ABSTRACT
Bispecific antibodies have emerged as therapeutic molecules with a multitude of modes of action and applications. Here, we present a novel approach to solve the light-chain problem for the generation of bispecific Ig-like antibodies using the second constant domain of IgE (EHD2) genetically modified to force heterodimerization. This was achieved by introducing a C14S mutation in one domain and a C102S mutation in the other domain, which removed of one of the crossover disulfide bonds. Substituting the CH1 and CL domains of an antigen binding fragment (Fab) with these heterodimerizing EHD2 (hetEHD2) domains resulted in Fab-like building blocks (eFab). These eFabs were used to generate different bispecific antibodies of varying valency and molecular composition employing variable domains with different specificities and from different origins. Formats included bivalent bispecific IgG-like molecules (eIgs) and Fc-less Fab-eFab fusion proteins, as well as tri- and tetravalent Fab-eIg fusion proteins. All proteins, including bispecific antibodies for dual receptor targeting and for retargeting of T cells, efficiently assembled into functional molecules. Furthermore, none of the hetEHD2-comprising molecules showed binding to the two Fcε receptors and are thus most likely do not induce receptor cross-linking and activation. In summary, we established the eIg technology as a versatile and robust platform for the generation of bispecific antibodies of varying valency, geometry, and composition, suitable for numerous applications.
Abbreviations: antibody drug conjugate (ADC), acute lymphocytic leukemia (ALL), constant domain of IgE (Cε), receptor of Cε (CεRI or CεRII), cluster of differentiation (CD), constant domain of heavy chain (CH), constant domain of light chain (CL), (single-chain) diabody ((sc)Db), diabody-immunoglobulin (Db-Ig), dynamic light scattering (DLS), Fragment antigen-binding (Fab), Fab with hetEHD2 (eFab), Fab-EHD2 with T121G in chain 1 and S10I in chain 2 (EFab), bispecific Ig domain containing hetEHD2 (eIg), extracellular domain (ECD), epidermal growth factor receptor 1, 2, 3 (EGFR, HER2, HER3), heavy chain domain 2 of IgE (EHD2), EHD2 domain with C102S (EHD2-1), EHD2 domain with C14S and N39Q (EHD2-2), (human or mouse) fragment crystalline ((hu or mo)Fc), heavy chain (HC), heterodimerized second domain of IgE (hetEHD2), high molecular weight (HMW), immunoglobulin (Ig), light chain (LC), liquid chromatography-mass spectrometry (LC-MS), mesenchymal epithelial transition factor (MET), heavy chain domain 2 of IgM (MHD2), peripheral blood mononuclear cell (PBMC), prolactin receptor (PRLP), Stokes radius (RS), single-chain Fragment variable (scFv), tumor necrosis factor (TNF), TNF receptor 2 (TNFR2), single-chain TNF-related apoptosis-inducing ligand (scTRAIL), variable domain of heavy chain (VH), variable domain of light chain (VL).
Introduction
Bispecific antibodies targeting two different epitopes enable diverse modes of action either in a combinatorial or obligate manner, such as dual targeting of growth factors and their receptors, retargeting of effector cells, transport through biological barriers, or mimicking the activity of natural proteins.Citation1 Three bispecific antibodies are currently approved for therapy: blinatumomab, targeting CD19 and CD3 for treatment of acute lymphatic leukemia (ALL), amivantamab for dual targeting of EGFR and MET to treat non-small cell lung cancer, and emicizumab as a substitute of factor VIIIa for the treatment of hemophilia A. Many more bispecific antibodies are in different stages of clinical development.Citation2
An entire zoo of different formats is available for the generation of bispecific antibodies, including Ig-like molecules comprising an Fc region and two or more antigen-binding sites.Citation3 For example, bispecific molecules with a symmetric architecture can be generated by fusing additional binding sites to an IgG molecule, thus being bispecific and tetravalent. In contrast, Ig-like molecules with a 1 + 1 binding mode, i.e., comprising one binding site for each antigen, require genetic engineering to force heterodimerization of the heavy chains (the heavy chain problem) and pairing of the light chains with their cognate heavy chains (the light chain problem). Various strategies have been established to solve the heavy chain problem, such as hydrophobic/steric complementarity, e.g., the knobs-into-holes technology, electrostatic complementarity, or combinations thereof.Citation3–5 Several strategies were also developed to force correct pairing of the light chain with its cognate heavy chain.Citation6,Citation7 Besides introducing mutations into the constant (CH1 and CL) and variable (VH and VL) domains of an antigen binding fragment (Fab) or applying the CrossMab technology,Citation8 approaches were developed to exchange these domains by alternative heterodimerization domains.
We recently established the heavy chain domain 2 of IgM (MHD2) and IgE (EHD2), which form disulfide-linked hinge-like homodimers in IgM and IgE, respectively, as antibody-derived dimerization modules lacking antibody effector functions.Citation9,Citation10 We demonstrated that different moieties, such as single-chain variable fragment (scFv) or single-chain derivatives of members of the tumor necrosis factor (TNF) superfamily can be fused to the N- and/or C-terminus of the MHD2 or EHD2 forming stable homodimers. For example, hexavalent EHD2 fusion proteins of scTRAIL or a TNFR2-selective TNF mutein, respectively, were shown to be potent activators of the respective receptors due to efficient receptor clustering.Citation11–14 Furthermore, the MHD2 and EHD2 domains were used to stabilize mono- or bispecific diabodies (Dab). Fusion of these Dab moieties to an Fc region resulted in tetravalent Ig-like molecules (Diabody-Ig, Db-Ig).Citation14 Heterodimerization of bispecific Diabody-EHD2 fusions were further facilitated by introducing single mutations into the EHD2 domains. Removal of one of the two disulfide bonds formed between Cys14 and Cys102 between the two domains by substituting Cys14 in a first domain and Cys102 in a second domain with serine lead to efficient formation of heterodimeric EHD2 domains (hetEHD2) ().
Figure 1. Generation of hetEHD2 domains. (a) Sequences of the human IgE heavy-chain domain 2 (EHD2) and the heterodimerization domains (hetEHD2 containing EHD2-1 and EHD2-2). Inter- and intrachain disulfide bonds as well as N-glycosylation site are marked. (b) Structure of the heterodimerization domain (hetEHD2) (modified from pdb entry 1O0V).Citation15 The two domains are colored in blue (EHD2-1) and red (EHD2-2). Cysteine residues in the two domains are shown as spheres in orange (EHD2-1) and yellow (EHD2-2). Cysteine residues on position 28 (Kabat: 261) and 86 (Kabat: 321) for intradomain disulfide bonds in each hetEHD2 domain, while the cysteine 14 (Kabat: 247) of EHD2-1 and the cysteine 102 (Kabat: 337) of EHD2-2 form interchain disulfide bond. The potential N-glycosylation site is show in green. Numbering of the residues according to A or the Kabat index.Citation16
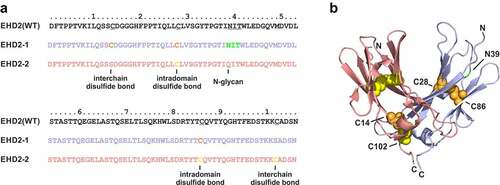
In this study, we applied the hetEHD2 domains as a solution to the light chain problem. We could demonstrate that fusion of a VH domain to a first hetEHD2 domain and a VL domain to a second hetEHD2 domain results in efficient formation of heterodimeric Fab-like moieties (eFab). These eFabs were then used to generate bispecific Ig-like molecules by combining them with natural Fab arms and an Fc region. Here, various molecular compositions were analyzed, including bivalent bispecific Fab-eFab fusions and Ig-like molecules (eIgs), as well as various trivalent and tetravalent configurations (Fab-eIgs). Proof of concept was obtained for bispecific molecules targeting different surface receptors (dual targeting) and for the retargeting of T cells to tumor cells (T-cell engagement). All molecules were produced in yields and purity comparable to normal IgGs. Furthermore, analysis for binding to FcεRI and FcεRII confirmed lack of interaction with the Fcε receptors, providing evidence that the molecules will not lead to Fcε receptor crosslinking of immune cells. Thus, with our study we established eFabs as robust building blocks to generate bispecific antibodies of varying valency and composition.
Results
Generation of eFab
Two different eFab molecules were generated by fusing the variable domains of an anti-MET antibody 5D5 to the hetEHD2 domains. The VH domain of 5D5 was fused to the EHD2-1 domain (carrying the C102S mutation and further comprising a His-tag) and the VL domain of 5D5 was fused to the EHD2-2 domain (carrying the C14S mutation and further an N39Q mutation to delete the N-glycosylation site), resulting in eFab1, or vice versa (resulting in eFab2) (). The eFabs were produced in transiently co-transfected HEK293-6E cells and purified by immobilized metal ion affinity chromatography (IMAC) with yields of 10.7 mg/L (eFab1) and 4.8 mg/L (eFab2), while the Fab5D5 molecule was purified with CaptureSelect CH1 with yields of 7.2 mg/L. SDS-PAGE analysis of purified proteins showed two bands under reducing conditions for the eFab1 molecule, one at ~35 kDa, corresponding to the N-glycosylated EHD2-1 comprising fragment and one band at ~27 kDa, corresponding to the non-glycosylated EHD2-2 comprising fragment, while for the eFab2 molecule only one band at ~30 kDa was observed under reducing conditions representing both chains. One major band in the range of 47͐–50 kDa was observed for both proteins under non-reducing conditions, corresponding to the heterodimeric eFab molecules with calculated molecular masses of 50.2 kDa (). The faint bands at ~30 (eFab1) and ~24 kDa (eFab2) under non-reducing conditions correspond to the purified monomeric VH-comprising fragment. In the case of the Fab5D5 molecule, one major band was detected at ~26 kDa under reducing conditions corresponding to both chains and one major band at ~40 kDa corresponding to the dimeric assembled Fab fragment. Dimeric assembly and integrity were further confirmed by size-exclusion chromatography (SEC) analysis showing one major peak at 2.4 nm (33.2 kDa) for eFab1, 1.8 nm (29.1 kDa) for eFab2 and 2.2 nm (31.7 kDa) for Fab (). Aggregation temperatures of 63°C were determined by dynamic light scattering (DLS) for both eFab molecules, while the Fab molecule showed an aggregation temperature of 67°C ().
Figure 2. Generation of hetEHD2-derived eFab molecules. (a) Schematic illustration of the eFab molecules containing the anti-MET variable domains (from 5D5)Citation17 and the hetEHD2 domains. In this experiment, we used the VL5D5-comprising chain in combination either with non-glycosylated EHD2-2 or with the glycosylated version EHD2-1. For the Fd-like fragment, the VH5D5-containing chain was either combined with EHD2-1 (eFab1) or with the non-glycosylated version EHD2-2 (eFab2) fused to a His-tag. As control, we included a Fab fragment using the same variable domains. (b) Schematic illustration of both eFab molecules (eFab1: VL5D5-EHD2-2 + VH5D5-EHD2-1-His; eFab2: VL5D5-EHD2-1 + VH5D5-EHD2-2-His) and the Fab5D5. (c) SDS-PAGE analysis of the purified eFab and Fab molecules under reducing (R) and non-reducing (NR) conditions (12% PAA, 4 µg of protein, M: marker). (d) Size-exclusion chromatography (SEC) profile with a TSKgel G2000SWXL column under native conditions using HPLC analysis. (e) Aggregation temperature of both eFab molecules and the Fab5D5 using dynamic light scattering (DLS). Dotted line indicates the aggregation point. (f) ELISA binding experiment using MET-huFc fusion protein as immobilized antigen. Bound antibodies were detected with an anti-His-HRP detection antibody. Mean ± SD, n = 3.
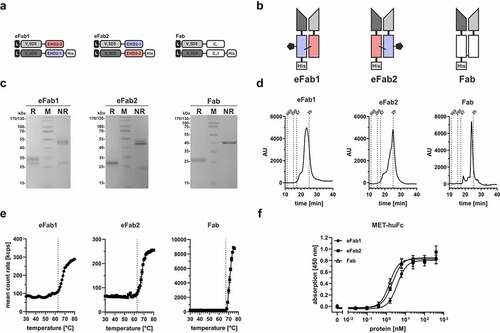
Binding of all molecules was analyzed in an enzyme-linked immunosorbent assay (ELISA) using MET-huFc fusion protein as immobilized antigen. All proteins showed concentration-dependent binding to MET-huFc with EC50 values of 3.8 ± 0.2 nM for eFab1, 2.1 ± 0.1 nM for eFab2 and 1.5 ± 0.1 nM for Fab, similar as described for a one-armed anti-Met 5D5 antibody ().Citation18 In summary, both eFab configurations were produced in mammalian cells and efficiently assembled into dimeric Fab-like molecules retaining antigen-binding activity.
Generation of bispecific and bivalent eIg molecules
The anti-MET eFab molecules were used in combination with an anti-HER3 Fab arm and a heterodimerizing Fc region to generate bivalent bispecific Ig-like molecules (eIgs).Citation19,Citation20 Two types of eIg molecules were produced. In eIg1, the eFab1 fragment directed against MET was fused through the EHD2-1 domain to a human γ1 CH2-CH3hole chain and the anti-HER3 Fab through the CH1 domain to a complementary human γ1 CH2-CH3knob chain. In eIg2, the eFab2 fragment directed against MET was fused through the EHD2-2 domain to a human γ1 CH2-CH3hole chain and the anti-HER3 Fab through the CH1 domain to a complementary human γ1 CH2-CH3knob chain (). The eIg molecules were produced in transiently co-transfected HEK293-6E cells and purified with protein A with yields of 21.3 mg/L (eIg1) and 17.9 mg/L (eIg2). SDS-PAGE analysis of the purified molecules showed one band under non-reducing conditions at ~200 kDa representing the tetrameric assembled bispecific antibody with a calculated molecular mass of 147.2 kDa. Under reducing conditions, we observed bands between 52 and 60 kDa and between 24 and 30 kDa for both eIg molecules corresponding to the two different heavy and light chains (). In SEC analysis, both eIg molecules showed a purity of 93% and 92%, respectively (). By dynamic light scattering an aggregation temperature of 64°C was determined for both molecules ().
Figure 3. Generation of eIg molecules with dual specificity and bivalency. (a) Schematic presentation of the used light (LC) and heavy chain (HC) in these molecules. (b) Schematic illustration of the two different eIg molecules that are specific for MET and HER3. The eIg molecules were generated with different MET-specific hetEHD2 domains (eIg1: VH5D5-EHD2-1-CH2-CH3hole + VL5D5-EHD2-2; eIg2: VH5D5-EHD2-2-CH2-CH3hole + VL5D5-EHD2-1) in combination with the HER3-specific VH3-43-CH1-CH2-CH3knob and VL3-43-CLk. (c) SDS-PAGE analysis of the protein A purified eIg molecules under reducing (R) and non-reducing (NR) conditions (12% PAA, 6 µg for R, 4 µg for NR, M: marker). (d) SEC profile with a TSKgel SuperSW mAb HR column under native conditions of the purified eIg molecules using HPLC analysis. (e) Determination of the aggregation point by dynamic light scattering (DLS) of the purified eIg molecules. Dotted line indicates the aggregation point. (f) ELISA binding experiments using either MET-huFc or HER3-His fusion protein as immobilized antigen. Bound antibodies were detected either with anti-human Fab (MET-huFc) or with an anti-human Fc (for HER3-His) as detection antibodies. (g) Sandwich-ELISA testing binding of HER3-moFc to titrated eIg molecules bound to immobilized MET-huFc as antigen. Bound HER3-moFc was detected with an HRP-conjugated anti-mouse Fc detection antibody. Mean ± SD; n = 3.
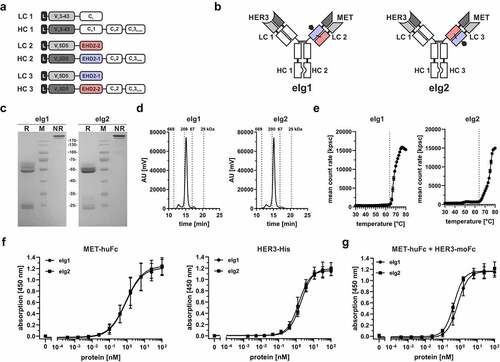
A non-glycosylated eIg1 molecule was further analyzed by liquid chromatography-mass spectrometry (LC-MS), demonstrating that the major component (93%) of the sample corresponds to the intact bispecific antibody molecule composed of the two different heavy and two different light chains, with 7% of the molecules composed of the two different heavy chains and two VL-EHD2-2 chains (Fig. S1A +B). In this analysis small amounts of free cysteinylated VL-EHD2-2 molecule were identified (data not shown). This bispecific antibody was additionally subjected to an affinity chromatography polishing step using CaptureSelect Lambda XP resin for the purification of bispecific antibodies comprising a natural Fab arm. An LC-MS analysis of this two-step purified eIg1 revealed complete removal of incorrectly assembled molecules and the absence of mispaired species (Fig. S1C). Antigen-binding activity was confirmed in ELISA using MET-huFc or His-tagged HER3 as immobilized antigens. Both eIg molecules showed a concentration-dependent binding with EC50 values of 1.1 nM to MET-Fc (with anti-human Fab detection antibody) and 1.5 nM (eIg1) and 2.0 nM (eIg2) to HER3 (with anti-human Fc detection antibody) using eIg1 and eIg2 molecules (). Finally, binding to both antigens was confirmed in sandwich-ELISA using MET-huFc as immobilized antigen, titrated eIg molecules and HER3-moFc as soluble antigen with EC50 values of 0.7 nM (eIg1) and 0.5 nM (eIg2) (). In summary, both eFabs could be applied in combination with normal Fab arms to efficiently generate bivalent bispecific Ig-like molecules. Because differences between eFab1 and eFab2 were marginal, we decided to use EHD2-1 for the generation of heavy chain fusions and EHD2-2 for making light chain equivalents.
Generation of bispecific bivalent or tetravalent Fab-eFab fusion proteins
Next, we combined an anti-EGFR targeting Fab (hu225, a humanized version of cetuximab) with the anti-HER3 targeting eFab to generate bispecific molecules for dual targeting of these two epidermal growth receptors. In a first approach, a bivalent bispecific Fab-eFab fusion protein was generated by fusing the VH-CH1 chain of the Fab through a flexible 10 amino acid glycine-serine linker to the VH-EHD2-2 chain (HC1) co-expressed with the corresponding light chains LC1 and LC2. In a second approach, a tetravalent bispecific Fab-eIg fusion protein was generated by further fusing the HC1 to a human γ1 Fc chain (HC2) (). Proteins were produced in transiently co-transfected HEK293-6E cells and purified either with IMAC (Fab-eFab) or protein A affinity chromatography (Fab-eIg) with yields of 1.2 mg/L for Fab-eFab and 0.5 mg/L for the Fab-eIg. In SDS-PAGE analysis, two major bands at 130 and 100 kDa for Fab-eFab and one major band at ~250 kDa for Fab-eIg molecule were observed under non-reducing conditions, with calculated molecular masses of 96.9 kDa for Fab-eFab and 242.2 kDa for the Fab-eIg molecule. Under reducing conditions, we observed two single bands at 24 kDa and 26 kDa representing the two light chains LC1 and LC2 and one band at 55 kDa for Fab-eFab and at ~75 kDa for Fab-eIg molecule corresponding to the HC1 and HC2, respectively (). Integrity was further confirmed by SEC analysis showing one peak with a RS of 4.5 nm (100 kDa) for Fab-eFab and 6.7 nm (440 kDa) for the Fab-eIg molecule (). For the Fab-eIg molecule, there was also a small shoulder in the SEC analysis.
Figure 4. Generation of different antibody geometries using hetEHD2. (a) Schematic presentation of the used light (LC; LC1: VL3-43-EHD2-2; LC2: VLhu225-CLk) and heavy chain (HC; HC1: VHhu225-CH1-VH3-43-EHD2-1; HC2: VHhu225-CH1-VH3-43-EHD2-1-CH2-CH3) for the Fc-less Fab-eFab (1 + 1) and tetravalent Fab-eIg (2 + 2) molecules. (b) Schematic illustration of the Fc-less Fab-eFab (1 + 1) and the Fab-eIg (2 + 2) specific for EGFR and HER3. (c) SDS-PAGE analysis of the Fab-eFab and Fab-eIg molecule using reducing (R) and non-reducing (NR) conditions. (12% PAA; 6 µg for R; 4 µg for NR; M: marker). (d) SEC profile of the Fab-eFab and the Fab-eIg molecule with a TSKgel SuperSW mAb HR column analyzed by HPLC. (e) ELISA binding experiment using either EGFR-His or HER3-His as immobilized antigen. Bound antibodies were detected with an anti-human Fc detection antibody. Parental antibodies were included as control. (f) Sandwich-ELISA testing binding of HER3-moFc to titrated Fab-eFab or Fab-eIg molecule bound to immobilized EGFR-huFc as antigen. Bound HER3-moFc was detected with an HRP-conjugated anti-mouse Fc detection antibody. Parental antibodies were included as control. (g) Flow cytometry analysis of both eIg molecules using FaDu cells. Bound antibodies were detected with PE-labeled anti-His (Fab-eFab) or anti-human Fc (Fab-eIg) antibodies. Parental antibodies were included as control. (h) Proliferation assay of Fab-eFab (100 nM) and Fab-eIg (50 nM) using FaDu cells in starvation medium after incubation of 5 days. Cells were kept unstimulated. Parental antibodies were included as control (single treatment: 50 nM; combination: 50 nM each). Cell viability was measured using CellTiterGlo 2.0. Mean ± SD; n = 3.
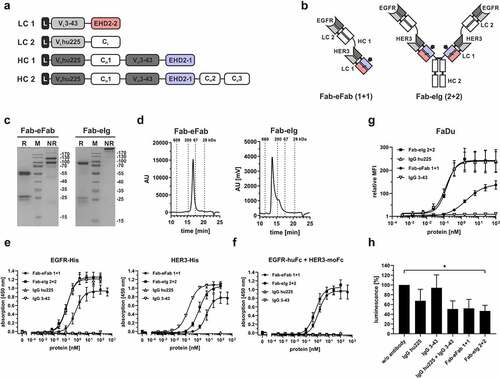
Antigen binding was confirmed by ELISA using the His-tagged extracellular domain of EGFR and HER3. The bivalent Fab-eFab molecule showed binding with EC50 values of 742 pM for EGFR and 6,204 pM for HER3, while the tetravalent Fab-eIg molecule showed an approximately 5-fold increased binding to both antigens (EC50 value of 132 pM for EGFR and 1,409 pM for HER3). The parental antibodies were included as control. Here, IgG hu225 showed similar binding to EGFR with EC50 value of 126 pM as the tetravalent Fab-eIg molecule, while binding of IgG 3–43 to HER3 was approximately 11-fold stronger (EC50 value of 127 pM) (). Furthermore, sequential binding has been shown in a sandwich-ELISA confirming binding to both antigens (). As expected, the parental antibodies did not show binding to both antigens. Thus, Fab-eFab and Fab-eIg molecules were efficiently produced and assembled into functional molecules.
The effects of dual targeting of EGFR and HER3 were analyzed using FaDu cells with high EGFR-expression (~140,000 EGFR/cell) and low HER3-expression (~3,000 HER3/cell).Citation19 The tetravalent bispecific Fab-eIg molecule and the parental antibodies IgG hu225 showed strong binding to FaDu cells, with EC50 values of 139 pM and 127 pM, respectively, while binding of the bivalent bispecific Fab-eFab was approximately 15-fold weaker (EC50 value of 1,882 pM). IgG 3–43 showed very strong binding with an EC50 value of 9 pM, in accordance with previous findings, although with a low mean fluorescence intensity due to low HER3 expression levels ().Citation19 Bioactivity of the Fab-eFab and Fab-eIg molecules was further analyzed in a proliferation assay. Here, the tetravalent Fab-eIg molecule showed strong reduction of proliferation by approximately 55%, significantly different to the untreated control. The bivalent Fab-eFab molecule and the combination of both parental antibodies showed a similar reduction of proliferation by approximately 50%. IgG hu225 also showed a reduction in proliferation by 35%, while the anti-HER3 antibody showed only marginal reduction of proliferation by 5% (, ). In summary, both the Fab-eFab and Fab-eIg retained antigen-binding activity and were able to inhibit cell proliferation similar to the combination of the parental antibodies.
Table 1. Biochemical properties and bioactivity of eIg molecules targeting EGFR and HER3. Mean ± SD, n = 3, n.d.: not determined
Generation of bispecific bi- or trivalent T-cell engagers
Bispecific T-cell engagers were generated by combining one humanized anti-CD3 eFab (huU3) arm with one or two Fab arms directed against EGFR (hu225), respectively, using a bivalent eIg (1 + 1) or trivalent Fab-eIg (2 + 1) format. The Fc regions were modified to allow heterodimerization and ablate Fc effector functions ().Citation20,Citation21 Both proteins were purified with protein A chromatography with yields between 8.3 mg/L for eIg (high molecular weight (HMW) fraction: 7.7%) and 4.3 mg/L for Fab-eIg (HMW fraction: 6.3%), followed by a preparative SEC to remove the HMW species. SDS-PAGE analysis under reducing conditions revealed in total four different chains at 60 to 65 kDa representing the VHhuU3-EHD2-1-CH2-CH3knob (HC2), at 55 kDa representing the VHhu225-CH1-CH2-CH3hole (HC1), and at 25 kDa representing both light chains (VLhu225-CLκ (LC1) and VLhuU3-EHD2-2 (LC2)). Similarly, at 55 kDa the VHhu225-CH1-CH2-CH3hole (HC1) and both light chains were observed at 25 kDa, while the second heavy chain was observed at 80 kDa representing the VHhu225-CH1-VHhuU3-EHD2-1-CH2-CH3knob (HC3) (). Under non-reducing conditions one band of ~180 kDa was observed for the bivalent eIg molecule and of ~220 kDa for the trivalent molecule. Of note, in SEC analysis both molecules showed one single peak corresponding to a RS of approximately 5.4 nm (170 kDa) for the eIg and a RS of approximately 6.1 nm (241 kDa) for the Fab-eIg molecule ().
Figure 5. Generation of bispecific EGFR and CD3 eIg molecules with different geometry. (a) Schematic presentation of the used light (LC; LC1: VLhu225-CLκ; LC2: VLhuU3-EHD2-2) and heavy chain (HC; HC1: VHhu225-CH1-CH2-CH3hole; HC2: VHhuU3-EHD2-1-CH2-CH3knob; HC3: VHhu225-CH1-VHhuU3-EHD2-1-CH2-CH3knob) for the eIg molecules either in the 1 + 1 (LC1+ LC2+ HC1+ HC2) or in the 2 + 1 (LC1+ LC2+ HC1+ HC3) configuration. (b) Schematic illustration of the bispecific bivalent (1 + 1) eIg and the bispecific tetravalent Fab-eIg (2 + 1) specific for EGFR and CD3. (c) SDS-PAGE analysis of the eIg and Fab-eIg molecule using reducing (R) and non-reducing (NR) conditions (gradient gel; 4 µg of protein; M: marker; 1: eIg; 2: Fab-eIg). (d) SEC profile of the eIg (1 + 1) and the Fab-eIg (2 + 1) molecule with a TSKgel SuperSW mAb HR column analyzing in HPLC. (e) ELISA binding experiment using EGFR-mouse Fc as immobilized antigen. Bound antibodies were detected with an anti-human Fc detection antibody. Mean ± SD; n = 3. (f + g)G Flow cytometry analysis analyzing binding of the eIg (1 + 1) and Fab-eIg (2 + 1) molecules to CD3-positive Jurkat (f) or EGFR-positive LIM1215 and FaDu cells (g). Mean ± SD; n = 3. (h) Conjugate formation assay using 100 nM of the bispecific antibody in combination with CD3-expressing Jurkat and EGFR-expressing FaDu cells. Simultaneous binding to CD3- and EGFR-expressing cells is shown. Mean ± SD, n = 3. (i) Cytotoxic potential of PBMCs stimulated with eIg molecules. LIM1215 or FaDu cells were incubated with a serial dilution of eIg molecules in presence of PBMCs in an effector:target cell ratio E:T of 10:1. Cell viability was analyzed by crystal violet staining. Mean ± SD; n = 2.
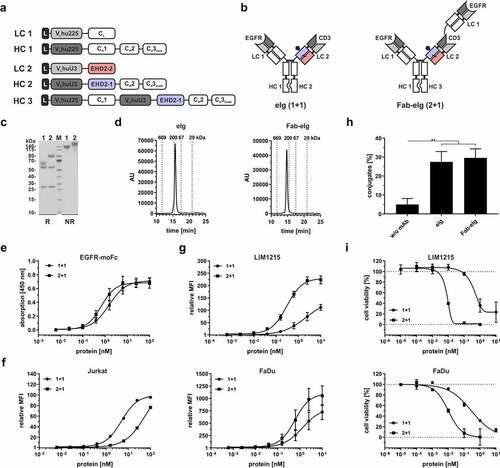
Concentration-dependent binding of both molecules to EGFR was shown in ELISA, with EC50 values of 1.6 nM for the bivalent molecule and 0.7 nM for the trivalent molecule with two binding moieties for EGFR (). Binding to CD3 was analyzed via flow cytometry analysis using CD3-expressing Jurkat cells. The bivalent eIg molecule showed strongest binding to Jurkat with EC50 values of ~4.5 nM, while the trivalent molecule showed reduced binding with EC50 value of ~54 nM (). Furthermore, both molecules showed binding to tumor cell lines expressing moderate amounts of EGFR (LIM1215; ~36,000 EGFR/cell) and high amounts of EGFR (FaDu; ~140,000 EGFR/cell). Due to the avidity effect, the trivalent Fab-eIg molecules showed strongest binding with EC50 values between 0.3 and 0.6 nM, while the eIg molecules showed 3- to 10-fold reduced binding (). Crosslinking of the CD3-expressing Jurkat cells and the EGFR-expressing FaDu cells by the bispecific antibodies was detected by flow cytometry in a conjugate formation assay (). Here, incubation with 100 nM of the bispecific antibodies led to conjugate formation between the cells of ~28% for the eIg and of ~30% for the Fab-eIg (compared to ~5% without antibody). Finally, the cytotoxic effects of peripheral blood mononuclear cells (PBMCs) on LIM1215 and FaDu cells (effector to target cell ratio (E:T) of 10:1) mediated by the eIg molecules was determined. For both cell lines, concentration-dependent activity was detected with increased potency for the trivalent Fab-eIg molecule on LIM1215 cells with 8.5 pM (EC50 value of eIg 1,231 pM; 144-fold increased) and FaDu cells with 10.5 pM (EC50 value of eIg 282 pM; 26-fold increased) () (). In summary, we generated two different molecules capable of binding to CD3 and EGFR that efficiently induced T-cell-mediated tumor cell killing.
Table 2. Binding and bioactivity of eIg molecules targeting EGFR and CD3. Mean ± SD, n = 3
Lack of binding of eIg molecules to Fcε receptors
Crosslinked IgE molecules are prominent activators of both Fcε receptors, FcεRI and FcεRII (CD23), which can lead to effector phase activation of mast cells and B-cell differentiation.Citation21,Citation22 Here, we analyzed whether eIg molecules, comprising the heterodimerizing CH2 domain of IgE, are able to interact with the two Fcε receptors. In a first experiment, we tested binding of a Fab-eFab, lacking an Fc region, and a bispecific tetravalent Fab-eIg, comprising a wild-type human γ1 Fc region, to both Fcε receptors (). No binding to both Fcε receptors was observed for the analyzed eIg molecules, while human IgE included as control showed strong binding to both Fcε receptors. Furthermore, the parental IgG1 antibodies hu225 and 3–43 included as further controls showed binding to EGFR or HER3, but not to the Fcε receptors. Thus, we could demonstrate that hetEHD2-comprising molecules lack binding to FcεRI and FcεRII and should therefore not be able to induce receptor crosslinking.
Figure 6. Binding of hetEHD2 to Fcε receptors. Binding of different eIg molecules for dual targeting approach (EGFR and HER3 specific) were used in ELISA experiments. Here, we used immobilized Fcε receptor I fused to mouse Fc and Fcε receptor II fused to His-tag as immobilized antigens and tested binding to these receptors. As control, we also included IgE molecule that binds to both receptors and the parental antibodies IgG hu225 and IgG 3–43. Additionally, bioactivity of the eIg molecules was confirmed by using EGFR and HER3 as Fc fusion proteins. Mean ± SD, n = 3.
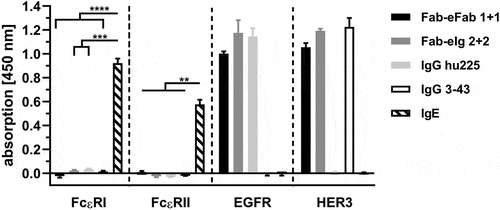
Discussion
The heavy chain domain 2 of IgE (EHD2, Cε2) is a versatile dimerization domain for the generation of dimeric fusion proteins.Citation9 The domain is fully human, forms covalently linked dimers, and lacks effector functions.Citation22 Because of its natural function as a hinge domain between the Fabs and the CH3-CH4 domains mediating the IgE Fc effector functions, it is ideally suited to fuse additional polypeptides to the N- and/or C-terminus. Structurally, it closely resembles the CH1-CL domain of Fab arms, with a similar positioning of the N-termini. Here, we provide further evidence that the CH1 and CL domains can be substituted by the EHD2 domain without affecting the formation of functional antigen-binding sites. Of note, our examples demonstrate that no further engineering is required for the VH and VL domains or to connect these domains to the EHD2 domains. To force heterodimerization of the EHD2-comprising Fab chains (eFabs), we showed that substitution of one of the two interdomain crossover disulfide bonds is sufficient. In the study reported here, we substituted Cys14 in one domain and Cys102 in the other domain by serine. Thus, only two mutations, one in each domain, have to be introduced at the domain interface. Cooke et al. recently reported the use of modified EHD2 domains to generate Fab-like molecules (EFabs).Citation23 Here, the two disulfide bonds were maintained and two mutations, T121G in the first domain and S10I in the second domain, were introduced, resembling a knobs-into-holes strategy. Interestingly, the crystal structure of an adalimumab EFab revealed an asymmetric bending of the Cε2 dimer with respect to the VH/VL pseudo-axis and the appearance of an interface between the VH and Cε2 domains.Citation23 This disorder also made the EFab more susceptible for proteolysis. It was postulated that this disorder is not caused by the knob-and-hole heterodimerization mutations, which was well resolved in the crystal structure, but by structural constraints imposed on the Cε2 domain by the VH domain. Currently, no crystal structures are available for our eFab molecules. It should be noted, however, that the sequences connecting the VH and VL domain to the hetEHD2 domains differ slightly from that described by Cooke and coworkers. Thus, the hetEHD2 domains start at position 234 (DFTPPTV …), while in the EFabs they start with position 238 (PTV …) based on the Kabat numbering of IgE molecules.Citation16 Furthermore, in our molecules the C-terminus is slightly longer ending with amino acid 341 (… KCADSN), while EFabs end at position 338 (… KCA) (). This might have effects on the structural organization of our eFab building blocks.
The question arises as to why it is sufficient to simply remove one of the two disulfide bonds to force heterodimerization. Analysis of the crystal structure of the human IgE Fc region (Cε2-Cε4) revealed for the Cε2 domain a distinct mode of domain association compared to other immunoglobulin constant domains, such as Cε1:CL, Cε3:Cε3, or Cε4:Cε4.Citation15 It was found that the Cε2:Cε2 buried surface area is much smaller and dominated by polar rather than the usual nonpolar residues, involving 11 hydrogen bonds and additional 10 bridged by water molecules. Furthermore, in another study it was described that in the absence of the two interdomain disulfide bonds the Cε2 domains have no measurable affinity for each other.Citation24 Thus, disulfide bond formation is required for a stable domain association. Consequently, the EDH2-1 or EDH2-2 domains, respectively, will not be able to assemble into stable homodimers because no disulfide bonds between two identical domains can be formed, and only the heterodimers will be covalently linked.
Various eFabs derived from one human λ-antibody (3–43 directed against HER3) and two humanized κ-antibodies (5D5 directed against MET, huU3 directed against CD3) were combined with various Fabs (human anti-HER3 antibody 3–43, humanized anti-EGFR antibody hu225) to generate different bispecific antibodies, including an Fc-less Fab-eFab molecule and various Ig-like bispecific molecules with 2, 3, or 4 valencies. This demonstrates that the eIg technology can be applied to parental antibody sequences of different origin and can also be combined with normal Fabs without affecting correct pairing of Fabs and eFabs with their cognate heavy chain sequences. Furthermore, the various eIg molecules generated in this study showed expression yields and purities after a one-step protein A chromatography comparable to IgG molecules. Thus, the eIg platform should allow manufacturing of bispecific antibodies with drug-like properties using established up- and downstream processes.
The LC-MS data identified 93% of correctly assembled bispecific eIg1 antibody of the protein A purified molecule. The 7% mispaired fraction resulted from incorrect assembly of the VH3-43-CH1-Fc(knob)WT heavy chain and the VL5D5-EHD2-2 light chain. For a bivalent eIg molecule, these unwanted side products could be efficiently removed by an additional lambda light chain affinity chromatography polishing step, resulting in 100% correctly assembled bispecific molecules. All of our bispecific eIg-based antibodies comprise eFab moieties and additionally natural Fab moieties with the light chain constant domains of either a lambda or kappa isotype. Thus, a polishing step with one additional affinity chromatography based on capturing of CL chains can be applied to remove species with incorrectly paired chains from any eIg molecule. Mispaired light chains to various extend were also described for other technologies after protein A affinity chromatography.Citation8,Citation25–27 The formation of mispaired light chains of the eIgs might be due to the low amounts of free cysteinylated molecules of this light chain. Thus, in this oxidized form the VL5D5-EHD2-2 light chain will not be able to form stable heterodimers with the corresponding heavy chain formation, but rather interact with its VL domain with one of the VH domains of the heterodimeric heavy chains. These mispaired species might be avoided by optimizing the ratio of the plasmids encoding for the different chains in the transfection procedure, or removed by using an additional affinity chromatography purification step that incorporates, for example, affinity resins specific for the constant kappa or lambda light chain, as shown here.
Applications of bispecific eIg molecules that we generated in this study include dual receptor targeting and the retargeting of T cells to tumor cells. Thus, we could demonstrate that a 1 + 1 Fab-eFab as well as a 2 + 2 Fab-eIg molecule directed against EGFR and HER3 retained the antigen-binding activity of the parental antibodies, leading to efficient inhibition of cell proliferation, although ELISA data indicate that binding of the inner eFab arm is reduced. This might be caused by steric hindrance of binding caused by the outer Fab arm in accordance with findings for other tetravalent bispecific antibody formats, e.g., DVD-Ig or COVD-Ig.Citation28,Citation29 Further engineering of the connecting linker sequences or swapping of the antigen-binding sites might improve binding of the inner eFab arms. Nevertheless, sequential binding was confirmed in our study for the 1 + 1 and 2 + 2 molecules, demonstrating that both antigens can be bound simultaneously.
Bispecific eIgs directed against EGFR and CD3 were able to retarget T cells to EGFR-expressing tumor cells, leading to efficient killing of target cells. Here, the 2 + 1 Fab-eIg was more potent than the 1 + 1 eIg, in accordance with findings for 2 + 1 Fab-IgGs targeting HER2 and CD3.Citation30 These 2 + 1 bispecific antibodies might be especially useful to improve tumor selectivity by increased target-cell binding, resulting in an avidity-mediated specificity gain. Thus, we have recently shown that an Fc-less trivalent bispecific scDb-scFv fusion protein targeting HER3 and CD3 exhibited strongly increased binding and cytotoxicity compared to a bivalent bispecific scDb.Citation31
An additional feature of the EHD2 domains is the presence of an N-glycosylation site. In our study, we removed the N-glycosylation site in one of the hetEHD2 domains to allow better discrimination of glycosylated and non-glycosylated chains. Preliminary data show that eIg molecules can be produced comprising both N-glycosylation sites, only one or none, indicating that the presence or absence of these site does not affect expression and assembly of the bispecific antibodies.
The natural N-glycans within the hetEHD2 domains might be useful for site-directed modification of bispecific antibodies, such as conjugation with drugs to generate antibody-drug conjugates (ADCs), including antibodies lacking an Fc region and, thus the CH2-comprising N-glycans.Citation32 Several bispecific ADCs are currently under investigation, including a bispecific antibody combining specificities for a HER2 and the prolactin receptor (PRLP) for efficient internalization and increased cytotoxicity and a bispecific ADC targeting HER2 and CD63 to increase the lysosomal degradation of the molecule.Citation33,Citation34
The Fc region of IgE is capable of binding with high affinity to FcεRI expressed on immune cells such as mast cells and basophils and with lower affinity to FcεRII.Citation35 Recent studies showed that the conformation of the Fc part of IgE affects binding to the FcεRs, with binding to FcεRI by an “open” state and binding to FcεRII by a “closed” conformation.Citation22 The CH3 domain of the IgE molecule was identified as major interaction site in binding FcεRICitation36,Citation37 and FcεRII.Citation36,Citation37 The CH2 domain does not contribute to binding to FcεRII and interacts extremely weakly with FcεRI (Kd of 200 µM).Citation24 Here, we could confirm that eIg molecules comprising the CH2 domain of IgE (EHD2) lack binding to the two Fcε receptors and are, thus, most likely not able to induce receptor cross-linking. Nevertheless, further cell-based assays might be necessary to confirm this in a cellular context. Furthermore, because the EHD2 is fully human, with the two cysteine mutations located at the domain interface, it is rather unlikely that anti-drug antibodies directed against the EHD2 are formed when administered to humans, especially since the only described allotype Em1 does not reside in the CH2 domain.Citation38
In summary, we established the eIg technology as a versatile platform for the generation of Ig-like bispecific antibodies of varying valency, geometry, and composition, which complements various other approaches to solve the light chain problem in the generation of bispecific Ig-like molecules. Our results provide first evidence that variable domains of different specificity and origin, for example, humanized and fully human, can be fused to the hetEHD2 domain without affecting correct assembly into functional Fab-like moieties. Further studies are warranted to demonstrate efficacy and safety for therapeutic applications.
Materials and methods
Antibody production and purification
Heavy and light chains of different antibody molecules were cloned into variants of pSecTagA vector, and were transiently transfected into HEK293-6E suspension cells using polyethyleneimine (PEI; linear, 25 kDa, Sigma-Aldrich) for transfection. HEK293-6E cells were provided by National Research Council of Canada (Ottawa, Canada), and cultivated in F17 Freestyle expression medium (ThermoFisher) supplemented with 0.1% Kolliphor P-118 (Sigma), 4 mM GlutaMAX (ThermoFisher), and 25 µg/ml G418. By adding TN1 (20% tryptone N1 (Organotechnie S.A.S., France) in F17 medium) to feed the culture 24 hours post transfection, protein production was initiated; cells were cultivated for additional 4 days at 37°C. Supernatants were harvested, and antibody molecules were either dialyzed against phosphate-buffered saline (PBS) and purified by IMAC for His-tag molecules, or purified via CaptureSelect CH1 or protein A affinity chromatography also in combination with CaptureSelect Lambda XP resin (ThermoFisher). Eluted preparations were dialyzed against PBS at 4°C. For the preparation of CD3-containing T-cell engager, proteins were further purified with a preparative SEC using FPLC analysis.
Antibody characterization
Antibodies were analyzed by SDS-PAGE (4 or 6 µg of molecule) and stained with Coomassie-Brilliant Blue G-250. The purity and integrity of molecules (30 µl) were analyzed via SEC using a Waters 2695 HPLC in combination with a TSKgel G2000SWXL (for eFab molecules) or with a TSKgel SuperSW mAb HR column (for all other molecules) (Tosoh Bioscience) at a flow rate of 0.5 ml/min using 0.1 M Na2HPO4/NaH2PO4, 0.1 M Na2SO4, pH 6.7 as mobile phase. Standard proteins: thyroglobulin (669 kDa, RS 8.5 nm), β-amylase (200 kDa, RS 5.4 nm), bovine serum albumin (67 kDa, RS 3.55 nm), carbonic anhydrase (29 kDa, RS 2.35 nm). Stokes radii and molecular weight of analyzed antibodies were interpolated from standard proteins.
Thermal stability
ZetaSizer Nano ZS (Malvern) was used to analyze the thermal stability of the proteins by dynamic light scattering. Purified protein was exposed to increasing temperature (30°C to 80°C) in 1°C intervals with 2-minute equilibration steps. The aggregation point was defined by the starting point of the increase in the mean count rate.
Analysis of protein integrity by LC-MS
To determine the molecular composition of a bispecific eIg, the sample was N-deglycosylated and analyzed in non-reduced state by LC-MS. For that, 25 µg of the antibody (1.25 µg/µL) were diluted 1:4 (v:v) with non-reducing sample buffer provided with the RapidTMPNGase F enzyme kit (New England Biolabs, #P0711) and incubated for 5 minutes at 75°C. 0.3 µL RapidTMPNGase F enzyme were added and deglycosylation was performed over night at 37°C. Thereafter, the sample was diluted with histidine/NaCl buffer (pH 6.0, 20 mM histidine, 140 mM NaCl) to a final volume of 62.5 µL. The N-deglycosylated antibody was analyzed by liquid chromatography (HPLC) coupled to electrospray ionization (ESI) quadrupole time-of-flight (QTOF) MS. 4 μl sample were desalted using reversed phase chromatography on a Dionex U3000 RSLC system (Thermo Scientific, Dreieich, Germany). The separation was performed on an Acquity BEH300 C4 column (1 × 50 mm; 1.7 μm particle diameter, Waters) at 75°C and 150 μl/min applying a 7-min linear gradient with varying slopes. In detail, the gradient steps were applied as following (min/% Eluent B): 0/5, 0.4/5, 2.55/30, 7/50, 7.5/99, 8/5, 8.75/99, 9/99, 9.5/5). Eluent B was acetonitrile (Honeywell, LCMS grade quality) with 0.1% formic acid, and solvent A was water (Honeywell, LCMS grade quality) with 0.1% formic acid. Continuous MS detection was performed using a QTOF mass spectrometer (Maxis UHR-TOF; Bruker, Bremen, Germany) with an ESI source operating in positive ion mode. Spectra were taken in the mass range of 1000–4000 m/z. External calibration was applied by infusion of tune mix via a syringe pump during a short time segment at the beginning of the run. Raw MS data were lock-mass corrected (at m/z 1221.9906) and further processed using Data Analysis 5.3 and MaxEnt Deconvolution software tools (Bruker).
Enzyme-linked immunosorbent assay
96-well plates were coated with MET-huFc (extracellular domain of MET: aa 25–787 with Fc fusion), EGFR-huFc, EGFR-moFc or EGFR-His (ECD of EGFR: aa 25–645 with C-terminal Fc fusion or His-tag), HER3-moFc or HER3-His (ECD of HER3: aa 20–643 with C-terminal Fc fusion or His-tag), or with FcεRI-moFc (ECD of FcεRI: aa 26–205 with C-terminal Fc fusion protein), or with His-tagged FcεRII (ECD of FcεRII: aa 48–321 with N-terminal His-tag) (200 ng/well in PBS) overnight at 4°C and residual binding site were blocked with 2% (w/v) skim milk in PBS (MPBS, 200 µl/well). The antibodies were diluted in MPBS and titrated in duplicates starting from 400 or 100 nM and incubated for 1 hour at room temperature. For sequential binding of both antigens, HER3-moFc was added to wells for one additional hour at room temperature (RT). Bound antibodies were detected with horseradish peroxidase (HRP)-conjugated antibodies specific for His-tag (for eFab molecules; sc-8036, Santa Cruz Biotechnology), specific for human Fab (for bispecific antibodies and IgE molecule; 015M4761V, Sigma Aldrich), specific for human Fc (for the bispecific antibodies; A0170, Sigma Aldrich) or mouse Fc (for the sequential binding; A2554, Sigma Aldrich). Detection antibodies were incubated for one additional hour at room temperature. TMB (1 mg/ml; 0.006% (v/v) in 100 mM Na-acetate buffer, pH 6) was used as substrate, reaction was terminated using 50 µl 1 M H2SO4 and absorption was measured at a wavelength of 450 nM. In general, plates were washed three times with PBST (PBS + 0.005% Tween 20) and twice with PBS between each incubation step and in advance of the detection.
Flow cytometry analysis
CD3-expressing Jurkat cells or EGFR-expressing tumor cell lines (LIM1215, FaDu) (1x105 per well) were incubated with a serial dilution of different antibodies (starting from 10 or 100 nM) for 1 hour at 4°C. Incubation and washing steps were performed in PBS, 2% fetal bovine serum (FBS), and 0.02% sodium azide. After washing the cells twice, bound antibodies were detected using a R-phycoerythrin (PE)-labeled anti-human Fc antibody (109–115-098, Jackson ImmunoResearch). Flow cytometry was performed using MACSQuant Analyzer 10 or MACSQuant VYB (both Miltenyi Biotec) and data were analyzed using FlowJo (Tree Star). Relative mean fluorescence intensities (MFI) were calculated as followed: rel. MFI = ((MFIsample-(MFIdetection-MFIcells))/MFIcells).
Cell proliferation assays
FaDu cells (2x103 per well) were seeded in 96-well plate and cultivated at 37°C in rich-medium (10% FBS) for 24 hours, and subsequent medium exchange to starvation medium (0.2% FBS and 1x penicillin/streptomycin). After 24 hours of starvation, cells were treated with different eIg antibodies (1 + 1: 100 nM; 2 + 2: 50 nM each) or with the parental antibodies (50 nM) for 60 minutes at 37°C. Cells were kept unstimulated and incubated for 5 days at 37°C. Cell viability was measured using CellTiter-Glo 2.0 Assay (Promega). Luminescence of untreated cells was set as 100%.
Cytotoxicity
Previously seeded target cells (2x104 cells/well; LIM1215, FaDu) were incubated with a serial dilution of the bispecific antibodies for 15 min at RT prior to addition of PBMCs (E:T ratios of 10:1). After 3 days, supernatants were discarded and viable target cells were stained with crystal violet. Staining was solved in methanol (50 µl/well) and optical density measured at 550 nm using the Tecan spark (Tecan).
Conjugate formation assay
To determine simultaneous binding of the eIg molecules, CD3-expressing Jurkat cells and EGFR-expressing FaDu cells were stained with either carboxyfluorescein diacetate succinimidyl ester (CFSE) or Far Red at 625 nM/1x106 cells/ml following the manufacturer’s instructions. 5 × 104 cells/well from each cell line were mixed in a 1:1 ratio with 100 nM of the respective protein. After incubation for 30 min at 37°C, 5% CO2, formed cell conjugates were determined in flow cytometry.
Statistics
All data are represented as mean ± SD. Significances were calculated using GraphPad Prism 7.0 and results were compared by one-way ANOVA followed by Tukey’s multiple comparison test (posttest) or t-test. p < .05 (*), p < .01 (**), p < .001 (***), p < .0001 (****) and n.d. (not determined).
Supplemental Material
Download Zip (1.1 MB)Acknowledgments
We would like to thank Nadine Heidel and Sabine Münkel for excellent technical assistance. We thank Dr. Anne Zeck (NMI - Natural and Medical Science Institute at the University of Tübingen) for performing the LC-MS analysis.
Disclosure statement
No potential conflict of interest was reported by the author(s). R.E.K. and O.S. are named inventors on a patent application covering the eIg technology.
Supplementary material
Supplemental data for this article can be accessed on the publisher’s website
Additional information
Funding
References
- Brinkmann U, Kontermann RE. Bispecific antibodies. Science. 2021;372(6545):916–13. PMID:34045345. doi:10.1126/science.abg1209.
- Labrijn AF, Janmaat ML, Reichert JM, Pwhi P. Bispecific antibodies: a mechanistic review of the pipeline. Nat Rev Drug Discov. 2019;18(8):585–608. PMID:31175342. doi:10.1038/s41573-019-0028-1.
- Brinkmann U, Kontermann RE. The making of bispecific antibodies. MAbs. 2017;9(2):182–212. PMID:28071970. doi:10.1080/19420862.2016.1268307.
- Spiess C, Zhai Q, Carter PJ. Alternative molecular formats and therapeutic applications for bispecific antibodies. Mol Immunol. 2015;67(2):95–106. PMID:25637431. doi:10.1016/j.molimm.2015.01.003.
- J-h H, Kim J-E, Kim Y-S. Immunoglobulin Fc heterodimer platform technology: from design to applications in therapeutic antibodies and proteins. Front Immunol. 2016;7:394. PMID:27766096. doi:10.3389/fimmu.2016.00394.
- Krah S, Sellmann C, Rhiel L, Schröter C, Dickgiesser S, Beck J, Zielonka S, Toleikis L, Hock B, Kolmar H, et al. Engineering bispecific antibodies with defined chain pairing. N Biotechnol. 2017;39:167–73. PMID:28137467. doi:10.1016/j.nbt.2016.12.010.
- Nie S, Wang Z, Moscoso-Castro M, D’Souza P, Lei C, Xu J, Gu J. Biology drives the discovery of bispecific antibodies as innovative therapeutics. Antib Ther. 2020;3(1):18–62. PMID:33928225. doi:10.1093/abt/tbaa003.
- Schaefer W, Regula JT, Bähner M, Schanzer J, Croasdale R, Dürr H, Gassner C, Georges G, Kettenberger H, Imhof-Jung S, et al. Immunoglobulin domain crossover as a generic approach for the production of bispecific IgG antibodies. Proc Natl Acad Sci U S A. 2011;108(27):11187–92. PMID:21690412. doi:10.1073/pnas.1019002108.
- Seifert O, Plappert A, Fellermeier S, Siegemund M, Pfizenmaier K, Kontermann RE. Tetravalent antibody-scTRAIL fusion proteins with improved properties. Mol Cancer Ther. 2014;13(1):101–11. PMID:24092811. doi:10.1158/1535-7163.MCT-13-0396.
- Seifert O, Plappert A, Heidel N, Fellermeier S, Messerschmidt SKE, Richter F, Kontermann RE. The IgM CH2 domain as covalently linked homodimerization module for the generation of fusion proteins with dual specificity. Protein Eng Des Sel. 2012;25(10):603–12. PMID:22988132. doi:10.1093/protein/gzs059.
- Hutt M, Marquardt L, Seifert O, Siegemund M, Müller I, Kulms D, Pfizenmaier K, Kontermann RE. Superior properties of Fc-comprising scTRAIL fusion proteins. Mol Cancer Ther. 2017;16(12):2792–802. PMID:28904131. doi:10.1158/1535-7163.MCT-17-0551.
- Dong F, Gu J, Ghayur T. IgM- or IgE-Modified binding proteins and uses thereof. 2016. US 2018 / 0194861 A1.
- Fischer R, Sendetski M, Del Rivero T, Martinez GF, Bracchi-Ricard V, Swanson KA, Pruzinsky EK, Delguercio N, Rosalino MJ, Padutsch T, et al. TNFR2 promotes Treg-mediated recovery from neuropathic pain across sexes. Proc Natl Acad Sci U S A. 2019;116(34):17045–50. PMID:31391309. doi:10.1073/pnas.1902091116.
- Seifert O, Rau A, Beha N, Richter F, Kontermann RE. Diabody-Ig: a novel platform for the generation of multivalent and multispecific antibody molecules. MAbs. 2019;11(5):919–29. PMID:30951400. doi:10.1080/19420862.2019.1603024.
- Wan T, Beavil RL, Fabiane SM, Beavil AJ, Sohi MK, Keown M, Young RJ, Henry AJ, Owens RJ, Gould HJ, et al. The crystal structure of IgE Fc reveals an asymmetrically bent conformation. Nat Immunol. 2002;3(7):681–86. PMID:12068291. doi:10.1038/ni811.
- Kabat EA, Wu, TT, Perry, HH, Gottesman, KS, Foeller, C. Sequences of proteins of immunological interest. In: Bd, edition 5 (pp. 91–3242). NIH Publication; 1991.
- Martens T, Schmidt N-O, Eckerich C, Fillbrandt R, Merchant M, Schwall R, Westphal M, Lamszus K. A novel one-armed anti-c-met antibody inhibits glioblastoma growth in vivo. Clin Cancer Res. 2006;12(20):6144–52. PMID:17062691. doi:10.1158/1078-0432.CCR-05-1418. PMID:17062691
- Merchant M, Ma X, Maun HR, Zheng Z, Peng J, Romero M, Huang A, Yang N, Nishimura M, Greve J, et al. Monovalent antibody design and mechanism of action of onartuzumab, a MET antagonist with anti-tumor activity as a therapeutic agent. Proc Natl Acad Sci U S A. 2013;110(32):E2987–96. PMID:23882082. doi:10.1073/pnas.1302725110.
- Schmitt LC, Rau A, Seifert O, Honer J, Hutt M, Schmid S, Zantow J, Hust M, Dübel S, Olayioye MA, et al. Inhibition of HER3 activation and tumor growth with a human antibody binding to a conserved epitope formed by domain III and IV. MAbs. 2017;9(5):831–43. PMID:28421882. doi:10.1080/19420862.2017.1319023.
- Merchant AM, Zhu Z, Yuan JQ, Goddard A, Adams CW, Presta LG, Carter P. An efficient route to human bispecific IgG. Nat Biotechnol. 1998;16(7):677–81. PMID:9661204. doi:10.1038/nbt0798-677.
- Rivera J, Olivera A. A current understanding of Fc epsilon RI-dependent mast cell activation. Curr Allergy Asthma Rep. 2008;8(1):14–20. PMID:18377769. doi:10.1007/s11882-008-0004-z.
- Sutton BJ, Davies AM, Bax HJ, Karagiannis SN. IgE antibodies: from structure to function and clinical translation. Antibodies (Basel). 2019;8(1):19. PMID:31544825. doi:10.3390/antib8010019.
- Cooke HA, Arndt J, Quan C, Shapiro RI, Wen D, Foley S, Vecchi MM, Preyer M. EFab domain substitution as a solution to the light-chain pairing problem of bispecific antibodies. MAbs. 2018;10(8):1248–59. PMID:30215570. doi:10.1080/19420862.2018.1519631.
- McDonnell JM, Calvert R, Beavil RL, Beavil AJ, Henry AJ, Sutton BJ, Gould HJ, Cowburn D. The structure of the IgE cepsilon2 domain and its role in stabilizing the complex with its high-affinity receptor Fcepsilonrialpha. Nat Struct Biol. 2001;8(5):437–41. PMID:11323720. doi:10.1038/87603.
- Lewis SM, Wu X, Pustilnik A, Sereno A, Huang F, Rick HL, Guntas G, Leaver-Fay A, Smith EM, Ho C, et al. Generation of bispecific IgG antibodies by structure-based design of an orthogonal Fab interface. Nat Biotechnol. 2014;32(2):191–98. PMID:24463572. doi:10.1038/nbt.2797.
- Wang C, Vemulapalli B, Cao M, Gadre D, Wang J, Hunter A, Wang X, Liu D. A systematic approach for analysis and characterization of mispairing in bispecific antibodies with asymmetric architecture. MAbs. 2018;10(8):1226–35. PMID:30153083. doi:10.1080/19420862.2018.1511198.
- Wu X, Sereno AJ, Huang F, Zhang K, Batt M, Fitchett JR, He D, Rick HL, Conner EM, Demarest SJ. Protein design of IgG/TCR chimeras for the co-expression of Fab-like moieties within bispecific antibodies. MAbs. 2015;7(2):364–76. PMID:25611120. doi:10.1080/19420862.2015.1007826.
- Digiammarino EL, Harlan JE, Walter KA, Ladror US, Edalji RP, Hutchins CW, Lake MR, Greischar AJ, Liu J, Ghayur T, et al. Ligand association rates to the inner-variable-domain of a dual-variable-domain immunoglobulin are significantly impacted by linker design. MAbs. 2011;3(5):487–94. PMID:21814039. doi:10.4161/mabs.3.5.16326.
- Steinmetz A, Vallée F, Beil C, Lange C, Baurin N, Beninga J, Capdevila C, Corvey C, Dupuy A, Ferrari P, et al. CODV-Ig, a universal bispecific tetravalent and multifunctional immunoglobulin format for medical applications. MAbs. 2016;8(5):867–78. PMID:26984268. doi:10.1080/19420862.2016.1162932.
- Slaga D, Ellerman D, Lombana TN, Vij R, Li J, Hristopoulos M, Clark R, Johnston J, Shelton A, Mai E, et al. Avidity-based binding to HER2 results in selective killing of HER2-overexpressing cells by anti-HER2/CD3. Sci Transl Med. 2018;10(463). PMID:30333240. doi:10.1126/scitranslmed.aat5775.
- Aschmoneit N, Steinlein S, Kühl L, Seifert O, Kontermann RE. A scDb-based trivalent bispecific antibody for T-cell-mediated killing of HER3-expressing cancer cells. Sci Rep. 2021;11(1):13880. PMID:34230555. doi:10.1038/s41598-021-93351-0.
- Zhu Z, Ramakrishnan B, Li J, Wang Y, Feng Y, Prabakaran P, Colantonio S, Dyba MA, Qasba PK, Dimitrov DS. Site-specific antibody-drug conjugation through an engineered glycotransferase and a chemically reactive sugar. MAbs. 2014;6(5):1190–200. PMID:25517304. doi:10.4161/mabs.29889.
- Andreev J, Thambi N, Perez Bay AE, Delfino F, Martin J, Kelly MP, Kirshner JR, Rafique A, Kunz A, Nittoli T, et al. Bispecific antibodies and antibody-drug conjugates (ADCs) bridging HER2 and prolactin receptor improve efficacy of HER2 ADCs. Mol Cancer Ther. 2017;16(4):681–93. PMID:28108597. doi:10.1158/1535-7163.MCT-16-0658.
- de GBECG, Vink T, ten NH, Breij ECW, Satijn D, Wubbolts R, Miao D, Pwhi P. Efficient payload delivery by a bispecific antibody-drug conjugate targeting HER2 and CD63. Mol Cancer Ther. 2016;15(11):2688–97. PMID:27559142. doi:10.1158/1535-7163.MCT-16-0364.
- Takemoto H, Nishimura S, Kosada Y, Hata S, Takagi S, Hosoi S, Ezumi K, Ide M, Harada S. Anti-human IgE monoclonal antibodies recognizing epitopes related to the binding sites of high and low affinity IgE receptors. Microbiol Immunol. 1994;38(1):63–71. PMID:7519718. doi:10.1111/j.1348-0421.1994.tb01745.x.
- Garman SC, Wurzburg BA, Tarchevskaya SS, Kinet JP, Jardetzky TS. Structure of the Fc fragment of human IgE bound to its high-affinity receptor Fc epsilonRI alpha. Nature. 2000;406(6793):259–66. PMID:10917520. doi:10.1038/35018500.
- Wurzburg BA, Jardetzky TS. Structural insights into the interactions between human IgE and its high affinity receptor FcepsilonRI. Mol Immunol. 2002;38(14):1063–72. PMID:11955598. doi:10.1016/s0161-5890(02)00035-4.
- van Loghem E, Aalberse RC, Matsumoto H. A genetic marker of human IgE heavy chains, Em(1). Vox Sang. 1984;46(4):195–206. PMID:6201004. doi:10.1111/j.1423-0410.1984.tb00075.x.