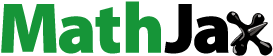
ABSTRACT
The blood-brain barrier (BBB) largely excludes antibodies from entering the central nervous system, thus limiting the potential of therapeutic antibodies to treat conditions such as neurodegenerative diseases and neuro-psychiatric disorders. Here, we demonstrate that the transport of human antibodies across the BBB in mice can be enhanced by modulating their interactions with the neonatal Fc receptor (FcRn). When M252Y/S254T/T246E substitutions are introduced on the antibody Fc domain, immunohistochemical assays reveal widespread distribution of the engineered antibodies throughout the mouse brain. These engineered antibodies remain specific for their antigens and retain pharmacological activity. We propose that novel brain-targeted therapeutic antibodies can be engineered to differentially engage FcRn for receptor-mediated transcytosis across the BBB in order to improve neurological disease therapeutics in the future.
Introduction
The exchange of many macromolecules, including immunoglobulins, between the circulatory system and the central nervous system (CNS) is restricted by the blood-brain barrier (BBB). The BBB is a biological interface formed, in part, by the tight and adherens junctions found between microvascular endothelial cells that line cerebral capillaries.Citation1–3 Since these intercellular contacts prevent the free diffusion of most molecules across the BBB, the transport of macromolecules from the blood into the brain is tightly regulated by intracellular transport pathways found in the endothelial cells.Citation4 Receptor-mediated transport (RMT) is one mechanism by which macromolecules can be transported across the BBB through a process termed transcytosis. In this process, ligands to be transported across the BBB specifically interact with receptors found on the luminal endothelial cell surface. Upon binding, the receptor-ligand complex is internalized by endocytosis and transported along the cytoskeleton in vesicles, which then fuses with the basolateral cell membrane. Through a SNARE-mediated exocytotic process, the ligand is ultimately released into the extracellular space of the brain parenchyma.Citation5,Citation6
Monoclonal antibody-based therapeutics have limited potential for treating neurological conditions because these molecules do not naturally undergo significant RMT and are generally unable to cross the BBB when dosed peripherally. A number of groups have proposed strategies to engineer these drugs so that they can take advantage of endogenous RMT pathways.Citation7,Citation8 In these strategies, the therapeutic molecule would comprise a moiety that binds to RMT receptors expressed on brain endothelial capillary cells. As these receptors shuttle their endogenous ligands across the BBB by transcytosis, any bound antibody drugs would also be shuttled across the BBB. Proposed drugs that follow such design principles include moieties that target proteins such as the transferrin receptor (TfR),Citation9–16 the insulin receptor (IR),Citation17,Citation18 low density lipoprotein receptor-related protein 1 (LRP1),Citation19,Citation20 glucose transporter 1 (GLUT1),Citation21 basigin (BSG),Citation21,Citation22 and CD98hc,Citation21 among others.
The neonatal crystallizable fragment receptor (FcRn) is a major histocompatibility complex Class I-related receptor that interacts with the fragment crystallizable (Fc) domain of immunoglobulin G (IgG) class proteins. It is known to facilitate the bidirectional transport of antibody proteins across many biological barriers, including the epithelia found at the placenta,Citation23,Citation24 the lungs,Citation25 the kidneys,Citation26,Citation27 and the intestines,Citation28–30 but not across the BBB. Instead, at the BBB, FcRn is thought to be involved only in the efflux of antibodies from the brain parenchyma into the luminal compartment of brain capillaries. To date, studies characterizing this unidirectional FcRn-mediated transport of antibody proteins at the BBB have primarily focused on the transport of IgG proteins with wild type (WT) Fc domains.Citation31–35 However, FcRn-mediated transcytosis of IgG proteins with modified FcRn interactions have largely remained unexplored.
In this study, we test the hypothesis that, under the appropriate conditions, FcRn can also mediate the influx of antibody proteins from brain capillaries into the brain parenchyma. We show that FcRn can be used to facilitate antibody RMT by the appropriate engineering of antibody Fc domains. We demonstrate that M252Y, S254T, and T246E substitutions on the Fc domain of immunoglobulin proteinsCitation36 is one example that successfully uses Fc engineering to improve the bulk transport of antibodies across mouse cerebral capillaries both in vitro and in vivo. Interaction between the antibody Fc domain and FcRn is both necessary and sufficient for the observed transcytosis. When these substitutions are made on the Fc domain of therapeutic antibodies designed to modulate brain physiology, we observe functional changes in brain signaling that demonstrate improved antibody-target engagement in the mouse brain parenchyma.
Results
YTE substitutions increase BBB transport
To study the transport of antibody proteins across the BBB in living systems, we developed an immunohistochemical (IHC) assay to detect human immunoglobulin (hIgG) proteins in the mouse brain. In this assay, we used the O4 antibodyCitation37 that was raised against myelin-rich white matter from bovine corpus callosum and that labels mouse and rat oligodendrocytes (Figure S1a). The antibody was reformatted by fusing the variable regions of the heavy and light chains from the original hybridoma onto a human immunoglobulin G1 (hIgG1) heavy chain and a human immunoglobulin kappa (hIgGκ) light chain, respectively. The resulting recombinant antibody retains affinity for oligodendrocytes in rat cortical cultures (Figure S1b, c) and labels myelin-rich regions in the mouse brain (Figure S1d-f).
We then used the IHC assay to assess how this O4-WT hIgG1 antibody with an unmodified (WT) human Fc region is distributed in the mouse brain after intravenous administration. Typically, the IHC assay has almost no background and detects little to no immunoreactivity in the brain of WT CD-1 mice, such as when mice are dosed with a phosphate-buffered saline (PBS) vehicle control (). When the O4-WT hIgG1 antibody is administered at 30 mg/kg, however, human immunoglobulin proteins are detectable at low levels by the assay (). These antibodies are generally localized to periventricular regions, where the BBB is leakier,Citation38,Citation39 and do not fully access areas in the brain parenchyma.
Figure 1. YTE variant increases distribution of O4 antibodies into mouse brain. Representative images of brains dissected from WT CD-1 mice 48 hours after administration with (a) PBS, (b) O4-WT hIgG1, (c) O4-YTE hIgG1, or (d) O4-AA hIgG1 test articles and immunostained for human IgGκ chain. Immunoreactivity appears as brown diaminobenzidine (DAB) deposits. Scale bar shows 1 mm. (e) Quantification of immunostaining in brains from groups represented in panels b-d. Four matching sections along the rostral-caudal axis were selected and quantified for each of two animals per group. Error bars represent SEM. Statistical analysis was calculated using an ordinary one-way ANOVA with Dunnett’s multiple comparisons test. **, p < 0.01.
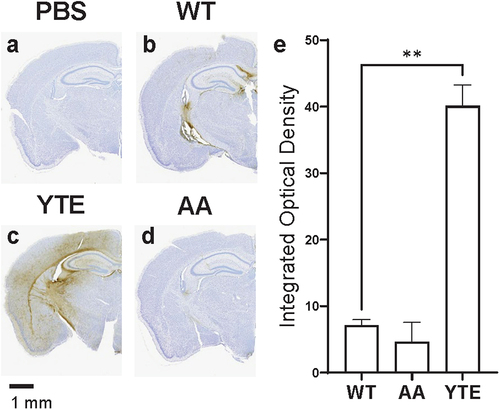
To test whether interactions between antibodies and FcRn can contribute to transcytosis across the BBB, we introduced M252Y/S254T/T256E (YTE) mutations on the Fc region of the antibody protein. These substitutions are known to increase antibody affinity for mouse FcRn protein at both pH 6.0 and pH 7.4.Citation36 To our surprise, intense staining for hIgG was observed throughout the parenchyma in the brains of mice dosed with O4-YTE hIgG1 antibody test articles (). The staining was primarily localized to myelin-rich regions of the brain, such as the internal capsule, consistent with the antigen of the O4 antibody variable region. In contrast, brain sections from mice dosed with O4 antibodies incorporating mutations known to disrupt antibody-FcRn interactions, such as the I253A/H435A (AA) substitutions,Citation40 showed almost no staining for hIgG ().
To examine the kinetics at which the O4-YTE hIgG1 antibody partitions into the brain, we administered the test article to additional mice and collected the brains at varying intervals after dosing. The amount of antibody found in the brain parenchymal compartment reached a maximum approximately 48 hours after dosing and was still detectable up to 504 hours later (). This contrasted with its distribution in cerebral spinal fluid (CSF) tissue, where it was readily detectable immediately after dosing and was slowly eliminated over the course of the experiment (). The concentration time profile of the antibody in serum was characterized by an initial rapid distribution phase followed by a terminal elimination phase with a half-life of 129 hours (), similar to previously reported observations.Citation36
Figure 2. O4-YTE hIgG1 antibodies persist in the brain for up to 504 hours after dosing. (a) Representative images of brains dissected from WT CD-1 mice at 2, 4, 6, 12, 24, 48, 96, 168, 336, and 504 hours (h) after administration of O4-YTE hIgG1 test articles and immunostained for human IgGκ chain. (b) Quantification of human IgGκ immunostaining in brains from groups represented in panel a. Four matching sections along the rostral-caudal axis were selected and quantified for each of two animals per group. (c) Quantification of hIgG protein in the CSF and (d) serum of animals from groups represented in panel a. Antibody concentration was estimated with a sandwich ELISA assay using antibodies specific for hIgG protein. Error bars represent SEM.
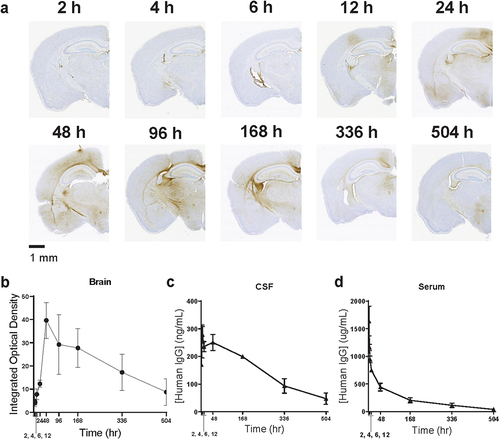
Antibody-FcRn interactions facilitate BBB transcytosis
We next asked whether antibody-FcRn interactions are necessary for the observed distribution of O4-YTE hIgG1 antibody in the mouse brain. To test this question, we repeated the experiment in FCRN-deficient mice carrying a knock-out mutation for Fcgrt (Fc Fragment of IgG Receptor and Transporter) (Fcgrt-/-).Citation41 Because these knockout mice were engineered in a C57BL/6J background, we also tested the O4-WT hIgG1 and O4-YTE hIgG1 test articles in WT C57BL/6J animals as a control to confirm that the BBB transport function of the YTE mutation is not strain-specific.
Indeed, we found that O4-YTE hIgG1 test articles more readily cross the BBB than O4-WT hIgG1 test articles and are widely distributed in Fcgrt+/+ C57BL/6J mouse brains 48 h after administration (). In contrast, virtually no immunoreactivity for human antibodies are found in the brains of Fcgrt−/− C57BL/6J mice regardless of the test article administered (). Thus, the interactions between the YTE mutations on the antibody Fc domain and the FcRn receptor are necessary and sufficient for improved BBB transport in the mouse.
Figure 3. Antibody-FcRn interaction is necessary for YTE-mediated improvement in brain delivery. Representative images of brains dissected from either WT C57BL/6J (FCGRT+/+) mice dosed with (a) O4-WT hIgG1 or (b) O4-YTE hIgG1 test articles or FcRn knockout (FCGRT−/−) mice dosed with (c) O4-WT hIgG1 or (d) O4-YTE hIgG1 test articles. Brains were dissected 48 h after antibody administration and immunostained for human IgGκ chain with the DAB chromogen. Scale bar shows 1 mm. (e) Quantification of immunostaining in brains from groups represented in panels a-d. Four matching sections along the rostral-caudal axis were selected and quantified for each of two animals per group. Error bars represent SEM. Statistical analysis was calculated with a 2-way ANOVA followed by Sidak’s multiple comparisons test. ***, p < 0.001.

FcRn-mediated BBB transport occurs through a cellular internalization pathway
Protein transport across the BBB can occur via several different pathways broadly divided into paracellular and transcellular mechanisms.Citation42 Since RMT is a transcellular process, we reasoned that it can only occur if an antibody protein is internalized by brain endothelial cells. We therefore established an antibody internalization assay based on previous workCitation43 to investigate whether O4-YTE hIgG1 transport is accompanied by cellular internalization of the antibody. Since other groups have noted that FcRn-mediated transport is best studied in systems that overexpress both the FcRn heavy chain and β2-microglobulin (β2 M),Citation44–46 we generated a stable HEK293 cell line expressing mouse FcRn and mouse β2 M. In this assay, antibodies were first incubated with cells for up to 4.25 h, after which unbound antibodies were washed off and the cells fixed. Extracellular antibodies were then blocked with unlabeled anti-human Fab, and the cells were washed and fixed again. Finally, cells were permeabilized and stained with anti-human IgG fluorescent conjugates to reveal only intracellularly stained antibodies (see methods for more details).
We tested the internalization of the O4-WT hIgG1, O4-AA hIgG1, and O4-YTE hIgG1 antibodies in these cell lines. After four hours of incubation of the antibody test articles in the assay, O4-YTE hIgG1 antibodies were internalized by the HEK293 mFcRn/mβ2 M cells to a much greater extent than O4-WT hIgG1 antibodies. In contrast, virtually no O4-AA hIgG1 antibodies were found to be internalized by HEK293 mFcRn/mβ2 M cells (). This correlation between the internalization data and the immunohistochemistry data suggests that antibodies are transported from the blood into the brain through a transcellular pathway in endothelial cells.
Figure 4. YTE mutations increase antibody internalization in mFcRn-expressing cells. (a) O4-WT hIgG1, O4-AA hIgG1, or O4-YTE hIgG1 test articles were added to wells containing HEK293 cells stably expressing mFcRn/m2 M and incubated at 37°C for 4 h. Cells were washed, blocked, fixed, and permeabilized, and internalized antibodies were identified by immunostaining for hIgG protein. Top panels, Representative images of internalized antibody after 4 h of incubation at 37°C. Lower panels, Nuclei staining with DAPI demonstrating the confluence of cells in the image. White bar = 50 μm. (b) Quantification of antibody staining in panel a. Summed fluorescence intensity values (thresholded density × area)/number of nuclei were calculated for each image, 4 images per well, 4 wells/condition and graphed. Error bars represent SEM. Statistical analysis was calculated using an ordinary one-way ANOVA with Dunnett’s multiple comparisons test. ****, p < 0.0001. (c) The internalization assay was repeated, but the length of time that antibodies were incubated with the cells was varied to 0.75, 1.25, 3.25, or 4.25 h. By four hours of incubation, the quantity of internalized antibody neared a maximum for cells treated with the O4-YTE hIgG1 antibody.

In addition, we tested the degree to which O4-WT hIgG1, O4-AA hIgG1, and O4-YTE hIgG1 test molecules were internalized by HEK293 mFcRn/mβ2 M cells over the course of 4.25 h. During the experiment, the accumulation of O4-YTE hIgG1 test articles in HEK293 mFcRn/mβ2 M cells was three-fold higher compared to that of O4-WT hIgG1 test articles, and we observed no detectable internalization of O4-AA hIgG1 antibodies (), consistent with our previous observations. The kinetics of the O4-YTE hIgG1 internalization process appeared faster at the beginning of the experimental timeline and then slowly approached a maximum level, suggesting that O4-YTE hIgG1 internalization is likely facilitated by a saturable receptor-mediated process.
Antibodies with other variable regions can be functionalized with YTE substitutions
An Fc modification can be usefully exploited to deliver antibody proteins across the BBB only if it can be applied reliably to other molecules bearing different variable regions. To test whether the YTE substitutions can be generalized to deliver other IgG molecules across the BBB, we expressed antibody proteins bearing the variable region of an isotype control antibody (KLH), which does not target any specific mouse antigen, fused to either a WT human IgG1 heavy chain constant region or a human IgG1 heavy chain constant region with the YTE substitutions.
Similar to our observations with the O4-WT hIgG1 and O4-YTE hIgG1 test articles (), immunoreactivity to hIgG protein in CD-1 mouse brains that were treated with KLH-WT hIgG1 antibodies was faint and mainly localized to periventricular regions (). In contrast, hIgG immunoreactivity was greatly increased in mice that were treated with KLH-YTE hIgG1 antibodies (). The staining of KLH-YTE hIgG1 appeared more diffuse compared to that of O4-YTE hIgG1 (), possibly because the variable region of the KLH-YTE hIgG1 antibody does not target any specific antigen in the mouse brain. Altogether, these results suggest that Fc modifications that alter FcRn interaction can be used to shuttle any therapeutic antibody across the BBB.
Figure 5. YTE mutations can increase the brain distribution of other antibodies with different variable regions. The variable regions of the O4-WT hIgG1 and O4-YTE hIgG1 antibodies were replaced with the variable region of an isotype control (KLH) antibody. Representative images show sections from brains dissected from WT CD-1 mice 48 h after administration with either the (a) KLH-WT hIgG1 or (b) KLH-YTE hIgG1 test articles and immunostained for human IgGκ chain. Immunoreactivity appears as brown diaminobenzidine (DAB) deposits. Scale bar shows 1 mm. (c) Quantification of immunostaining in brains from groups represented in panels a and b. Four matching sections along the rostral-caudal axis were selected and quantified for each of two animals per group. Error bars represent SEM. Statistical analysis was calculated with an unpaired t-test. *, p < 0.05.

YTE substitutions increase signaling function of an anti-TrkB agonist antibody in the brain
We next investigated whether the YTE substitutions result in improved delivery of therapeutically active antibodies to the brain. To address this question, we used the variable region of an agonist antibody raised against a tyrosine receptor kinase B (TrkB) antigen that was previously described.Citation47 Since this antigen protein is a brain-derived neurotrophic factor (BDNF) receptor that signals through the mitogen-activated protein kinase (MAPK)/extracellular signal-regulated kinase (ERK) pathway, we could easily monitor the pharmacological activity of test antibodies in the brain by measuring the extent to which ERK protein is phosphorylated. We focused our quantification on the hippocampal region, where ERK1/2 signaling is reportedly most robust.Citation48,Citation49
We dosed CD-1 mice at 10 mg/kg with either anti-TrkB-WT hIgG1 or anti-TrkB-YTE hIgG1 antibody, and collected brains for histology 48 h after dosing. We then stained for phosphorylated ERK1/2 (pERK) protein, indicative of downstream TrkB signaling. The resulting pERK staining clearly marked neuronal cell bodies in the dentate gyrus of dosed animals (). We observed a few pERK-positive cells in animals that received the anti-TrkB-WT hIgG1 test article (), likely resulting from basal neurotrophin signaling in the hippocampus and low levels of anti-TrkB-WT hIgG1 antibodies that enter the brain parenchyma around the lateral ventricles (). In contrast, we detected significantly more pERK-positive cells in the brains of mice that were administered anti-TrkB-YTE hIgG1 antibodies (), indicating that the YTE Fc domains were able to shuttle pharmacologically active antibodies across the BBB.
Figure 6. YTE variant increases function of anti-TrkB agonist antibody in the brain. Representative images of hippocampus region in WT CD-1 mice 48 hours after administration of 10 mg/kg (a) anti-TrkB-WT hIgG1 or (b) anti-TrkB-YTE hIgG1 test articles and immunostained for pERK. Immunoreactivity appears as black nickel deposits. Scale bar shows 1 mm. Inset shows 3X magnification. (c) Quantification of immunostaining in brains from groups represented in panels a and b. Three sections from each hippocampus region were selected and quantified for each of two animals per group. Error bars represent SEM. Statistical analysis was calculated with an unpaired t-test. **, p < 0.01.
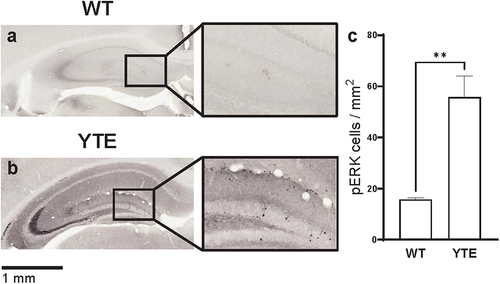
Discussion
In this study, we demonstrated that human IgG1 proteins with M252Y/S254T/T246E substitutions in their Fc domains can efficiently cross the BBB of both CD-1 and C57BL/6 WT mice. Antibodies with this modification can be widely distributed in the brain and persist for at least 504 h after administration. The transport of these antibody proteins occurs through an FcRn-mediated mechanism that follows a transcellular route. These substitutions can be introduced in antibody proteins with a variety of variable regions without affecting their target specificity and function after entering the brain parenchyma. Given the conservation of both IgG and FcRn proteins between rodents and primates, we believe that testing mutations similar to M252Y/S254T/T246E in preclinical models will further the development of brain-targeted drugs for the treatment of neurological conditions (data not shown). We propose that modulating antibody-FcRn interactions may contribute to the discovery of novel BBB-crossing antibody drugs in the future.
Our current findings are consistent with previous reports that FcRn is not involved in WT antibody transcytosis at the BBB. For example, Ruano-Salguero and LeeCitation33 did not observe any difference between the trafficking of molecules with or without WT Fc domains across brain endothelial-like cells derived from induced pluripotent stem cells. Garg and BalthasarCitation31 also did not observe any difference in the distribution of 125I-labeled WT monoclonal IgG molecules into FcRn-knockout or WT mouse brains. Similarly, we detected only negligible transport of antibody proteins into the mouse brain in the absence of the YTE substitutions. Based on our observations, increased FcRn-mediated BBB transport would be expected only when antibody-FcRn interactions are appropriately modified.
Additionally, other authors such as Zhang and PardridgeCitation34 and Copper et al.Citation35 have observed the rapid efflux of IgG protein with a functional Fc domain after intracerebral injection. Based on these observations, they posited that FcRn facilitates only the unidirectional export of antibody proteins from the brain. In light of our current study, however, we propose that FcRn can facilitate the bidirectional transport of antibody proteins across the BBB under the appropriate conditions. Whereas these previous studies have focused on the transport of antibodies with WT Fc domains, our data indicate that certain amino acid substitutions on the Fc domain can result in antibody influx into the brain and antibody efflux out of the brain.
Our study used HEK 293 cells transduced to express mouse FcRn in order to characterize the movement of antibody proteins during RMT. Use of a cell line that overexpresses the FcRn receptor increased the dynamic range of the system and improved the signal-to-noise ratio of our antibody internalization assay. We note, however, that endogenous levels of FcRn receptor expression naturally expressed in mouse endothelial cells are sufficient to transport therapeutically relevant amounts of antibody protein to the brain, as seen in . Since comparable levels of FcRn expression are observed in both human and mouse endothelial cells,Citation50 we expect that FcRn-mediated BBB transport will be a relevant clinical strategy for therapeutic monoclonal antibodies as well.
The M252Y/S254T/T246E substitutions used in this study have been previously shown to differentially alter antibody-FcRn interaction in different species. In humans, these substitutions selectively increase antibody-human FcRn interactions at pH 6.0 but not at pH 7.4, thereby enhancing FcRn-mediated antibody recycling from endosomes and increasing antibody half-life. In mice, on the other hand, these same substitutions non-selectively increase antibody-mouse FcRn interactions at both pH 6.0 and pH 7.4, reducing antibody half-life.Citation36 This species-dependent antibody-receptor interaction thus suggests that the M252Y/S254T/T246E substitutions would not improve BBB transport in humans like it does in mice. Similarly, other mutations that enhance antibody-FcRn interactions only at acidic pH to extend serum antibody half-life, such as T250Q/M428LCitation51 and M428L/N434SCitation52 in humans and T252L/T254S/T256FCitation53 in mice would also likely not improve antibody BBB transport.
On the other hand, several Fc mutations, such as the M252Y/S254T/T246E/H433K/N434F substitutions found on efgartigimod (VYVGARTTM),Citation54 have been shown to enhance antibody-FcRn interactions in both low and high pH environments. Although testing whether antibodies with these substitutions would have enhanced BBB-transcytosis properties in humans is beyond the scope of this study, we propose that site-directed mutagenesis along the Fc-FcRn interface to search for mutations that enhance antibody-FcRn interactions in a pH-independent manner may identify substitutions that improve antibody BBB transport in humans. However, because antibodies with enhanced FcRn interaction at neutral pH tend to have short half-lives and can compete with endogenous antibodies for FcRn binding sites, it will be important to titrate the strength of antibody-FcRn interactions to limit unwanted on-target side effects.
Materials and methods
Cloning and purification of antibodies
The coding sequences for the variable regions of the O4 antibody heavy and light chains were sequenced from its hybridoma.Citation37 Those of the KLH antibody were sequenced from a hybridoma derived from a rat immunized with an antigen conjugated to keyhole limpet hemocyanin. Those of the anti-TrkB antibody were previously published.Citation55 Double-stranded gene fragments (gBlocks, Integrated DNA Technology) of the heavy chains were synthesized by fusing the VH region of each antibody to the constant region of either the WT human immunoglobulin G1 heavy chain,Citation56,Citation57 the human immunoglobulin G1 heavy chain with substitutions at M252Y/S254T/T256E,Citation58 or the human immunoglobulin G1 heavy chain with substitutions at I253A/H435A.Citation40 Gene fragments of the light chains were synthesized by fusing the VL region of each antibody to the constant region of the human immunoglobulin kappa light chain.Citation59 The heavy chain and light chain gene fragments were separately cloned into an in-house pUNI expression vector under the control of a CMV promotor by restriction digestion followed by DNA ligation. The resulting products were transformed into One ShotMach 1 cells (ThermoFisher, #C8620–03) and selected on Luria Bertani broth (LB) agar plates supplemented with 100 μg/mL Carbenicillin (Teknova, #L1010). Transformants were sequenced by colony PCR with in-house forward and reverse sequencing primers (Sequetech). Plasmids were purified using Qiagen miniprep kits (#27104).
Antibodies were expressed by transient transfection in the Expi293 expression system (ThermoFisher, #A14635). The culture supernatants containing expressed antibody were filtered through 0.2 μm filters, and antibody yield was measured using Protein A sensors on an Octet system (ForteBio).
Antibodies were purified by affinity chromatography using a Protein A Sepharose resin (Cytiva, #17127907) into 100 mM arginine, 10 mM histidine, 150 mM NaCl, 20 mM Na2HPO4, pH 6 buffer, and the final product was concentrated through 30 kDa centrifugal Amicon filters (Millipore, #UFC903096) to greater than 5 mg/mL.
In vivo administration in mice
Six- to eight-week-old male CD-1 (Charles River Laboratories), C57BL/6 (The Jackson Laboratory), and B6.129X1-Fcgrttm1Dcr/DcrJCitation41 mice were used in this study. Animals were allowed to acclimate to the test facility for at least 2 d prior to dosing with antibody. Animal body weights were recorded prior to dose administration. Test antibodies were administered intravenously at 10 or 30 mg/kg. All animals were observed at dosing and at scheduled collection times, and all abnormalities were recorded. All studies were conducted under the guidance and approval of the Charles River Worcester IACUC.
In plasma pharmacokinetic (PK) studies, serial blood samples were collected by tail vein snip in accordance with test facility standard operating procedures. Terminal blood samples were collected by cardiac venipuncture following inhalation anesthesia. Blood samples were stored at ambient conditions for at least 30 min to allow for clotting and processed to serum by centrifugation (3500 rpm at 5°C for 10 min) within 1 h of collection. Blood serum samples were stored at nominal −80°C until further processing.
In CSF PK studies, immediately following the collection of the blood sample, CSF samples were also collected. Individual CSF weights were calculated, the tubes were placed immediately on dry ice and then stored at −80°C until further analysis. The designs of the experiments shown in each figure is summarized in .
Table 1. Designs of animal studies.
Immunohistochemistry
Following inhalation anesthesia, the animals were transcardially perfused with 60 mL of saline and again with 60 mL of 4% paraformaldehyde (PFA) solution. Brains were dissected out from each animal following perfusion. Whole brains were then post-fixed in a 4% PFA solution for 24 h, after which they were transferred into PBS for storage.
Brains were cryoprotected overnight in 20% glycerol and 2% dimethylsulfoxide and then freeze-sectioned at 35 μm thickness in the coronal plane. Staining was performed through the entire mouse brain on every 12th section (spaced at approximately 420 μm intervals). For human immunoglobulin kappa chain staining, brain sections were treated with hydrogen peroxide to inactivate endogenous peroxidases (Fisher Scientific, #H325–500), after which sections were labeled with a biotinylated goat anti-human IgG kappa light chain primary antibody (Southern Biotech, #2061–08) overnight at room temperature. All incubation solutions from the primary antibody onward used tris-buffered saline (TBS; 50 mM Tris-Cl, 150 mM NaCl, pH 7.4) and Triton X-100 (Promega, #H5141). Following rinses, brain sections were complexed to an avidin-biotin-horseradish peroxidase (HRP) complex using the VECTASTAIN® Elite ABC-HRP kit (Vector Laboratories, #PK-6100). The sections were rinsed and then stained with the chromagen, 3,3’-diaminobenzidine tetrahydrochloride (DAB) (Electron Microscopy Sciences, #13080) and hydrogen peroxide to create a visible reaction product. Following further rinses, the sections were mounted on gelatin-coated glass slides, air-dried and counterstained using a thionine-Nissl stain. For the counterstaining, sections were carried through the following sequence: 95% ethanol, 95% ethanol/formaldehyde; 95% ethanol, chloroform/ether/absolute ethanol (8:1:1), 95% ethanol; 10% HCl/ethanol, 95% ethanol, 70% ethanol, deionized water, thionine (0.05% thionine/acetate buffer, pH 4.5), deionized water, 70% ethanol, 95% ethanol, acetic acid/ethanol, 95% ethanol, 100% ethanol, 100% ethanol, 1:1 100% ethanol/xylene, xylene, xylene. Sections were coverslipped prior to imaging.
For pERK staining, brain slices were labeled overnight at room temperature with rabbit anti-phosphorylated extracellular signal-regulated kinase 1/2 antibody (anti-pERK 1/2) (Neuromics, #RA15002) diluted 1:5000 to reveal pERK1/2, a biomarker downstream of the TrkB pathway. Following rinses, a biotinylated goat anti-rabbit IgG (Vector Laboratories, #BA-1000-1.5) secondary antibody was applied at a dilution of 1:1000. After further rinses, sections were treated with the VECTASTAIN® Elite ABC-HRP Kit. Sections were rinsed with TBS and then treated with DAB, hydrogen peroxide, and nickel (Sigma, # N4882). After another TBS rinse, sections were mounted on gelatin-coated glass slides and air dried. Slides were not counterstained and only dehydrated in alcohols and cleared in xylene. Sections were coverslipped prior to imaging.
Stained slides were imaged at 20X on a TissueScopeTM LE120 slide scanner (Huron Digital Pathology). Analysis of the resulting TIFF images was performed on HALO (v3.2, Indica Labs). Three to four serial rostral to caudal coronal brain sections were selected from each animal. To ensure approximately the same brain regions were compared between different animals, images were also matched based on gross anatomical landmarks. For sections stained for hIgG kappa chain, an annotation contour was drawn along the margins of each coronal brain section in order to include all brain regions in the IHC staining analysis of the section. This approach was selected as it allowed assessing multiple brain regions and was established empirically that whole-section analysis resulted in lower inter- and intra-animal coefficient of variance, as opposed to restricting the analysis to individual brain regions (e.g., cortex, striatum). For pERK1/2 analysis, the annotation contour was drawn along the margins of the hippocampal formation. In order to quantify cell-specific IHC signal only, areas in the Cornu Ammonis 3 (CA3) region where intense fiber staining was present were manually excluded from the analysis by using the HALO™ Exclusion Pen tool.
The HALO tissue classifier module was then applied to the images, which allowed separation of the tissue in the image from non-tissue classes (i.e., glass) based on an artificial intelligence random forest algorithmCitation60 trained during a learning session on several representative images. The resulting Tissue-Glass Classifier parameters were then applied to all subsequent images analyzed.
For the sections stained for human IgG kappa, the Area Quantification module of HALO was used to quantify the intensity of the DAB chromophore within the tissue portion of the selected brain section images. Similar to the Tissue-Glass Classifier, parameters for the Area Quantification module were established over a training session on a few representative images in order to teach the algorithm to identify and separate the DAB stain from other tissue. During the training session, parameters were established categorizing the IHC staining signal as ‘weak, ‘moderate’, or ‘strong’ based on pixel density. The resulting customized Area Quantification analysis module was then applied to all subsequent images analyzed. Following the HALO analysis completion, the computed ‘DAB Average Positive Optical Density’ was multiplied by the value for the ‘% DAB Positive Tissue’ for each section image in order to calculate the ‘Integrated Optical Density (OD)’ for that section image. The average value for the ‘Integrated OD’ of all section images for each analyzed brain was used to compare HALO image analysis results between treatment groups dosed with different antibody variants.
For the sections stained for pERK, in order to quantify the number of pERK1/2-expressing cells in the hippocampus, the HALO™ Object Colocalization module was applied following Tissue-Glass classification. Parameters for the Object Colocalization module were established over a training session on a few representative images in order to teach the algorithm to identify stained cells and ‘count’ them as objects based on factors, such as size, morphology and IHC staining intensity, excluding diffused non-cellular staining in the process. The resulting customized Object Colocalization analysis modules were then applied to all subsequent images analyzed. Following HALO™ analysis completion, the average number of pERK1/2 objects (cells) identified per mm2 area of hippocampus was plotted for each analyzed brain. Quantified data was graphed in Prism 9 software (GraphPad Software, Inc.).
Enzyme-linked immunosorbent assay (ELISA) for PK analysis
Antibody concentrations in murine serum and CSF were measured with a human IgG ELISA kit (Abcam, #195215) following manufacturer’s standard protocol. In brief, the test antibody was used as a standard to quantify antibody concentration in serum and CSF samples. Standards and test samples were diluted to a concentration below 15 ng/mL and loaded at 50 μL/well into 96-well plates coated with the primary antibody provided by the kit. Kit secondary antibodies were then added to each well, and plates were sealed and incubated at room temperature for 40 min on a plate shaker set at 400 revolutions per minute (rpm). Plates were washed and 3,3‘,5,5’-tetramethylbenzidine was added as a chromogen at 100 μL/well. Sealed plates were incubated at room temperature for 5 min on a plate shaker set at 400 rpm to develop a color reaction, after which the color reaction was stopped. The intensity of the color reaction was assessed by absorbance at a wavelength of 450 nm. Sample protein concentrations were determined by interpolating the blank control absorbance values subtracted against the respective antibody standard curve with a second order polynomial model. PK parameters were calculated using standard noncompartmental methods with Phoenix® WinNonlin® software (Pharsight Corporation, a Certara Company).
Antibody internalization assay
HEK293 cells (ATCC) were stably transduced with lentiviral particles harboring a mouse Fcgrt (Fc Fragment of IgG Receptor and Transporter, FcRn) gene expression construct with puromycin resistance (Genecopoeia, #Mm34224) and a mouse B2M (beta 2 microglobulin, β2 M) gene expression construct with hygromycin resistance (Genecopoeia, #Mm01377).
The following methods are adapted from Corrodus NL et al.Citation43 HEK293 mFcRn/mβ2 M cells were plated at 100,000 cells/well in a 96 well plate and grown for 1–2 d in Dulbecco’s Modified Essential Media (DMEM), 10% fetal bovine serum, 1% penicillin/streptomycin, 10 µg/ml puromycin, and 100 ug/ml hygromycin. The cells were washed with Hanks Buffered Saline Solution (HBSS, Hyclone #SH30588.02) using a plate washer leaving 50 µl HBSS.
Purified antibody was diluted in HBSS as a 2X stock, and 50 µl were added to each well to a final concentration of 10 µg/ml. Cells were incubated for up to 4.25 h (as indicated in figure legend) at 37°C and then washed with HBSS, leaving a final volume of 50 µl. Washed and labeled cells were fixed by incubating in 4% PFA (Electron Microscopy Sciences #15710) for 5 min at room temperature. Fixed cells were washed again PBS, leaving a final volume of 50 µl. Non-specifically bound external antibody was blocked with excess unlabeled secondary anti-human Fab (goat anti-human IgG Fab, Jackson ImmunoResearch Inc., #109-007-003) diluted in Antibody Blocking Buffer (150 mM NaCl, 50 mM Tris, 1% bovine serum albumin, 100 mM L-lysine, 0.04% sodium azide, pH 7.4) overnight at room temperature. Cells were then washed with PBS, post fixed in 4% PFA for 5 min at room temperature, and washed again with PBS leaving a final volume of cells in PBS of 50 µl. Fixed cells were permeabilized by incubating for 30 min in 50 µl 0.2% Triton X-100 diluted in Antibody Blocking Buffer and then washed with PBS, leaving a final volume of cells in PBS of 50 µl. Fifty µl of 2X DAPI (2 µg/ml, ThermoFisher #62248) and anti-human-AlexaFluor-647 (Invitrogen, #A-21445) diluted 1:500 in Antibody Blocking Buffer/0.2% TX-100 was added and incubated for 2 h, labeling nuclei and internalized antibodies only.
Stained cells were imaged at 20X using an InCell Analyzer (GE). Using the InCell Developer toolbox software, internalized antibody was quantified by creating a threshold above background staining as determined by secondary antibody alone to create a mask. The product of the (density × area) under the mask was then divided by the number of nuclei, which gave the staining intensity of internalized antibody/cell.
Author contributions
JT, JS, and DW were responsible for the conception of research; JT, DL, RP, and VT designed the research, analyzed data, and interpreted experimental results with significant help from DS, LJ and HH; TT designed and prepared all the test materials with significant help from AS, YY, ED, and AJ; JT drafted the manuscript with assistance from DL and RP; all authors contributed to editing and revising the manuscript.
Abbreviations
AA | = | I253A/H435A |
β2 M | = | β2-microglobulin |
BBB | = | blood-brain barrier |
BDNF | = | brain-derived neurotrophic factor |
BSG | = | basigin |
CA3 | = | Cornu Ammonis 3 |
CNS | = | central nervous system |
CSF | = | cerebral spinal fluid |
DAB | = | 3,3’-diaminobenzidine tetrahydrochloride |
DMEM | = | Dulbecco’s modified essential media |
ELISA | = | enzyme-linked immunosorbent assay |
ERK | = | extracellular signal-regulated kinase |
Fc | = | fragment crystallizable |
Fcgrt | = | Fc Fragment of IgG Receptor and Transporter |
FcRn | = | neonatal Fc receptor |
GLUT1 | = | glucose transporter 1 |
HBSS | = | Hanks buffered saline solution |
hIgG1 | = | human immunoglobulin G1 |
hIgGk | = | human immunoglobulin kappa |
IHC | = | immunohistochemistry |
IR | = | insulin receptor |
KLH | = | keyhole limpet hemocyanin |
LB | = | Luria Bertani |
LRP1 | = | low density lipoprotein receptor-related protein 1 |
MAPK | = | mitogen-activated protein kinase |
OD | = | optical density |
pERK | = | phosphorylated extracellular signal-regulated kinase |
PBS | = | phosphate-buffered saline |
PFA | = | paraformaldehyde |
PK | = | pharmacokinetics |
RMT | = | receptor-mediated transport |
rpm | = | revolutions per minute |
TBS | = | tris-buffered saline |
TfR | = | transferrin receptor |
TMB | = | tetramethylbenzidine |
TrkB | = | tyrosine receptor kinase B |
WT | = | wild type |
YTE | = | M252Y/S254T/T256E |
Supplemental Material
Download TIFF Image (2.2 MB)Supplemental Material
Download MS Word (14 KB)Acknowledgments
The authors thank Pippa Loupe and Simon Tout for their careful review of this manuscript, Robert Switzer and his team at NeuroScience Associates, Inc. for their assistance with IHC assays, and Brian Tower and his team at Charles River Laboratories International, Inc. for their assistance with the in-life procedures performed in this study.
Disclosure statement
All authors were employees of Teva Pharmaceuticals Ltd.
Supplementary material
Supplemental data for this article can be accessed online at https://doi.org/10.1080/19420862.2023.2229098
References
- Abbott NJ. Blood-brain barrier structure and function and the challenges for CNS drug delivery. J Inherit Metab Dis. 2013;36(3):437–12. doi:10.1007/s10545-013-9608-0. PMID: 23609350.
- Abbott NJ, Rönnbäck L, Hansson E. Astrocyte-endothelial interactions at the blood-brain barrier. Nat Rev Neurosci. 2006;7(1):41–53. doi:10.1038/nrn1824. PMID: 16371949.
- Daneman R, Prat A. The blood-brain barrier. Cold Spring Harb Perspect Biol. 2015;7(1):a020412. doi:10.1101/cshperspect.a020412. PMID: 25561720.
- Villaseñor R, Lampe J, Schwaninger M, Collin L. Intracellular transport and regulation of transcytosis across the blood-brain barrier. Cell Mol Life Sci. 2019;76(6):1081–92. doi:10.1007/s00018-018-2982-x. PMID: 30523362.
- Preston JE, Joan Abbott N, Begley DJ. Transcytosis of macromolecules at the blood-brain barrier. Adv Pharmacol. 2014;71:147–63. doi:10.1016/bs.apha.2014.06.001. PMID: 25307216.
- Lajoie JM, Shusta EV. Targeting receptor-mediated transport for delivery of biologics across the blood-brain barrier. Annu Rev Pharmacol Toxicol. 2015;55:613–31. doi:10.1146/annurev-pharmtox-010814-124852. PMID: 25340933.
- Pardridge WM. Re-engineering biopharmaceuticals for delivery to brain with molecular Trojan horses. Bioconjug Chem. 2008;19(7):1327–38. doi:10.1021/bc800148t. PMID: 18547095.
- Boado RJ. IgG fusion proteins for brain delivery of biologics via blood–brain barrier receptor-mediated transport. Pharmaceutics. 2022;14(7):1476. doi:10.3390/pharmaceutics14071476. PMID: 35890374.
- Friden PM, Walus LR, Musso GF, Taylor MA, Malfroy B, Starzyk RM. Anti-transferrin receptor antibody and antibody-drug conjugates cross the blood-brain barrier. Proc Natl Acad Sci USA. 1991;88(11):4771–75. doi:10.1073/pnas.88.11.4771. PMID: 2052557.
- Shin SU, Friden P, Moran M, Olson T, Kang YS, Pardridge WM, Morrison SL. Transferrin-antibody fusion proteins are effective in brain targeting. Proc Natl Acad Sci USA. 1995;92(7):2820–24. doi:10.1073/pnas.92.7.2820. PMID: 7708731.
- Yu YJ, Zhang Y, Kenrick M, Hoyte K, Luk W, Lu Y, Atwal J, Elliott JM, Prabhu S, Watts RJ, et al. Boosting brain uptake of a therapeutic antibody by reducing its affinity for a transcytosis target. Sci Transl Med. 2011;3(84):84ra44. doi:10.1126/scitranslmed.3002230. PMID: 21613623.
- Niewoehner J, Bohrmann B, Collin L, Urich E, Sade H, Maier P, Rueger P, Stracke JO, Lau W, Tissot AC, et al. Increased brain penetration and potency of a therapeutic antibody using a monovalent molecular shuttle. Neuron. 2014;81(1):49–60. doi:10.1016/j.neuron.2013.10.061. PMID: 24411731.
- Thom G, Burrell M, Haqqani AS, Yogi A, Lessard E, Brunette E, Delaney C, Baumann E, Callaghan D, Rodrigo N, et al. Enhanced delivery of galanin conjugates to the brain through bioengineering of the anti-transferrin receptor antibody OX26. Mol Pharm. 2018;15(4):1420–31. doi:10.1021/acs.molpharmaceut.7b00937. PMID: 29485883.
- Kariolis MS, Wells RC, Getz JA, Kwan W, Mahon CS, Tong R, Kim DJ, Srivastava A, Bedard C, Henne KR, et al. Brain delivery of therapeutic proteins using an Fc fragment blood-brain barrier transport vehicle in mice and monkeys. Sci Transl Med. 2020;12(545): doi: 10.1126/scitranslmed.aay1359. PMID: 32461332.
- Sehlin D, Stocki P, Gustavsson T, Hultqvist G, Walsh FS, Rutkowski JL, Syvänen S. Brain delivery of biologics using a cross-species reactive transferrin receptor 1 VNAR shuttle. Faseb J. 2020;34(10):13272–83. doi:10.1096/fj.202000610RR. PMID: 32779267.
- Okuyama T, Eto Y, Sakai N, Minami K, Yamamoto T, Sonoda H, Yamaoka M, Tachibana K, Hirato T, Sato Y. Iduronate-2-sulfatase with anti-human transferrin receptor antibody for neuropathic mucopolysaccharidosis II: a phase 1/2 trial. Mol Ther. 2019;27(2):456–64. doi:10.1016/j.ymthe.2018.12.005. PMID: 30595526.
- Pardridge WM, Kang YS, Buciak JL, Yang J. Human insulin receptor monoclonal antibody undergoes high affinity binding to human brain capillaries in vitro and rapid transcytosis through the blood-brain barrier in vivo in the primate. Pharm Res. 1995;12(6):807–16. doi:10.1023/a:1016244500596. PMID: 7667183.
- Pardridge WM, Boado RJ, Giugliani R, Schmidt M. Plasma pharmacokinetics of valanafusp alpha, a human insulin receptor antibody-iduronidase fusion protein, in patients with mucopolysaccharidosis type i. BioDrugs. 2018;32(2):169–76. doi:10.1007/s40259-018-0264-7. PMID: 29442294.
- Sakamoto K, Shinohara T, Adachi Y, Asami T, Ohtaki T. A novel LRP1-binding peptide L57 that crosses the blood brain barrier. Biochem Biophys Rep. 2017;12:135–39. doi:10.1016/j.bbrep.2017.07.003. PMID: 29090274.
- Demeule M, Currie JC, Bertrand Y, Ché C, Nguyen T, Régina A, Gabathuler R, Castaigne JP, Béliveau R. Involvement of the low-density lipoprotein receptor-related protein in the transcytosis of the brain delivery vector angiopep-2. J Neurochem. 2008;106(4):1534–44. doi:10.1111/j.1471-4159.2008.05492.x. PMID: 18489712.
- Zuchero YJ, Chen X, Bien-Ly N, Bumbaca D, Tong RK, Gao X, Zhang S, Hoyte K, Luk W, Huntley MA, et al. Discovery of novel blood-brain barrier targets to enhance brain uptake of therapeutic antibodies. Neuron. 2016;89(1):70–82. doi:10.1016/j.neuron.2015.11.024. PMID: 26687840.
- Christensen SC, Krogh BO, Jensen A, Andersen CBF, Christensen S, Nielsen MS. Characterization of basigin monoclonal antibodies for receptor-mediated drug delivery to the brain. Sci Rep. 2020;10(1):14582. doi:10.1038/s41598-020-71286-2. PMID: 32884039.
- Story CM, Mikulska JE, Simister NE. A major histocompatibility complex class I-like Fc receptor cloned from human placenta: possible role in transfer of immunoglobulin G from mother to fetus. J Exp Med. 1994;180(6):2377–81. doi:10.1084/jem.180.6.2377. PMID: 7964511.
- Leach JL, Sedmak DD, Osborne JM, Rahill B, Lairmore MD, Anderson CL. Isolation from human placenta of the IgG transporter, FcRn, and localization to the syncytiotrophoblast: implications for maternal-fetal antibody transport. J Immunol. 1996;157(8):3317–22. doi:10.4049/jimmunol.157.8.3317. PMID: 8871627.
- Spiekermann GM, Finn PW, Ward ES, Dumont J, Dickinson BL, Blumberg RS, Lencer WI. Receptor-mediated immunoglobulin g transport across mucosal barriers in adult life: functional expression of FcRn in the mammalian lung. J Exp Med. 2002;196(3):303–10. doi:10.1084/jem.20020400. PMID: 12163559.
- McCarthy KM, Yoong Y, Simister NE. Bidirectional transcytosis of IgG by the rat neonatal Fc receptor expressed in a rat kidney cell line: a system to study protein transport across epithelia. J Cell Sci. 2000;113(Pt 7):1277–85. doi:10.1242/jcs.113.7.1277. PMID: 10704378.
- Kobayashi N, Suzuki Y, Tsuge T, Okumura K, Ra C, Tomino Y. FcRn-mediated transcytosis of immunoglobulin G in human renal proximal tubular epithelial cells. Am J Physiol Renal Physiol. 2002;282(2):F358–365. doi:10.1152/ajprenal.0164.2001. PMID: 11788451.
- Simister NE, Mostov KE. An Fc receptor structurally related to MHC class I antigens. Nature. 1989;337(6203):184–87. doi:10.1038/337184a0. PMID: 2911353.
- Rodewald R. Ph-dependent binding of immunoglobulins to intestinal cells of the neonatal rat. J Cell Biol. 1976;71(2):666–69. doi:10.1083/jcb.71.2.666. PMID: 11223.
- Medesan C, Radu C, Kim JK, Ghetie V, Ward ES. Localization of the site of the IgG molecule that regulates maternofetal transmission in mice. Eur J Immunol. 1996;26(10):2533–36. doi:10.1002/eji.1830261038. PMID: 8898970.
- Garg A, Balthasar JP. Investigation of the influence of FcRn on the distribution of IgG to the brain. Aaps J. 2009;11(3):553–57. doi:10.1208/s12248-009-9129-9. PMID: 19636712.
- Abuqayyas L, Balthasar JP. Investigation of the role of FcγR and FcRn in mAb distribution to the brain. Mol Pharm. 2013;10(5):1505–13. doi:10.1021/mp300214k. PMID: 22838637.
- Ruano-Salguero JS, Lee KH. Antibody transcytosis across brain endothelial-like cells occurs nonspecifically and independent of FcRn. Sci Rep. 2020;10(1):3685. doi:10.1038/s41598-020-60438-z. PMID: 32111886.
- Zhang Y, Pardridge WM. Mediated efflux of IgG molecules from brain to blood across the blood-brain barrier. J Neuroimmunol. 2001;114(1–2):168–72. doi:10.1016/s0165-5728(01)00242-9. PMID: 11240028.
- Cooper PR, Ciambrone GJ, Kliwinski CM, Maze E, Johnson L, Li Q, Feng Y, Hornby PJ. Efflux of monoclonal antibodies from rat brain by neonatal Fc receptor, FcRn. Brain Res. 2013;1534:13–21. doi:10.1016/j.brainres.2013.08.035. PMID: 23978455.
- Dall’acqua WF, Woods RM, Ward ES, Palaszynski SR, Patel NK, Brewah YA, Wu H, Kiener PA, Langermann S. Increasing the affinity of a human IgG1 for the neonatal Fc receptor: biological consequences. J Immunol. 2002;169(9):5171–80. doi:10.4049/jimmunol.169.9.5171. PMID: 12391234.
- Sommer I, Schachner M. Monoclonal antibodies (O1 to O4) to oligodendrocyte cell surfaces: an immunocytological study in the central nervous system. Dev Biol. 1981;83(2):311–27. doi:10.1016/0012-1606(81)90477-2. PMID: 6786942.
- Maxwell DS, Pease DC. The electron microscopy of the choroid plexus. J Biophys Biochem Cytol. 1956;2(4):467–74. doi:10.1083/jcb.2.4.467. PMID: 13357511.
- Hurley JV, Anderson RM, Sexton PT. The fate of plasma protein which escapes from blood vessels of the choroid plexus of the rat–an electron microscope study. J Pathol. 1981;134(1):57–70. doi:10.1002/path.1711340107. PMID: 7288528.
- Shields RL, Namenuk AK, Hong K, Meng YG, Rae J, Briggs J, Xie D, Lai J, Stadlen A, Li B, et al. High resolution mapping of the binding site on human IgG1 for Fc gamma RI, Fc gamma RII, Fc gamma RIII, and FcRn and design of IgG1 variants with improved binding to the Fc gamma R. J Biol Chem. 2001;276(9):6591–604. doi:10.1074/jbc.M009483200. PMID: 11096108.
- Roopenian DC, Christianson GJ, Sproule TJ, Brown AC, Akilesh S, Jung N, Petkova S, Avanessian L, Choi EY, Shaffer DJ, et al. The MHC class I-like IgG receptor controls perinatal IgG transport, IgG homeostasis, and fate of IgG-Fc-coupled drugs. J Immunol. 2003;170(7):3528–33. doi:10.4049/jimmunol.170.7.3528. PMID: 12646614.
- Jamieson JJ, Searson PC, Gerecht S. Engineering the human blood-brain barrier in vitro. J Biol Eng. 2017;11:37. doi:10.1186/s13036-017-0076-1. PMID: 29213304.
- Carrodus NL, Teng KS, Munro KM, Kennedy MJ, Gunnersen JM. Differential labeling of cell-surface and internalized proteins after antibody feeding of live cultured neurons. J Vis Exp. 2014;84(84):e51139. doi:10.3791/51139-v. PMID: 24561550.
- Chung S, Nguyen V, Lin YL, Lafrance-Vanasse J, Scales SJ, Lin K, Deng R, Williams K, Sperinde G, Li JJ, et al. An in vitro FcRn-dependent transcytosis assay as a screening tool for predictive assessment of nonspecific clearance of antibody therapeutics in humans. MAbs. 2019;11(5):942–55. doi:10.1080/19420862.2019.1605270. PMID: 30982394.
- Grevys A, Nilsen J, Sand KMK, Daba MB, Øynebråten I, Bern M, McAdam MB, Foss S, Schlothauer T, Michaelsen TE, et al. A human endothelial cell-based recycling assay for screening of FcRn targeted molecules. Nat Commun. 2018;9(1):621. doi:10.1038/s41467-018-03061-x. PMID: 29434196.
- Weflen AW, Baier N, Tang QJ, Van den Hof M, Blumberg RS, Lencer WI, Massol RH, Gruenberg JE. Multivalent immune complexes divert FcRn to lysosomes by exclusion from recycling sorting tubules. Mol Biol Cell. 2013;24(15):2398–405. doi:10.1091/mbc.E13-04-0174. PMID: 23741050.
- Tsao D, Thomsen HK, Chou J, Stratton J, Hagen M, Loo C, Garcia C, Sloane DL, Rosenthal A, Lin JC. TrkB agonists ameliorate obesity and associated metabolic conditions in mice. Endocrinology. 2008;149(3):1038–48. doi:10.1210/en.2007-1166. PMID: 18063676.
- Ortiz J, Harris HW, Guitart X, Terwilliger RZ, Haycock JW, Nestler EJ. Extracellular signal-regulated protein kinases (erks) and erk kinase (mek) in brain: regional distribution and regulation by chronic morphine. J Neurosci. 1995;15(2):1285–97. doi:10.1523/JNEUROSCI.15-02-01285.1995. PMID: 7532701.
- Kim GS, Cho S, Nelson JW, Zipfel GJ, Han BH, Gressens P. Trkb agonist antibody pretreatment enhances neuronal survival and long-term sensory motor function following hypoxic ischemic injury in neonatal rats. PLoS One. 2014;9(2):e88962. doi:10.1371/journal.pone.0088962. PMID: 24551199.
- Latvala S, Jacobsen B, Otteneder MB, Herrmann A, Kronenberg S. Distribution of FcRn across species and tissues. J Histochem Cytochem. 2017;65(6):321–33. doi:10.1369/0022155417705095. PMID: 28402755.
- Datta-Mannan A, Witcher DR, Tang Y, Watkins J, Wroblewski VJ. Impact of modulating the interaction of IgG with the neonatal Fc receptor. J Biol Chem. 2007;282(3):1709–17. doi:10.1074/jbc.M607161200. PMID: 17135257.
- Zalevsky J, Chamberlain AK, Horton HM, Karki S, Leung IWL, Sproule TJ, Lazar GA, Roopenian DC, Desjarlais JR. Enhanced antibody half-life improves in vivo activity. Nat Biotechnol. 2010;28(2):157–59. doi:10.1038/nbt.1601. PMID: 20081867.
- Ghetie V, Popov S, Borvak J, Radu C, Matesoi D, Medesan C, Ober RJ, Ward ES. Increasing the serum persistence of an IgG fragment by random mutagenesis. Nat Biotechnol. 1997;15(7):637–40. doi:10.1038/nbt0797-637. PMID: 9219265.
- Vaccaro C, Zhou J, Ober RJ, Ward ES. Engineering the Fc region of immunoglobulin G to modulate in vivo antibody levels. Nat Biotechnol. 2005;23(10):1283–88. doi:10.1038/nbt1143. PMID: 16186811.
- Lin C-Y, Riggers JFC, Grishanin RN, Stratton JR, Zhai W. Agonist anti-trkb monoclonal antibodies. International patent application WO2010086828A2; 2010 Jan 29.
- Kabat EA, Wu TT, Perry HM, Gottesman KS, Foeller C. Sequences of proteins of immunological interest. Maryland (USA): National Institutes of Health; 1991.
- Edelman GM, Cunningham BA, Gall WE, Gottlieb PD, Rutishauser U, Waxdal MJ. The covalent structure of an entire gammaG immunoglobulin molecule. Proc Natl Acad Sci USA. 1969;63(1):78–85. doi:10.1073/pnas.63.1.78. PMID: 5257969.
- Dall’acqua WF, Kiener PA, Wu H. Properties of human IgG1s engineered for enhanced binding to the neonatal Fc receptor (FcRn). J Biol Chem. 2006;281(33):23514–24. doi:10.1074/jbc.M604292200. PMID: 16793771.
- Titani K, Shinoda T, Putnam FW. The amino acid sequence of a kappa type Bence-Jones protein. 3. The complete sequence and the location of the disulfide bridges. J Biol Chem. 1969;244(13):3550–60. doi:10.1016/S0021-9258(18)83405-6. PMID: 4893682.
- Breiman L. Random forests. Mach Learn. 2001;45(1):5–32. doi:10.1023/A:1010933404324.