L.T performed experiments. G.V. and G.D. devised the study. All authors designed the experiments. G.D. and G.V. wrote the manuscript, which was co-edited by all authors.
Supplemental_Materials.pptx
Download MS Power Point (3.8 MB)Acknowledgments
We would like to thank Pleuni Heer-Ooijevaar for supplying the BetV1 constructs and Remco Visser for BetV1 and Gdob1 purification.
References
- Weiner GJ. Building better monoclonal antibody-based therapeutics. Nat Rev Cancer 2015; 15:361-70; PMID:25998715; https://doi.org/10.1038/nrc3930
- Nimmerjahn F, Ravetch JV. Anti-inflammatory actions of intravenous immunoglobulin. Annu Rev Immunol 2008; 26:513-33; PMID:18370923; https://doi.org/10.1146/annurev.immunol.26.021607.090232
- Barahona Afonso AF, João CMP. The production processes and biological effects of intravenous immunoglobulin. Biomolecules 2016; 6:15; PMID:27005671; https://doi.org/10.3390/biom6010015
- Loisel S, Ohresser M, Pallardy M, Daydé D, Berthou C, Cartron G, Watier H. Relevance, advantages and limitations of animal models used in the development of monoclonal antibodies for cancer treatment. Crit Rev Oncol Hematol 2007; 62:34-42; PMID:17197192; https://doi.org/10.1016/j.critrevonc.2006.11.010
- Cheon D-J, Orsulic S. Mouse models of cancer. Annu Rev Pathol 2011; 6:95-119; PMID:20936938; https://doi.org/10.1146/annurev.pathol.3.121806.154244
- Walsh NC, Kenney LL, Jangalwe S, Aryee K-E, Greiner DL, Brehm MA, Shultz LD. Humanized mouse models of clinical disease. Annu Rev Pathol 2016; 187–215; 12: annurev-pathol-052016-100332; PMID:27959627; https://doi.org/10.1146/annurev-pathol-052016-100332
- Merle NS, Church SE, Fremeaux-Bacchi V, Roumenina LT. Complement system part I - molecular mechanisms of activation and regulation. Front Immunol 2015; 6:262; PMID:26082779; https://doi.org/10.3389/fimmu.2015.00262
- James LC, Keeble AH, Khan Z, Rhodes DA, Trowsdale J. Structural basis for PRYSPRY-mediated tripartite motif (TRIM) protein function. Proc Natl Acad Sci U S A 2007; 104:6200-5; PMID:17400754; https://doi.org/10.1073/pnas.0609174104
- Roopenian DC, Akilesh S. FcRn: The neonatal Fc receptor comes of age. Nat Rev Immunol 2007; 7:715-25; PMID:17703228; https://doi.org/10.1038/nri2155
- Bruhns P. Properties of mouse and human IgG receptors and their contribution to disease models. Blood 2012; 119:5640-9; PMID:22535666; https://doi.org/10.1182/blood-2012-01-380121
- Vidarsson G, Dekkers G, Rispens T. IgG subclasses and allotypes: From structure to effector functions. Front Immunol 2014; 5:520; PMID:25368619; https://doi.org/10.3389/fimmu.2014.00520
- Nimmerjahn F, Bruhns P, Horiuchi K, Ravetch JV. FcyRIV: A novel FcR with distinct IgG subclass specificity. Immunity 2005; 23:41-51; PMID:16039578; https://doi.org/10.1016/j.immuni.2005.05.010
- Zhang Z, Goldschmidt T, Salter H. Possible allelic structure of IgG2a and IgG2c in mice. Mol Immunol 2012; 50:169-71; PMID:22177661; https://doi.org/10.1016/j.molimm.2011.11.006
- Bruhns P, Iannascoli B, England P, Mancardi DA, Fernandez N, Jorieux S, Daëron M. Specificity and affinity of human Fcgamma receptors and their polymorphic variants for human IgG subclasses. Blood 2009; 113:3716-25; PMID:19018092; https://doi.org/10.1182/blood-2008-09-179754
- Bindon CI, Hale G, Brüggemann M, Waldmann H. Human monoclonal IgG isotypes differ in complement activating function at the level of C4 as well as C1q. J Exp Med 1988; 168:127-42; PMID:3260935; https://doi.org/10.1084/jem.168.1.127
- Nimmerjahn F, Ravetch JV. Divergent immunoglobulin g subclass activity through selective Fc receptor binding. Science 2005; 310:1510-2; PMID:16322460; https://doi.org/10.1126/science.1118948
- Reichert JM. Marketed therapeutic antibodies compendium. MAbs 2012; 4:413-5; PMID:22531442; https://doi.org/10.4161/mabs.19931
- Reichert JM. Antibodies to watch in 2017. MAbs 2015; 9:167-81; PMID:27960628; https://doi.org/10.1080/19420862.2016.1269580
- Reichert JM. Antibodies to watch in 2016. MAbs 2016; 8:197-204; PMID:26651519; https://doi.org/10.1080/19420862.2015.1125583
- Ecker DM, Jones SD, Levine HL. The therapeutic monoclonal antibody market. MAbs 2015; 7:9-14; PMID:25529996; https://doi.org/10.4161/19420862.2015.989042
- Bruhns P, Jönsson F. Mouse and human FcR effector functions. Immunol Rev 2015; 268:25-51; PMID:26497511; https://doi.org/10.1111/imr.12350
- Vidarsson G, van de Winkel JG. Fc receptor and complement receptor-mediated phagocytosis in host defence. Curr Opin Infect Dis 1998; 11:271-8; PMID:17033391; https://doi.org/10.1097/00001432-199806000-00002
- Overdijk MB, Verploegen S, Ortiz Buijsse A, Vink T, Leusen JHW, Bleeker WK, Parren PWHI. Crosstalk between human IgG isotypes and murine effector cells. J Immunol 2012; 189:3430-8; PMID:22956577; https://doi.org/10.4049/jimmunol.1200356
- Steplewski Z, Sun LK, Shearman CW, Ghrayeb J, Daddona P, Koprowski H. Biological activity of human-mouse IgG1, IgG2, IgG3, and IgG4 chimeric monoclonal antibodies with antitumor specificity. Proc Natl Acad Sci U S A 1988; 85:4852-6; PMID:3387441; https://doi.org/10.1073/pnas.85.13.4852
- Smith P, DiLillo DJ, Bournazos S, Li F, Ravetch JV. Mouse model recapitulating human Fcγ receptor structural and functional diversity. Proc Natl Acad Sci U S A 2012; 109:6181-6; PMID:22474370; https://doi.org/10.1073/pnas.1203954109
- Hayes JM, Frostell A, Cosgrave EFJ, Struwe WB, Potter O, Davey GP, Karlsson R, Anneren C, Rudd PM. Fc gamma receptor glycosylation modulates the binding of IgG glycoforms: A requirement for stable antibody interactions. J Proteome Res 2014; 13:5471-85; PMID:25345863; https://doi.org/10.1021/pr500414q
- Dashivets T, Thomann M, Rueger P, Knaupp A, Buchner J, Schlothauer T. Multi-angle effector function analysis of human monoclonal IgG glycovariants. PLoS One 2015; 10:e0143520; PMID:26657484; https://doi.org/10.1371/journal.pone.0143520
- Subedi GP, Barb AW. The immunoglobulin G1 N-glycan composition affects binding to each low affinity Fc γ receptor. MAbs 2016; 1:1512–24; PMID:27492264; https://doi.org/10.1080/19420862.2016.1218586
- Zeck A, Pohlentz G, Schlothauer T, Peter-Katalinić J, Regula JT. Cell type-specific and site directed N-glycosylation pattern of Fc RIIIa. J Proteome Res 2011; 10:3031-9; PMID:21561106; https://doi.org/10.1021/pr1012653
- Schasfoort RBM, Andree KC, van der Velde N, van der Kooi A, Stojanović I, Terstappen LWMM. Interpolation method for accurate affinity ranking of arrayed ligand-analyte interactions. Anal Biochem 2016; 500:21-3; PMID:26878776; https://doi.org/10.1016/j.ab.2016.01.023
- Baudino L, Nimmerjahn F, Shinohara Y, Furukawa J-I, Petry F, Verbeek JS, Nishimura S-I, Ravetch JV, Izui S. Impact of a three amino acid deletion in the CH2 domain of murine IgG1 on Fc-associated effector functions. J Immunol 2008; 181:4107-12; PMID:18768867; https://doi.org/10.4049/jimmunol.181.6.4107
- White AL, Chan HTC, Roghanian A, French RR, Mockridge CI, Tutt AL, Dixon SV, Ajona D, Verbeek JS, Al-Shamkhani A, et al. Interaction with FcγRIIB is critical for the agonistic activity of anti-CD40 monoclonal antibody. J Immunol 2011; 187:1754-63; PMID:21742972; https://doi.org/10.4049/jimmunol.1101135
- Kaneko Y, Nimmerjahn F, Ravetch JV. Anti-inflammatory activity of immunoglobulin G resulting from Fc sialylation. Science 2006; 313:670-3; PMID:16888140; https://doi.org/10.1126/science.1129594
- Gavin AL, Leiter EH, Hogarth PM. Mouse fcgammaRI: Identification and functional characterization of five new alleles. Immunogenetics 2000; 51:206-11; PMID:10752630; https://doi.org/10.1007/s002510050033
- Gavin AL, Tan PS, Hogarth PM. Gain-of-function mutations in FcgammaRI of NOD mice: Implications for the evolution of the Ig superfamily. EMBO J 1998; 17:3850-7; PMID:9670002; https://doi.org/10.1093/emboj/17.14.3850
- Maresch D, Altmann F. Isotype-specific glycosylation analysis of mouse IgG by LC-MS. Proteomics 2016; 16:1321-30; PMID:26960168; https://doi.org/10.1002/pmic.201500367
- Diebolder CA, Beurskens FJ, de Jong RN, Koning RI, Strumane K, Lindorfer MA, Voorhorst M, Ugurlar D, Rosati S, Heck AJR, et al. Complement is activated by IgG hexamers assembled at the cell surface. Science 2014; 343:1260-3; PMID:24626930; https://doi.org/10.1126/science.1248943
- Rösner T, Derer S, Kellner C, Dechant M, Lohse S, Vidarsson G, Peipp M, Valerius T. An IgG3 switch variant of rituximab mediates enhanced complement-dependent cytotoxicity against tumour cells with low CD20 expression levels. Br J Haematol 2013; 161:282-6; PMID:23294176; https://doi.org/10.1111/bjh.12209
- Natsume A, In M, Takamura H, Nakagawa T, Shimizu Y, Kitajima K, Wakitani M, Ohta S, Satoh M, Shitara K, et al. Engineered antibodies of IgG1/IgG3 mixed isotype with enhanced cytotoxic activities. Cancer Res 2008; 68:3863-72; PMID:18483271; https://doi.org/10.1158/0008-5472.CAN-07-6297
- Stapleton NM, Andersen JT, Stemerding AM, Bjarnarson SP, Verheul RC, Gerritsen J, Zhao Y, Kleijer M, Sandlie I, de Haas M, et al. Competition for FcRn-mediated transport gives rise to short half-life of human IgG3 and offers therapeutic potential. Nat Commun 2011; 2:599; PMID:22186895; https://doi.org/10.1038/ncomms1608
- Saeland E, Vidarsson G, Leusen JHW, Van Garderen E, Nahm MH, Vile-Weekhout H, Walraven V, Stemerding AM, Verbeek JS, Rijkers GT, et al. Central role of complement in passive protection by human IgG1 and IgG2 anti-pneumococcal antibodies in mice. J Immunol 2003; 170:6158-64; PMID:12794146; https://doi.org/10.4049/jimmunol.170.12.6158
- Della Valle L, Dohmen SE, Verhagen OJHM, Berkowska MA, Vidarsson G, Ellen van der Schoot C. The majority of human memory B cells recognizing RhD and tetanus resides in IgM+ B cells. J Immunol 2014; 193:1071-9; PMID:24965774; https://doi.org/10.4049/jimmunol.1400706
- Kruijsen D, Einarsdottir HK, Schijf MA, Coenjaerts FE, van der Schoot EC, Vidarsson G, van Bleek GM. Intranasal administration of antibody-bound respiratory syncytial virus particles efficiently primes virus-specific immune responses in mice. J Virol 2013; 87:7550-7; PMID:23637394; https://doi.org/10.1128/JVI.00493-13
- Sun Y, Park MK, Kim J, Diamond B, Solomon A, Nahm MH. Repertoire of human antibodies against the polysaccharide capsule of streptococcus pneumoniae serotype 6B. Infect Immun 1999; 67:1172-9; PMID:10024557
- van der Neut Kolfschoten M, Schuurman J, Losen M, Bleeker WK, Martínez-Martínez P, Vermeulen E, den Bleker TH, Wiegman L, Vink T, Aarden LA, et al. Anti-inflammatory activity of human IgG4 antibodies by dynamic fab arm exchange. Science 2007; 317:1554-7; PMID:17872445; https://doi.org/10.1126/science.1144603
- Howie HL, Delaney M, Wang X, Er LS, Vidarsson G, Stegmann TC, Kapp L, Lebedev JN, Wu Y, AuBuchon JP, et al. Serological blind spots for variants of human IgG3 and IgG4 by a commonly used anti-immunoglobulin reagent. Transfusion 2016; 56:2953-62; PMID:27632931; https://doi.org/10.1111/trf.13812
- Vink T, Oudshoorn-Dickmann M, Roza M, Reitsma JJ, de Jong RN. A simple, robust and highly efficient transient expression system for producing antibodies. Methods 2014; 65:5-10; PMID:23872061; https://doi.org/10.1016/j.ymeth.2013.07.018
- Dekkers G, Plomp R, Koeleman CAM, Visser R, von Horsten HH, Sandig V, Rispens T, Wuhrer M, Vidarsson G. Multi-level glyco-engineering techniques to generate IgG with defined Fc-glycans. Sci Rep 2016; 6:36964; PMID:27872474; https://doi.org/10.1038/srep36964
- de Lau W, Barker N, Low TY, Koo B-K, Li VSW, Teunissen H, Kujala P, Haegebarth A, Peters PJ, van de Wetering M, et al. Lgr5 homologues associate with Wnt receptors and mediate R-spondin signalling. Nature 2011; 476:293-7; PMID:21727895; https://doi.org/10.1038/nature10337