It has been proposed, that ‘the better running economy and personal best time obtained with the VP4 [Vaporfly 4% or similar advanced shoe constructions] cannot be currently explained from a [functional] biomechanical standpoint’ (Patoz et al., Citation2022). It seems that the biomechanics research community doesn’t know (a) which footwear features are the most important with respect to improvements in running performance and (b) don’t know why specific features influence running performance. A combination of footwear features like compliant and resilient midsole material (Healey and Hoogkamer, Citation2021; Hoogkamer et al., Citation2019), heel drop (Burns & Tam, Citation2020; Nigg et al., Citation2020), stiff curved carbon-fibre plate (Burns & Tam, Citation2020; Hoogkamer et al., Citation2019; Nigg et al., Citation2020; Subramanium & Nigg, Citation2021) and/or forefoot lift (Nigg et al., Citation2020) have been proposed as major reasons for the performance improvement achieved when running in footwear with these features. However, explanations for why these footwear features would improve performance have typically not been proposed by researchers. One exception is the ‘teeter-totter effect’ (Nigg et al., Citation2020), which has been proposed as a mechanism for how these shoes improve performance. However, the ‘teeter-totter effect’ mechanism needs still to be demonstrated with experimental evidence.
Additionally, sport shoe manufacturers provide some suggestions as to what footwear features result in improved performance. Nike, for instance, suggests (in their publicly available comments about these shoes) that the combination of their Pebax foam and curved carbon-fibre plate in the midsole is the game changer (Alger, Citation2019). However, they do not (publicly) provide a functional explanation of the mechanism by which these features could improve running performance.
It seems, therefore, important that we try to understand the footwear features that are important to improve running performance and specially to understand the mechanism by which each feature or combination of features influences performance. An understanding of the relevant footwear features and their functioning would inform future running shoe designs that continue to elevate the limits of human performance. This short paper is an attempt to try to understand why the biomechanics community, and possibly also the shoe manufacturers, don’t understand which shoe features improve running performance and the biomechanical explanation for why they influence running performance.
We see three factors as the main reasons for this shortcoming:
Most studies compare group means of the variables of interest and neglect discussing individual subjects.
Most studies identify differences in group means, but don’t base their studies on a proposed biomechanical mechanism.
Most studies quantify running economy and biomechanical changes using treadmill running.
We will discuss these three aspects in the following.
Comparison of group means
Some of the problems that exist when comparing group means has already been discussed earlier (Patoz et al., Citation2022). Shortly, the typical approach in the studies evaluating advanced running shoes was to compare running economy for two or more specific shoe constructions and identify significant differences, on average, between shoe constructions (e.g., Hoogkamer et al., Citation2018, Citation2019). As the Patoz group outlined:
… as large interindividual variations were reported in terms of running economy in response to the VP4 shoe (Barnes & Kilding, Citation2019; Hébert-Losier et al., Citation2022), pooling all runners together might mask an effect that would be observed when only considering high responders, ….
The limitation of using group means for analyses is also prevalent in clinical research examining the relationship between footwear characteristics and running related injuries (Ramsey et al., Citation2019). The complex interactions between each runner’s unique technique, anatomy, injury and training history makes it challenging to understand the contribution of each potential factor to injuries when only differences in group means between injured and non-injured are compared. Comparison of mean values acts like a filter and limits the understanding of individual specific response to an intervention (e.g. a new footwear construction). However, the strategy of comparing ‘high responders’ and ‘non responders’ still does not provide us with a biomechanical explanation, because the basic mechanical or physiological functioning of an intervention was not included in the study design. This approach will only provide us with the information about differences in average outcome measures between shoe conditions. For example, that running economy was on average different between the two tested shoe constructions. It will, however, not provide any information why it was different.
Propose a biomechanical mechanism
As highlighted by Patoz et al. (Citation2022), there is currently an ‘absence of any biomechanical explanation for the better performance’. Rather, most studies compare biomechanical variables and running economy across multiple shoe constructions and report when there are statistical differences between various shoe constructions. To demonstrate why a specific shoe construction or footwear feature improves running performance, a biomechanical model or a mechanism has to be proposed. Such a model would connect shoe construction features to specific biomechanical variables that influence running performance (i.e. running economy). We will illustrate this approach with an example of one proposed biomechanical mechanism: the ‘teeter-totter effect’ (Nigg et al., Citation2020).
The proposed system of interest includes a running shoe and a foot. The shoe has a toe construction with an upward curvature (toe-spring), such that the runner rocks over the sole of the shoe throughout the running stance phase. During heel-to-toe running, the point of application of the resultant force under the foot translates from the heel region to the forefoot region. The proposed teeter-totter mechanism suggests that when the point of application of the resultant force moves forward, the relatively stiff construction of the total shoe (plate, connection between sole and upper, upper) enables a forward rotation of the rear part of the shoe (). The model assumes that the rotation occurs about a pivot axis that is a region of the shoe outsole currently in contact with the ground. The pivot axis may change throughout the duration of the running stance phase as the runner rocks over the sole of the shoe.
Figure 1. Schematic illustration of the teeter-totter effect including the applied force of the runner (solid arrow) at the forefoot, the reaction force at the mid-rearfoot (hollow arrow) and the pivot axis (black dot) during two time-points in stance phase. This is not a free body diagram.
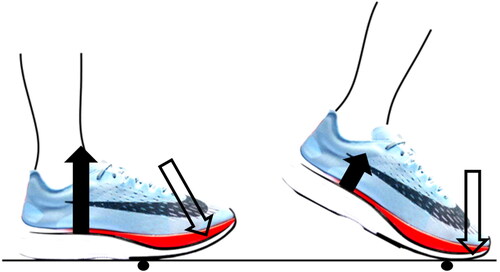
Once a biomechanical mechanism is proposed, to connect the mechanism (such as the teeter-totter effect) to changes in running performance, calculations must be performed to quantify specific biomechanical variables that influence running performance. With the proposed tetter-totter mechanism, specifically, a method must first be developed to quantify the ‘teeter-totter moment’, the moment of a force acting at the mid-rearfoot about a pivot axis during take-off. To achieve this, the resultant force acting on the mid-rearfoot must be quantified. This is methodologically challenging since (i) the mid-rearfoot forces during late stance are small and close to the noise of the measuring insoles if pressure insoles are used, (ii) assigning portions of the complete ground reaction force to individual foot segments is challenging if force plates are used (Bruening et al., Citation2012) and (iii) quantifying foot segment kinematics during shod locomotion is challenging (McDonald et al., Citation2019). Next, ‘teeter-totter moments’ would have to be synthesized with whole-body mechanical work to understand changes in the work performed by the entire runner-shoe system. We hypothesize an increase in the ‘teeter-totter moment’ may provide a performance benefit by propelling the runner’s heel upwards and forward during take-off. If such teeter-totter effect exists, then the work required by the runner during take-off may decrease (e.g., decreased muscle forces during push-off). This may improve running economy and allow for increased running performance.
In summary, first a biomechanical mechanism is proposed. Next, calculations are performed that quantify the effect of the proposed mechanism. Then, such calculations are related to expected changes in running economy and/or running performance. If the mechanism calculations are in agreement with the measured running economy differences for the specific shoe, one has a possible biomechanical explanation for the changes in running economy and resulting running performance. If the mechanism calculations are not in agreement with the running economy differences, one cannot (entirely) attribute the proposed mechanism to the changes in running economy. Furthermore, a biomechanical mechanism by definition needs to hold true in all situations (e.g., across all individuals). As such, if the mechanism expectations do not match reality for all individuals (see first point), then the model cannot (entirely) explain the changes in running economy and resulting running performance.
Note, the ‘teeter-totter effect’ has been proposed as a possible functional explanation for performance improvements seen in several advanced running shoes. However, experimental or simulation calculations for the existence of the teeter-totter effect are still missing. The point that is important in this context is that proposing a biomechanical mechanism and comparing the experimental results to the measured running economy results may help provide functional/mechanistic explanations for the improvements in running performance.
Treadmill and overground
Due to methodological constraints, running economy and/or changes in biomechanical variables in various shoe constructions are often measured during lab-based treadmill running. Treadmill running is typically necessary for quantifying running economy because protocols require multiple minutes (e.g. 5 min) of continuous steady-state running (Morgan et al., Citation1989). Treadmill running is also convenient for quantifying changes in biomechanical variables during steady-state (often high speed) running. On the other hand, the extraordinary running performances achieved in advanced shoes occur during overground running (e.g. during an actual marathon). Considering there are differences between actual improvement of performance and measured differences in running economy (Kipp et al., Citation2019), there may be limitations to correlating lab-based treadmill results to overground running performance times.
One limitation is that running mechanics may differ during overground vs. treadmill running. Furthermore, the effects of certain shoe constructions on running mechanics may vary between these two scenarios. To illustrate this, we return to the proposed ‘teeter-totter effect’. One important indicator of the ‘teeter-totter effect’ is that the centre-of-pressure (COP) moves posteriorly during late stance, indicating a reaction force under the mid-rearfoot during take-off. demonstrates that for a representative athlete running in the Nike Vaporfly 4% shoe, a posterior movement of the COP occurs during late stance while running overground (dashed line), but not while running on the treadmill (solid line). This result suggests that runners may be using different movement strategies while running overground versus running on the treadmill (García-Pérez et al., Citation2013; van Caekenberghe et al., Citation2013; Van Hooren et al., Citation2020), even in the same footwear (Chambon et al., Citation2015). If the COP at late stance is a key variable in a proposed biomechanical mechanism (such as the ‘teeter-totter effect’), it would be challenging to demonstrate this mechanism during treadmill running. Thus, it may be wise to interpret treadmill measurements with caution. Furthermore, one can develop methods to extrapolate treadmill running measurements to expectations during overground running and/or develop a convenient method for quantifying running economy when running overground.
Final comments
A missing biomechanical understanding of the effect of specific interventions is not only a sport shoe biomechanics problem. It is present in many biomechanical studies. One example is understanding the effect of forces on the development of osteo-arthritis (D’Lima et al., Citation2012; Neogi, Citation2013). Three factors that may contribute to this missing biomechanical understanding of advanced running shoes on performance include: focus on comparing group means, study designs that lack a proposed biomechanical mechanism and challenges with associating treadmill running results to overground running performances. Moving forward, researchers exploring the effect of footwear constructions on running performance should aim to understand individual specific adaptations, propose biomechanical mechanisms (such as the ‘teeter-totter effect’) and continue to understand how we can connect treadmill measurements to overground running performance.
Disclosure statement
No potential conflict of interest was reported by the author(s).
References
- Alger, K. (2019, May 15). Sole sensations: The bottom bits of the latest running shoes, explained. Runner’s World. https://www.runnersworld.com/uk/gear/shoes/a27476498/running-shoes-sole-explained/
- Barnes, K. R., & Kilding, A. E. (2019). A randomized crossover study investigating the running economy of highly-trained male and female distance runners in marathon racing shoes versus track spikes. Sports Medicine, 49(2), 331–342. https://doi.org/10.1007/s40279-018-1012-3
- Bruening, D. A., Cooney, K. M., & Buczek, F. L. (2012). Analysis of a kinetic multi-segment foot model part II: Kinetics and clinical implications. Gait & Posture, 35(4), 535–540. https://doi.org/10.1016/J.GAITPOST.2011.11.012
- Burns, G. T., & Tam, N. (2020). Is it the shoes? A simple proposal for regulating footwear in road running. British Journal of Sports Medicine, 54(8), 439–440. https://doi.org/10.1136/bjsports-2018-100480
- Chambon, N., Delattre, N., Guéguen, N., Berton, E., & Rao, G. (2015). Shoe drop has opposite influence on running pattern when running overground or on a treadmill. European Journal of Applied Physiology, 115(5), 911–918. https://doi.org/10.1007/S00421-014-3072-X
- D’Lima, D. D., Fregly, B. J., Patil, S., Steklov, N., & Colwell, C. W., Jr (2012). Knee joint forces: Prediction, measurement, and significance. Proceedings of the Institution of Mechanical Engineers. Part H, Journal of Engineering in Medicine, 226(2), 95–102. https://doi.org/10.1177/0954411911433372
- García-Pérez, J. A., Pérez-Soriano, P., Llana, S., Martínez-Nova, A., & Sánchez-Zuriaga, D. (2013). Effect of overground vs treadmill running on plantar pressure: Influence of fatigue. Gait & Posture, 38(4), 929–933. https://doi.org/10.1016/J.GAITPOST.2013.04.026
- Healey, L., & Hoogkamer, W. (2021). Longitudinal bending stiffness does not affect running economy in Nike Vaporfly shoes. SportRxiv. https://doi.org/10.1016/j.jshs.2021.07.002
- Hébert-Losier, K., Finlayson, S. J., Driller, M. W., Dubois, B., Esculier, J. F., & Beaven, C. M. (2022). Metabolic and performance responses of male runners wearing 3 types of footwear: Nike Vaporfly 4%, Saucony Endorphin racing flats, and their own shoes. Journal of Sport and Health Science, 11(3), 275–284. https://doi.org/10.1016/j.jshs.2020.11.012
- Hoogkamer, W., Kipp, S., Frank, J. H., Farina, E. M., Luo, G., & Kram, R. (2018). A comparison of the energetic cost of running in marathon racing shoes. Sports Medicine, 48, 1009–1019. https://doi.org/10.1007/s40279-017-0811-2
- Hoogkamer, W., Kipp, S., & Kram, R. (2019). The biomechanics of competitive male runners in three marathon racing shoes: A randomized crossover study. Sports Medicine, 49(1), 133–143. https://doi.org/10.1007/s40279-018-1024-z
- Kipp, S., Kram, R., & Hoogkamer, W. (2019). Extrapolating metabolic savings in running: Implications for performance predictions. Frontiers in Physiology, 10, 79. https://doi.org/10.3389/fphys.2019.00079
- McDonald, K. A., Honert, E. C., Cook, O. S., & Zelik, K. E. (2019). Unholey shoes: Experimental considerations when estimating ankle joint complex power during walking and running. Journal of Biomechanics, 92, 61–66. https://doi.org/10.1016/J.JBIOMECH.2019.05.031
- Morgan, D. W., Martin, P. E., & Krahenbuhl, G. S. (1989). Factors affecting running economy. Sports Medicine, 7(5), 310–330. https://doi.org/10.2165/00007256-198907050-00003
- Neogi, T. (2013). The epidemiology and impact of pain in osteoarthritis. Osteoarthritis and Cartilage, 21(9), 1145–1153. https://doi.org/10.1016/J.JOCA.2013.03.018
- Nigg, B. M., Cigoja, S., & Nigg, S. R. (2020). Effects of running shoe construction on performance in long distance running. Footwear Science, 12(3), 133–138. https://doi.org/10.1080/19424280.2020.1778799
- Nigg, B. M., Cigoja, S., & Nigg, S. R. (2021). Teeter-totter effect: A new mechanism to understand shoe-related improvements in long-distance running. British Journal of Sports Medicine, 55(9), 462–463. https://doi.org/10.1136/bjsports-2020-102550
- Patoz, A., Lussiana, T., Breine, B., & Gindre, C. (2022). The Nike Vaporfly 4%: A game changer to improve performance without biomechanical explanation yet. Footwear Science, 14, 1–4. https://doi.org/10.1080/19424280.2022.2077844
- Ramsey, C. A., Lamb, P., Kaur, M., David Baxter, G., Cury, D., & Cury Ribeiro, D. (2019). How are running shoes assessed? A systematic review of characteristics and measurement tools used to describe running footwear. Journal of Sports Sciences. 37(14), 1617–1629. https://doi.org/10.1080/02640414.2019.1578449
- Subramanium, A., & Nigg, B. (2021). Exploring the teeter-totter effect in shoes with curved carbon fibre plates. Footwear Science, 13(sup1), S74–S75. https://doi.org/10.1080/19424280.2021.1917689
- Van Caekenberghe, I., Segers, V., Willems, P., Gosseye, T., Aerts, P., & De Clercq, D. (2013). Mechanics of overground accelerated running vs. running on an accelerated treadmill. Gait & Posture, 38(1), 125–131. https://doi.org/10.1016/J.GAITPOST.2012.10.022
- Van Hooren, B., Fuller, J. T., Buckley, J. D., Miller, J. R., Sewell, K., Rao, G., Barton, C., Bishop, C., & Willy, R. W. (2020). Is motorized treadmill running biomechanically comparable to overground running? A systematic review and meta-analysis of cross-over studies. Sports Medicine, 50(4), 785–813. https://doi.org/10.1007/s40279-019-01237-z