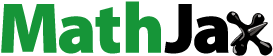
Abstract
The 5 August 2014 Orkney earthquake in South Africa caused significant damage to low-cost unreinforced masonry buildings. After the earthquake, post-earthquake surveys were conducted to assess damage and deduce the intensity experienced by these buildings. During these surveys, only buildings that were reported as damaged were investigated. This study conducted an empirical seismic vulnerability assessment using data collected from those surveys. However, for a comprehensive assessment, the data should include all buildings in the study area. Hence in this study, a first-order approximation was applied to gather sufficient data to construct fragility curves for low-cost unreinforced masonry buildings in South Africa. The damage probability matrix technique was used for fragility curve construction. The fragility curves were constructed using the intensity and damage data, statistical models, and model fitting techniques. The fragility curves obtained in this study predicted comparable but slightly lower damage compared to other curves for unreinforced masonry buildings of similar typology. Although the method applied was able to produce fragility curves comparable with other studies, it is recommended that in order to get a reliable fragility curves all the buildings in the study area should be investigated including those that suffered little to no damage.
1. Introduction
Around the world, masonry buildings have a long and rich construction history, well-established construction methods and materials, and are relatively low-priced. These buildings are extensively used both in developed and developing countries worldwide. Sorrentino et al. (Citation2019) argued that even though masonry buildings have several advantages, they are extremely vulnerable to seismic damage. Damages to these buildings have been documented in several studies (Işık, E., 2023; Işık et al. Citation2023; Bilgin et al. Citation2022; Kiyono and Kalantari Citation2004). Halder et al. (Citation2020) showed that unreinforced masonry (URM) buildings would experience heavy damage even for a seismic event having peak ground acceleration (PGA) as low as 0.18 g, which is the design parameter for buildings in the Northeast region of India as per the Indian seismic code. Meanwhile, Bilgin et al. (Citation2022) established that low-rise masonry buildings could reach the near collapse damage state at a PGA of 0.16 g. Yön (Citation2021) determined that after an earthquake some sections of the affected structure may be unsafe for habitation, depending on the level of damage experienced by the structure. The damage experienced by the structure depends on the amount of shaking, local geological conditions, building design, construction methods and materials of the building (Panzera et al. Citation2018). Assessing post-earthquake damage for vulnerability assessment comes with many challenges, one being that methods that produce very detailed damage data are associated with small sample size while methods involving large samples tend to not record details about damage.
In this study an empirical seismic vulnerability assessment was used to characterize seismic vulnerability of low-cost unreinforced masonry (URM) buildings that were damaged during the 2014 Orkney seismic event using data collected from a post-earthquake field survey. In that survey, damage data was obtained from buildings that were reported to have been damaged while other buildings were not surveyed. However, for a comprehensive assessment, the data should include all buildings in the study area, regardless of whether they were damaged or undamaged. Hence in this study a first order approach was applied to gather sufficient data to construct fragility curves for low-cost unreinforced masonry buildings in South Africa.
Efforts have been made for many years to determine the seismic vulnerability of structures to earthquake damage. Seismic vulnerability assessment is utilized in seismic risk management and prioritizing pre-earthquake strengthening of structures (Menegon et al. Citation2019). Three key methods are used for expressing seismic vulnerability of structures to earthquake damage: empirical assessment approach, analytical assessment approach, and hybrid assessment approach (Kassem et al. Citation2020). The empirical method was chosen for this study since it utilises visual observations to estimate seismic vulnerability. Empirical seismic vulnerability assessment requires three primary components: probable ground motions, damage, and building typology.
Unreinforced masonry buildings are the most common type of buildings in the Orkney area. These buildings are particularly vulnerable to seismic activities due to their structural typology and sub-standard construction materials such as masonry materials that are not reinforced, weak mortar between the bricks and unsupported walls (Khoyratty Citation2016). The reliability of the empirical method largely depends on the quantity and quality of the observed data; hence this study used first order approximation for improving the quantity of data for reliable fragility curve construction. The aim of the study was to predict damage of unreinforced masonry buildings in future earthquakes and provide guidelines for the design parameters of these buildings in terms of seismic shaking. Such information could inform emergency response teams and disaster planning by the state authority.
The Orkney region is situated in a seismically active zone, and the majority of inhabitants around this area reside in low-cost, URM buildings provided by the government and other relevant stakeholders. These structures are highly vulnerable to seismic activities, contributing to increased seismic risk as they are exposed to significant seismic hazards. To enhance the design of these buildings, it is crucial to investigate the level of seismic hazard that can result in damage. This is typically achieved through the use of fragility curves. While fragility curves for URM buildings have been developed for several studies (Ademović et al. Citation2020; Biglari and Formisano; 2020; Rosti et al. Citation2018), however, there are currently no existing fragility curves for these types of buildings in South Africa. This gap can be attributed to the insufficient data available for constructing fragility curves. The proposed study aims not only to construct fragility curves for unreinforced masonry buildings in South Africa but also to utilize a first-order approach in working with the available data to construct empirical fragility curves.
1.1. Seismicity in the Orkney region
The Orkney region in South Africa, is located in an intraplate region, these regions are characterized with low frequency of seismic activities. According to Jogunoori (Citation2012), intraplate seismic events occurrence is 6 times lower than interplate earthquakes. Between the years 1980 and 2014, the Orkney region experienced close to 400 seismic events with local magnitudes (ML) equal to or greater than 2.5, two having a local magnitude greater than (ML) of 5 (see ). These events included the 2005 Stilfontein event and the 2014 Orkney event. The seismic activities in this region are suspected to be caused by mining activities (Brandt Citation2011), since there was no clear evidence of seismic activities in the area before mining activities began. On March 9, 2005, an earthquake with a local magnitude (ML) of 5.3 struck approximately 2,400 meters deep, in a mining area in Stilfontein. This earthquake caused damage to several buildings most notably to four apartment blocks, two schools and an old age home, leaving more than 50 people injured and claimed the life of two mine workers. It is crucial to highlight that seismic events are a periodic occurrence in the Orkney region, the 2014 earthquake being the largest one so far.
Figure 1. Seismic events around Orkney regions with local magnitude equal to or greater than (ML) 2.5 for the period between 1980 and 2014.
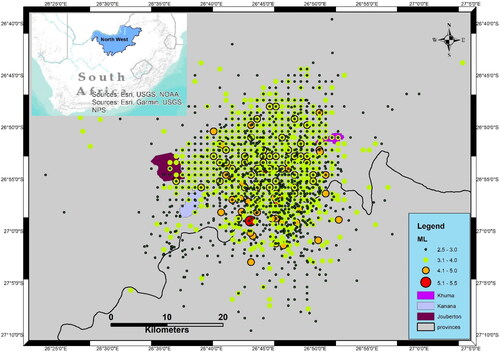
The 2014 Orkney earthquake had a local magnitude of (ML) 5.5 with a focal depth of 5 km. It occurred on 5 August 2014 in the city of Matlosana, North West province in South Africa (see ). This earthquake was felt throughout South Africa and in some neighbouring countries such as Lesotho, Swaziland, Botswana and Mozambique. It had a European macro-seismic scale (EMS-98) intensity range of between I and VIII (see ). It resulted in one casualty and damaged more than 600 structures, the vast majority being low-cost URM buildings within the radius of 50 km from the epicenter, most notably Khuma, Kanana and Jourberton (see ). This earthquake highlighted the vulnerability of such buildings to earthquake damage, as some better constructed buildings closer to the epicentre were not damaged. Over 1000 shocks were documented in the month following this earthquake, it was suspected that these were aftershocks triggered by the main event (Manzunzu et al. Citation2017). The effects of the 2014 Orkney earthquake varied significantly across the region. Mulabisana et al. (Citation2019) reported variations of frequency and amplitude ratios across different sites; frequencies varying between 0.5 and 35 Hz with an average of 7.9 Hz. The amplitude ratios varied strongly from 1.66 to 11.69, with only two with maximum peaks smaller than 2.
Figure 2. Outline of the areas mostly affected by the 2014 Orkney event and intensity map of the event.
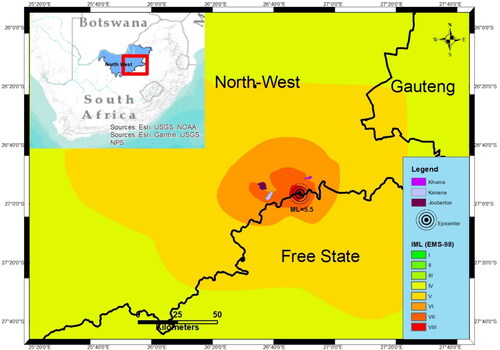
Geological conditions play a significant role in the variation of earthquake effects. The average shear wave velocity is greater on hard rocks such as granite and slower through less compacted rocks such as silt stone and unconsolidated sediments. Similarly, seismic waves travel at higher velocities through highly elastic rocks and slower through those with lower elasticity. Additionally, the geological composition of the Earth’s crust plays a role in how far the effects of an earthquake are perceptible. Aged, well-consolidated, and less fractured crust allows seismic energy to propagate more efficiently. The characteristics of the geology of the area may affect the intensity of the ground motion during an earthquake. Soft, loose soils such as clay, sand, and gravel may cause seismic waves to increase in amplitude and duration while hard and dense rock such as granite can attenuate seismic waves, causing them to decrease in amplitude and duration. Borcherdt (Citation1970) reported that maximum horizontal velocities for a site underlain by younger mud were ten times greater than those recorded in bedrock.
The Orkney region is prone to seismic activities, possibly due to extensive mining operations in the area. The allure of mining opportunities has attracted numerous residents, leading to a surge in demand for affordable housing. Consequently, the government and other stakeholders invested in low-cost housing for disadvantaged individuals. This has created a precarious situation where earthquake-vulnerable, low-cost buildings are being constructed in a seismically active region, resulting in an elevated seismic risk for these buildings.
1.2. Unreinforced masonry buildings structural typology
Unreinforced masonry (URM) buildings exist globally and have been used since 4000 BCE for providing shelter. These buildings are found throughout Latin America, the Himalayan region, Africa, Europe, the Indian subcontinent and Asia. This type of buildings has several advantages, such as fire resistance, inexpensive construction, weather protection and providing thermal insulation. There are several variations of URM buildings, In Seattle in the United States, the most common URM buildings are three story buildings constructed from bricks and mortar which have wooden-frames and roofs without structural reinforcement, and these are prone to collapse during an earthquake (Perbix and Burke Citation1989). These are old buildings that were constructed without the considerations of building codes and are more prone to earthquake damage (Bruneau Citation1994). In preliminary surveys carried out in 2007 and 2012, it was determined that more than 800 old URM buildings were vulnerable to collapse during a big earthquake such as the 2001 Nisqually earthquake with a moment magnitude of 6.8 (Doughton and Raghavendran Citation2015). In India the most common URM buildings are two story buildings constructed from bricks and mortar which have wooden-frames and roofs (Sinha and Brzev Citation2002). These buildings are also constructed without the consideration of any building codes and guidelines and as a result they suffered devastating damage during the 1997 Jabalpur earthquake of moment magnitude 5.8 (Bhattacharya et al. Citation2014).
Extensive research has been conducted in recent decades to reduce building vulnerability to earthquake damage, resulting in new building codes, however, buildings built in terms of these codes are characteristically found in wealthy countries. Several hospitals in Turkey were constructed considering the latest buildings codes, including features such as beams and columns that absorb the energy of the earthquake and earthquake resilient systems such as seismic isolation system and seismic dampers which minimize the energy that goes into the buildings by absorbing or diverting it. During the 6 February 2023 Turkey–Syria earthquakes of moment magnitude 7.8 and 7.7 these buildings saw a 75% reduction in earthquake shaking, survived the earthquake with minimal damage and remained functional after it (Ozbulut Citation2023). Over the past 30 years Iran saw a decrease in the use of masonry materials in buildings that were designed according to the Iranian standard seismic code (Standard No. 2800), however, buildings constructed from masonry materials and without the implementation of buildings codes still exist in rural areas and some monumental and historical buildings. One of the features that make these buildings vulnerable to earthquake damage is that they have no horizontal and vertical confining elements (Biglari and Formisano Citation2020). For most developing regions in Latin America, Africa, Asia, the Middle East and Southern Europe, the most common type of URM buildings are single story buildings constructed from brick and mortar. These buildings are generally constructed from basic masonry materials and are built without following any building regulations or building codes, resulting in continuing vulnerability to earthquake damage (Bhattacharya et al., 2014). Residents in these areas typically have limited financial resources and limited expertise in engineering or construction, so there has been minimal effort to safeguard them from the seismic risks posed by earthquakes.
One of the most common types of URM buildings in South Africa are houses provided by the Housing Development Agency to those who need basic shelter (see ). These are rectangular buildings constructed from concrete bricks and cement mortar plastered with one coat cement plaster. They typically have an area of 35 square meters with dimensions of ca. 7 m by 5 m and a base height of 2.5 m with 1 external door, three interior doors, four exterior windows and a total of 4 rooms (). These were the buildings that were most damaged by the 2014 Orkney earthquake and were the ones assessed in this study. They were constructed between 1998-2006 in accordance with the South African Bureau of Standards (SABS) 1200 building standards. These standards were developed in the 1970s and covered various aspects of building construction and safety, including structural design, materials, and building practices but did not have any specification for seismic actions. SABS 1200 remains the primary reference for construction in the country, however the building standards landscape in South Africa has evolved since then, and newer standards have been introduced. In 2011 the South African Bureau of Standards introduced SABS 10160-4, which provides strategies and guidelines for designing buildings that are subject to seismic actions, mainly to protect against major catastrophic structural failures and loss of life. These standards also specify the seismic zones within which structures must be designed and constructed to withstand the impact of seismic ground motion. To sum up, the buildings in this study were constructed prior to the introduction of the building codes that consider the impact of seismic events. Prior the 2014 Orkney earthquake there were no reported damages to these buildings, hence this the first study to investigate earthquake damage to them. Pule et al. (Citation2015) discussed that most buildings and structures in South Africa are vulnerable to seismic activities since they lack design elements to withstand even mild seismic activities. Seismic resistance is generally not regarded as a standard design consideration by most architects, engineers, and builders in the country.
Figure 3. Structural layout of a common unreinforced masonry building in South Africa, (top left) floor plan; (top right) side elevation; (bottom left) front elevation and (bottom right) photograph.
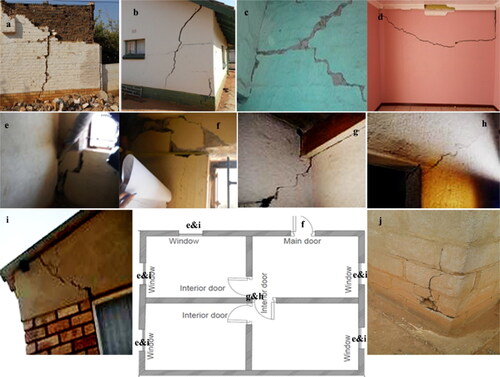
While URM buildings are a favourable for simple and affordability housing, they pose a significant risk when exposed to seismic activities, especially those constructed without the implementation of building codes
1.3. Vulnerabilities of URM buildings to earthquake damage
Although masonry buildings have several advantages that make them a suitable choice for providing basic shelter they have disadvantages, one being their vulnerability to earthquake-generated ground vibration. On 06 February 2023, two earthquakes in Turkey with moment magnitudes of 7.8 and 7.7 resulted in significant building damage across 10 provinces (Erdik et al. Citation2023). Most of these buildings were unreinforced masonry buildings that were constructed prior the implementation of earthquake-resistant design codes (Erdik et al. Citation2023; Işık Citation2023). The site survey of damage of buildings by the 5 August 2014 Orkney event, confirmed some of the known vulnerabilities of URM buildings to earthquake damage (Khoyratty Citation2016). Some of the factors that validate their vulnerability to seismic activities are poor mortar (), weak load bearing walls (), weak unsupported walls (), lack of vertical confining elements, poorly supported wall openings (), inadequate brick strength (), as well as heavy and stiff structural design.
Figure 4. Floor plan of unreinforced masonry buildings and damages that were observed after the 2014 Orkney seismic event. (a) vertical crack, (b, c) diagonal crack, (d) horizontal crack, (e) crack propagating from window frame; (f–i) crack propagating from door frame, and j) brick failure.
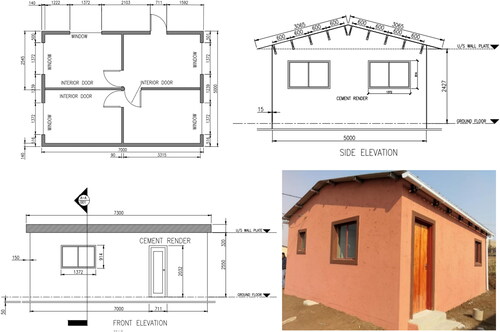
Both load bearing and non-load bearing walls of masonry buildings are made of heavy masonry materials like bricks or stones, bound together with mortar. If the strength of these brick units is less than that of the design strength, they become weak points of the structure and may fail when subjected to seismic loading (). Unreinforced masonry walls are typically stiff and brittle, excessive bending and shearing during an earthquake can result in failing of these walls, these failures are usually noticed as horizontal, vertical and diagonal cracks (). This common weakness of unreinforced masonry walls can cause the building to become unstable which subsequently may lead to collapse. Horizontal cracks are typically found at the intersection of the walls with the roof or floor and vertical cracks are common where walls intersect other walls. These cracks may form as a result of weak finish that is unable to hold together the difference in movement between walls. Most buildings that collapsed or experienced severe damage during earthquakes in Turkey between 1992 and 2004 had failure in load bearing walls (Doğangün et al. Citation2008). Unreinforced masonry buildings usually lack vertical confining elements, which can lead to the formation of diagonal cracks propagating from openings of the building (). Most buildings must have wall openings such as windows and doors, which introduce weak-points where most of the damage starts to occur, since these areas act as hinges and may rotate as the building undergoes back and forth movements. In URM buildings, most mortar that is used to hold bricks together may not be strong enough. This was observed in the Northern part of the Netherlands, where the buildings were not designed and constructed to resist earthquake loading and where some of the building materials were below standard for resisting earthquake loading (Jafari et al. Citation2017). During the 2014 Orkney earthquake one person was killed as a result of a wall collapsing on him (Khoyratty Citation2016). Weak mortar may also cause masonry elements to peel from the building and fall onto occupants ().
Masonry buildings are generally heavy and rigid, making them effective in withstanding hurricanes and strong winds, however, the rigidity makes the structure more vulnerable to earthquake damage. This was evident in unreinforced masonry structures in Haiti that withstood heavy hurricanes but not seismic activities (Marshall et al. Citation2011). During the 2014 Orkney earthquake, Khoyratty (Citation2016) noted a pattern of damage patterns which were mainly: corner cracks, damage above windows and above door openings, falling plaster and diagonal cracks. In many cases there was an absence of confining elements, weak brick force and not enough mortar between the bricks, which contributed to the severity of damage observed ().
Most URM buildings were initially built without sufficient measures to withstand seismic events. As a result, these buildings have several features that make them vulnerable to seismic activities. The buildings in the Orkney region had majority of these features, as a result they were heavily damaged during the 2014 Orkney earthquake
1.4. Seismic vulnerability assessments methods
There are three main methods for seismic vulnerability assessments: analytical methods, empirical methods, and hybrid approaches (Shabani et al. Citation2021). The analytical method utilizes detailed structural parameters and seismic hazard or simulation tools to construct fragility curves. This method has been applied in several studies (Domaneschi et al. Citation2021; Marasco et al. Citation2021), including an assessment of the seismic vulnerability of existing school buildings in Northern Italy conducted by Domaneschi et al. (Citation2021). While this method is beneficial in situations where no damage data from past earthquakes is available, it necessitates seismic hazard calculation tools or well-established ground motion models (GMMs) for accurate hazard estimation. Unfortunately, these tools are often lacking in regions with less advanced seismic systems. Furthermore, generating analytical vulnerability curves is a time-intensive process that requires significant computational resources. Empirical methods utilize the correlation between seismic intensity and observed damage for seismic vulnerability assessment. This approach relies on damage data from past earthquakes, and it is further discussed in section 1.4.1. The hybrid approach involves integrating fragility curves obtained from the empirical method with the results from analytical methods (Yepes-Estrada et al. Citation2017; Yepes-Estrada and Silva, 2017; Kappos et al. Citation2006). This method is also employed for the calibration of fragility curves.
Both the analytical method and hybrid approach necessitate the calculation or simulation of seismic hazard for the investigated region. This calculation involves the use of GMMs. Notably, South Africa lacks its own GMMs, opting to adopt models from other regions. This practice introduces uncertainty into the seismic hazard calculation and, consequently, introduces uncertainty into the fragility curves. In contrast, the empirical method can be applied in developing regions that lack advanced technologies such as earthquake simulation tools and well-established GMMs. Therefore, in this study, the empirical method was employed for seismic vulnerability assessment.
1.4.1. Empirical seismic vulnerability assessments in URM buildings
Since seismic events may cause damage to structures which can be seen and assessed, the most appropriate methods to predict future damage are empirical. Empirical methods as opposed to simulation, are particularly suitable for non-engineering structures that are made up of materials of uncertain strength whose earthquake resistance is difficult to calculate. There are several methods for empirical seismic vulnerability assessment. These methods include rapid visual screening assessment methods, vulnerability index (VI), and damage probability matrixes (DPMs).
A rapid visual screening assessment procedure provides information about seismic vulnerability of a large number of buildings in a region. It is based on the correlations between the predicted seismic performance of the building and building structural typology, materials and design methods. The estimates of seismic performance are based on expert opinions (Alam and Haque Citation2020), pushover analyses (D'Altri et al. Citation2021), dynamic response studies (Chácara et al. Citation2018) and the performance of similar building types in past seismic events (Batalha et al. Citation2019). Since this method assesses a large number of buildings it can only assess clear and visible damage that can be seen from aerial imagery. When rapid visual screening calculates the probability of building collapse, it may not be suitable for calculating probabilities for lower levels of damage that cannot be seen from aerial images.
This method has been employed in various regions for seismic vulnerability assessments, such as Croatia (Pavić et al. Citation2020) and Turkey (Arkan et al. Citation2023). While it can offer a seismic vulnerability overview for a large number of buildings in a region, it lacks the specificity required for a detailed vulnerability assessment. Additionally, its application necessitates the availability of the exposure model for the study area (Arkan et al. Citation2023; Pavić et al. Citation2020). Given the limited progress in developing an exposure model for the Orkney region, this method may not be suitable for use in this study.
The vulnerability index measures the seismic behaviour of different building types. It ranges between 0 for the least vulnerable structures designed according to the most recent seismic design standards, and 1 for the most vulnerable structures. This method uses numerical analysis of the building structural typology to assess vulnerability and it is used when there is no observed past earthquake building damage data (Kassem et al. Citation2020). The vulnerability assessment of the building is carried out by finite element analysis and structural integrity calculations. This method has been successfully used by Ferreira et al. (Citation2019) to model seismic vulnerability of URM. It has also been employed to assess the seismic vulnerability of historic buildings in Croatia (Hadzima-Nyarko et al. Citation2017). It proves valuable for determining seismic vulnerability in cases where there is no information on the performance of buildings in past earthquakes. It is important to note that this method provides only an estimation and does not rely on real damage data.
The DPM method uses a matrix where each number expresses the probability that a building will experience a level of damage equal to or greater than a certain damage state index for a given Intensity Measure Level (IML) (Rosti et al. Citation2018). The DPM method uses damage observed from past earthquakes to determine vulnerability in terms of fragility curves or vulnerability functions (Biglari and Formisano Citation2020). The given damage state index (dsi) from past earthquakes are used to estimate the expected damage from future earthquakes. For this study fragility curves were used, which described the probability of a certain dsi being reached or exceeded for a specified IML. The results of this method are highly sensitive to the building typologies (Rosti et al. Citation2022). The procedure for this method involves assessment and preparation of damage data; selecting intensity measure type; selecting a statistical model for vulnerability or fragility curve; selecting and applying an appropriate statistical model fitting procedures and assessment of the model’s goodness of fit.
The DPM method is used when a seismic vulnerability assessment is conducted for detailed damage classes (Rosti et al. Citation2018) and can be used in any building type where the damage and intensity data is available (Rossetto et al. Citation2014). This method has been successfully used in several studies for seismic vulnerability assessment in URM buildings for example, after the 2017 Iran-Iraq earthquake that occurred along the in the Zagros fold and thrust belt (Biglari and Formisano., 2020), 1962 and 1969 Sarajevo and Banja Luka earthquakes (Ademović et al. Citation2020), and 2009 L’Aquila earthquake (Rosti et al. Citation2018).
1.5. Assessing post-earthquake damage data
The reliability of vulnerability and fragility curves depends strongly on the quality and quantity of observations of damage and intensity data. The quantity and quality of data depends in turn on the survey method; hence the reliability of the fragility curve depends on the survey method. There are four main survey methods for post-earthquake damage assessment, namely rapid surveys; detailed engineering surveys; surveys by reconnaissance teams and remotely sensed surveys. Rapid surveys are types of surveys for preliminary assessment of the usability of the buildings. These surveys are suitable for all building types and they result in large sample sizes, however, they have substantial errors and are considered less reliable (Lulić et al. Citation2021). Remotely sensed surveys use data such as aerial imagery to assess damage. This method is mostly suitable for all building types and results in a high sample size; however it is only able to capture clear and visible damage that can be seen in the imagery (Stone et al. Citation2018). Detailed engineering surveys and surveys by reconnaissance teams are reliable, relatively detailed and are suitable for all building types. The sample size from these surveys varies from small to large depending on the available resources, in most cases they result in small sample size (Rossetto et al. Citation2014). Following the 2014 Orkney earthquake, surveys by reconnaissance teams were used to investigate damage in buildings.
1.5.1. Orkney earthquake post event damage survey
In the aftermath of the 2014 Orkney earthquake, Khoyratty (Citation2016) conducted post-earthquake field assessments to investigate the extent of damage caused on low-cost unreinforced masonry (URM) buildings in the three townships in the Orkney region, namely Khuma, Kanana, and Jouberton. The survey was carried out by reconnaissance teams. As described in section 1.5, this method is considered relatively reliable and yields detailed damage data. However, it requires a significant amount of time and resources and may result in a low sample size in situations where resources are limited. In this case the team, consisting of five individuals, had only three days to survey over 3,000 hectares of land with over 75,000 buildings. Given the time and resource constraints, it was not feasible to survey all of the buildings in the area. As a result, the survey targeted only those buildings that had been listed in the municipality database as damaged. This limitation made the data somewhat unsuitable for constructing a reliable fragility curve. To construct a reliable empirical fragility curve, a complete dataset of all buildings in the sample area is necessary, regardless of whether they were damaged or undamaged. Hence in this study a first-order approximation method was applied to supplement the datasets, this was done by applying the first order approximation method to estimate the damage to buildings that had not been surveyed.
Approximations in science and engineering are employed whenever a numerical value, model or function is unknown or difficult to calculate. Various levels of approximations come with varying levels of precision and can be categorized according to the order of magnitude of the associated approximation errors. Four orders of approximation may be applied: zeroth order, first order, second order and higher order. A zeroth-order approximation signifies the initial scientific estimation. This preliminary estimate involves numerous simplifications, and when a numerical value is required, it is typically devoid of significant figures. A first-order approximation represents a more informed estimate in scientific contexts. This approach involves making certain simplifying assumptions, and when a numerical value is required, it is often provided with only one significant digit. A second-order approximation is scientific estimation that describe a relatively accurate answer. This approach involves making few assumptions and when a numerical value is required, the answer usually includes two or more significant figures. Higher order approximations are generally not categorized by numerical designations, but they are more advanced approximations that are vital for enhancing comprehension and portrayal of reality. In this study a first order approximation was used to estimate the damage, yielding a single significant digit estimation.
After the 2014 Orkney earthquake residents were urged by the councillors to report any damage to their buildings that might have occurred as the results of an earthquake. A total of 61 residents reported damage. All these buildings were government- built houses with typologies as described in Section 1.2. It was assumed that buildings in close proximity to the identified buildings were not damaged, and these were given a dsi of 0.
1.6. Selecting intensity measure type
Earthquake shaking can be defined in terms of Intensity measure levels (IMLs). Generally, intensity is highest near the epicentre and weakens with increasing distance from it. Several methods for measuring earthquake intensity are used. In regions with well advanced systems of seismic monitoring, the intensity can be expressed in terms of ground motion and predicted by the ground motion models (GMMs). In regions with less advanced systems of seismic monitoring, IMLs are estimated according to standardised scales that give discrete interval numbers to observations of the effect of ground motion on people, objects and buildings. There are several of these intensity scales. The most commonly used being Modified Mercalli Intensity (MMI) and the European Macroseismic Scale (EMS-98). The advantage of these scales is that they relate the felt intensity to observed damage. EMS-98 has been used in several studies, it was used for damage assessment after the 6 February 2023 Turkey earthquake (Işık et al. Citation2023)
2. Methodology
For this study the damage probability matrix (DMP) method was used for direct empirical fragility curve construction. The method consisted of four major steps. The first step was to prepare damage and intensity data and to select the damage and intensity measuring scale. The second step was to select the statistical model for the fragility curve; the third step was to select statistical model fitting techniques; and the last step was the assessment of the model’s goodness of fit.
2.1. Preparation of damage data and selecting intensity measure type
Direct fragility curves construction requires two main inputs, namely, intensity (explanatory variable) and damage (response variable). The damage data was collected from the surveys carried out following the 2014 Orkney earthquake. After the 2014 Orkney earthquake, buildings in the affected area were not repaired immediately. This delay allowed for the collection of accurate damage data. This contained a total of 61 buildings that were surveyed by a reconnaissance team. The data was collected in three townships namely, Khuma, Kanan and Jourberton. Information was gathered from residents about the damage to their properties. The overall data recorded included location of the investigated buildings, pictures, and descriptions of structural damage. The recorded structural damage was used to determine dsi of the buildings. Rossetto et al. (Citation2014) recommended a minimum of 100 data points to be used in the process of reliable fragility curve construction, this ensures that the fragility curve is reliable and the significance of the trend is justifiable. Hence in this study a first-order approximation method was used to supplement the data.
From the post-earthquake survey, it was reported that only buildings that were reported to be damaged were surveyed. This was a major shortcoming encountered while constructing the fragility curves. Therefore, a first-order approach was used to supplement the dataset. In this approach, it was assumed that the buildings within the same street of the damaged buildings that were not reported to be damaged to the municipality officials, did not experience any damage and so were given a dsi of 0 (see ). This was a reasonable assumption since the residents were urged to report to the municipality any damage that had been caused by the earthquake in their buildings. Moreover, nearby residents had a chance to report if their buildings experienced any damage to the surveying team during the field survey. The location of the buildings that were assumed to have no damage were collected from georeferenced orthophotographs with a maximum ground resolution of 30 cm and horizontal accuracy on the orthophotos of 5 m CE90. This accuracy was more than adequate for this application since the dimensions of the properties were 25 m x 15 m, hence a radius of 5 m will always fall within the boundaries of the property when the centre of the property is selected (see ). The data obtained from first-order approximation was then combined with the field data to prepare data for constructing the fragility curves. A total of 553 supposedly undamaged buildings were collected from orthophotographs, making up a total of 614 buildings to be used for fragility curve construction. The IML of all the buildings were extracted from the intensity map of the 2014 Orkney earthquake. The EMS-98 scale was used to measure damage and intensity (see and ). This scale is widely used in seismic vulnerability assessment of unreinforced masonry buildings, due to its implicit correlation between damage and felt intensity (Hill and Rossetto Citation2008). The damage data was then prepared for fragility curves construction by constructing binary responses, of 1 if the building had suffered damage equal to or greater than dsi and 0 if not. The final data for constructing fragility curves was then expressed the as IML with its binary response.
Table 1. EMS-98 abbreviated damage scale (Grünthal Citation1998).
Table 2. EMS-98 abbreviated intensity scale (Grünthal Citation1998).
2.2. Selection of statistical model and fitting procedures for fragility curve
Among the several statistical models for constructing fragility curves, Rossetto et al. (Citation2014) discussed three statistical models: Generalized Linear Model (GLM), Generalized Additive Models (GAM) and Gaussian Kernel Smoothers (GKSs). GLMs were used in this study since their models are well established and have strong predictive capacity. They have been used for fragility curve construction in various structures including buildings (Ioannou et al. Citation2012). The fitting technique used was the maximum likelihood method. GLMs together with the maximum likelihood method are considered suitable when the data is collected from a single seismic event (Rossetto et al. Citation2014).
Since building by building data was available, the Bernoulli distribution was applied to describe the GLM data. The Bernoulli distribution is a special case of the binomial distribution. The Bernoulli distribution can be linked with 3 functions: Logit (EquationEquation 1(1)
(1) ), Probit (EquationEquation 2
(2)
(2) ), and Cloglog (EquationEquation 3
(3)
(3) ). Fragility curves were constructed for all the link functions in R software package (R Core Team Citation2013). Assessment of the model’s goodness of fit was based on the Akaike information criterion (AIC).
(1)
(1)
(2)
(2)
(3)
(3)
where:
is the probability density function
is the mean damage for a given IML
is an intensity measure level of a data point i
if the building sustained a damage
and
if the building sustained damage
are model parameters corresponding to the damage state index (dsi)
3. Results and analysis
A total of 614 data points were collected, of which 61 related to houses with reported damage. The surveyed buildings had a dsi and IML that ranged from 0 to 5 and V to VIII respectively (see ). Most damaged buildings had a dsi of 2 and IML of VI, three buildings being totally destroyed. The intensity range for destroyed buildings is between VII and VIII (see and ). The buildings closer to the epicentre observed higher shaking and had higher damage (). There was a small correlation between IML and dsi as shown by smaller R-squared value (see ).
Figure 9. Shows relationship between earthquake intensity and damage state index (dsi) for buildings.
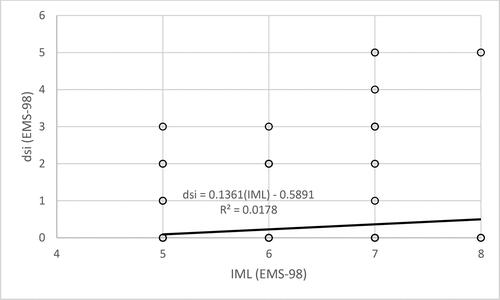
As detailed in the methodology section, fragility curves were constructed from mean damage per IML () and number of buildings reaching or exceeding a certain dsi per IML (). illustrates the relationship between IML and mean dsi. From this figure it is observed that an increasing IML resulted in increasing mean dsi. There was a strong correlation between these two variables, highlighted by the higher R squared value. The number of buildings reaching or exceeding a particular dsi was constructed from , this figure summarises the data points grouped according to IML and dsi. shows the cumulative number of damaged buildings. and were used in fragility curve construction. Curves were constructed for 3 link functions for all dsi: Logit, Probit and Cloglog link functions. was used to identify an optimum fragility curve based on the AIC value, as noted from this table, Cloglog had the best fit for ds1 to ds3, Logit had the best fit for ds4 and Probit had the best fit for ds5. The best fitting fragility curves are shown in . From this figure, probabilities of reaching or exceeding dsi were relatively low from IML 4 to 6 and was a sharp increase between IML 7 to 8. The probability of exceedance increased with increasing IML for all dsi. Maximum probabilities were observed at intensity 9 for all dsi. The probability of exceedance decreased with increasing dsi e.g. ds5 had the lowest probabilities for all IMLs and ds1 had the highest probabilities for all IMLs.
Figure 10. (a) Weighted mean damage state index per IML (b) summary of number of buildings reaching or exceeding dsi for all IML.
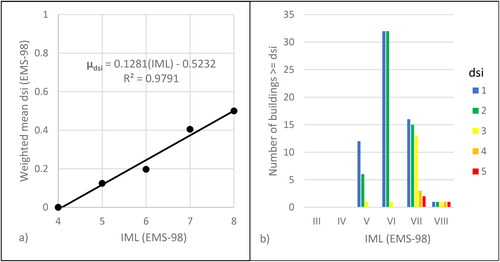
Table 3. The AIC value for all fragility curve for three link functions.
4. Discussion
The study predicted significantly higher damage of the URM buildings for higher ground shaking. Similar occurrence is observed in other published studies in different parts of the world. This can be explained by the fact that the stronger seismic shaking causes more displacement to the structural elements of the buildings, and this results in more damage to the rigid elements of the building. The predicted mean damage for each IML was somewhat lower compared to other studies conducted in URM buildings. Ademović et al. (Citation2020) obtained a mean damage of 1 for IML 7 on similar type of buildings in Banja Luka and Sarajevo. Biglari and Formisano (Citation2020) predicted a mean damage of 0.8 for IML 7 for similar class of buildings in Iran. In this study a mean damage of 0.4 for obtained for IML 7 (). This implies that buildings in the study area experienced less damage for similar levels of seismic shaking compared to similar buildings in other regions. The reason for the lower damage value might be bias due to most buildings in the study being assumed to have suffered no damage. Other reasons might include local building construction materials and local geological effects. Most buildings are constructed from earth materials such as soils and rocks, and the specific materials used can vary depending on the region and local geology, the buildings in this study were constructed from 140 mm, 3.5 mPa concrete blocks with cement mortar joints. Buildings in the Biglari and Formisano (Citation2020) study were constructed from 10 mPa sand lime bricks with cement mortar. When considering the building materials, the buildings in this study were more vulnerable than buildings assessed by Biglari and Formisano (Citation2020).
Local geological conditions can also have a significant effect on the damage of structures during earthquakes. The ground motion during an earthquake is influenced by the characteristics of the local geology, which can amplify or reduce the seismic waves as they travel through the earth. In the study area, Mulabisana et al. (Citation2019) reported significant site amplifications during the 2014 Orkney earthquake.
The fragility curves were constructed for all damage states for higher intensity measure levels (IV to VIII), since the study area was relatively close to the epicenter. From the constructed curves, the probability of exceedance increased with increasing seismic intensity for all damage states and increased with decreasing dsi. This was also observed in fragility curves constructed by Ademović et al. (Citation2020) and Biglari and Formisano (Citation2020). The reason for this observation is that buildings experiencing higher intensity are more likely to be damaged, and smaller damage is more likely to occur than higher damage. This study found a slightly lower probability of exceedance for all dsi compared to the study published by Biglari and Formisano (Citation2020). At intensity 7, Biglari and Formisano (Citation2020) published probability of 0.45 for ds1 while in this study ds1 has a probability of 0.3, this was due to this study having lower mean damage compared to the study by Biglari and Formisano (Citation2020).
South Africa has more three million low-cost houses that are provided by the government for low income citizen. In addition, majority of South Africans in townships and rural areas reside in unreinforced masonry buildings. The results of this study indicate that buildings closer to the epicenter are more likely to experience higher intensity and greater damage. This information can inform future developments of low-cost unreinforced masonry (URM) buildings, ensuring they are situated farther from potential seismic sources. The findings reveal potential intensity levels (IMLs) more likely to cause damage, and this information can be incorporated into design codes to ensure approved building designs can withstand specific seismic intensities. The obtained results can also assist disaster response teams in estimating damage to URM buildings and the effect of this damage after an earthquake or in assessing the seismic risks associated with these structures (Domaneschi et al. Citation2019).
These results can also aid in identifying existing buildings that require retrofitting, typically those exposed to higher intensity during seismic events. As mentioned in the introduction section, buildings in the study area are vulnerable to seismic activities and face elevated seismic hazards. Therefore, it is advisable for these structures to undergo retrofitting to mitigate their vulnerability to earthquake damage. Various retrofitting methods exist, and the choice depends on the type of unreinforced masonry (URM) buildings. Some well-researched reinforcement methods include wall reinforcement (Dadras Eslamlou et al. Citation2019), addition of confining elements (Borah et al. Citation2023; Gkournelos et al. Citation2022), stiffening of roof and floor framing (Senaldi et al. Citation2014), incorporation of base isolation systems (D'Amato et al. Citation2019; Nakamura and Okada Citation2019), and soil improvements (Kempfert and Raithel Citation2005). As highlighted in section 1.3, the predominant damage observed in buildings after the 2014 Orkney earthquake was on the walls. Therefore, the most suitable retrofitting method involves reinforcing these walls. Masonry wall reinforcement has demonstrated a 1.5 times improvement in a building’s resistance to seismic loading (Borah et al. Citation2023). Various methods are available for reinforcing masonry walls, including adding plastering, epoxy injection, buttressing, and incorporating external steel or fibre-based reinforcements (Gkournelos et al. Citation2022).
In this study the investigated buildings were assumed to have similar vulnerability to earthquake damage, since they had similar structural typology. However, even though the buildings originally had similar typology, some residents had made renovations in them. These renovations can strength or weaken the buildings, for example, adding a door or a window to the building, will weaken the building, while plastering the building will strengthen it. First-order approximation was used on buildings that were not surveyed, this was done by assuming that these buildings had no damage, since they were not reported by the residents and the damage was not seen from orthophotos. However, it might happen that the was damage but the residents did not find it significant to report it. Also damage that ranges from ds1 to ds4 cannot be seen from orthophotos, hence this may result in unreliable fragility curves for these damage states if indeed there was some damage to these buildings. It is evident that these buildings did not reach ds5 because ds5 could be seen from orthophotos, and from the available orthophotos that were taken after the 2014 Orkney earthquake the buildings look like they have no damage, hence the approximation made in this study is best applicable for constructing reliable fragility curve for ds5.
5. Conclusion and recommendations
The Orkney area is susceptible to medium seismic activities, linked with the extensive mining activities. The 2014 Orkney earthquake resulted in damage to a number of buildings. A total of 61 buildings were reported to be damaged, with damage ranging from minor damage to total collapse, most damaged buildings had moderate damage, and three buildings being totally destroyed. The earthquake underlined the deficiencies of current URM buildings in the area. Several factors contributed to the worsening of damage, these included absence of confining elements, weak brick force and not enough mortar between the bricks. The shaking caused by the event was stronger closer to the epicenter. Damage was also worse closer to the epicentre, where a total of 3 buildings collapsed.
In this study, data from a post-earthquake damage survey conducted after the 2014 Orkney earthquake was used for empirical vulnerability assessment. However, due to the small sample size of the data, a first-order approximation was used to increase the sample size. This was achieved by assuming that all buildings that were not reported to be damaged had no damage. A total of 553 buildings were collected using this method and these buildings were assumed to be undamaged. In total 614 buildings were used for fragility curve construction.
Empirical fragility curves were constructed using the DPM method. The newly derived fragility curves predicted somewhat lower damage levels compared to other studies. The lower predicted damage levels were attributed to most buildings in the study area having been assumed to have had no damage, which significantly reduced the mean damage and resulted in lower predicted damages. The method used in this study enabled relatively reliable fragility curves to be constructed using data that would have been unusable for empirical fragility curve construction. This would be useful in constructing empirical fragility curves using data from past earthquake where there were large sampling errors as was the case in this study. However, the method used was only possible given the availability of remotely sensed data like orthophotos, and an intensity map of the event.
In order to improve the fragility curves, it is recommended that future post-earthquake damage surveys assess all buildings in the study area, where possible. Alternatively, where there is limited amount of time and resources, a smaller sample size can be taken and all buildings in the sample should be assessed. It is also recommended to employ an analytical approach for constructing fragility curves in the same type of buildings so as to validate the newly constructed curves. The advantage of analytical seismic vulnerability assessment is that it uses structural properties to construct fragility curves and is not affected by additional factors such as local site effects and building vulnerability. Such additional factors also need to be investigated to obtain a holistic understanding of damage trends.
Disclosure statement
No potential conflict of interest was reported by the author(s).
Author contributions
Thando Nqasha and Mayshree Singh contributed to the study conception and design. The first draft of the manuscript was written by Thando Nqasha and all authors commented on previous versions of the manuscript. All authors have read and approved the final manuscript.
Data availability statement
The datasets generated during and/or analysed during the current study can be available from the corresponding author on reasonable request upon agreement with the institution.
Additional information
Funding
References
- Ademović N, Hadzima-Nyarko M, Zagora N. 2020. Seismic vulnerability assessment of masonry buildings in Banja Luka and Sarajevo (Bosnia and Herzegovina) using the macroseismic model. Bull Earthquake Eng. 18(8):3897–3933. doi: 10.1007/s10518-020-00846-8.
- Alam MS, Haque SM. 2020. Seismic vulnerability evaluation of educational buildings of Mymensingh city, Bangladesh using rapid visual screening and index based approach. IJDRBE. 11(3):379–402. doi: 10.1108/IJDRBE-07-2019-0043.
- Arkan E, Işık E, Harirchian E, Topçubaşı M, Avcil F. 2023. Architectural characteristics and determination seismic risk priorities of traditional masonry structures: a case study for Bitlis (Eastern Türkiye). Buildings. 13(4):1042. doi: 10.3390/buildings13041042.
- Batalha N, Rodrigues H, Varum H. 2019. Seismic performance of RC precast industrial buildings—learning with the past earthquakes. Innov Infrastruct Solut. 4(1):1–13. doi: 10.1007/s41062-018-0191-y.
- Bhattacharya S,Nayak S,Dutta SC. 2014. A critical review of retrofitting methods for unreinforced masonry structures. Int J Disaster Risk Reduct. 7:51–67. doi: 10.1016/j.ijdrr.2013.12.004.
- Biglari M, Formisano A. 2020. Damage probability matrices and empirical fragility curves from damage data on masonry buildings after Sarpol-e-zahab and Bam Earthquakes of Iran. Front Built Environ. 6:2. doi: 10.3389/fbuil.2020.00002.
- Bilgin H, Shkodrani N, Hysenlliu M, Ozmen HB, Isik E, Harirchian E. 2022. Damage and performance evaluation of masonry buildings constructed in 1970s during the 2019 Albania earthquakes. Eng Fail Anal. 131:105824. doi: 10.1016/j.engfailanal.2021.105824.
- Borah B, Kaushik HB, Singhal V. 2023. Analysis and design of confined masonry structures: review and future research directions. Buildings. 13(5):1282. doi: 10.3390/buildings13051282.
- Borcherdt RD. 1970. Effects of local geology on ground motion near San Francisco Bay. Bull Seismol Soc Am. 60(1):29–61.
- Brandt M. 2011. Seismic Hazard in South Africa. Counc Geosci Rep. 61:1–22.
- Bruneau M. 1994. State-of-the-art report on seismic performance of unreinforced masonry buildings. J Struct Eng. 120(1):230–251. doi: 10.1061/(ASCE)0733-9445(1994)120:1(230).
- Chácara C, Cannizzaro F, Pantò B, Caliò I, Lourenço PB. 2018. Assessment of the dynamic response of unreinforced masonry structures using a macroelement modeling approach. Earthq Engng Struct Dyn. 47(12):2426–2446. doi: 10.1002/eqe.3091.
- Dadras Eslamlou S, Masia MJ, Totoev YZ, Page AW. 2019. Effect of retrofitting on the structural factors for seismic assessment of unreinforced masonry structures: a review. Aust J Struct Eng. 20(1):26–53. doi: 10.1080/13287982.2019.1565288.
- D'Altri AM, Presti NL, Grillanda N, Castellazzi G, de Miranda S, Milani G. 2021. A two-step automated procedure based on adaptive limit and pushover analyses for the seismic assessment of masonry structures. Comput Struct. 252:106561. doi: 10.1016/j.compstruc.2021.106561.
- D'Amato M, Gigliotti R, Laguardia R. 2019. Seismic isolation for protecting historical buildings: a case study. Front Built Environ. 5:87. doi: 10.3389/fbuil.2019.00087.
- Doğangün A, Ural A, Livaoğlu R. 2008. Seismic performance of masonry buildings during recent earthquakes in Turkey. The 14thWorld Conference on Earthquake Engineering October . p. 12 – 17.
- Domaneschi M, Cimellaro GP, Scutiero G. 2019. A simplified method to assess generation of seismic debris for masonry structures. Eng Struct. 186:306–320. doi: 10.1016/j.engstruct.2019.01.092.
- Domaneschi M, Noori AZ, Pietropinto MV, Cimellaro GP. 2021. Seismic vulnerability assessment of existing school buildings. Comput. Struct. 248:106522. doi: 10.1016/j.compstruc.2021.106522.
- Doughton S, Raghavendran B. 2015. Seattle’s old brick buildings could see huge damage in big quake, The Seattle Times, 10 August 2015, viewed 11 May 2023. https://www.seattletimes.com/business/real-estate/citys-old-brick-buildings-could-see-huge-damage-in-bigquake/#:∼:text=Preliminary%20surveys%20in%202007%20and,spend%20time%20in%20those%20buildings.
- Erdik M, Tümsa MBD, Pınar A, Altunel E, Zülfikar AC. 2023. A preliminary report on the February 6, 2023 earthquakes in Türkiye. Temblor. https://temblor.net/temblor/preliminary-report-2023-turkey-earthquakes-15027/
- Ferreira TM, Mendes N, Silva R. 2019. Multiscale seismic vulnerability assessment and retrofit of existing masonry buildings. Buildings. 9(4):91. doi: 10.3390/buildings9040091.
- Gkournelos PD, Triantafillou TC, Bournas DA. 2022. Seismic upgrading of existing masonry structures: a state-of-the-art review. Soil Dyn Earthquake Eng. 161:107428. doi: 10.1016/j.soildyn.2022.107428.
- Grünthal G. (1998). European macroseismic scale 1998 (EMS-98). European Seismological Commission. 15:1–99.
- Hadzima-Nyarko M, Mišetić V, Morić D. 2017. Seismic vulnerability assessment of an old historical masonry building in Osijek, Croatia, using Damage Index. J Cult Heritage. 28:140–150. doi: 10.1016/j.culher.2017.05.012.
- Halder L, Dutta SC, Sharma RP. 2020. Damage study and seismic vulnerability assessment of existing masonry buildings in Northeast India. J Build Eng. 29:101190. doi: 10.1016/j.jobe.2020.101190.
- Hill MP, Rossetto T. 2008. Do existing damage scales meet the needs of seismic loss estimation. Bull Earthquake Eng. 6(2):335–365. doi: 10.1007/s10518-007-9057-y.
- Ioannou I, Rossetto T, Grant DN. 2012, September. Use of regression analysis for the construction of empirical fragility curves. Proceedings of the Fifteenth World Conference on Earthquake Engineering, Lisbon, Portugal . p. 24 – 28.
- Işık E. 2023. Structural failures of adobe buildings during the February 2023 Kahramanmaraş (Türkiye) earthquakes. Appl Sci. 13(15):8937. doi: 10.3390/app13158937.
- Işık E, Avcil F, Büyüksaraç A, İzol R, Hakan Arslan M, Aksoylu C, Harirchian E, Eyisüren O, Arkan E, Şakir Güngür M, et al. 2023. Structural damages in masonry buildings in Adıyaman during the Kahramanmaraş (Turkiye) earthquakes (Mw 7.7 and Mw 7.6) on 06 February 2023. Eng Fail Anal. 151:107405. doi: 10.1016/j.engfailanal.2023.107405.
- Işık E, Shendkar MR, Avcil F, Büyüksaraç A, Deshpande SS. 2023. A study on the determination of damage levels in reinforced concrete structures during the Kahramanmaraş Earthquake on February 06, 2023. E3S Web of Conferences . Vol. 405 . EDP Sciences. p. 04029. doi: 10.1051/e3sconf/202340504029.
- Jafari S, Rots JG, Esposito R, Messali F. 2017. Characterizing the material properties of Dutch unreinforced masonry. Procedia Eng. 193:250–257. doi: 10.1016/j.proeng.2017.06.211.
- Jogunoori P. 2012. Analysis of deaths caused by interplate and intraplate earthquakes [doctoral dissertation]. Texas A & M University.
- Kappos AJ, Panagopoulos G, Panagiotopoulos C, Penelis G. 2006. A hybrid method for the vulnerability assessment of R/C and URM buildings. Bull Earthquake Eng. 4(4):391–413. doi: 10.1007/s10518-006-9023-0.
- Kassem MM, Nazri FM, Farsangi EN. 2020. The seismic vulnerability assessment methodologies: a state-of-the-art review. Ain Shams Eng J. 11(4):849–864. doi: 10.1016/j.asej.2020.04.001.
- Kempfert HG, Raithel M. 2005. Soil improvement and foundation systems with encased columns and reinforced bearing layers. In Elsevier geo-engineering book series. Vol. 3. Kassel: Elsevier. p. 923–946.
- Khoyratty Z. 2016. Seismic vulnerability investigation of RDP homes in the city of Matlosana, North West province, South Africa [Degree of MSc]. University of KwaZuluu-Natal.
- Kiyono J, Kalantari A. 2004. Collapse mechanism of adobe and masonry structures during the 2003 Iran Bam earthquake. Bull Earthq Res Inst Univ Tokyo. 79(157):161.
- Lulić L, Ožić K, Kišiček T, Hafner I, Stepinac M. 2021. Post-earthquake damage assessment—case study of the educational building after the Zagreb Earthquake. Sustainability. 13(11):6353. doi: 10.3390/su13116353.
- Manzunzu B, Midzi V, Mangongolo A, Essrich F. 2017. The aftershock sequence of the 5 August 2014 Orkney earthquake (ML 5.5), South Africa. J Seismol. 21(6):1323–1334. doi: 10.1007/s10950-017-9667-z.
- Marasco S, Noori AZ, Domaneschi M, Cimellaro GP. 2021. A computational framework for large-scale seismic simulations of residential building stock. Eng Struct. 244:112690. doi: 10.1016/j.engstruct.2021.112690.
- Marshall JD, Lang AF, Baldridge SM, Popp DR. 2011. Recipe for disaster: construction methods, materials, and building performance in the January 2010 Haiti earthquake. Earthquake Spectra. 27(1_suppl1):323–343. doi: 10.1193/1.3637031.
- Menegon SJ, Tsang HH, Lumantarna E, Lam NTK, Wilson JL, Gad EF. 2019. Framework for seismic vulnerability assessment of reinforced concrete buildings in Australia. Aust J Struct Eng. 20(2):143–158. doi: 10.1080/13287982.2019.1611034.
- Mulabisana T, Midzi V, Manzunzu B. 2019. Local site effects during the Orkney earthquake of 5 August 2014. Pure Appl Geophys. 176(1):147–164. doi: 10.1007/s00024-018-1959-x.
- Nakamura Y, Okada K. 2019. Review on seismic isolation and response control methods of buildings in Japan. Geoenviron Disasters. 6(1):1–10. doi: 10.1186/s40677-019-0123-y.
- Ozbulut OE. 2023. Buildings left standing in Turkey offer design guidance for future earthquake-resilient construction, The Conversation. [accessed 2023 Sep 4]. Available from: https://theconversation.com/buildings-left-standing-in-turkey-offer-design-guidance-for-future-earthquake-resilient-construction-202089#:∼:text=Much%20of%20the%20damage%20in,reinforcing%20bars%20at%20critical%20locations.
- Panzera F, Lombardo G, Imposa S, Grassi S, Gresta S, Catalano S, Romagnoli G, Tortorici G, Patti F, Di Maio E. 2018. Correlation between earthquake damage and seismic site effects: the study case of Lentini and Carlentini, Italy. Eng Geol. 240:149–162. doi: 10.1016/j.enggeo.2018.04.014.
- Pavić G, Hadzima-Nyarko M, Bulajić B, Jurković Ž. 2020. Development of seismic vulnerability and exposure models—a case study of Croatia. Sustainability. 12(3):973. doi: 10.3390/su12030973.
- Perbix TW, Burke P. 1989. Toward a philosophy for seismic retrofit: the Seattle experience. Earthquake Spectra. 5(3):557–569. doi: 10.1193/1.1585540.
- Pule T, Fourie CJS, Kijko A, Midzi V. 2015. Comparison and quantitative study of vulnerability/damage curves in South Africa. South Afr J Geol. 118(4):335–354. doi: 10.2113/gssajg.118.4.335.
- R Core Team. 2013. R: a language and environment for statistical computing. Vienna, Austria: R Foundation for Statistical Computing. http://www.R-project.org/.
- Rossetto T, Ioannou I, Grant DN, Maqsood T. 2014. Guidelines for the empirical vulnerability assessment, GEM Technical Report 2014-08 V1.0.0, 140 pp., GEM Foundation, Pavia, Italy, doi: 10.13117/GEM.VULN-MOD.TR2014.11.
- Rosti A, Rota M, Penna A. 2018. Damage classification and derivation of damage probability matrices from L’Aquila (2009) post-earthquake survey data. Bull Earthquake Eng. 16(9):3687–3720. doi: 10.1007/s10518-018-0352-6.
- Rosti A, Rota M, Penna A. 2022. An empirical seismic vulnerability model. Bull Earthquake Eng. 20(8):4147–4173. doi: 10.1007/s10518-022-01374-3
- Sorrentino L, Cattari S, Da Porto F, Magenes G, Penna A. 2019. Seismic behaviour of ordinary masonry buildings during the 2016 central Italy earthquakes. Bull Earthquake Eng. 17(10):5583–5607. doi: 10.1007/s10518-018-0370-4.
- Senaldi I, Magenes G, Penna A, Galasco A, Rota M. 2014. The effect of stiffened floor and roof diaphragms on the experimental seismic response of a full-scale unreinforced stone masonry building. J Earthquake Eng. 18(3):407–443. doi: 10.1080/13632469.2013.876946.
- Shabani A, Kioumarsi M, Zucconi M. 2021. State of the art of simplified analytical methods for seismic vulnerability assessment of unreinforced masonry buildings. Eng Struct. 239:112280. doi: 10.1016/j.engstruct.2021.112280.
- Sinha R, Brzev SN. 2002. Housing report: unreinforced brick masonry building with reinforced concrete roof slab. Delhi: World Housing Encyclopedia.
- Stone H, Putrino V, D'Ayala D. 2018. Earthquake damage data collection using omnidirectional imagery. Front Built Environ. 4:51. doi: 10.3389/fbuil.2018.00051.
- Yepes-Estrada C, Silva V. 2017, January. Probabilistic seismic risk assessment of the residential building stock in South America. In 16th World Conference on Earthquake Engineering.
- Yepes-Estrada C, Silva V, Valcárcel J, Acevedo AB, Tarque N, Hube MA, Coronel G, María HS. 2017. Modeling the residential building inventory in South America for seismic risk assessment. Earthquake Spectra. 33(1):299–322. doi: 10.1193/101915eqs155dp.
- Yön B. 2021. Identification of failure mechanisms in existing unreinforced masonry buildings in rural areas after April 4, 2019 earthquake in Turkey. J Build Eng. 43:102586. doi: 10.1016/j.jobe.2021.102586.