ABSTRACT
Therapeutic antibodies sometimes elicit anti-drug antibodies (ADAs) that can affect efficacy and safety. Engineered antibodies that contain artificial amino acid sequences are potentially highly immunogenic, but this is currently difficult to predict. Therefore, it is important to efficiently assess immunogenicity during the development of complex antibody-based formats. Here, we present an in vitro peripheral blood mononuclear cell-based assay that can be used to assess immunogenicity potential within 3 days. This method involves examining the frequency and function of interleukin (IL)-2-secreting CD4+ T cells induced by therapeutic antibodies. IL-2-secreting CD4+ T cells seem to be functionally relevant to the immunogenic potential due to their proliferative activity and the expression of several cytokines. The rates of the donors responding to low and high immunogenic proteins, mAb1, and keyhole limpet hemocyanin were 1.3% and 93.5%, respectively. Seven antibodies with known rates of immunogenicity (etanercept, emicizumab, abciximab, romosozumab, blosozumab, humanized anti-human A33 antibody, and bococizumab) induced responses in 1.9%, 3.8%, 6.4%, 10.0%, 29.2%, 43.8%, and 89.5% of donors, respectively. These data are comparable with ADA incidences in clinical settings. Our results show that this assay can contribute to the swift assessment and mechanistic understanding of the immunogenicity of therapeutic antibodies.
Introduction
Immunogenicity, which refers to the ability of therapeutic antibodies to produce anti-drug antibodies (ADAs), is a critical factor in drug development.Citation1 ADAs sometimes neutralize the therapeutic antibody and accelerate its clearance, thereby affecting the pharmacokinetic profile, effectiveness, and safety.Citation2,Citation3 For example, a large proportion of patients who were treated with bococizumab, a humanized antibody against proprotein convertase subtilisin/kexin type 9 (PCSK9), developed high-titer ADAs. Accordingly, the clinical development of bococizumab was discontinued due to, at least in part, this immunogenicity.Citation4 Moreover, given the increasing number of extensively engineered antibodies, including bispecific antibodies which generate potentially immunogenic neoepitopes due to modified amino acid sequences,Citation5–7 the effective prediction of ADA potential is critical for efficient drug development.
T cell-dependent responses are the primary drivers of ADAs,Citation2,Citation8 as evidenced by reports that the removal of T cell epitopes from biotherapeutic candidates ameliorates immunogenicity.Citation9–11 This T-cell dependent mechanism has inspired several methods for evaluating CD4+ T cells as an indicator of immunogenicity. Some T-cell features, including cytokine secretion, expression of activation markers, and proliferation, are associated with peripheral blood mononuclear cell (PBMC)-based in vitro assays for immunogenicity assessment.Citation12–20 However, as these assays require long culture times, often over 1 week, there is still a need to improve the efficiency of immunogenicity assessment.
Early phases of T cell responses are divided into mainly three stages. First, T cells encounter antigen-presenting cells (APCs), which then induce the production of cytokines such as interleukin (IL)-2 and interferon (IFN)-γ, finally culminating in the third phase, that of rapid proliferation.Citation21,Citation22 Given these primary T-cell responses, evaluating cytokine release, which occurs before cell proliferation, can reduce culture time. This is a promising way to speed up immunogenicity assessment. IL-2 and IFN-γ are secreted by T cells which are specific for therapeutic antibodies, and this phenomenon is one of the endpoints of immunogenicity assessment. However, in previous reports, these cytokines were measured using ELISA and ELISPOT in the proliferation phase after repeated antigen stimulation, which also required long culture times.Citation17,Citation18 Consequently, an effective assessment of immunogenic potential would benefit from more sensitive approaches that can detect low-frequency cytokine secretion before cell proliferation.
In this study, we detected IL-2 secretion from CD4+ T cells as early as Day 3 after stimulation with immunogenic therapeutic antibodies using a cytokine secretion assay, a highly sensitive method for identifying IL-2-producing cells. Seven therapeutic antibodies with known clinical ADA incidence were tested, and the frequency of IL-2 secreting CD4+ T cells was comparable with clinical immunogenicity. Furthermore, we revealed phenotypic features of IL-2-secreting CD4+ T cells, including cytokine expression and proliferation, supporting the idea that functionally relevant cells were detected in our assay.
Results
Induction of IL-2-secreting CD4+ T cells by KLH on day 3
We sought to determine whether T cell activation in vitro can be used to identify the immunogenic potential of therapeutic antibodies. As a positive control, we examined whether treatment with T cell-dependent antigen keyhole limpet hemocyanin (KLH) can induce activation in vitro using PBMCs depleted with CD8+ cells and CD25High cells, hereafter referred to as CD8−CD25Low PBMCs, which have been used for in vitro immunogenicity assays.Citation19,Citation23 CD8−CD25Low PBMCs were cultured with or without KLH from Day 0 and followed by flow cytometric analysis of IL-2-secreting CD4+ T cells on Day 3 ( and Fig. S1). KLH treatment robustly elicited IL-2 secretion from CD4+ T cells (). These results suggest that the T-cell response can be detected on Day 3 by quantifying IL-2 secretion from CD4+ T cells.
Figure 1. Induction of IL-2-secreting CD4+ T cells by KLH on Day3. (a) Representative flow cytometry plots of the IL-2 secretion on CD4+ cells. (b) Frequency of IL-2-secreting cells among CD4+ T cells (NA; n = 87, KLH; n = 46) **** p < 0.0001 (Student’s t test).

Induction of IL-2-secreting CD4+ T cells with therapeutic antibodies on day 3
To examine whether therapeutic antibodies also induce IL-2 secretion from CD4+ T cells within 3 days, CD8−CD25Low PBMCs were cultured with humanized anti-human A33 antibody (hA33), mAb1, which consists of the optimized variable region of nemolizumab and human IgG1/kappa constant region and was used in this study as a low-immunogenic control, and etanercept in addition to KLH. Flow cytometric analysis revealed that all donors tested in this experiment responded on Day 3 against not only KLH but also hA33, which is known to induce high ADA incidence in humans ( and ). mAb1 and etanercept, which are associated with low ADAs in clinic, did not elicit donor responses until Day 3 ( and ). The difference in donor responses to hA33, low-immunogenic mAb1, and etanercept was successfully detected by quantifying IL-2-secreting CD4+ T cells on Day 3.
Figure 2. Induction of IL-2-secreting CD4+ T cells by therapeutic antibodies on Day 3. (a) Frequency of IL-2-secreting cells among CD4+ T cells (n = 1–3 donors/time point). (b) Frequency of IL-2-secreting cells among CD4+ T cells and (c) frequency of responding donors (mAb1; n = 78, emicizumab; n = 53, etanercept; n = 52, abciximab; n = 47, romosozumab; n = 20, blosozumab; n = 24, hA33; n = 89, bococizumab; n = 19) **** p < 0.0001, *** p < 0.001 (binomial test, expected probability = 5%).
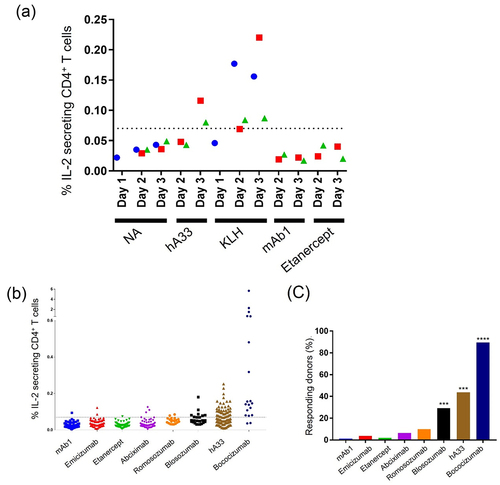
In order to appraise our assay system as an effective tool for assessing the relative potential of immunogenicity in humans, CD8−CD25Low PBMCs, which include a variety of HLA-DRB1 allele groups (Fig. S2), were cultured with mAb1 and seven therapeutic antibodies with known rates of clinical immunogenicity (), followed by flow cytometric analysis of IL-2-secreting CD4+ T cells on Day 3 (Fig. S1). As the 95th percentile of the IL-2-secreting CD4+ T cells elicited by an antibody with low clinical immunogenicity, emicizumab, was 0.07%, donors containing more than 0.07% IL-2-secreting CD4+ T cells were treated as positive. With this threshold, no antigen (NA) and KLH treatment elicited IL-2 secretion from CD4+ T cells at rates of 0% (0/87) and 93.5% (43/46) of donors with a positive response, respectively (). mAb1, emicizumab, etanercept, abciximab, romosozumab, blosozumab, hA33, and bococizumab induced responses in 1.3% (1/78), 3.8% (2/53), 1.9% (1/52) 6.4% (3/47), 10.0% (2/20), 29.2% (7/24), 43.8% (39/89), and 89.5% (17/19) of donors (). These rates tended to be comparable with the rate of ADA incidence in humans (), suggesting that this assay is a reasonable tool for evaluating immunogenicity.
To assess the reproducibility of this assay, we tested several antibodies in six cohorts, which include donors with diverse HLA-DRB1 allele groups (Fig. S2). In all additional cohorts, statistically significant responses were observed with relatively high immunogenic antibodies, blosozumab, hA33, and bococizumab, while no significant responses were induced by mAb1, emicizumab, and romosozumab (). These consisted the results in , confirming the reproducibility of this assay.
Characteristic of IL-2-secreting CD4+ T cells elicited by immunogenic protein
To further characterize this assay, we examined the phenotypic features of IL-2-secreting CD4+ T cells. RNA-Seq analysis of IL-2-secreting CD4+ T cells yielded approximately 1000 differentially expressed genes (DEGs) (fold change >5) (). To dissect the significance of DEGs in IL-2-secreting CD4+ T cells, we performed a Gene Ontology (GO) analysis using DAVID (Database for Annotation, Visualization, and Integrated Discovery). The GO terms significantly enriched in IL-2-secreting CD4+ T cells by KLH and hA33 () were mainly related to proliferation and immune activation, which aligns with T cell-dependent responses that are the primary drivers of ADAs. Consequently, we conducted a more detailed investigation of genes closely associated with immunogenicity responses. In addition to IL-2, proliferation and cytokines including IFN-γ and IL-4 are known to be indicators of T-cell responses and therefore immunogenicity.Citation17–19 To examine whether the IL-2-secreting CD4+ T cells detected in this assay also exhibited these features, a gene-expression profile of cytokines and cell cycle-related genesCitation33 was elucidated using RNA-Seq. Both IL2 and IFNG mRNA are highly expressed in isolated IL-2-secreting cells that were induced by KLH and hA33 (). IL4 expression was found in isolated IL-2-secreting cells elicited by hA33 but not in KLH-treated cells (). These expressions in cells treated with the therapeutic antibody hA33 were consistent with previous reports assessing immunogenicity.
Figure 3. Gene-expression profile of IL-2-secreting CD4+ T cells. (a, b) Scatter plot of RNA-seq analysis; genes upregulated and downregulated fivefold are denoted by red and blue, respectively. (c, d) The top 25 of gene ontology (GO) terms significantly enriched by differentially expressed genes (DEGs) by KLH (c) and hA33 (d). (e, f) RNA-Seq analysis of purified CD4+ T cells. (e) A heatmap of Log10 (FPKM +1) of cytokines and (f) a heatmap and hierarchical clustering of the Z-score of cell cycle-related genes are indicated. Expression values were Z-score transformed across samples.
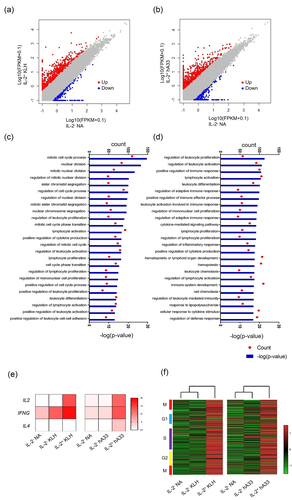
Regarding proliferation, most cell cycle-related genes, including regulators of M, G1, S, and G2 phases, were robustly induced in isolated IL-2-secreting cells induced by KLH and hA33 (). To further examine the proliferative activity of IL-2-secreting CD4+ T cells, CD8−CD25Low PBMCs, which were stained with cell proliferation dye (CPD), were treated with KLH. CD4+ T cells with or without IL-2 secretion were isolated on Day 3 and further cultured until Day 8. Cell division occurred almost exclusively in IL-2-secreting CD4+ T cells (), consistent with the gene expressions (). These results suggested that the IL-2-secreting CD4+ T cells detected in our assay were functionally relevant to immunogenicity.
Figure 4. Proliferation of IL-2-secreting CD4+ T cells induced by KLH. (a) Representative histogram of isolated CD4+ T cells on Day 8, which were stained with CPD. (b) Frequency of CPDLow cells from IL-2− cells NA control in sextuplicate, and IL-2− and IL-2+ cells induced by KLH in triplicate are shown. Data are presented as mean ± SD. *p < 0.05, ***p < 0.001 (one-way ANOVA followed by Tukey’s test).

Discussion
ADAs influence both safety and efficacy; consequently, immunogenicity is one of the key factors considered during the development of therapeutic antibodies. Here, we proposed an in vitro assay for swiftly evaluating the immunogenicity of therapeutic antibodies. We found that immune responses induced by a T cell-dependent antigen, KLH, could be measured within 3 days using IL-2 secretion from CD4+ T cells (). Furthermore, the proportion of IL-2-secreting CD4+ T cells in this assay was associated with ADAs induced by therapeutic antibodies in clinical settings ( and ).
Engineered antibodies that contain substituted amino acid sequences for better medicinal profiles, including bispecific antibodies,Citation5,Citation6 are potentially highly immunogenic.Citation7 Consequently, in order to reduce the immunogenicity risk of an antibody, especially an extensively modified one, it would be critical to select antibodies with low immunogenicity during lead selection and examine them repeatedly during lead optimization. However, commonly used in vitro T cell assays are often time-consuming, making it hard to conduct multiple examinations. One of the major advantages of our assay is that it can be completed within 3 days, allowing multiple samples to be measured in a shorter cycle than ever, which improves throughput. In this study, IL-2 secretion from CD4+ T cells was consistent with the incidence of clinical ADAs for engineered antibodies including emicizumab ().Citation34–36 Based on these results, this assay will be a very efficient tool for assessing the immunogenicity of extensively engineered antibodies.
The assay can be used for the ranking and selection of therapeutic antibody candidates that would have low immunogenic potential in future clinical use based on their ability to induce IL-2 secretion from CD4+ T cells in vitro. For example, antibodies with relatively low IL-2 secretion (<5% of donors responded), including mAb1, etanercept, and emicizumab, would be ranked as having low immunogenicity risk. Similarly, abciximab, romosozumab, blosozumab, hA33, and bococizumab, which showed higher T-cell responses (>5% of donors responded), would be ranked as having higher immunogenicity risk. The threshold between low and high immunogenicity for biotherapeutics varies depending on the purpose of the assay. For example, an antibody with a relatively lower IL-2 response could be selected from among multiple candidates, and then this lead antibody could be repeatedly optimized until it yielded a response in <5% of donors.
Results on romosozumab and blosozumab further support the usefulness of this assay for ranking and selecting therapeutic antibody candidates. During lead identification/optimization, one of the most important objectives is to select candidate(s) with low immunogenicity from among several candidate antibodies that have the same target molecule but different amino acid sequences. Romosozumab and blosozumab are both anti-sclerostin antibodies, but their clinical incidence of ADA is different. In our assay, blosozumab elicited higher T-cell responses than romosozumab, reflecting blosozumab’s higher ADA rate ( and ). These results suggest that the assay can be used to clearly identify less immunogenic candidates.
Cytokines such as IL-2 and IFN-γ, which are secreted by antigen-primed T cells, are present before proliferation,Citation21 leading to shorter cell culture times in immunogenicity assays. Such T cell activation helps antibody response by B cells, and, in particular, IL-2 is crucial for the differentiation and clonal proliferation of T cells.Citation21,Citation37 In this study, we found that IL-2-secreting CD4+ T cells exhibited proliferative activity (), which reflects this T cell activation process.
Further gene-expression profiling revealed that IL4 mRNA was highly expressed solely in IL-2-secreting CD4+ T cells induced by hA33. While IFNG mRNA was induced in IL-2-secreting CD4+ T cells induced by both KLH and hA33, its expression was stronger with KLH (). Given that IFN-γ and IL-4 are typical Th1 and Th2 cytokines, respectively, this different pattern of cytokine expression might be related to Th2 cell differentiation induced by hA33. Th2 cytokines including IL-4 drive immunoglobulin isotype switching to IgG4,Citation38 and high affinity neutralizing antibodies that reduce compound efficacy have been associated with a highly matured IgG4-based immune response.Citation39–42 Accordingly, IL-4 expression in hA33-elicited IL-2-secreting CD4+ T cells might be associated with risks of neutralizing ADA production in humans. More work is needed, however, to understand the mechanisms underlying the development of neutralizing ADAs. Also, the number of donors and tested antibodies for investigating the mechanisms in this study is limited, so these implications need to be validated on additional samples, but these results shed light on an additional application of our assay, including further characterization of T cell population, which may lead to a better mechanistic understanding of immunogenicity.
ADA responses include complex immune responses such as antigen uptake/presentation by APCs and B-cell responses in addition to T-cell activation. Accordingly, although our assay could be used to assess immunogenicity potential and improve mechanistic understanding of T-cell activation, it still cannot precisely predict or explain immunogenicity in the clinic. Furthermore, immunomodulatory antibodies, including etanercept, can occasionally influence T-cell responses. Hence, it is crucial to evaluate the immunogenicity potential from multiple perspectives, taking into account the characteristics of the tested antibody. Thus, the results from this assay should be combined with those of other assays, such as in silico T cell epitope prediction, HLA typing, identification of presented peptides by APCs with proteomic analysis of major histocompatibility complex-associated peptide proteomics, to determine the distinct immune stimulatory mechanisms of immunogenic antibodies.Citation9,Citation23,Citation43,Citation44 Also, many factors, including the type of disease, concurrent medication, and administration regimen, affect ADA incidence in patients.Citation2,Citation45 These factors cannot be taken into account by currently available in vitro assays, and thus continue to limit the precise prediction of immunogenicity risk in individual patients.
In this study, we found that clinical ADA incidence was related with T-cell activation as indicated by IL-2 secretion from CD4+ T cells in vitro. The shorter culture time enables the swift and frequent assessment of multiple therapeutic antibodies. This assay will be useful for mitigating ADA incidence in the clinic.
Materials and methods
Proteins
Mariculture KLH was purchased from Thermo Fisher Scientific (#77600). Etanercept (Enbrel) and abciximab (Reopro) were purchased from Pfizer and Eli Lilly, respectively. Anti-human A33 humanized antibody (hA33) and bococizumab were prepared according to their previously disclosed amino acid sequences.Citation46 Emicizumab was expressed in CHO cells and purified with MabSelect SuRe column chromatography followed by column chromatography and filtration. Bococizumab and mAb1 were transiently expressed using the FreeStyle 293 Expressing System (Thermo Fisher Scientific) and purified with a conventional method using protein A followed by gel filtration. mAb1 consists of the optimized variable region of nemolizumab and human IgG1/kappa constant region. Romosozumab and blosozumab were produced by the GS Xceed® expression system (Lonza) and purified from the culture supernatant by MabSelect Sure column chromatography. Gel filtration chromatography or Sartobind Q Membrane chromatography was then conducted to further purify romosozumab and blosozumab, respectively. Endotoxin and monomer of these produced antibodies were <100 mEU/mg and >99.7%, respectively. These proteins were used in the assay at final concentrations of 100 μg/ml.
Human cells
Cryopreserved PBMCs were purchased from Lonza. CD8−CD25Low PBMCs were obtained from cryopreserved PBMCs by depleting CD8+ and CD25High cells using anti-CD8-conjugated beads (Thermo Fisher Scientific, #11147D) and anti-CD25-conjugated beads (Thermo Fisher Scientific, # 11157D), respectively, according to the manufacturer’s protocol.
Cell culture
CD8−CD25low PBMCs were seeded on a 24-well plate at 1 × 10Citation6 cells/ml/well in OpTmizer™ CTS™ T-Cell Expansion medium (Thermo Fisher Scientific, A10485–01) and incubated in a humidified atmosphere of 5% CO2 at 37°C for 1 h. Subsequently, test articles were added, and the cell population was cultured further for 67 h or the durations indicated in the figures. In the case of the proliferation assay, CD8−CD25low PBMC stained with the CellTraceTM violet cell proliferation kit (Thermo Fisher Scientific, #C34557) were cultured. Then, 500 cells were sorted with FACSAria IIu from the indicated population on Day 3 and further cultured until Day 8 in 96-well round plates.
Measurement of IL-2-secreting CD4+ T cells
For labeling IL-2-secreting cells, IL-2 catch reagent (IL-2 secretion assay kit, Miltenyi Biotec, #130-090-763) was added at 10 µL/well, and the culture was continued for one more hour. Then, the cell suspension was collected and subjected to centrifugation at 4°C for culture medium removal and washing. Cells were stained with the following antibodies: anti-CD3 antibody (BD, #557705/#557749), anti-CD4 antibody (BD, #56083), anti-CD14 antibody (BD, #555398), and IL-2 Detection Antibody (IL-2 secretion assay kit, Miltenyi Biotec, #130-090-763). Cells were suspended in buffer containing 7AAD (7-Amino-Actinomycin D) (BD, #51-2359KC) before analysis to exclude dead cells. The stained cells were subjected to flow cytometry measurements under conditions where the maximum number of measurements in the lymphocyte gate is 200,000. Data were analyzed with FlowJo software (Tomy Digital Biology).
RNA isolation and RNA sequencing
Stained cells were sorted with a FACSAria III. Total RNAs were isolated using MagMAX™-96 for Microarrays Total RNA Isolation Kit (Thermo Fisher Scientific). Isolated RNAs, whose RIN values were confirmed to be greater than 8 using an Agilent RNA 6000 pico kit (Agilent), were subjected to prepare amplified cDNA and RNA-Seq library using the SMART-Seq v4 Ultra Low Input RNA Kit for Sequencing (Takara Bio), and Nextera XT DNA Library Preparation Kit (Illumina). The libraries were sequenced using HiSeq 2500 or NextSeq 500 (Illumina). The RNA-seq reads were mapped to GRCh38 with RefSeq transcript annotation using bowtie version 1.1.2, and fragments per kilobase of exon per million mapped fragments (FPKM) were calculated based on RNA-seq by Expectation-Maximization (RSEM v1.2.31).
Abbreviations
ADAs | = | anti-drug antibodies |
APCs | = | antigen presenting cells |
CPD | = | cell proliferation dye |
DAVID | = | Database for Annotation, Visualization, and Integrated Discovery |
DEGs | = | differentially expressed genes |
GO | = | Gene Ontology |
IL | = | interleukin |
KLH | = | keyhole limpet hemocyanin |
PCSK9 | = | proprotein convertase subtilisin/kexin type 9 |
PBMC | = | peripheral blood mononuclear cell |
7AAD | = | 7-amino-actinomycin D |
Author contributions
C.K. designed the study; Y.A. and C.K. wrote the manuscript; Y.A., S.M., T.I, S.I., M.Y., A.T., and C.K. executed the cellular assay and analyzed the assay data; Y.A., T.M., H.M., N.Y., and Y.N. executed the RNA-Seq experiment and analyzed the data; Z.S. produced and characterized the antibodies; M.T., S.C., M.M., J.K., H.S., and M.H. supervised the study.
Supplemental Material
Download MS Word (135.6 KB)Supplemental Material
Download MS Word (539.7 KB)Acknowledgments
The authors would also like to thank Jacob Davis for his technical editing.
Disclosure statement
No potential conflict of interest was reported by the author(s).
Supplementary material
Supplemental data for this article can be accessed online at https://doi.org/10.1080/19420862.2023.2253570.
Additional information
Funding
References
- Carter PJ, Lazar GA. Next generation antibody drugs: pursuit of the ‘high-hanging fruit’. Nat Rev Drug Discov. 2018;17(3):197–9. doi:10.1038/nrd.2017.227.
- Jawa V, Terry F, Gokemeijer J, Mitra-Kaushik S, Roberts BJ, Tourdot S, De Groot AS. T-Cell dependent immunogenicity of protein therapeutics pre-clinical assessment and mitigation-updated consensus and review 2020. Front Immunol. 2020;11:1301. doi:10.3389/fimmu.2020.01301.
- Smith A, Manoli H, Jaw S, Frutoz K, Epstein AL, Khawli LA, Theil F-P. Unraveling the effect of immunogenicity on the PK/PD, efficacy, and safety of therapeutic proteins. J Immunol Res. 2016;2016:1–9. doi:10.1155/2016/2342187.
- Ridker PM, Tardif J-C, Amarenco P, Duggan W, Glynn RJ, Jukema JW, Kastelein JJP, Kim AM, Koenig W, Nissen S, et al. Lipid-reduction variability and antidrug-antibody formation with bococizumab. N Engl J Med. 2017;376(16):1517–26. doi:10.1056/NEJMoa1614062.
- Brinkmann U, Kontermann RE. The making of bispecific antibodies. MAbs. 2017;9(2):182–212. doi:10.1080/19420862.2016.1268307.
- Spiess C, Zhai Q, Carter PJ. Alternative molecular formats and therapeutic applications for bispecific antibodies. Mol Immunol. 2015;67(2):95–106. doi:10.1016/j.molimm.2015.01.003.
- Rosenberg AS, Sauna ZE. Immunogenicity assessment during the development of protein therapeutics. J Pharm Pharmacol. 2018;70(5):584–94. doi:10.1111/jphp.12810.
- Barbosa MD, Vielmetter J, Chu S, Smith DD, Jacinto J. Clinical link between MHC class II haplotype and interferon-beta (IFN-β) immunogenicity. Clinical Immunology. 2006;118(1):42–50. doi:10.1016/j.clim.2005.08.017.
- Sekiguchi N, Kubo C, Takahashi A, Muraoka K, Takeiri A, Ito S, Yano M, Mimoto F, Maeda A, Iwayanagi Y, et al. MHC-associated peptide proteomics enabling highly sensitive detection of immunogenic sequences for the development of therapeutic antibodies with low immunogenicity. MAbs. 2018;10(8):1168–81. doi:10.1080/19420862.2018.1518888.
- Yeung VP, Chang J, Miller J, Barnett C, Stickler M, Harding FA. Elimination of an immunodominant CD4+ T cell epitope in human IFN-β does not result in an in vivo response directed at the subdominant epitope. The Journal Of Immunology. 2004;172(11):6658–65. doi:10.4049/jimmunol.172.11.6658.
- Harding FA, Stickler MM, Razo J, DuBridge RB. The immunogenicity of humanized and fully human antibodies: residual immunogenicity resides in the CDR regions. MAbs. 2010;2(3):256–65. doi:10.4161/mabs.2.3.11641.
- Wullner D, Zhou L, Bramhall E, Kuck A, Goletz TJ, Swanson S, Chirmule N, Jawa V. Considerations for optimization and validation of an in vitro PBMC derived T cell assay for immunogenicity prediction of biotherapeutics. Clinical Immunology. 2010;137(1):5–14. doi:10.1016/j.clim.2010.06.018.
- Moser JM, Sassano ER, Leistritz DC, Eatrides JM, Phogat S, Koff W, Drake DR. Optimization of a dendritic cell-based assay for the in vitro priming of naïve human CD4+ T cells. Journal Of Immunological Methods. 2010;353(1–2):8–19. doi:10.1016/j.jim.2009.11.006.
- Stickler M, Rochanayon N, Razo OJ, Mucha J, Gebel W, Faravashi N, Chin R, Holmes S, Harding FA. An in vitro human cell-based assay to rank the relative immunogenicity of proteins. Toxicol Sci. 2004;77(2):280–89. doi:10.1093/toxsci/kfh021.
- Geiger R, Duhen T, Lanzavecchia A, Sallusto F. Human naive and memory CD4+ T cell repertoires specific for naturally processed antigens analyzed using libraries of amplified T cells. J Exp Med. 2009;206(7):1525–34. doi:10.1084/jem.20090504.
- Delluc S, Ravot G, Maillere B. Quantitative analysis of the CD4 T-cell repertoire specific to therapeutic antibodies in healthy donors. FASEB J. 2011;25(6):2040–48. doi:10.1096/fj.10-173872.
- Schultz HS, Reedtz-Runge SL, Bäckström BT, Lamberth K, Pedersen CR, Kvarnhammar AM. Quantitative analysis of the CD4+ T cell response to therapeutic antibodies in healthy donors using a novel T cell: PBMC assay. PLoS One. 2017;12(5):e0178544. doi:10.1371/journal.pone.0178544.
- Joubert MK, Deshpande M, Yang J, Reynolds H, Bryson C, Fogg M, Baker MP, Herskovitz J, Goletz TJ, Zhou L, et al. Use of in vitro assays to assess immunogenicity risk of antibody-based biotherapeutics. PLoS One. 2016;11(8):e0159328. doi:10.1371/journal.pone.0159328.
- Ito S, Ikuno T, Mishima M, Yano M, Hara T, Kuramochi T, Sampei Z, Wakabayashi T, Tabo M, Chiba S, et al. In vitro human helper T-cell assay to screen antibody drug candidates for immunogenicity. J Immunotoxicol. 2019;16(1):125–32. doi:10.1080/1547691X.2019.1604586.
- Cohen S, Myneni S, Batt A, Guerrero J, Brumm J, Chung S. Immunogenicity risk assessment for biotherapeutics through in vitro detection of CD134 and CD137 on T helper cells. MAbs. 2021;13(1):1898831. doi:10.1080/19420862.2021.1898831.
- Mempel TR, Henrickson SE, Von Andrian UH. T-cell priming by dendritic cells in lymph nodes occurs in three distinct phases. Nature. 2004;427(6970):154–59. doi:10.1038/nature02238.
- Wuest SC, Edwan JH, Martin JF, Han S, Perry JSA, Cartagena CM, Matsuura E, Maric D, Waldmann TA, Bielekova B. A role for interleukin-2 trans-presentation in dendritic cell–mediated T cell activation in humans, as revealed by daclizumab therapy. Nat Med. 2011;17(5):604–09. doi:10.1038/nm.2365.
- Walsh RE, Lannan M, Wen Y, Wang X, Moreland CA, Willency J, Knierman MD, Spindler L, Liu L, Zeng W, et al. Post-hoc assessment of the immunogenicity of three antibodies reveals distinct immune stimulatory mechanisms. MAbs. 2020;12(1):1764829. doi:10.1080/19420862.2020.1764829.
- Kaymakcalan Z, Sakorafas P, Bose S, Scesney S, Xiong L, Hanzatian DK, Salfeld J, Sasso EH. Comparisons of affinities, avidities, and complement activation of adalimumab, infliximab, and etanercept in binding to soluble and membrane tumor necrosis factor. Clin Immunol. 2009;131:308–16. doi:10.1016/j.clim.2009.01.002.
- Tam SH, Sassoli PM, Jordan RE, Nakada MT. Abciximab (ReoPro, chimeric 7E3 Fab) demonstrates equivalent affinity and functional blockade of glycoprotein IIb/IIIa and α v β 3 integrins. Circulation. 1998;98(11):1085–91. doi:10.1161/01.cir.98.11.1085.
- Chouinard L, Felx M, Mellal N, Varela A, Mann P, Jolette J, Samadfam R, Smith SY, Locher K, Buntich S, et al. Carcinogenicity risk assessment of romosozumab: a review of scientific weight-of-evidence and findings in a rat lifetime pharmacology study. Regul Toxicol Pharmacol. 2016;81:212–22. doi:10.1016/j.yrtph.2016.08.010.
- Recker RR, Benson CT, Matsumoto T, Bolognese MA, Robins DA, Alam J, Chiang AY, Hu L, Krege JH, Sowa H, et al. A randomized, double-blind phase 2 clinical trial of blosozumab, a sclerostin antibody, in postmenopausal women with low bone mineral density. J Bone Miner Res. 2015;30(2):216–24. doi:10.1002/jbmr.2351.
- Bhattacharyya S, Pal S, Chattopadhyay N. Targeted inhibition of sclerostin for post-menopausal osteoporosis therapy: a critical assessment of the mechanism of action. Eur J Pharmacol. 2018;826:39–47. doi:10.1016/j.ejphar.2018.02.028.
- Ritter G, Cohen LS, Williams C, Richards EC, Old LJ, Welt S. Serological analysis of human anti-human antibody responses in colon cancer patients treated with repeated doses of humanized monoclonal antibody A33. Cancer Res. 2001;61:6851–59.
- Welt S, Ritter G, Williams Jr C, Cohen LS, John M, Jungbluth A, Richards EA, Old LJ, Kemeny NE. Phase I study of anticolon cancer humanized antibody A33. Clin Cancer Res. 2003;9:1338–46.
- King DJ, Antoniw P, Owens RJ, Adair JR, Haines A, Farnsworth A, Finney H, Lawson A, Lyons A, Baker TS, et al. Preparation and preclinical evaluation of humanised A33 immunoconjugates for radioimmunotherapy. Br J Cancer. 1995;72(6):1364–72. doi:10.1038/bjc.1995.516.
- Liang H, Chaparro-Riggers J, Strop P, Geng T, Sutton JE, Tsai D, Bai L, Abdiche Y, Dilley J, Yu J, et al. Proprotein convertase substilisin/kexin type 9 antagonism reduces low-density lipoprotein cholesterol in statin-treated hypercholesterolemic nonhuman primates. J Pharmacol Exp Ther. 2012;340(2):228–36. doi:10.1124/jpet.111.187419.
- Mizuno H, Nakanishi Y, Ishii N, Sarai A, Kitada K. A signature-based method for indexing cell cycle phase distribution from microarray profiles. BMC Genom. 2009;10(1):137. doi:10.1186/1471-2164-10-137.
- Kitazawa T, Igawa T, Sampei Z, Muto A, Kojima T, Soeda T, Yoshihashi K, Okuyama-Nishida Y, Saito H, Tsunoda H, et al. A bispecific antibody to factors IXa and X restores factor VIII hemostatic activity in a hemophilia a model. Nat Med. 2012;18(10):1570–74. doi:10.1038/nm.2942.
- Oldenburg J, Mahlangu JN, Kim B, Schmitt C, Callaghan MU, Young G, Santagostino E, Kruse-Jarres R, Negrier C, Kessler C, et al. Emicizumab prophylaxis in hemophilia a with inhibitors. N Engl J Med. 2017;377(9):809–18. doi:10.1056/NEJMoa1703068.
- Sampei Z, Igawa T, Soeda T, Okuyama-Nishida Y, Moriyama C, Wakabayashi T, Tanaka E, Muto A, Kojima T, Kitazawa T, et al. Identification and multidimensional optimization of an asymmetric bispecific IgG antibody mimicking the function of factor VIII cofactor activity. PLoS One. 2013;8(2):e57479. doi:10.1371/journal.pone.0057479.
- Boyman O, Sprent J. The role of interleukin-2 during homeostasis and activation of the immune system. Nat Rev Immunol. 2012;12(3):180–90. doi:10.1038/nri3156.
- Stone JH, Zen Y, Deshpande V. IgG4-related disease. N Engl J Med. 2012;366(6):539–51. doi:10.1056/NEJMra1104650.
- Gorovits B. Current considerations for immunoglobulin isotype characterization of antibody response against biotherapeutics. AAPS J. 2020;22(6):144. doi:10.1208/s12248-020-00530-4.
- Krishna M, Nadler SG. Immunogenicity to biotherapeutics - the role of anti-drug immune complexes. Front Immunol. 2016;7:21. doi:10.3389/fimmu.2016.00021.
- Weeraratne DK, Kuck AJ, Chirmule N, Mytych DT. Measurement of anti-erythropoiesis-stimulating agent IgG4 antibody as an indicator of antibody-mediated pure red cell aplasia. Clin Vaccine Immunol. 2013;20(1):46–51. doi:10.1128/CVI.00435-12.
- Whelan SF, Hofbauer CJ, Horling FM, Allacher P, Wolfsegger MJ, Oldenburg J, Male C, Windyga J, Tiede A, Schwarz HP, et al. Distinct characteristics of antibody responses against factor VIII in healthy individuals and in different cohorts of hemophilia a patients. Blood. 2013;121(6):1039–48. doi:10.1182/blood-2012-07-444877.
- Quarmby V, Phung QT, Lill JR. MAPPs for the identification of immunogenic hotspots of biotherapeutics; an overview of the technology and its application to the biopharmaceutical arena. Expert Rev Proteomics. 2018;15(9):733–48. doi:10.1080/14789450.2018.1521279.
- Wen Y, Cahya S, Zeng W, Lin J, Wang X, Liu L, Malherbe L, Siegel R, Ferrante A, Kaliyaperumal A, et al. Development of a FRET-Based assay for analysis of mAbs Internalization and processing by dendritic cells in preclinical immunogenicity risk assessment. AAPS J. 2020;22(3):68. doi:10.1208/s12248-020-00444-1.
- Singh SK. Impact of product-related factors on immunogenicity of biotherapeutics. J Pharm Sci. 2011;100(2):354–87. doi:10.1002/jps.22276.
- King DJ, Adair J, Owens RJ Humanized antibodies directed against A33 antigen. International Patent, Application No.: PCT/GB93/02529; 1993 filed; http://www.google.co.in/patents/WO1994013805A1?cl=en&hl=ja.