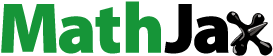
ABSTRACT
Discovery of the upregulation of fibroblast growth factor-inducible-14 (Fn14) receptor following tissue injury has prompted investigation into biotherapeutic targeting of the Fn14 receptor for the treatment of conditions such as chronic kidney diseases. In the development of monoclonal antibody (mAb) therapeutics, there is an increasing trend to use biomeasures combined with mechanistic pharmacokinetic/pharmacodynamic (PK/PD) modeling to enable decision making in early discovery. With the aim of guiding preclinical efforts on designing an antibody with optimized properties, we developed a mechanistic site-of-action (SoA) PK/PD model for human application. This model incorporates experimental biomeasures, including concentration of soluble Fn14 (sFn14) in human plasma and membrane Fn14 (mFn14) in human kidney tissue, and turnover rate of human sFn14. Pulse-chase studies using stable isotope-labeled amino acids and mass spectrometry indicated the sFn14 half-life to be approximately 5 hours in healthy volunteers. The biomeasures (concentration, turnover) of sFn14 in plasma reveals a significant hurdle in designing an antibody against Fn14 with desired characteristics. The projected dose (>1 mg/kg/wk for 90% target coverage) derived from the human PK/PD model revealed potential high and frequent dosing requirements under certain conditions. The PK/PD model suggested a unique bell-shaped relationship between target coverage and antibody affinity for anti-Fn14 mAb, which could be applied to direct the antibody engineering towards an optimized affinity. This investigation highlighted potential applications, including assessment of PK/PD risks during early target validation, human dose prediction and drug candidate optimization.
Introduction
Successful development of monoclonal antibody (mAb) therapeutics requires thorough characterization and optimization of their properties, not only to achieve the desired pharmacokinetics but also to enable optimal target interaction, which is a prerequisite for enabling a pharmacodynamic effect.Citation1 Knowledge of relevant characteristics of the therapeutic target can be equally important for designing appropriate drug properties, such as required affinity ranges. Target properties, also referred to as biomeasures, include target concentration and turnover, and can be incorporated into mathematical modelling of the pharmacokinetic/pharmacodynamic (PK/PD) relationship, for example using quantitative site-of-action (SoA) model.Citation2 The models predictions are frequently sensitive to the accuracy of the biomeasures, which may have to be determined experimentally when no prior information is available. Herein, we describe the application of this strategy to assist with PK/PD risk assessment of an anti-Fn14 mAb therapeutic in early discovery.
The cytokine, tumor necrosis factor-like weak inducer of apoptosis (TWEAK, Apo3L, TNFSF12) and its cognate receptor, fibroblast growth factor-inducible 14 (Fn14, TWEAK receptor, TNFRSF12A, CD266) are members of the TNF/TNFR superfamily.Citation3–5 Fn14 is the only characterized TWEAK receptor that can transduce both soluble and membrane TWEAK.Citation4,6–8 The human Fn14 gene encodes a type I transmembrane protein (129 amino acids) that is processed into a mature protein (102 amino acids). The extracellular domain (53 amino acids) contains a cysteine-rich domain necessary for TWEAK binding.Citation9 The intracellular domain (29 amino acids) of Fn14 lacks a death domain. However, it contains TNFR-associated factor (TRAF)-binding sites that can initiate the signaling events through recruitment of TRAF2/5 and activation of IKK and MAP kinase pathways.Citation10,11 While Fn14 is expressed at low levels in healthy tissue, it is upregulated in tissue injury to mediate local tissue responses. Similar to many TNF receptors, Fn14 has only recently been reported to undergo proteolytic cleavage in the extracellular domain. The soluble sFn14 (sFn14) levels are markedly increased in plasma from mouse models of kidney disease and are correlated with disease biomarkers including proteinuria and MCP-1. Additionally sFn14 was detected in human plasma and urine.Citation12 Moreover, sFn14 levels in urine are significantly increased in diabetic nephropathy (DN) patients and correlated with proteinuria and MCP-1 levels.Citation12
Fn14 was originally described as an immediate-early response gene regulated by growth factors in fibroblasts.Citation13,14 Accumulating evidence has suggested a role for TWEAK activation of Fn14 receptors in the pathogenesis of acute and chronic kidney injury, contributing to both glomerular and tubulointerstitial damage in non-immune and immune-medipt?>ated kidney diseases.Citation15–23 Several studies have supported the pathogenic role of TWEAK/Fn14 pathway by using mouse knockout or antibody blockade in various mouse models of kidney diseases, including acute kidney disease (AKI) and chronic kidney disease (CKD). In particular, TWEAK antibody and Fn14 KO studies have demonstrated a protective role in various systemic lupus erythematosus (SLE)/lupus nephritis (LN) models, including NTN, MRL/lpr and cGvHD models.Citation17,21,22,24–26 In addition to kidney diseases, TWEAK/Fn14 has a potential role in the diseases of different organs, including heart, skeletal muscles, the central nervous system, liver, and gut.Citation27–36 Indeed, several reports have provided data to demonstrate that tissue expression of TWEAK and Fn14, as well as circulating TWEAK levels, are associated with these diseases in both mouse models and human patients.Citation23,24,37–39 While the neutralizing anti-TWEAK antibody (BIIB023; Biogen) Phase 2 clinical trial exploring the potential for kidney protection in LN has been discontinued,Citation40 there has been limited exploration of the therapeutic potential of direct antagonistic targeting of Fn14.
The experimental data described above support the rationale for targeting Fn14 for the treatment of renal disease. However, it is possible that the soluble form of the receptor may impact the ability to attain the desired membrane-bound target coverage by occupying mAb binding sites and reducing the number of mAb binding sites available to bind the membrane Fn14.Citation41 Additionally, efficient neutralization of sFn14 in plasma may not translate to sufficient target coverage in tissue and human efficacy.Citation42,43 It is possible to evaluate the feasibility of a target without actually producing the neutralizing antibody by constructing mechanistic models that describe the kinetics of target, antibody, and target-antibody interaction. Since the pharmacokinetics of the antibody can be assumed to be typical IgG mAb kinetics, the sensitive parameters are the target turnover, target abundance and the affinity of the antibody for the target. The interplay between soluble target, membrane-bound target and antibody, as well as the effects of key biomeasures such as target turnover have been integrated using a quantitative site-of-action (SoA) model for an anti-CXCL13 antibody.Citation2 To aid in the model development, the sFn14 turnover (balance between protein synthesis and protein clearance) was determined for healthy volunteers using pulse-chase administration of a stable isotope labelled (5,5,5-2H3)-leucine (D3-leucine) coupled with immunoaffinity liquid chromatography tandem mass spectrometry (IA-LC-MS/MS) using the method described by Bateman et al.Citation44 In addition, human sFn14 and human kidney tissue Fn14 concentrations were determined using a quantitative IA-LC-MS/MS method. These experimentally determined biomeasures were subsequently incorporated by the SoA model with a goal of evaluating the feasibility of the target and the optimum Kd for the neutralizing antibody.
Results
Determining levels of kidney Fn14 and serum sFN14 in human
Quantification of Fn14 in the human kidney has not previously been reported; hence, kidney FN14 concentrations were determined experimentally. The levels of Fn14 in human kidney were measured by IA-LC-MS/MS, and determined to be 63±10 nM (mean±SD) in tissue samples collected from 5 diabetic nephropathy patients (). Soluble Fn14 concentration in human serum, also measured by IA-LC-MS/MS, was determined to be 1.7±0.3 nM (mean±SD) in 16 healthy individuals (). This value is similar to the sFn14 concentration range previously reported.Citation12 In the previous publication, the serum level of sFn14 in 12 healthy individuals was determined to be 2.8±1.4 nM (14±7 ng/ml) (mean±SD) using an ELISA assay.Citation12
Serum turnover rate for sFn14
The turnover of sFn14 was identified as a critical biomeasure for the SoA model through sensitivity analysis as it significantly affected the required clinical dose by model simulation. For instance, a 3-fold higher dose could be required when the half-life of sFn14 was changed from 50 hour to 0.5 hour (simulation result not shown). Therefore, the physiologically relevant turnover of sFn14 was experimentally determined in healthy human volunteers using a pulse-chase study combined with a MS-based measurement of tracer incorporation changes into a sFn14-specific peptide over time (). The resulting data were fitted to first order kinetics equations () and an average half-life of 5±0.5 hours was determined for sFn14 in the serum of three healthy volunteers. This half-life was considered fairly rapid and could be rationalized based on the small size of sFn14 (molecular weight of approximately 5 kDa), which might be subject to rapid renal filtration.Citation45,46
Optimal Kd identification for antibody engineering
During the early development of antibody therapeutics, the optimal binding affinity against the therapeutic target is a critical point to address. It is known that reduction in Kd could help reduce clinical dose in many circumstances.Citation47 In order to explore the effect of antibody Kd on the target coverage, it requires an understanding of the interplay between anti-Fn14 antibody, mFn14 and sFn14. Hence a mechanistic SoA model was established for human (), by incorporating parameters listed in , which are based on bioanalytical measurements presented earlier in the results and assumptions of typical antibody PK.
Figure 1. The mechanistic SoA model developed for predicting target coverage on membrane Fn14 in kidney in the presence of circulating soluble Fn14.
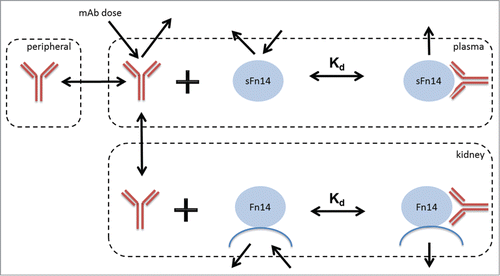
Figure 2. Experimental measures for mFn14 and sFn14 levels in human. Left bar: concentration of mFn14 in human kidney tissue from diabetic nephropathy patients. Right bar: concentration of sFn14 in human serum from healthy subjects. The bar height represents the mean value and error bar represents the standard deviation. The y-axis is in log scale.
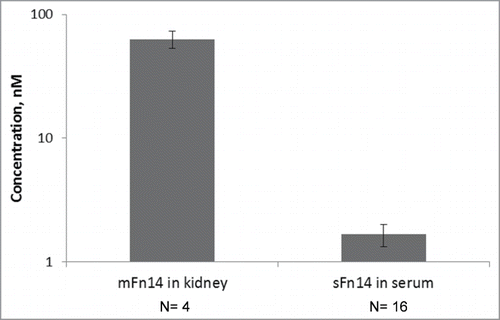
Figure 3. Pulse-chase experiment for determining serum turnover rate of sFn14. a: Chromatograms at selected time points showing the growth and decay of the newly synthesized peptide (blue) ratio to light peptide (red). b: The enrichment of heavy leucine (▪) and heavy sFn14 peptide (▴) in human serum and the corresponding fitted curves. The graph is from one representative subject. Accordingly, the calculated average half-life of sFn14 was 5 ± 0.5 hours in three human subjects.
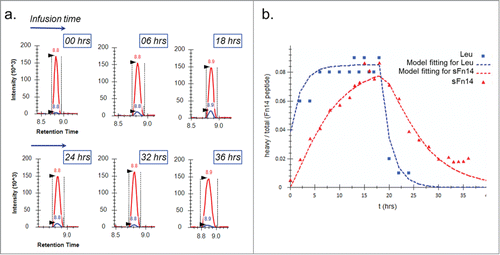
Table 1. Model parameters for mechanistic SoA model.
The relationship between Kd of anti-Fn14 mAb and the clinical dose required to achieve specific target coverage at trough was explored using SoA model simulations. Due to the uncertainty in mFn14 turnover in the kidney, simulations were conducted for a range of mFn14 half-lives (0.5, 5, and 50 h, shown as 3 panels) (). In addition, the effect of sFn14 levels on clinical dose versus Kd relationship was also investigated (shown as the band width of the simulation curves), since sFn14 level was not determined in kidney patients. The sFn14 levels used in the simulation ranges from the measured level in healthy volunteers to a 10-fold higher level, based on the observations that sFn14 levels are markedly increased in plasma from mouse models of kidney disease compared to healthy mouse.Citation12
Figure 4. Simulated relationship between required dose and Kd of anti-Fn14 mAb as a function of 1) mFn14 half-life in kidney (0.5, 5, or 50 hours as illustrated in panels), 2) pre-specified target coverage (90% or 50% as illustrated as blue or yellow curve), and 3) sFn14 concentrations (baseline level in healthy subjects to 10 times baseline, as expressed in width of bands). Optimal Kd of anti-Fn14 mAb is the Kd at which the required dose is the lowest (illustrated as vertical lines).
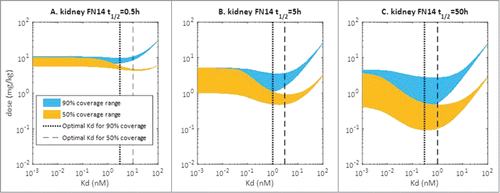
Interestingly, there appears to be a reversed bell-shaped relationship between dose of anti-Fn14 mAb and its Kd. The bell-shape is most pronounced when the mFn14 turnover is slow, i.e., 50 h and is minimal when the mFn14 turnover is rapid, i.e., 0.5 h. Additionally, the dose range due to the 10-fold range in sFn14 levels increases as the mFn14 half-life increases, which is visualized by the band width of the prediction curves. The optimal Kd is defined as the Kd at which the lowest anti-Fn14 mAb dose is required to achieve specified target coverage (90% or 50%). The optimal Kd varied depending on the mFn14 turnover. When target turnover for mFn14 is relatively slow (t1/2 Fn14 = 50 hr), an optimal Kd of about 0.3 nM allows for ≥ 90% target coverage. When target turnover for mFn14 is 5 h, an optimal Kd of 1 nM provides 90% target coverage. When target turnover for mFn14 is 0.5 h, clinical dose versus Kd relationship appears to be monotonic for 90% target coverage, and there is no advantage for having an intermediate Kd. In this scenario, Kd ≤ 5 nM provides similar target coverage. In summary, an optimal Kd of 0.3 to 1 nM was suggested to the antibody engineers, as it requires the lowest dose for achieving ≥ 90% target coverage in all scenarios (Fn14 half-life varies between 0.5 to 50 hr).
Human efficacious dose prediction and risk assessment
Using the SoA model, human efficacious dose was projected for anti-Fn14 antibodies to evaluate developmental risk, in light of the high circulating levels of sFn14 and its potential impact on target coverage. Again, a range of mFn14 turnover half-lives (0.5, 5 and 50 h) were investigated during the simulation. It is assumed that anti-Fn14 antibodies obtain the optimal Kd for target coverage (Kd = 1 nM if t1/2,Fn14 = 0.5 and 5 hr, Kd = 0.3 nM if t1/2,Fn14 = 50 hr). The predicted target coverage for mFn14 in kidney and sFn14 in plasma under weekly subcutaneous dosing were presented in . The turnover half-life of mFn14 has a large impact on target coverage at site of action. If mFn14 turns over quickly (t1/2,Fn14 = 0.5 hr), a high dose of 10 mg/kg/week is predicted for achieving >90% target coverage. With a medium to slow turnover (t1/2,Fn14 = 5 or 50 hr), sufficient target coverage (>90% at trough) can be achieved at 1 mg/kg/week.
Discussion
With the maturation of antibody engineering technologies, the development pipeline for mAbs and related modalities is very rich and diverse. PK/PD modeling coupled with critical bioanalytical measures has gained wide applications in all stages of mAb therapeutics development. Although many publications have touched upon the general guiding principles for applying PK/PD modeling and biomeasures in antibody projects, real-life examples with comprehensive investigation are still scarce, especially for early discovery antibody projects.
In this report, a mechanistic SoA model was first established to capture the interplay between anti-Fn14 antibody, the shed Fn14, and membrane Fn14 targets. The model captures crucial elements affecting the target coverage, including the antibody PK and tissue distribution, target levels and kinetics, as well as the binding affinity for the anti-Fn14 antibody. The presented fit-for-purpose SoA model, where sFn14 in the kidney compartment is not considered, was chosen as the final model. The choice was based on the comparison against a “full model”, where sFn14 in kidney is modeled for its potential neutralizing effect on anti-Fn14 antibody. Our evaluations suggest that, due to the limited amount of sFn14 present in kidney (tissue volume of 0.1 LCitation48) compared to plasma (tissue volume of 3 LCitation48), the effect of sFn14 in kidney on target coverage is negligible (simulations performed; results not presented). Therefore, sFn14 in kidney is not considered in the final model based on the principle of parsimony. Mechanistic SoA models are applied earlier during early drug discovery, for example, toward a quantitative understanding of target pharmacology in preclinical models,Citation2 or in evaluating the impact of baseline tissue target concentration measurement on target coverage prediction.Citation49 This report, on the other hand, provides an example for applying the SoA model, in conjunction with critical biomeasures, to aid antibody engineers and early risk assessment for the target.
Bioanalytical experiments were conducted to generate pivotal model parameters. Since moderate level of sFn14 (22.6 ng/ml) was identified in mouse,Citation12 plasma sFn14 level in human was measured. The sFn14 was present at significant level in healthy subjects (median level at 1.7 nM). The mFn14 level in target tissue (kidney) from diabetic nephropathy patients is high (63 nM) as determined by MS. In addition, considering the potential fast turnover of sFn14 due to its small size (molecular weight of 5 kD), a pulse-chase experiment was conducted to determine the in vivo turnover rate of sFn14 in three healthy human volunteers. Stable isotope labeled amino acids pulse-chase, with minimal system perturbation in conjunction with sensitive MS measurements led to highly reliable physiological turnover data. The biomeasures were integrated into the SoA model, providing more confidence in model prediction.
Our report provides a case study for applying modeling and biomeasure in early drug discovery. The first example is for therapeutic antibody design/optimization, particularly optimal Kd identification. Having guidance on the optimal Kd could greatly facilitate the antibody selection and engineering process, and avoid “over-optimization” situations, where improvement in antibody affinity cannot further reduce the clinical dose but may delay the drug development timeline.Citation47 Interestingly for anti-Fn14 antibodies, there appears to be a reversed bell-shaped relationship between Kd and required dose (). This observation is unique due to the presence of a high amount of soluble target (sFn14) in the circulation considering large plasma volume. In the current case, sFn14 competes against membrane target Fn14 for binding to anti-Fn14 mAb. When the anti-Fn14 binds extremely tightly to targets (both soluble and membrane Fn14), large amounts of antibody are absorbed by the sFn14 “sink” in the circulation. As a result, the required dose for covering the membrane target is high. Conversely, an antibody with weak affinity cannot sufficiently neutralize either soluble or membrane target, leading to a high dose requirement. It is only when Kd is at an intermediate value that the dose for desired target coverage (e.g., 90%) is minimal, since antibodies are not trapped to a significant extent by the shed target, but still have the necessary potency to bind to the membrane target. For anti-Fn14 antibody, an optimal affinity of 0.3 to 1 nM was suggested. It would be counter-productive to have developed super potent (e.g., single digit picomolar) antibody without modeling and biomeasures. Considering many membrane-bound targets shed their extracellular domain (e.g., HER2, CD20),Citation50 the current case study can be applied to these categories of therapeutic targets. It is also worth noting that optimal Kd can be dependent on all the model parameters, including levels and turnover rates of soluble and membrane target. For example, optimal Kd can vary with different turnover half-life of membrane Fn14 (). Thus, it is important to determine the relevant bio-measures to have confidence in the prediction of optimal Kd. The early application of modeling may help select the best candidate for entering the clinic.
Coupling critical biomeasures and modeling, potential risk factors for developing anti-Fn14 antibody as a therapeutic agent were investigated during early drug discovery. Biomeasures, including levels for sFn14 and mFn14, and sFn14 turnover rate, were integrated into the SoA model, providing confidence in model prediction. The clinical dose requirement was evaluated, in light of the high level of circulating sFn14. The simulations indicate a relatively high and frequent clinical doses (>>1 mg/kg/week) are likely to be required for anti-Fn14 Ab to achieve over 90% target coverage over the dosing interval in case of fast mFn14 turnover (). If close to complete target coverage (> 99%) is required for sufficient pharmacology, the predicted dose will likely be 3 mg/kg/week and above (results not shown). It is also worth pointing out that, in the current SoA model, it is assumed that mFn14 (in injured kidney and other healthy tissues) does not have a significant effect on the disposition of anti-Fn14 antibody. As a result, the half-life of the anti-Fn14 mAb is assumed to be similar to a typical mAb. Depending on the amount of mFn14 target present in patients and the binding/internalization property of anti-Fn14 antibody, target-mediated drug disposition (TMDD) may reduce the drug exposure. In case of significant TMDD, the required clinical dose could be even higher than what is currently predicted. This information can be helpful to evaluate the anti-Fn14 mAbs against other antibodies with similar indication, e.g., anti-TWEAK antibodies. In addition, early projection of the clinical dose can influence strategies on molecular properties (e.g., viscosity) and formulations. Modeling also suggests that the clinical dose could be highly dependent on the internalization rate of Fn14 and drug-Fn14 complex in the kidney (). Because of the high level of Fn14 in kidney (63 nM), rapid internalization (if t1/2,Fn14 = 0.5 hr) of the drug-Fn14 complex can significantly increase drug clearance, leading to high clinical dose (10 mg/kg/week). Thus, Fn14 and anti-Fn14 antibodies should be carefully evaluated for their internalization properties using in vitro cell assays and in vivo PK experiments. For instance, if Fn14 is rapidly turning over, an antibody with slow internalization rate may be preferable, as it has less target-mediated clearance. On the other hand, if Fn14 is turning over slowly, an antibody with rapid internalization may be better, since it can efficiently deplete Fn14 through internalization, and thus permits higher target coverage. The internalization properties are critical for membrane-targeted antibodies and should be studied in great detail during early drug discovery.
The SoA model could be further validated using experimental approaches, such as preclinical animal experiments. Key model assumptions, such as the neutralizing effect of sFn14, could be tested using a pharmacology study in relevant animal models by measuring the concentration-time profile of total sFn14 (both free and antibody bound). An accumulation of total sFn14 can be supportive of the soluble target binding assumption. Model simulations can be tested preclinically as well. For instance, to test the projection of a bell-shaped relationship between affinity and efficacious dose, a few antibodies with different binding affinities (low, median and high) can be compared side-by-side in animal models. Furthermore, a model parameter that is hard to obtain in human can be estimated by translating the animal parameter determined in preclinical studies.
The changing environment for drug discovery and development (e.g., increased cost, substantial competition when developing drugs against the same target) requires smarter strategies to be more efficient. Although antibody engineering techniques have been matured over decades, rational design and selection of antibody drug is still an evolving field. PK/PD modeling, together with critical biomarker measurement, could provide valuable guidance during early development of mAbs. By integrating directly relevant information, such as the target level and physiological turnover, and disease population, the model simulations can suggest optimal characteristics for the antibody drug, such as modality choices, optimal Kd, and required half-life. This report illustrates an integrated approach that can be applied to many antibody programs.
Materials and methods
Immunoaffinity-LC-MS/MS assay
sFn14 levels were measured in human serum obtained from healthy subjects and Fn14 levels were measured in human kidney tissue from diabetic nephropathy patients. Recombinant human Fn14 (Uniprot ID Q9NP84, amino acids 27–75 corresponding to the predicted extracellular domain of Fn14 fused to a C terminal His tag) was obtained from Pfizer Pharmaceuticals. Anti-Fn14 mAb P4A8 was obtained from Pfizer and biotinylated using EZ-link Sulfo-NHS-LC-biotin (Pierce Protein Research Products/Thermo Scientific; Rockford, IL). Dynabeads Streptavidin MyOne T1 was purchased from Invitrogen Life Technologies (Carlsbad, CA). Serum samples were either provided by Pfizer (Cambridge, MA) or purchased from Bioreclamation (Long Island, NY). The reference stable isotope-labeled (SIL) peptide was obtained from Thermo Fisher.
A 20 μL aliquot of serum sample, calibrant or quality control (QC) sample was diluted with 680 μL phosphate-buffered saline (PBS) in a 2 ml 96-deep well plate. One μg of biotinylated anti-Fn14 (P4A8 antibody) was added to each well and samples were incubated overnight at 4°C while shaking at 900 rpm. Twenty μL of MyOne T1 streptavidin magnetic beads were added to each well and the plate was incubated for 45 min at room temperature (RT) on a shaker. Magnetic bead and sample processing was performed on an automated liquid handling system (Microlab Star, Hamilton, Bonaduz, Switzerland). The beads were washed twice with 280 μL CHAPS buffer followed by one wash with 280 μL 10 mM PBS. Captured proteins were eluted with 140 μL of 30 mM HCl and immediately neutralized with 30 μL of 2 M Tris–HCl, pH 8.0. A 10 μL aliquot of 0.9 fmol/μL stable isotope-labeled reference peptide was added to each sample. Reduction was performed by the addition of 15 μL of 75 mM TCEP and incubation at 56 °C for 45 min. Fifteen μL of 150 mM iodoacetamide was added to each sample and incubated in the dark at RT for 30 min for alkylation. Samples were then digested overnight at 37 °C using 1 μg of trypsin prior to LC-MS/MS analysis.
The calibration consisted of a six point standard curve analyzed in duplicates across a Fn14 quantification range from 110.2 to 8930 pM. The highest calibrant was prepared by diluting recombinant human Fn14 in 5% bovine serum albumin (BSA). The remaining calibrants were prepared by 3-fold serial dilution of the highest calibrant. QC samples were analyzed in quadruplicates and prepared using human control serum that was either unspiked, spiked with 858.6 pM recombinant human Fn14 or diluted 3 fold using 5% BSA buffer.
A Dionex Ultimate 3000 HPLC system was used for the separation of protein digests. It was configured with a WPS-3000 thermostatted well-plate autosampler, a FLM-3300 flow manager containing a temperature-controlled column compartment and a quaternary micropump, a NCS-3500 module composed of a binary high pressure nanopump, and a tertiary loading pump combined with a temperature-controlled dual 6-port valve column compartment. The system was controlled by Chromeleon software (version 6.8).
One hundred ul of the sample was first loaded on a PepMap300 C18 trap column (5 × 0.3 mm, 5 μm, 300 Å, Dionex) followed by chromatographic separation on a PepMap C18 RSLC nanocolumn (15 cm × 75 μm, 2 μm, 100 Å, Dionex) by gradient elution at a flow rate of 600 nL/min using Solvent A (0.1% formic acid in 2% acetonitrile) and Solvent B (0.1% formic acid in 90% acetonitrile). From 0 to 4 min, the solvent composition was held at 5% B and then ramped up from 5% to 40% B during 4 to 13.5 mins of the cycle, reaching 90% B at 16 mins and then brought back to initial conditions of 5% B for re-equilibration. The total chromatography run time was about 15 min.
A 5500 Qtrap mass spectrometer (AB Sciex) was operated in multiple reaction monitoring (MRM) mode for detection of Fn14 tryptic peptides. The eluate from nanoflow chromatography was introduced into a nanospray III source (AB Sciex) using a nanospray stainless steel emitter (50 mm × 30 μm ID, Proxeon, West Palm Beach, FL). The following instrument parameters were employed: Ion spray voltage: 3700 V; nebulizing gas: 5 psi; curtain gas: 10 psi and Interface heater temperature: 180 °C. Q1 and Q3 were operated in unit and low resolution, respectively. The transitions monitored for the target peptide, GSSWSADLDK were: Q1 533.2 to Q3 921.4 (y8+), 834.4 (y7+), 648.3 (y6+) and 561.2 (y5+) representing the doubly charged precursor ion. Q1 533.2 to Q3 648.3 (y6+) was used for quantitation of human Fn14.
AB Sciex Analyst software (Version 1.5.2) was used for data acquisition and peak areas integration. For each sample, peak area ratios (PAR) of the Fn14 to the reference SIL peptide were calculated and the PAR's of calibration samples were used to construct calibration curves by applying a 1/(concentration) weighted nonlinear regression model using Labstats (Excel add-on). The Fn14 concentration of calibrants was back-calculated to assess the quality of the curve fit. All sample concentrations were then calculated from their PARs against the calibration curve.
sFn14 serum turnover rate measurement
A pulse-chase study combined with IA-LC-MS/MS was utilized for the measurement of the half-life of sFn14 in three healthy male human volunteers between 21 and 40 years old. Briefly, D3-leucine tracer was administered to the subjects with primed (1.3 mg/kg body weight), continuous (0.022 mg/kg body weight per minute) intravenous infusion for 18 hours. Twenty-four blood samples were collected longitudinally from each subject starting at time 0 before bolus infusion and continued during the 18 hours of infusion, followed by 18 hours sample collection after withdrawal of the infusion. The study was conducted at Clinical Pharmacology of Miami with the consent from the volunteers and approved by the Western Institutional Review Board (Olympia, Washington, USA).
Measurements were made for determination of serum leucine tracer enrichment, as well as incorporation of D3-leucine into the sFn14 -specific peptide (GSSWSADLDK). Gas chromatography-mass spectrometry (GC-MS) was employed to measure the serum leucine isotopologue ratios at each time point.Citation51 Akin to the method described above for the quantification of sFn14, the incorporation of the leucine tracer into sFn14 was measured using IA-LC-MS/MS analysis. Briefly, 200 µL aliquots of serum samples diluted by addition of 200 µL of PBST were spiked by 1 µg of biotinylated anti-Fn14 antibody (Anti-Human/Mouse CD266 (mouse IgG2b, 12–9018) from eBioscience (San Diego, CA) and incubated overnight at 4 °C. Streptavidin T1 Dynabeads were added to the samples and incubated for 45 min at RT while mixing at 900 rpm. Magnetic bead wash and protein elution were processed on Microlab Star Hamilton as described above. 1 µg trypsin/Lys C mixture (Promega) was added to the immunoaffinity enriched samples and incubated for overnight digestion and the resulted peptide mixtures were analyzed on a Dionex Ultimate 3000 (Thermo Scientific) linked to a triple quadrupole mass spectrometer, TSQ-Quantiva (Thermo Scientific, USA) equipped with an EZ-spray ionization source. Three MRM transitions for single charge y5, y6 and y7 fragments of GSSWSADLDK doubly charged peptide for both light and heavy were monitored. Raw data were imported into Skyline v2.5 software (University of Washington) for peak integration and area ratios were exported and converted to H/(H+L).
A tracee-tracer model introduced by Foster et. al for estimating the fractional synthetic rate of plasma proteinsCitation52 was implemented in SAAM II software (The Epsilon Group, University of Washington). The model captures the kinetic processes for the synthesis and degradation of the protein. By fitting the profiles of labeled leucine as well as the labeled protein of interest, the model provides estimations for the synthesis and degradation rates of the target protein.
Mechanistic SoA model
Mechanistic SoA models were established for human () using species-specific parameters. The model describes the PK processes of the anti-Fn14 mAb, its distribution into the target tissue and binding to the tissue target Fn14. Since the shed target, sFn14 was detected in the plasma, the SoA model also incorporates the binding between sFn14 and mAb to account for the potential impact of sFn14 on bioactive anti-Fn14 mAb. The set of ordinary differential equations that describe the mouse SoA model are as follows:(1)
(1)
(2)
(2)
(3)
(3)
(4)
(4)
(5)
(5)
(6)
(6)
(7)
(7)
,
, and
represent the drug concentration in plasma, peripheral tissue and site of action (kidney).
and
represent the concentrations of free membrane Fn14 in kidney and free sFn14 in plasma, respectively.
represent the drug-mFn14 complex in kidney, and drug-sFn14 complex in plasma, respectively. Free drug in the plasma (Vp) distributes into peripheral
and SoA
compartments. The binding kinetics between drug and mFn14/sFn14 is characterized by a second-order association
and first-order dissociation
rate constants. Parameters
and
represent the zero-order synthesis rate for the mFn14 and sFn14 respectively, and are calculated based on steady state constrain (EquationEquation 8
(8)
(8) and Equation9
(9)
(9) ). The model parameters are listed in . Fn14 target coverage by the drug is calculated by equation Equation10
(10)
(10) , where Ts is the predicted free mFn14 concentration and Ts0 is the baseline concentration for mFn14 in kidney.
(8)
(8)
(9)
(9)
(10)
(10)
Disclosure
XC, VF, MFO, LLL, HN, and JB are employed by Pfizer, and hold stock in Pfizer. PS and JP were working at Pfizer during their work on this publication and PS held stock in Pfizer.
References
- Kamath AV. Translational pharmacokinetics and pharmacodynamics of monoclonal antibodies. Drug discovery today Technologies 2016; 21–22:75–83.
- Brodfuehrer J, Rankin A, Edmonds J, Keegan S, Andreyeva T, Lawrence-Henderson R, et al. Quantitative analysis of target coverage and germinal center response by a CXCL13 neutralizing antibody in a T-dependent mouse immunization model. Pharmaceutical research 2014; 31:635–48.
- Wiley SR, Cassiano L, Lofton T, Davis-Smith T, Winkles JA, Lindner V, et al. A novel TNF receptor family member binds TWEAK and is implicated in angiogenesis. Immunity 2001; 15:837–46.
- Winkles JA. The TWEAK-Fn14 cytokine-receptor axis: discovery, biology and therapeutic targeting. Nature reviews Drug discovery 2008;7:411–25.
- Nakayama M, Ishidoh K, Kojima Y, Harada N, Kominami E, Okumura K, et al. Fibroblast growth factor-inducible 14 mediates multiple pathways of TWEAK-induced cell death. Journal of immunology 2003;170:341–8.
- Cheng E, Armstrong CL, Galisteo R, Winkles JA. TWEAK/Fn14 Axis-Targeted Therapeutics: Moving Basic Science Discoveries to the Clinic. Frontiers in immunology 2013;4:473.
- Roos C, Wicovsky A, Muller N, Salzmann S, Rosenthal T, Kalthoff H, et al. Soluble and transmembrane TNF-like weak inducer of apoptosis differentially activate the classical and noncanonical NF-kappa B pathway. Journal of immunology 2010;185:1593–605.
- Salzmann S, Seher A, Trebing J, Weisenberger D, Rosenthal A, Siegmund D, et al. Fibroblast growth factor inducible (Fn14)-specific antibodies concomitantly display signaling pathway-specific agonistic and antagonistic activity. The Journal of biological chemistry 2013;288:13455–66.
- Brown SA, Hanscom HN, Vu H, Brew SA, Winkles JA. TWEAK binding to the Fn14 cysteine-rich domain depends on charged residues located in both the A1 and D2 modules. The Biochemical journal 2006;397:297–304.
- Brown SA, Richards CM, Hanscom HN, Feng SL, Winkles JA. The Fn14 cytoplasmic tail binds tumour-necrosis-factor-receptor-associated factors 1, 2, 3 and 5 and mediates nuclear factor-kappaB activation. The Biochemical journal 2003;371:395–403.
- Han S, Yoon K, Lee K, Kim K, Jang H, Lee NK, et al. TNF-related weak inducer of apoptosis receptor, a TNF receptor superfamily member, activates NF-kappa B through TNF receptor-associated factors. Biochemical and biophysical research communications 2003;305:789–96.
- Sharif MN, Campanholle G, Nagiec EE, Wang J, Syed J, O'Neil SP, et al. Soluble Fn14 Is Detected and Elevated in Mouse and Human Kidney Disease. PloS one 2016;11:e0155368.
- Meighan-Mantha RL, Hsu DK, Guo Y, Brown SA, Feng SL, Peifley KA, et al. The mitogen-inducible Fn14 gene encodes a type I transmembrane protein that modulates fibroblast adhesion and migration. The Journal of biological chemistry 1999;274:33166–76.
- Feng SL, Guo Y, Factor VM, Thorgeirsson SS, Bell DW, Testa JR, et al. The Fn14 immediate-early response gene is induced during liver regeneration and highly expressed in both human and murine hepatocellular carcinomas. The American journal of pathology 2000;156:1253–61.
- Justo P, Sanz AB, Sanchez-Nino MD, Winkles JA, Lorz C, Egido J, et al. Cytokine cooperation in renal tubular cell injury: the role of TWEAK. Kidney international 2006;70:1750–8.
- Weinberg JM. TWEAK-Fn14 as a mediator of acute kidney injury. Kidney international 2011;79:151–3.
- Zhao Z, Burkly LC, Campbell S, Schwartz N, Molano A, Choudhury A, et al. TWEAK/Fn14 interactions are instrumental in the pathogenesis of nephritis in the chronic graft-versus-host model of systemic lupus erythematosus. Journal of immunology 2007;179:7949–58.
- Molano A, Lakhani P, Aran A, Burkly LC, Michaelson JS, Putterman C. TWEAK stimulation of kidney resident cells in the pathogenesis of graft versus host induced lupus nephritis. Immunology letters 2009;125:119–28.
- Sanz AB, Sanchez-Nino MD, Izquierdo MC, Jakubowski A, Justo P, Blanco-Colio LM, et al. Tweak induces proliferation in renal tubular epithelium: a role in uninephrectomy induced renal hyperplasia. Journal of cellular and molecular medicine 2009;13:3329–42.
- Sanz AB, Sanchez-Nino MD, Ortiz A. TWEAK, a multifunctional cytokine in kidney injury. Kidney international 2011;80:708–18.
- Xia Y, Campbell SR, Broder A, Herlitz L, Abadi M, Wu P, et al. Inhibition of the TWEAK/Fn14 pathway attenuates renal disease in nephrotoxic serum nephritis. Clinical immunology 2012;145:108–21.
- Sanchez-Nino MD, Poveda J, Sanz AB, Mezzano S, Carrasco S, Fernandez-Fernandez B, et al. Fn14 in podocytes and proteinuric kidney disease. Biochimica et biophysica acta 2013;1832:2232–43.
- Sanz AB, Izquierdo MC, Sanchez-Nino MD, Ucero AC, Egido J, Ruiz-Ortega M, et al. TWEAK and the progression of renal disease: clinical translation. Nephrology, dialysis, transplantation: official publication of the European Dialysis and Transplant Association – European Renal Association 2014;29:Suppl 1:i54–i62.
- Michaelson JS, Wisniacki N, Burkly LC, Putterman C. Role of TWEAK in lupus nephritis: a bench-to-bedside review. Journal of autoimmunity 2012;39:130–42.
- Sanz AB, Justo P, Sanchez-Nino MD, Blanco-Colio LM, Winkles JA, Kreztler M, et al. The cytokine TWEAK modulates renal tubulointerstitial inflammation. Journal of the American Society of Nephrology: JASN 2008;19:695–703.
- Xia Y, Herlitz LC, Gindea S, Wen J, Pawar RD, Misharin A, et al. Deficiency of fibroblast growth factor-inducible 14 (Fn14) preserves the filtration barrier and ameliorates lupus nephritis. Journal of the American Society of Nephrology: JASN 2015;26:1053–70.
- Tajrishi MM, Zheng TS, Burkly LC, Kumar A. The TWEAK-Fn14 pathway: a potent regulator of skeletal muscle biology in health and disease. Cytokine & growth factor reviews 2014;25:215–25.
- Novoyatleva T, Sajjad A, Engel FB. TWEAK-Fn14 Cytokine-Receptor Axis: A New Player of Myocardial Remodeling and Cardiac Failure. Frontiers in immunology 2014;5:50.
- Yepes M. TWEAK and the central nervous system. Molecular neurobiology 2007;35:255–65.
- Tirnitz-Parker JE, Viebahn CS, Jakubowski A, Klopcic BR, Olynyk JK, Yeoh GC, et al. Tumor necrosis factor-like weak inducer of apoptosis is a mitogen for liver progenitor cells. Hepatology 2010;52:291–302.
- Yoriki R, Akashi S, Sho M, Nomi T, Yamato I, Hotta K, et al. Therapeutic potential of the TWEAK/Fn14 pathway in intractable gastrointestinal cancer. Experimental and therapeutic medicine 2011;2:103–8.
- Son A, Oshio T, Kawamura YI, Hagiwara T, Yamazaki M, Inagaki-Ohara K, et al. TWEAK/Fn14 pathway promotes a T helper 2-type chronic colitis with fibrosis in mice. Mucosal immunology 2013;6:1131–42.
- Karaca G, Xie G, Moylan C, Swiderska-Syn M, Guy CD, Kruger L, et al. Role of Fn14 in acute alcoholic steatohepatitis in mice. American journal of physiology Gastrointestinal and liver physiology 2015;308:G325–34.
- Wen J, Doerner J, Weidenheim K, Xia Y, Stock A, Michaelson JS, et al. TNF-like weak inducer of apoptosis promotes blood brain barrier disruption and increases neuronal cell death in MRL/lpr mice. Journal of autoimmunity 2015;60:40–50.
- Ren MY, Sui SJ. The role of TWEAK/Fn14 in cardiac remodeling. Molecular biology reports 2012;39:9971–7.
- Novoyatleva T, Janssen W, Wietelmann A, Schermuly RT, Engel FB. TWEAK/Fn14 axis is a positive regulator of cardiac hypertrophy. Cytokine 2013;64:43–5.
- Poveda J, Tabara LC, Fernandez-Fernandez B, Martin-Cleary C, Sanz AB, Selgas R, et al. TWEAK/Fn14 and Non-Canonical NF-kappaB Signaling in Kidney Disease. Frontiers in immunology 2013;4:447.
- Zheng TS, Burkly LC. No end in site: TWEAK/Fn14 activation and autoimmunity associated- end-organ pathologies. Journal of leukocyte biology 2008;84:338–47.
- Izumi Y, Yabe D, Taniguchi A, Fukushima M, Nakai Y, Hosokawa M, et al. Circulating TNF receptor 2 is associated with the development of chronic kidney disease in non-obese Japanese patients with type 2 diabetes. Diabetes research and clinical practice 2013;99:145–50.
- Galluppi GR, Wisniacki N, Stebbins C. Population pharmacokinetic and pharmacodynamic analysis of BIIB023, an anti-TNF-like weak inducer of apoptosis (anti-TWEAK) monoclonal antibody. British journal of clinical pharmacology 2016;82:118–28.
- Li L, Gardner I, Rose R, Jamei M. Incorporating Target Shedding Into a Minimal PBPK-TMDD Model for Monoclonal Antibodies. CPT: pharmacometrics & systems pharmacology 2014;3:e96.
- Bruno R, Washington CB, Lu JF, Lieberman G, Banken L, Klein P. Population pharmacokinetics of trastuzumab in patients with HER2+ metastatic breast cancer. Cancer chemotherapy and pharmacology 2005;56:361–9.
- Glassman PM, Balthasar JP. Mechanistic considerations for the use of monoclonal antibodies for cancer therapy. Cancer biology & medicine 2014;11:20–33.
- Bateman RJ, Munsell LY, Morris JC, Swarm R, Yarasheski KE, Holtzman DM. Human amyloid-beta synthesis and clearance rates as measured in cerebrospinal fluid in vivo. Nature medicine 2006;12:856–61.
- Knauf MJ, Bell DP, Hirtzer P, Luo ZP, Young JD, Katre NV. Relationship of effective molecular size to systemic clearance in rats of recombinant interleukin-2 chemically modified with water-soluble polymers. The Journal of biological chemistry 1988;263:15064–70.
- Wartiovaara J, Ofverstedt LG, Khoshnoodi J, Zhang J, Makela E, Sandin S, et al. Nephrin strands contribute to a porous slit diaphragm scaffold as revealed by electron tomography. The Journal of clinical investigation 2004;114:1475–83.
- Tabrizi MA, Bornstein GG, Klakamp SL. Development of antibody-based therapeutics: translational considerations. Springer Science & Business Media, 2012.
- Shah DK, Betts AM. Towards a platform PBPK model to characterize the plasma and tissue disposition of monoclonal antibodies in preclinical species and human. Journal of pharmacokinetics and pharmacodynamics 2012;39:67–86.
- Tiwari A, Luo H, Chen X, Singh P, Bhattacharya I, Jasper P, et al. Assessing the Impact of Tissue Target Concentration Data on Uncertainty in In Vivo Target Coverage Predictions. CPT: pharmacometrics & systems pharmacology 2016;5:565–74.
- Kuang B, King L, Wang HF. Therapeutic monoclonal antibody concentration monitoring: free or total? Bioanalysis 2010;2:1125–40.
- Price JC, Holmes WE, Li KW, Floreani NA, Neese RA, Turner SM, et al. Measurement of human plasma proteome dynamics with (2)H(2)O and liquid chromatography tandem mass spectrometry. Analytical biochemistry 2012;420:73–83.
- Foster DM, Barrett PH, Toffolo G, Beltz WF, Cobelli C. Estimating the fractional synthetic rate of plasma apolipoproteins and lipids from stable isotope data. Journal of lipid research 1993;34:2193–205.